DOI:
10.1039/D5RA01280E
(Review Article)
RSC Adv., 2025,
15, 11617-11638
Excavating medicinal virtues of chalcones to illuminate a new scope in cancer chemotherapy
Received
21st February 2025
, Accepted 31st March 2025
First published on 14th April 2025
Abstract
A common simple scaffold, 1,3-diaryl-2-propen-1-one (also known as chalcone), is present in many important natural products. This scaffold is the backbone of several flavonoids and isoflavonoids. Owing to the simple approachable synthetic strategies and the rigid backbone of chalcone and its derivatives, they have captivated the attention of researchers for several decades. Chalcone and its derivatives possess strong biological activities, viz. anti-tumor activity and anticancer potency. Accordingly, the in vitro and in vivo anticancer activities of several chalcone-based compounds with strong anticancer potential have been identified, which relies on mechanisms such as cell cycle arrest, regulation of autophagy, and induction of apoptosis. Therefore, researchers have successfully introduced chalcone derivatives to achieve enhanced anticancer activity in traditional chemotherapy. Nevertheless, in-depth research on this highly impactful scaffold is lacking to date. Thus, in this review, we shed light on the recent advances in the relatively unexplored scaffolds of chalcone and its derivatives, which have substantial anticancer activities. Furthermore, future perspectives of the mushrooming research on chalcones as potential anticancer agents (therapeutics) are emphasized.
 Nivedya T. | Nivedya T. received her Bachelor of Science degree from S. N. College, Kannur, University of Kannur, India, in 2018 and Master of Science degree in Chemistry from Pondicherry University, India, in 2021. She is currently pursuing her PhD under the guidance of Dr Ashok Kumar S. K and co-guidance of Dr Priyankar Paira at Vellore Institute of Technology, Vellore, India. Her research topics mainly involve “Synthesis, characterization and evaluation of various arene-N^N heterocycles and their Ru(II)/Ir(III) complexes on anticancer treatment and sensing”. |
 Nilmadhab Roy | Nilmadhab Roy completed his Bachelor of Science (BSc) degree in Chemistry (Honours) from Vidyasagar University. Then, he received his Master of Science (MSc) degree from the same university in 2014, with specialization in Physical Chemistry. In 2015, he was qualified for the All India GATE examination. This time, he was highly interested in anticancer research, and he wanted to pursue research in the field of Bioinorganic Chemistry. Thus, he joined a PhD programme as a Junior Research Fellow in 2018 under the supervision of Dr Priyankar Paira, MRSC, Associate Professor, Department of Chemistry, Vellore Institute of Technology (VIT), India. There, he focused on the development of various anticancer organometallic drugs by finding their cancer cell death pathways. He is now a Senior Research Scholar and is in the stage of submitting his PhD thesis. His research interest involves “Development of mitochondria targeting Ru(II)/Ir(III)/Re(I)-based half-sandwich and cyclometallated complexes for chemodynamic therapy”. Currently, he is a member of the Society of Biological Inorganic Chemistry (SBIC). He has 27 publications in various ACS, RSC and Elsevier journals with 501 citations and an h-index of 13. |
 Priyankar Paira | Dr Priyankar Paira, MRSC, Associate Professor, Vellore Institute of Technology (VIT), Vellore, Tamil Nadu, India, received his PhD from the Indian Institute of Chemical Biology (IICB), Jadavpur University, Kolkata and his Post Doctorate degree from the National University of Singapore (NUS). He is a Bioinorganic and Medicinal Chemist with 12 years of experience. He has over 100 publications in reputed international journals having citations (2600) and h-index (29) because of his significant contribution in research. He also has 3 patents in Medicinal Chemistry. He has successfully completed the DST-SERB-YS (YSS/2014/000842) project, DST-SERB project (EMR/2017/000816), DST-SERB-CRG project (CRG/2021/002267), and two ICMR project awards. At present, he is working with CSIR-EMR-II project. In particular, he has always been fascinated by research involving combination of Engineering, Medicine and Science, as demonstrated by his publications and collaborations with a number of people from different Institutes having diverse research expertise. He is associated with professional bodies like ACS, RSC and SBIC. He is associated as a reviewer with several RSC, ACS, Willey and Elsevier journals. He is also the Editorial Board Member of Current Electrocatalysis, Bentham Science and Frontiers in Chemistry Journals. |
 Rinku Chakrabarty | Dr Rinku Chakrabarty embarked on her academic journey at Kalyani University, West Bengal, India, followed by her PhD under the guidance of Prof. Shyamaprosad Goswami from the Indian Institute of Engineering Science and Technology, Shibpur, India in 2011, focusing on molecular recognition and supramolecular chemistry. She spent two years at the University of Witwatersrand, Johannesburg, South Africa, for her postdoctoral studies, where she was involved in the synthesis of rare earth-based molecular systems and their extensive application as MRI agents. Her passion for academia led her to assume the role of an Assistant Professor in the Department of Chemistry at Alipurduar University. Her published works encompass an impressive collection of research papers. Her research pursuits are centered on nanomaterials, dye degradation, molecular sensing and bioinorganic chemistry, showcasing her unwavering commitment to the advancement of the field of chemistry. |
Introduction
Cancer is considered the second leading cause of morbidity and mortality after cardiovascular diseases. It affects the lives of millions of people worldwide.1 Thus, several research groups have devoted significant efforts to the development of new strategies for the treatment of various types of cancer using novel therapeutic agents. It is of keen interest to scientists worldwide to explore new classes of compounds having anticancer potency. Cancer is mainly caused by the uncontrolled growth of abnormal cells. Environmental and genetic issues affect the growth of cancer within the human body. Factors such as smoking, obesity, excessive consumption of alcohol, lack of physical exercise, and dietary habits contribute to the growth of cancer.2 Cancer can be identified in many ways, including symptoms, signs, and screening tests, and can be treated using techniques such as surgery, radiation therapy or the widely used chemotherapy.3 Among them, chemotherapy is the most effective and is being applied worldwide despite its tremendous side effects. Chemotherapeutic agents destroy the normal cells along with the cancer cells. Nature is a treasure, which contains surplus of compounds having potent biological activities. Some important anticancer drugs, such as taxanes and epipodophyllotoxins, are extracted from nature and are proven to possess strong anticancer potency. Flavonoids, which are found in spices, fruits, vegetables, and nuts, are heterogeneous compounds and are recognized as an important class of naturally occurring compound having significant anticancer potency.4 They are polyphenolic compounds with high thermal stability. Epidemiological studies have proven that sufficient intake of flavonoids reduces the risk of tumor growth, thereby reducing the risk of cancer.5 Interestingly, the important scaffold present in flavonoids is chalcone. It acts as an intermediate for the biosynthesis of flavonoids. Furthermore, its additional features of structural heterogeneity and structural simplicity make it an attractive candidate to scientists as an ideal chemotherapeutic agent, hence attracting attention from many research groups.6,7 Chalcones are well-known for their important biological applications, viz. antioxidant, antidiabetic, anti-inflammatory, cancer chemopreventive, antileishmanial, antimalarial, and antimicrobial properties.8–13 Many chalcone-based compounds, such as metochalcone, sofalcone, and hesperidine methylchalcone,14–17 have been clinically tested and approved for commercial use (Fig. 1).
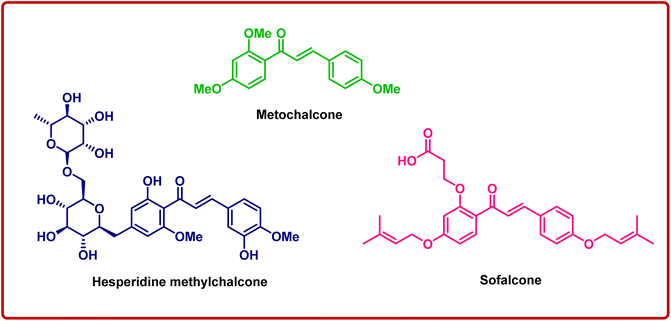 |
| Fig. 1 Structures of a few clinically implemented chalcones. | |
The chemically obtained chalcone is the 1,3-diaryl-2-propen-1-on scaffold (Fig. 2), which can be conveniently and easily modified by adding different functional groups, e.g. –OH, –Ar, –X, –CO, and –Ph, to enhance its biological properties. It has been observed that chalcone derivatives exhibit a considerably broad spectrum of biological activities. The core structure of chalcones contains an α,β-unsaturated carbonyl group.18 This α, β-unsaturated motif is a well-known Michael acceptor and is prone to undergo nucleophilic addition reactions.19 Due to the presence of a double bond in their structure, chalcones can exist in two isomeric forms, cis and trans, where trans is the more thermodynamically stable and dominant form over the corresponding cis isomer.20–22 Their uniqueness lies in their tolerance towards different biological molecules and active binding with specific molecules. Another feature is their modification. Chalcone moieties can be hybridized with important pharmacophores, e.g. imidazole, benzothiazole, indole, coumarin, and azole, to augment their efficiencies, thereby improving their drug resistance properties and therapeutic specificity for application as novel anticancer agents.
 |
| Fig. 2 General structure of chalcone. | |
The classical synthesis of chalcone involves the Claisen–Schmidt23 condensation reaction, which is an aldolization–crotonization reaction of acetophenone and benzaldehyde under strongly alkaline or acidic catalytic homogeneous conditions.24–27 Many modified pathways, e.g., Heck coupling, Suzuki coupling, Friedel–Crafts reaction, Wittig reaction, Sonogashira coupling reaction, and Julia–Kocienski reaction,28 can effectively produce chalcones (Fig. 3).
 |
| Fig. 3 Schematic of the preparation of chalcones. | |
The synthesis of chalcones in alkaline medium29 is more effective compared to using acidic medium. The Claisen–Schmidt condensation reaction in alkaline medium involves the formation of the corresponding anion of acetophenone, followed by the attack of the carbonyl group under slightly heating conditions with medium yield.30 However, this method has several disadvantages including long reaction time, harsh reaction conditions, and difficulties in product isolation and catalyst recovery.31 To address these issues, modification in methods have been carried out by replacing homogeneous catalysts with heterogeneous catalysts. To solve the selectivity issue, Lewis acids, Brønsted acids or solid acids or bases have been utilized in the modified method. The use of these catalysts reduces the possibility of reactions such as Cannizzaro reaction and Michael condensation reaction.32 Other condensation reactions, e.g., Heck coupling reaction, Sonogashira reaction, photo-Fries rearrangement, and Suzuki coupling reaction are also very effective for the synthesis of chalcones.33
Chalcone and its derivatives show significant in vitro and in vivo activity against therapy-resistant cancer and are susceptible to different cancer-targeting proteins and factors, e.g., breast cancer resistant protein and mesenchymal epithelial transition factor (MET).34–36 Chalcones play an important role in inhibiting the assembly of microtubules, which are responsible for the maintenance of the function and shape of cells during mitosis. Moreover, chalcone and its derivatives exhibit anticancer potency through different mechanisms such as regulating autophagy, induction of apoptosis, and inflammatory and immunomodulatory responses (Fig. 4).37
 |
| Fig. 4 Different mechanisms of action of chalcone. | |
Natural chalcones as cancer palliative agents
Decades of research on natural compounds unveiled the existence of chalcones as the core moieties of various biologically important compounds, remaining mostly in monomeric form with a considerably wide range of structural diversity. The compounds belonging to the chalcone family can be broadly categorised as follows: (1) classical or simple chalcones and (2) hybrid chalcones bearing the 1,3-diaryl-2-propen-1-one core framework. The structural diversity of these chalcones originates from the number and position of the substituents present in their framework and they are isolated from the various natural sources including roots, buds, leaves, seeds, flowers, and rhizomes of species of Dorstenia, Butea, Glycyrrhiza, Angelica, Desmodium, Helichrysum, Morus, Parartocarpus, Sophora, Neoraputia, Ficus, Humulus, Scutellaria, Tarenna, Calotropis, and Artocarpus genera. Commonly, chalcone-containing herbs have been used as medicines for the treatment of numerous diseases to date.38,39 Some naturally isolated important chalcones such as butein, isoliquiritigenin, and isobavachalcone (Fig. 5) exhibit effective anticancer activities, which are explicitly delineated in this section.
 |
| Fig. 5 Structures of naturally obtained butein, isoliquiritigenin, and isobavachalcone. | |
Butein (3,4,2′,4′-tetrahydroxychalcone) is a biologically important flavonoid of the chalcone family, which is isolated from several plants, such as Butea monosperma, Rhus verniciflua, Semecarpus anacardium and Caragana jubata. It is potentially used as traditional medicine to treat various types of ailments such as inflammatory diseases, cough, atherosclerosis and cancer.40 Butein exhibited antitumor activity against a variety of human tumor cells, including colon carcinoma, osteosarcoma, breast carcinoma, hepatocarcinoma, and lymphoma. Butein inhibits cancer cell growth by inducing G2/M phase arrest and apoptosis. Butein-induced G2/M phase arrest is associated with increased phosphorylation of the ataxia telangiectasia mutated (ATM) and Chk1/2 genes, and consequently with reduced cdc25C levels. In addition, butein-induced apoptosis is mediated through the activation of caspase-3, which is associated with changes in the expression of Bcl-2 and Bax proteins. Intriguingly, butein sensitizes cells to tumor necrosis factor-related ligand-induced apoptosis via ERK-mediated Sp1 activation, which promotes the transcription of specific death receptor 5. Butein also inhibits the migration and invasion of human cancer cells by suppressing nuclear factor-κB (Nf-κB) and extracellular signal-regulated kinase 1/2-mediated expression of matrix metalloproteinase-9 and vascular endothelial growth factor (VEGF). Additionally, butein downregulates the expression of human telomerase reverse transcriptase and causes a concomitant decrease in telomerase activity. Butein exhibits anticarcinogenic property towards non-small cell lung cancer (NSCLC) through an apoptosis pathway, mediated by ROS generation both in vitro and in vivo.41
Another important chalcone-based compound, isoliquiritigenin, has been isolated from licorice root and proven to exhibit strong therapeutic potential against various types of cancers including breast cancer, gastrointestinal cancer, ovarian cancer, colon cancer, lung cancer, and melanoma.42 An active and one of the most useful chalcone-based compounds, isobavachalcone, has been isolated from the Fabaceae and Moraceae families, which possesses strong antitumor activity towards various cancer types. Furthermore, it is very effective towards human prostate cancer,43 colorectal cancer cells,44 ER+ breast cancer,45 etc. cardamonin,46 xanthohumol, panduretin A, and lonchocarpin36 are few examples of chalcone-based compounds isolated from different natural resources, and also well known to be very effective for their strong anticancer activity towards various cancer types.
Chalcone in chemists' round-bottom flask
Inspired by the strong anticancer potency of naturally occurring chalcone molecules, researchers have developed synthetic chalcone derivatives, which have strong therapeutic application for the treatment of different forms of cancer. Modification of the structure of chalcone changes its physicochemical and biological properties to a large extent. Modification has been done primarily with the inclusion of a different number of substituents and at different positions in its benzene rings, and then several research groups replaced the aryl group with alicyclic, heteroaryl, and steroid scaffolds.47 Molecular hybridization is a very common strategy that has been employed by scientists in their continuous pursuit to obtain effective chalcone-based chemotherapeutic agents.
Important chalcone derivatives and their anticancer activities
Natural chalcones are known to possess marked anticancer properties. Therefore, researchers have shown keen interest in the development of new chalcone derivatives having superior physicochemical and biological properties. Modification of the activities of chalcones has been done by adopting three different strategies, which include modification of the associated aromatic rings, replacement of the aromatic rings with heteroaromatic rings, and coupling with other molecules having important biological activities. The presence of substituents at different positions of the aromatic rings of the chalcone backbone influences its biological properties. It has been proven by many research works that the introduction of –OH and –OMe groups in the aromatic ring of the chalcone moiety strongly affects its biological activities.48–50 Cuellar et al.51 synthesized methoxy chalcones for application as anticancer agents, among which two compounds (Fig. 6, compounds 1 and 2) have been found to possess considerable growth inhibition activity against the MCF-7, ZR-75-1 and MDA-MB-231 cancer cell lines with significant IC50 values. These chalcones are found to have differential activity against tumor cell lines compared to that of MCF-10F having IC50 values ∼75 μM.
 |
| Fig. 6 Structures of compounds 1 and 2. | |
Chavasiri and co-workers reported the synthesis of a series of methoxy-substituted chalcones through Claisen–Schmidt condensation reaction, and their assessment of the anticancer properties of these compounds against different cell lines revealed their corresponding potential. Among the compounds studied, four compounds (Fig. 7, compounds 3–6) were found to be the most potent, having slightly lower cytotoxicity against normal cell lines compared to that of the A549 cell line.52
 |
| Fig. 7 Structures of compounds 3–6. | |
Xanthohumol derivatives of chalcones
A series of seven mono and diacyl-xanthohumol was synthesized and their antiproliferative properties were checked against the HT-29 human cancer cell line. The SRB assay was used for this purpose. Among the compounds (Fig. 8), compounds 7–10 were found to exhibit the greatest activity, having IC50 values in the range of 10–12 μM, which were slightly higher in magnitude compared to that of the parent compound, xanthohumol, especially compound 7. Their antioxidant activity was determined using applicable method and compound 7 was found to possess lower antioxidant activity than that of the parent compound xanthohumol, whereas the others showed either comparable or higher antioxidant activity.53
 |
| Fig. 8 Structures of compounds 7–10. | |
Popłoński et al.54 synthesized a series of six derivatives of chalcone via the cyclisation of the prenyl group of xanthohumol and their antiproliferative properties were evaluated against three human cancer cell lines, PC-3, MCF-7 and HT-27, using the SRB assay technique. All the compounds tested exhibited moderate to increased bioactivity and the MCF-7 breast cancer cell line was found to be the most susceptible one among the human cancer cell lines tested. Compounds 11, 12, and 13 (Fig. 9) xanthohumol C, dihydroxanthohumol K and 2,3-dehydroisoxanthohumol were to exhibit the best results on the PC-3 cancer cell line, which is comparable to that of the most used cancer drug, cisplatin, for the treatment of cancer.
 |
| Fig. 9 Structures of compounds 11–13. | |
Diaryl ether moiety-containing chalcone derivatives
A series of sixteen diaryl ether-containing chalcone moieties was synthesized by Wang et al. Their antiproliferative properties were investigated using three human cancer cell lines, HepG2, MCF-7, and HCT116. The majority of the compounds possessed superior to moderate anticancer activity against the three human cancer cell lines, having IC50 values in the range of 3–9 μM. Compound 14 (Fig. 10) was found to be the most effective on the three cancer cell lines. The introduction of a 4-dialkylamino group in the place of the 4-OMe group decreased the activity. Compound 14 increased the cell percentage in the G2/M phase, thereby increasing the antiproliferative properties against the MCF-7 cells. The annexin V-FITC method was also applied to comprehend the chalcone-induced apoptosis of the cell line (MCF-7) studied. Additionally, the molecular docking study supported the binding phenomena, which adopted a Y-shaped conformation, where the strong binding of the compound was induced by hydrogen bonding, hydrophobic bond formation and cation–π interaction.25
 |
| Fig. 10 Structure of compound 14. | |
Chalcone derivatives containing a sulfonamide moiety
Castaño et al. designed a series of α,β-unsaturated sulphonamide-based chalcones, among which compounds 15–26 (Fig. 11) were found to possess strong anticancer properties against the K-562 cancer cell line. Compound 16 exhibited the best activity towards the human erythroleukemic cell line (K-562), human colorectal carcinoma cell line (HCT-116), and human melanoma cell line (LOX IMVI) with considerable GI50 values.55
 |
| Fig. 11 Structures of compounds 15–26. | |
Bischalcone derivatives
Compounds having two chalcone moieties in a single molecule are known as bischalcone. The cytotoxic effects of some bischalcones against various human cancer cell lines, e.g., KB, A549, DU145, KB-VN, and HeLa, have been established. Bischalcones with biphenyl residues within their molecules have been found to be active on cancer cell lines including MDA-MB 231, HEK-293, MCF-7, and HeLa. A series of bischalcone derivatives was synthesized by Burmaoglu et al. and reported in the journal, “Bioorganic Chemistry” in 2019. Their anticancer potencies were determined by the MTT assay technique against two cancer cell lines, Caco2 and MCF-7. The synthesized compounds possessed superior activity against the tested cancer cell lines. Among the compounds, the compounds having fluoro groups (compounds 27 and 28) (Fig. 12) were found to exhibit better IC50 values upon treatment against the MCF-7 cell line and Caco-2 cell line. The anticancer activity of these compounds was found to be seven times greater than that of allopurinol.56
 |
| Fig. 12 Structures of compounds 27 and 28. | |
The Claisen–Schmidt condensation reaction was applied by Li and co-workers57 for the synthesis of lysine-linked bischalcones, among which, one was found to be the most effective (compound 29) (Fig. 13) and screened for its application in different cancer cell lines. Their bischalcone conjugate was shown to have the greatest sensitivity towards the AGS cancer cell line, having an IC50 value of 22.1 μM and TMK1 having an IC50 value of 22.2 μM, compared to that of the standard reference drug, 5-fluoro-uracil. The in vivo studies on this compound also revealed its potential with negligible side effects.
 |
| Fig. 13 Structure of compound 29. | |
Chalcones containing nitrogen in their molecule
Chalcone, bearing an amino group in the aromatic ring of its structure, is well known for its biological activity. 2-Aminochalcones are known to possess proapoptotic activity and antiproliferative activity.58 Therefore, a library of eighteen amino chalcones was reported by Kozłowska et al. to act as anticancer agents, among which 10 were novel and not reported earlier in the scientific literature. Compounds 30–32 (Fig. 14) exhibited superior anticancer potency towards the HT-29 colon cancer cell line, having an IC50 value of ∼1.5–2 μg mL−1. The antiproliferative property was 12-times stronger than the widely used anticancer drug, cisplatin. Introduction of nitro groups at the para position of the aminochalcone also resulted in similar activities when tested against the LoVo/DX and LoVo human cancer cell lines by the SRB method. Cisplatin and doxorubicin were used as the standard for this study. The results showed that the introduction of carboxylic acids in the amino chalcone moiety affected the biological activity of the molecules. The position of the introduced carboxylic acid had a major role in dictating the biological property of the resulting aminocarboxylic chalcones. 2-Aminochalcone showed greater activity compared to that of the 3- and 4-aminochalcone. It was also reported that the introduction of a nitro group at the para position of the corresponding aldehyde of the chalcone decreased the bioactivity. These molecules followed Lipinski's rules, making them potent candidates to be applied as potential drugs. Their strong antiproliferative property on human colon cancer cell line and nature to prevent microbial growth also reinforced their application as potential anticancer agents.59
 |
| Fig. 14 Structures of compounds 30–32. | |
Wang et al. detected a series of twelve amino chalcones having anticancer properties against HCT116 and HepG2 cancer cell lines by performing the MTT assay. Compound 33 (Fig. 15) was found to possess superior anticancer activity towards the tested cancer cell lines. This compound also showed low cytotoxicity when it was tested against the LO2 normal human cell line. Substitution of the amino group with an alkyl group reduced the antiproliferative property. The in vitro tubulin polymerization inhibition assay unveiled that tubulin assembly inhibition took place in a concentration-dependent manner, having an IC50 value of 7.1 μM compared to that of the standard compound, colchicine. Its ability towards cell cycle arrest in the G2/M phase is noteworthy. Strong interaction of the abovementioned compound with tubulin with its active site colchicine was demonstrated by a molecular docking study. All the results reported in this research article proved that compound 33 is a potential alternative anticancer agent.60
 |
| Fig. 15 Structure of compound 33. | |
Chalcone hybrids
Despite their very strong effective anticancer properties, synthetic chalcones have many drawbacks including drug resistance properties.61–63 Alternatively, hybrids chalcone molecules have been proven to have increased specificity and strong activity towards the target cancer cells.64–66 Classical chalcone moieties have been effectively hybridised with important pharmacophores, e.g., azole, coumarin, indole, and imidazole, to obtain more effective chalcone hybrids. Some of the representative chalcone hybrids that have been recently evaluated and shown to exhibit strong anticancer property both in vitro and in vivo are summarized below.
Chalcone–azole hybrids
Azoles are an important class of nitrogen-containing heterocyclic compounds. This class of pharmacophores plays an important role in hybridisation with chalcone moieties to obtain a new class of more effective chemotherapeutic agents.67 Some chalcone–azole hybrid compounds, such as AZD8835, cefatrizine, and carboxyamidotriazole, have been used clinically for the treatment of cancer. Imidazole, tetrazole, oxazole, pyrazole, thiazole and triazole (Fig. 16) compounds will be covered under the discussion of chalcone–azole hybrid moieties. Some representative examples containing azole moieties are congregated here.
 |
| Fig. 16 Structures of different azoles. | |
Imidazole is a heteroaromatic compound having two annular nitrogen atoms. Due to the presence of two nitrogen atoms within its ring, imidazole is highly polar in nature. It also has amphoteric character, and thus it behaves as both an acid and base. Several imidazole-containing compounds are well-known for their marked anticancer properties against different carcinoma cell lines.68–70
Oskuei et al.71 reported a series of imidazole-based anticancer agents (compounds 34 and 35), which acted as tubulin polymerization inhibitors (Fig. 17). The assessment of these compounds was done against four cancer cell lines including A549, MCF-7, MCF-7/MX, and HEPG2. Compounds 34 and 35 were shown to be effective, having IC50 values in the range of 7.05 to 63.43 μM. Detailed studies revealed that their potentiality of tubulin polymerization inhibition was comparable to that of the trimethoxyphenyl pharmacophore CA-4. This research group had also performed a molecular docking study, proving the probable interactions of the compounds with tubulin.
 |
| Fig. 17 Structures of compounds 34 and 35. | |
Wang et al.72 designed and synthesized 24 compounds having potent antitumor activity. Their antitumor activity was checked against the HCT116, 143B, and MCF7 cell lines. Among the compounds, compound 36 (Fig. 18) was found to possess >90% inhibition for both Cat L and Cat K. The IC50 values were very impressive, which was supported by molecular docking. Thus, the antitumor activity of compound 36 was shown to be very effective targeting the Cat L, Cat K cell lines, and thus can be a potent candidate exhibiting strong anticancer properties.
 |
| Fig. 18 Structure of compound 36. | |
Tetrazole is an unsaturated heterocyclic compound having a five-membered ring, which consists of four nitrogen atoms and a carbon atom. Biological compounds containing tetrazole moieties have effective anticancer properties. Letrozole, a well-known tetrazole-based drug, has been used clinically for the treatment of tamoxifen-refractory breast cancers.73
Abd ElMonaem et al. reported a series of tetrazole chalcone hybrid molecules having in vitro antiproliferative property against the studied cancer cell lines including the HCT116 colon cell line and PC-3 prostate cell line. They were reported to possess better activity compared to that of the commonly used anticancer drugs cisplatin and 5-fluorouracil. Compounds 37, 38 and 39 (Fig. 19) displayed the best efficacies among the tested cancer cell lines, having appreciable IC50 values and high selectivity indices.74
 |
| Fig. 19 Structures of compounds 37–39. | |
Oxazole is an N- and O-containing five membered heterocyclic compound. It exhibits slightly basic character. It is considered the parent compound of many heterocyclic aromatic compounds. Oxazole and its derivatives are well-known for their important anticancer, antibacterial, anti-allergic, anticonvulsant, antiviral, anthelmintic, analgesic, antidepressant, and antioxidant properties.75
A series of oxazole-containing chalcone derivatives were prepared by Li et al. and their reversible and time-dependent LSD1 inhibitory activity was evaluated. Among the chalcones studied, compound 40 (Fig. 20) was found to exhibit the strongest LSD1 activity having an IC50 value of 0.14 μM. Compound 40 also possessed cell-proliferation inhibitory properties when treated against the HAL-01, KE-37, SUP-B15, P30-OHK, MOLT-4 and LC4-1 leukaemia cell lines. Its IC50 values were found to be 1.10 μM, 3.64 μM, 1.87 μM, 3.85 μM, 0.87 μM and 2.73 μM, respectively. Noteworthily, the in vivo inhibition of tumor growth upon treatment with compound 40 in the xenograft model revealed its negligible toxicity.76
 |
| Fig. 20 Structure of compound 40. | |
Pyrazole is a heterocyclic compound having a five-membered ring structure, which is composed of three carbon atoms and two nitrogen atoms at adjacent positions. It is slightly basic in nature. Extensive studies have shown that pyrazole-containing substances possess important pharmacological activities such as anti-fungal, anti-microbial, anti-inflammatory, anti-tubercular, anticancer, anti-convulsant, and anti-viral activities.77 Some important anticancer compounds such as axitinib, ruxolitinib, celecoxib, difenamizole, epirizole, fezolamine, isolan, lonazolac, pyrazofurin, rimonabant, tepoxalin, zoniporide, and crizotinib contain pyrazole as the key pharmacophore.78,79
A series of pyrazole-based chalcone moieties were reported by Prasad et al. Among the compounds tested, compounds 41, 42, 43 and 44 (Fig. 21) possessed the highest anticancer potency compared to that of the standard drug camptothecin.80 They also performed an ADMET (Absorption, Distribution, Metabolism, Excretion, and Toxicology) study, which revealed that the synthesized pharmacophores exhibit strong potency in cancer therapy. Notably, this study is one of the important key studies in drug discovery and the drug delivery process.
 |
| Fig. 21 Structures of compounds 41–44. | |
Hawash et al. synthesized a series of 42 pyrazole-based chalcone compounds. Their activity against different cancer cell lines was studied in detail. Among the compounds, compounds 45–48 (Fig. 22) exhibited strong antiproliferative properties and were found to exhibit better results compared to that of the popular drug, 5-fluorouracil. The IC50 values for compounds 45–48 for epithelial cells and in the HCC cell line were found to be satisfactory in the range of 0.4–3.4 μM. Among these four active compounds, compound 46 and compound 48 possessed the greatest activity against the tested HCC cell line and epithelial cells (e.g., the IC50 values of compounds 46 and 48 for HepG2 were of 3.4 and 4.8 μM, and the IC50 values of compounds 46 and 48 for MCF12A were 2.4 and 3.0 μM, respectively), which may be potent candidates for further clinical studies.81
 |
| Fig. 22 Structures of compounds 45–48. | |
Thiazole is a heterocyclic compound having a five-membered ring with nitrogen and sulphur in the 1 and in 3 positions, respectively. Thiazole-containing compounds are well-known for their important biological properties including antifungal, antitubercular, anticancer, antiparasitic, anti-inflammatory, antioxidant, antigout, antibacterial, and anticonvulsant properties.82 Thiazole-containing compounds exhibit antiproliferative properties through the inhibition of metalloprotease, BcL2 family proteins, and some kinases.83 Because of the presence of two heteroatoms, sulphur and nitrogen, the thiazole moiety can bind easily with the amino acid residues of the receptor proteins, which is responsible for the apoptotic function of thiazoles on cancer cells. Some well-known anticancer agents contain a thiazole group in their structure, e.g., epothilones, bleomycin, kud773, and thiazofurine.84
Suma et al. designed and synthesized thiazole-based chalcones having imidazopyridine scaffolds, which acted as important pharmacophores. These compounds were found to be effective towards all four cancer cell lines tested including MCF-7 and MDA-MB-231 breast carcinoma, A549 lung carcinoma, and DU-145 prostate carcinoma cell lines. The MTT assay was employed for this purpose using etoposide as the positive control. Among the compounds, compound 49 (Fig. 23) was found to be the most effective with IC50 values in the range of 0.1–1.0, which proved that it had stronger anticancer activity compared to that of the positive control, etoposide. Thus, compound 49 can be a potent anticancer agent, which can be used as a chemotherapeutic agent.85
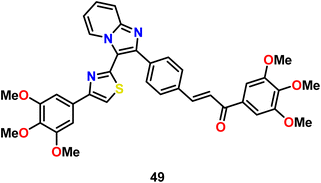 |
| Fig. 23 Structure of compound 49. | |
A series of thiazole-based chalcones and rigid chalcone derivatives was designed for successful application as anticancer agents. Their antiproliferative properties against human cancer cell lines, i.e., HepG-2, MCF-7, and A549, were investigated and doxorubicin was used as a control. Four thiazole-based chalcones were found to be the most effective. The high selectivity index as well as low toxicity on the WI38 normal lung cell line of these thiazole-based chalcones were also noteworthy. The CDK inhibition assay studies were carried out with these four compounds, compounds 50–53 (Fig. 24), where they were proven to be nonselective inhibitors towards the tested CDKs, having IC50 values of ∼2 μM. The annexin-V assay was carried out for the assessment of their apoptotic ability, where it was demonstrated that these compounds could influence apoptosis by increasing apoptotic cell-death. In the molecular docking study, it was revealed that three active thiazole-based chalcones were bound to the ATP of the CDK1 binding sites. The thiazole-based chalcones were bound to Leu83 by the formation of H-bonds among the thiazole sulphur, aminomethyl group and the specified amino acids. Again, two synthesized chalcones were bound to another amino acid residue (Glu81) of the targeted enzyme.86
 |
| Fig. 24 Structures of compounds 50–53. | |
Triazole is a heterocyclic compound containing three nitrogen atoms in the 1,2,3- or 1,2,4-positions. Heterocyclic organic compounds are well-known for their important biological properties, e.g., anticancer, anti-inflammatory, anti-HIV, and anti-tuberculosis.35
Gurrapu et al. synthesized a series of nine 1,2,3-triazole-containing chalcone hybrids as potential anticancer agents. Their effectivity was screened against three cancer cell lines, i.e., MCF-7, HeLa, and MDA-MB-231 cell lines. The MTT assay method was applied for the determination of in vitro cytotoxic activity, where these hybrid chalcones showed moderate to significant cytotoxicity. Among the chalcone hybrids studied, compounds 54–56 (Fig. 25) displayed the highest cytotoxicity, having lower IC50 values compared to that of the commonly used drug cisplatin. The molecular docking study revealed the hydrogen bonding interaction, π–π stacking interaction, and cation–π interaction of these compounds with ASP 800, PHE 856 and PHE 997, LYS 745, respectively. All these results demonstrated an alternative pathway towards the development of chemotherapeutic drugs.87
 |
| Fig. 25 Structures of compounds 54–56. | |
A series of 1,2,4-triazole-containing chalcone hybrids was designed by Ahmed et al. The cytotoxicity of compounds 57–61 (Fig. 26) was investigated against different cancer cell lines, which were observed to be the most effective towards the A549 lung cancer cell line. They exhibited the IC50 values in the range of 4.4 to 16.04 μM compared to that of the widely applied drug cisplatin having an IC50 value of 15.3 μM. It was visualised that the phenyl-substituted compounds were less active compared to that of the allyl substituted ones. These compounds were found to affect caspase-3-dependent apoptosis in both the intrinsic and extrinsic apoptosis pathways in a dose-dependent manner. Thus, the synthesized 1,2,4-triazole-based chalcone hybrids were found to possess strong anticancer potency with less side effects as a chemotherapeutic agent compared to that of the traditional drug molecules.88
 |
| Fig. 26 Structures of compounds 57–61. | |
A series of 12 quinoline-based 1,2,4-triazole chalcone hybrids was synthesized by Mohassab et al. Among them, 5 compounds (compounds 62–66) (Fig. 27) were found to possess significant antiproliferative properties and three compounds (compounds 63, 65, 66) were more potent compared to that of the positive control, erlotinib. The BRAFV600E inhibitory assay was carried out to determine the anticancer property and they were found to be effective towards inhibiting the BRAFV600E enzyme. The molecular docking study revealed the interaction of these compounds with the active sites of the EGFR and BRAFV600E enzymes, showing the suitable fitting of the compounds with the active sites of the enzymes. The binding free energy values towards EGFR were higher compared to that of the control drug erlotinib, and their binding affinities towards BRAFV600E were comparable to that of the standard drug vemurafenib.89
 |
| Fig. 27 Structures of compounds 62–66. | |
Chalcone heterocyclic hybrids
Pyrazole-, thiophene- and pyrrole-containing ferrocene-based chalcones (compounds 67–69) (Fig. 28) were synthesized by Montes-González et al.90 and their biological properties were evaluated, respectively. Three compounds were found to possess excellent IC50 values in the range of 6.59–12.51 μM, when tested against the MDA-MB-231 triple negative breast cancer cell line. Their cytotoxicity was also evaluated in the 4T1 cancer cell line, having IC50 values ranging from 13.23 to 213.7 μM as well as the MRC-5 normal cell line.
 |
| Fig. 28 Structures of compounds 67–69. | |
Chalcone indole hybrids
Indole bears a heterocyclic moiety, where a benzene ring is fused with a pyrrole ring. Indole compounds exhibit significant antifungal properties. Therefore, Bhale et al. synthesized indole-based chalcones and evaluated their potential application towards Vero normal monkey cells and the MCF7 breast cancer cell line.91 They reported that the two synthesized compounds (compounds 70 and 71) (Fig. 29) exhibited significant potential towards the MCF7 cancer cell line. The GI50 values were calculated for these compounds and were found to be 0.1 and 16.3 μM, respectively, which matched the efficiency of the commonly used standard drug Adriamycin, having a GI50 value of <0.1 μM.
 |
| Fig. 29 Structures of compounds 70 and 71. | |
Chalcone-like benzofuran hybrid
Ari and coworkers92 synthesized a benzofuran-based chalcone-like compound (compound 72) (Fig. 30) and tested its potential against the HCT116 and HT29 colon cancer and A549 and H1299 human lung cancer cell lines and found to be more effective compared with the drug 5-fluoro uracil.
 |
| Fig. 30 Structure of compound 72. | |
Chalcone triazine hybrids
Triazine is a well-known class of heterocyclic compounds having three nitrogen atoms in its six-membered aromatic ring. Melamine and cyanuric chlorides are the commonly used 1,3,5-triazine derivatives. The triazine derivative melamine is widely used as a precursor of resin.
Khattab et al.93 synthesized 1,3,5-triazine-based chalcones for their application as anticancer agents. The MTT assay was performed, revealing that two compounds (compounds 73 and 74) (Fig. 31) possess significant growth inhibition of A549 cancer cells with IC50 values of 24.5 μM and 17.0 μM, respectively, compared to that of the well-known cancer drug cisplatin, having an IC50 value of 21.5 μM (Table 1).
 |
| Fig. 31 Structures of compounds 73 and 74. | |
Table 1 Chalcone-containing compounds with anticancer potency
S. no. |
Details |
Compounds |
IC50 value (μM) |
Cell lines |
Reference |
1 |
Chalcone derivatives |
1 |
75.76 ± 11.52 |
MCF-7 |
51 |
2 |
75.11 ± 11.97 |
2 |
Chalcone derivatives |
3 |
2.93 |
A549 |
52 |
4 |
0.91 |
5 |
4.57 |
6 |
5.34 |
3 |
Xanthohumol derivative of chalcones |
7 |
9.74 |
HT-29 |
53 |
8 |
11.84 |
9 |
11.75 |
10 |
10.04 |
4 |
Xanthohumol derivative of chalcones |
11 |
15.09 ± 3.6 |
MCF-7 |
54 |
12 |
9.0 ± 6.4 |
13 |
7.0 ± 0.5 |
5 |
Diaryl ether moiety-containing chalcone derivatives |
14 |
3.44 ± 0.19 |
MCF-7 |
25 |
6.31 ± 0.27 |
HCT116 |
6 |
Chalcone derivatives containing a sulfonamide moiety |
16 |
0.5 (GI50) |
K562 |
55 |
1.28 (GI50) |
LOX IMVI |
7 |
Bischalcone derivatives |
27 |
1.9 |
MCF-7 |
56 |
28 |
6.8 |
Caco2 |
8 |
Bischalcone derivatives |
29 |
22.2 |
TMK1 |
57 |
9 |
Chalcones containing nitrogen in the molecule |
30 |
1.43 ± 0.16 |
HT-29 |
59 |
31 |
1.60 ± 0.01 |
32 |
1.98 ± 0.18 |
10 |
Chalcones containing nitrogen in the molecule |
33 |
0.28 ± 0.06 |
HCT116 |
60 |
11 |
Chalcone hybrid |
34 |
66.47 ± 4.85 |
A549 |
71 |
Chalcone–azole hybrid |
35 |
11.7 ± 1.62 |
Imidazole hybrid |
|
Chalcone hybrid |
36 |
0.597 |
HCT116 |
72 |
Chalcone–azole hybrid |
0.886 |
MCF7 |
Imidazole hybrid |
0.791 |
143B |
12 |
Tetrazole hybrid |
37 |
0.6 |
HCT116 |
74 |
1.6 |
PC-3 |
13 |
Oxazole hybrid |
40 |
1.10 |
HAL-01 |
76 |
3.64 |
KE-37 |
3.85 |
P30-0HK |
1.87 |
SUP-B15 |
0.87 |
MOLT-4 |
2.73 |
LC4-1 |
14 |
Pyrazole hybrid |
41 |
44.04 ± 0.01 |
— |
80 |
42 |
39.94 ± 0.01 |
43 |
48.35 ± 0.02 |
44 |
44.20 ± 0.01 |
15 |
Pyrazole hybrid |
45 |
1.1 ± 0.50 |
MCF-7 |
81 |
46 |
1.5 ± 0.52 |
47 |
1.6 ± 0.66 |
48 |
1.4 ± 0.38 |
16 |
Thiazole hybrid |
49 |
0.18 ± 0.094 |
MCF-7 |
85 |
0.66 ± 0.071 |
A549 |
1.2 ± 0.45 |
DU-145 |
0.069 ± 0.0082 |
MDA-MB-231 |
17 |
Thiazole hybrid |
50 |
1.97 ± 0.09 |
MCF-7 |
86 |
51 |
4.14 ± 0.35 |
52 |
2.38 ± 0.14 |
53 |
4.11 ± 0.3 |
50 |
1.39 ± 0.061 |
A549 |
51 |
3.17 ± 0.15 |
52 |
2.01 ± 0.1 |
53 |
2.37 ± 0.12 |
18 |
1,2,3-Triazole |
54 |
2.52 ± 0.49 |
MCF-7 |
87 |
55 |
1.27 ± 0.89 |
56 |
1.72 ± 0.75 |
54 |
0.78 ± 0 |
MDA-MB-231 |
55 |
0.31 ± 0.01 |
56 |
2.66 ± 0 |
19 |
1,2,4-Triazole |
57 |
6.06 ± 0.5 |
A549 |
88 |
58 |
4.4 ± 0.3 |
59 |
7.55 ± 0.8 |
60 |
16.04 ± 1.32 |
61 |
8.04 ± 0.59 |
|
|
|
20 |
1,2,4-Triazole |
62 |
7.5 ± 1.2 |
MCF-7 |
89 |
63 |
3.3 ± 0.08 |
64 |
6.8 ± 1.6 |
65 |
3.2 ± 0.08 |
66 |
4.9 ± 0.6 |
|
|
|
20 |
Chalcone heterocyclic hybrids |
67 |
6.59 |
MDA-MB-23 |
90 |
68 |
10.4 |
69 |
12.5 |
Chalcone heterocyclic hybrids |
67 |
32.9 |
4T1 |
68 |
76.5 |
69 |
13.2 |
22 |
Chalcone indole hybrid |
70 |
<0.1 |
MCF7 |
91 |
71 |
16.3 |
23 |
Chalcone-like benzofuran hybrid |
72 |
2.85 |
A-549 |
92 |
1.46 |
H1299 |
0.59 |
HCT116 |
0.35 |
HT29 |
24 |
Chalcone triazine hybrid |
73 |
24.5 |
A549 |
93 |
74 |
17.0 |
According to the above-mentioned studies on chalcone-based compounds by different research groups, it has been found that the incorporation of electron-donating substituents (e.g. –OMe) in the aromatic ring part of the chalcone moiety increases the anticancer potency. The naturally occurring chalcone, Licochalcone A, containing an –OMe group has been found to possess strong anticancer potency. The position of the substituent present in the aromatic ring also plays a crucial role regarding the use of compounds as anticancer agents. The efficiency is found to increase proportionally with an increase in the number of electron-donating substituents in the aromatic ring and incorporating aromatic and heterocyclic rings. On the contrary, the incorporation of electron-withdrawing groups may have some detrimental effects on the efficiency of chalcone-based compounds to act as potential anticancer agents.
Conclusion
Chalcones play a crucial role in the synthesis of many flavonoid-containing natural products. The rigid backbone of these Michael acceptors makes them more potent towards a wide range of biological activities including anticancer potency. Due to the simplicity of their structure, it is easy to tune their activity to target several cellular molecules including tubulin and MMP-2/9.94 In summary, this review article shed light on the potentiality of chalcones and their derivatives as anticancer agents. We highlighted the recent advancement in research on chalcones as chemotherapeutic agents. Emphasis was given to the recent advancements in chalcone hybrid research and substituted chalcones including synthetic chalcones. This discussion also covered the different procedures for the preparation of chalcones, including examples of naturally occurring chalcones (e.g., butein, xanthohumol and lonchocarpin) and synthetic chalcones (mainly derivatives of chalcones containing a diaryl ether or sulfonamide moiety and bischalcone derivatives). This review also included a variety of chalcones containing a nitrogen in their molecule, in the form of different groups including azole, imidazole, tetrazole, oxazole, pyrazole, thiazole, and triazole. In the continuous search for low-cost chemotherapeutic agents having low toxicity, chalcones may be proven to be promising candidates in the first row to meet the criteria of more target-specific nature, leading to less side effects in cancer patients. With the advancement of cancer treatment technology, novel and efficient motifs such as chalcones have become the focus, possessing important properties such as easy synthetic techniques, reduced toxicity and great potentiality. It is noteworthy that chalcones are capable of targeting different cell lines in diverse ways and via unique mechanisms of action, which make them potent candidates for cancer treatment. This mushrooming research area is expected to unveil a new era for novel chalcone-based efficient and safer compounds as potent anticancer agents, which may become a breakthrough in the research and development of the treatment modalities for deadly diseases, including cancer. Briefly, this review indicates the bright future in the development of chalcone-based anticancer research and extends the hope for reduced side effects of commonly used chemotherapeutic agents, thereby diminishing the suffering of cancer patients during the treatment process.
Abbreviations
MET | Mesenchymal epithelial transition factor |
ATM | Ataxia telangiectasia mutated |
NSCLC | Non-small cell lung cancer |
ROS | Reactive oxygen species |
PC-3 | Human prostate cancer cell line |
MCF-7 | Michigan Cancer Foundation-7. A breast cancer cell line |
HepG2 | Hepatoblastoma cell line |
HCT116 | Human colon cancer cell line |
U251 | Glioma cell line |
K562 | Cell line derived from chronic myeloid leukemia |
LOX IMVI | Human melanoma cell line |
A549 | Lung cancer cell line; human alveolar basal epithelial cell |
DU145 | Human prostate cancer cell line |
HeLa | Cervical cancer cell |
MDA-MB 231 | Breast cancer cell line |
HEK-293 | Human embryonic kidney 293 cells |
Caco2 | Cancer coli-2 |
TMK1 | Human gastric adenocarcinoma cell line |
HT-29 | Human colorectal adenocarcinoma cell line |
HTC116 | Human colorectal carcinoma cell line |
LO2 | Human fetal hepatocyte cell |
143B | Human osteosarcoma cell |
PC-3 | Human prostate cancer cell |
HAL-01 | Human leukemia cell line |
KE-37 | Acute lymphoblastic T cell leukemia cell line |
SUP-B15 | B lymphoblast cell line |
P30-OHK | B lymphoblast cell line |
MOLT-4 | Human acute T lymphoblastic leukemia cell |
LC4-1 | Acute lymphoblastic leukemia cell line |
ADMET | Absorption, distribution, metabolism, excretion, and toxicology |
HCC | Hepatocellular carcinoma |
MCF12A | Non-tumorigenic human breast epithelial cell line |
DU-145 | Human prostate cancer cell line |
MDA-MB-23 | Human breast cancer cell line |
MRC-5 | Cell line derived from lung tissue of a 14 week-old male fetus |
HCT116 | Human colorectal carcinoma cell line |
HT29 | Human colorectal adenocarcinoma cell line |
H1299 | Human non-small cell lung cancer cell line |
Data availability
No new data were generated in this review.
Conflicts of interest
There are no known conflicts to declare.
Acknowledgements
The authors, NR, NT and PP, are grateful to the Department of Science and Technology, Government of India for supporting the work through the DST-SERB CRG project grant (CRG/2021/002267), VIT University for VIT SEED funding, and DST New Delhi for DST-FIST project. RC would like to acknowledge the Department of Chemistry, Alipurduar University, West Bengal, India. RC acknowledges the SEED research grant (No. APDU/Reg./R. P./Notice/001/2024 dated 29.02.2024) of Alipurduar University for financial support.
References
- H. Nagai and Y. H. Kim, Cancer prevention from the perspective of global cancer burden patterns, J. Thorac. Dis., 2017, 9, 448 CrossRef PubMed.
- T. Constantinescu and C. N. Lungu, Anticancer Activity of Natural and Synthetic Chalcones, Int. J. Mol. Sci., 2021, 22, 11306 CrossRef CAS PubMed.
- Y. Ouyang, J. Li, X. Chen, X. Fu, S. Sun and Q. Wu, Chalcone Derivatives: Role in Anticancer Therapy, Biomolecules, 2021, 11, 894 CrossRef CAS PubMed.
- F. Truzzi, C. Tibaldi, Y. Zhang, G. Dinelli and E. D'Amen, An Overview on Dietary Polyphenols and Their Biopharmaceutical Classification System (BCS), Int. J. Mol. Sci., 2021, 22, 5514 CrossRef CAS PubMed.
- O. Praksah, A. Kumar and P. Kumar, Ajeet, Anticancer Potential of Plants and Natural Products: A Review, Am. J. Pharmacol. Sci., 2013, 6, 104–115 Search PubMed.
- S. Narwal, B. Devi, T. Dhanda, S. Kumar and S. Tahlan, Design, synthesis, anticancer and in silico assessment of 8-caffeinyl chalcone hybrid conjugates, J. Mol. Struct., 2024, 1303, 137554 CrossRef CAS.
- S. Kaur, J. Kaur, A. Anand, K. Kaur and V. Choudhary, Chalcones: Effective Agents for Fighting Cancer Across Multiple Cell Lines, ChemistrySelect, 2024, 9, e202304546 CrossRef CAS.
- H. A. Jasim, L. Nahar, M. A. Jasim, S. A. Moore, K. J. Ritchie and S. D. Sarker, Chalcones: Synthetic Chemistry Follows Where Nature Leads, Biomolecules, 2021, 11, 1203 CrossRef CAS PubMed.
- D. Elkhalifa, I. Al-Hashimi, A. E. Al Moustafa and A. Khalil, A comprehensive review on the antiviral activities of chalcones, J. Drug Targeting, 2021, 29, 403–419 CrossRef CAS PubMed.
- Y. Lin, M. Zhang, Q. Lu, J. Xie, J. Wu and C. Chen, A novel chalcone derivative exerts anti-inflammatory and anti-oxidant effects after acute lung injury, Aging, 2019, 11, 7805–7816 CrossRef CAS PubMed.
- D. K. Mahapatra, S. K. Bharti and V. Asati, Chalcone Derivatives: Anti-inflammatory Potential and Molecular Targets Perspectives, Curr. Top. Med. Chem., 2017, 17, 3146–3169 CrossRef CAS PubMed.
- S. Rocha, D. Ribeiro, E. Fernandes and M. Freitas, Bis-Chalcones: A Review On Synthetic Methodologies And Anti Inflammatory, Curr. Med. Chem., 2020, 27, 2257–2321 CrossRef CAS PubMed.
- B. Salehi, C. Quispe, I. Chamkhi, N. El Omari, A. Balahbib, J. Sharifi-Rad, A. Bouyahya, M. Akram, M. Iqbal, A. O. Docea, C. Caruntu, G. Leyva-Gómez, A. Dey, M. Martorell, D. Calina, V. López and F. Les, Pharmacological Properties of Chalcones: A Review of Preclinical Including Molecular Mechanisms and Clinical Evidence, Front. Pharmacol., 2021, 11, 592654 CrossRef PubMed.
- D. D. Jandial, C. A. Blair, S. Zhang, L. S. Krill, Y.-B. Zhang and X. Zi, Molecular targeted approaches to cancer therapy and prevention using chalcones, Curr. Cancer Drug Targets, 2014, 14, 181–200 CrossRef CAS PubMed.
- B. Zhou and C. Zhing, Diverse Molecular Targets for Chalcones with Varied Bioactivities, Med. Chem., 2015, 5, 388–404 Search PubMed.
- C. Zhuang, W. Zhang, C. Sheng, W. Zhang, C. Xing and Z. Miao, Chalcone: A Privileged Structure in Medicinal Chemistry, Chem. Rev., 2017, 117, 7762–7810 CrossRef CAS PubMed.
- M. N. Gomes, E. N. Muratov, M. Pereira, J. C. Peixoto, L. P. Rosseto, P. V. L. Cravo, C. H. Andrade and B. J. Neves, Chalcone Derivatives: Promising Starting Points for Drug Design, Molecules, 2017, 22, 1210 CrossRef PubMed.
- K. Zhou, S. Yang and S.-M. Li, Naturally occurring prenylated chalcones from plants: structural diversity, distribution, activities and biosynthesis, Nat. Prod. Rep., 2021, 38, 2236–2260 RSC.
- C. Halpani and S. Mishra, Lewis Acid Catalyst System for Claisen-Schmidt Reaction under Solvent Free Condition, Tetrahedron Lett., 2020, 61, 152175 CrossRef CAS.
- C. Zhuang, W. Zhang, C. Sheng, W. Zhang, C. Xing and Z. Miao, Chalcone: A Privileged Structure in Medicinal Chemistry, Chem. Rev., 2017, 117, 7762–7810 CrossRef CAS PubMed.
- M. Gomes, E. Muratov, M. Pereira, J. Peixoto, L. Rosseto, P. Cravo, C. Andrade and B. Neves, Chalcone Derivatives: Promising Starting Points for Drug Design, Molecules, 2017, 22, 1210 CrossRef PubMed.
- N. Singh, N. Kumar, G. Rathee, D. Sood, A. Singh, V. Tomar, S. Dass and R. Chandra, Privileged Scaffold Chalcone: Synthesis, Characterization and Its Mechanistic Interaction Studies with BSA Employing Spectroscopic and Chemoinformatics Approaches, ACS Omega, 2020, 5, 2267–2279 CrossRef CAS PubMed.
- A. A. Farooqui, Phytochemicals, Signal Transduction, and Neurological Disorders, Springer Science & Business Media, 2012 Search PubMed.
- R. Arif, M. Rana, S. Yasmeen, M. S. Khan, M. Abid and M. S. Khan, Facile synthesis of chalcone derivatives as antibacterial agents: Synthesis, DNA binding, molecular docking, DFT and antioxidant studies, J. Mol. Struct., 2020, 1208, 127905 CrossRef.
- G. Wang, W. Liu, Z. Gong, Y. Huang, Y. Li and Z. Peng, Design, synthesis, biological evaluation and molecular docking studies of new chalcone derivatives containing diaryl ether moiety as potential anticancer agents and tubulin polymerization inhibitors, Bioorg. Chem., 2020, 95, 103565 CrossRef CAS PubMed.
- S. N. Kumar, S. R. Bavikar, C. N. S. S. P. Kumar, I. F. Yu and R. J. Chein, From Carbamate to Chalcone: Consecutive Anionic Fries Rearrangement, Anionic Si → C Alkyl Rearrangement, and Claisen-Schmidt Condensation, Org. Lett., 2018, 20, 5362–5366 CrossRef CAS PubMed.
- S. Bentahar, M. A. Taleb, A. Sabour, A. Dbik, M. El Khomri, N. El Messaoudi and A. Lacherai, The Use of Modified Clay as an Efficient Heterogeneous and Ecofriendly Catalyst for the Synthesis of Chalcones via Claisen-Schmidt Condensation, Russ. J. Appl. Chem., 2020, 93, 983–990 CrossRef CAS.
- A. Kumar, S. Sharma, V. D. Tripathi and S. Srivastava, Synthesis of chalcones and flavanones using Julia–Kocienski olefination, Tetrahedron, 2010, 66, 9445–9449 CrossRef CAS.
- M. Mellado, A. Madrid, Ú. Martínez, J. Mella, C. Salas and M. Cuellar, Hansch's analysis application to chalcone synthesis by Claisen–Schmidt reaction based in DFT methodology, Chem. Pap., 2018, 72, 703–709 CrossRef CAS.
- Y. Ouyang, J. Li, X. Chen, X. Fu, S. Sun and Q. Wu, Chalcone Derivatives: Role in Anticancer Therapy, Biomolecules, 2021, 11, 894 CrossRef CAS PubMed.
- S. Majumdar, S. Basak, C. Lungu, M. Diudea and G. Grunwald, Applications of Multidimensional Space of Mathematical Molecular Descriptors in Large-Scale Bioactivity and Toxicity Prediction- Applications to Prediction of Mutagenicity and Blood-Brain Barrier Entry of Chemicals, Mol. Inf., 2019, 38, 1800164 CrossRef CAS PubMed.
- S. Das, B. Porashar, S. Saikia and R. Borah, Anticancer Activity of Natural and Synthetic Chalcones, ChemistrySelect, 2020, 5, 3041–3047 CrossRef CAS.
- M. Das and K. Manna, Chalcone Scaffold in Anticancer Armamentarium: A Molecular Insight, J. Toxicol., 2016, 2016, 7651047 Search PubMed.
- F. Gao, G. Hung and J. Xiao, Chalcone hybrids as potential anticancer agents: Current development, mechanism of action, and structure-activity
relationship, Med. Res. Rev., 2020, 40, 2049–2084 CrossRef CAS PubMed.
- T. Constantinescu and C. N. Lungu, Anticancer Activity of Natural and Synthetic Chalcones, Int. J. Mol. Sci., 2021, 22, 11306 CrossRef CAS PubMed.
- A. Rammohan, J. S. Reddy, G. Sravya, C. N. Rao and G. V. Zyryanov, Chalcone synthesis, properties and medicinal applications: a review, Environ. Chem. Lett., 2020, 18, 433–458 CrossRef CAS.
- E. H. Zhang, R. F. Wang, S. Z. Guo and B. Liu, An Update on Antitumor Activity of Naturally Occurring Chalcones, J. Evidence-Based Integr. Med., 2013, 2013, 815621 Search PubMed.
- Z. Rozmer and P. Perjési, Naturally occurring chalcones and their biological activities, Phytochem. Rev., 2014, 15, 87–120 CrossRef.
- G. Padmavathi, S. R. Rathnakaram, J. Monisha, D. Bordoloi, N. K. Roy and A. B. Kunnumakkara, Potential of butein, a tetrahydroxychalcone to obliterate cancer, Phytomedicine, 2015, 22, 1163–1171 CrossRef CAS PubMed.
- S. Di, C. Fan, Z. Ma, M. Li, K. Guo, D. Han, X. Li, D. Mu and X. Yan, PERK/eIF-2α/CHOP Pathway Dependent ROS Generation Mediates Butein-induced Non-small-cell Lung Cancer Apoptosis and G2/M Phase Arrest, Int. J. Biol. Sci., 2019, 15, 1637–1653 CrossRef CAS PubMed.
- K. L. Wang, Y. C. Yu and S. M. Hsia, Perspectives on the Role of Isoliquiritigenin in Cancer, Cancers, 2021, 13, 115 CrossRef CAS PubMed.
- K. Li, Q. Zheng, X. Chen, Y. Wang, D. Wang and J. Wang, Isobavachalcone Induces ROS-Mediated Apoptosis via Targeting Thioredoxin Reductase 1 in Human Prostate Cancer PC-3 Cells, Oxid. Med. Cell. Longevity, 2018, 2018, 1915828 CrossRef PubMed.
- Y. Li, X. Qin, P. Li, H. Zhang, T. Lin, Z. Miao and S. Ma, Isobavachalcone isolated from Psoralea corylifolia inhibits cell proliferation and induces apoptosis via inhibiting the AKT/GSK-3β/β-catenin pathway in colorectal cancer cells, Drug Des., Dev. Ther., 2019, 13, 1449–1460 CrossRef CAS PubMed.
- J. Shi, Y. Chen, W. Chen, C. Tang, H. Zhang, Y. Chen, X. Yang, Z. Xu, J. Wei and J. Chen, Isobavachalcone sensitizes cells to E2-induced paclitaxel resistance by down-regulating CD44 expression in ER+ breast cancer cells, J. Cell. Mol. Med., 2018, 22, 5220–5230 CrossRef CAS PubMed.
- M. A. Aziz, M. S. Sarwar, T. Akter, M. S. Uddin, S. Xun, Y. Zhu, M. S. Islam and Z. Hongjie, Polyphenolic molecules targeting STAT3 pathway for the treatment of cancer, Life Sci., 2021, 268, 118999 CrossRef CAS PubMed.
- C. Karthikeyan, N. S. H. N. Moorthy, S. Ramasamy, U. Vanam, E. Manivannan, D. Karunagaran and P. Trivedi, Advances in chalcones with anticancer activities, Recent Pat. Anti-Cancer Drug Discovery, 2015, 10, 97–115 CrossRef CAS PubMed.
- Y. Ouyang, J. Li, X. Chen, X. Fu, S. Sun and Q. Wu, Chalcone Derivatives: Role in Anticancer Therapy, Biomolecules, 2021, 11, 894 CrossRef CAS PubMed.
- G. Yadav and D. Wagh, Claisen-Schmidt Condensation using Green Catalytic Processes: A Critical Review, ChemistrySelect, 2020, 5, 9059–9085 CrossRef CAS.
- M. Das and K. Manna, Chalcone Scaffold in Anticancer Armamentarium: A Molecular Insight, J. Toxicol., 2016, 2016, 7651047 Search PubMed.
- S. Shenvi, K. Kumar, K. Hatti, K. Rijesh, L. Diwakar and C. Reddy, Synthesis, anticancer and antioxidant activities of 2,4,5-trimethoxy chalcones and analogues from asaronaldehyde: Structure–activity relationship, Eur. J. Med. Chem., 2013, 62, 435–442 CrossRef CAS PubMed.
- L. Bustos, C. Echiburú-Chau, A. Castro-Alvarez, B. Bradshaw, M. J. Simirgiotis, M. Mellado, C. Parra and M. Cuellar, Cytotoxic Effects on Breast Cancer Cell Lines of Chalcones Derived from a Natural Precursor and Their Molecular Docking Analysis, Molecules, 2022, 27, 4387–4397 CrossRef CAS PubMed.
- A. Danova, D. V. Nguyen, R. Toyoda, P. Mahalapbutr, T. Rungrotmongkol, P. Wonganan and W. Chavasiri, 3′, 4′, 5′-trimethoxy-and 3,4-dimethoxychalcones targeting A549 cells: Synthesis, cytotoxic activity, and molecular docking, J. Mol. Struct., 2023, 1275, 134572–134579 CrossRef CAS.
- A. Zołnierczyk, D. Baczńska, B. Potaniec, J. Kozłowska, M. Grabarczyk, E. Woźniak and M. Anioł, Antiproliferative and antioxidant activity of xanthohumol acyl derivatives, Med. Chem. Res., 2017, 26, 1764–1771 CrossRef.
- J. Popłoński, E. Turlej, S. Sordon, T. Tronina, A. Bartmańska, J. Wietrzyk and E. Huszcza, Synthesis and Antiproliferative Activity of Minor Hops Prenylflavonoids and New Insights on Prenyl Group Cyclization, Molecules, 2018, 23, 776 CrossRef PubMed.
- L. F. Castaño, V. Cuartas, A. Bernal, A. Insuasty, J. Guzman, O. Vidal, V. Rubio, G. Puerto, P. Lukác, V. Vimbarg, G. Balíková-Novtoná, L. Vannucci, J. Janata, J. Quiroga, R. Abonia, M. Nogueras, J. Cobo and B. Insuasty, New chalcone-sulfonamide hybrids exhibiting anticancer and antituberculosis activity, Eur. J. Med. Chem., 2019, 176, 50–60 CrossRef PubMed.
- S. Burmaoglu, S. Ozcan, S. Balcioglu, M. Gencel, S. A. A. Noma, S. Essiz, B. Attes and O. Algul, Synthesis, biological evaluation and molecular docking studies of bischalcone derivatives as xanthine oxidase inhibitors and anticancer agents, Bioorg. Chem., 2019, 91, 103149 CrossRef PubMed.
- Z. Li, M. Tian, J. Ma, S. Xia, X. Lv, P. Xia, X. Xu, Y. Jiang, J. Wang and Z. Li, Synthesis and biological evaluation of bis-chalcone conjugates containing lysine linker as potential anticancer agents, J. Mol. Struct., 2023, 1288, 135785–135793 CrossRef CAS.
- M. Santos, V. Pinhanelli, M. Garcia, G. Silva, S. Baek, S. França, A. Fachin, M. Marins and L. Regasini, Antiproliferative and pro-apoptotic activities of 2′- and 4′-aminochalcones against tumor canine cells, Eur. J. Med. Chem., 2017, 138, 884–889 CrossRef CAS PubMed.
- J. Kozłowska, B. Potaniec, D. Baczńska, B. Zarawska and M. Anioł, Synthesis and Biological Evaluation of Novel Aminochalcones as Potential Anticancer and Antimicrobial Agents, Molecules, 2019, 24, 4129 CrossRef PubMed.
- G. Wang, Z. Peng, J. Zhang, J. Qiu, Z. Xie and Z. Gong, Synthesis, biological evaluation and molecular docking studies of aminochalcone derivatives as potential anticancer agents by targeting tubulin colchicine binding site, Bioorg. Chem., 2018, 78, 332–340 CrossRef CAS PubMed.
- M. A. A. Fathi, A. A. Abd El-Hafeez, D. Abdelhamid, S. H. Abbas, M. M. Montano and M. Abdel-Aziz, 1,3,4-oxadiazole/chalcone hybrids: Design, synthesis, and inhibition of leukemia cell growth and EGFR, Src, IL-6 and STAT3 activities, Bioorg. Chem., 2019, 84, 150–163 CrossRef CAS PubMed.
- M. M. Hawash, D. C. Kahraman, F. Eren, R. C. Atalay and S. N. Baytas, Synthesis and biological evaluation of novel pyrazolic chalcone derivatives as novel hepatocellular carcinoma therapeutics, Eur. J. Med. Chem., 2017, 129, 12–26 CrossRef CAS PubMed.
- H. S. Abd ElMonaem, N. I. Abdel-Aziz, M. A. Morsy, F. A. Badria, F. ElSenduny, M. B. El-Ashmawy and M. A. Moustafa, Synthesis and biological evaluation of novel pyrazolic chalcone derivatives as novel hepatocellular carcinoma therapeutics, J. Appl. Pharm. Sci., 2018, 8, 075–087 CAS.
- Shaveta, S. Mishra and P. Singh, Hybrid molecules: The privileged scaffolds for various pharmaceuticals, Eur. J. Med. Chem., 2016, 124, 500–536 CrossRef CAS PubMed.
- L.-S. Feng, Z. Xu, L. Chang, C. Li, X.-F. Yan, C. Gao, C. Ding, F. Zhao, F. Shi and X. Wu, Hybrid molecules with potential in vitro antiplasmodial and in vivo antimalarial activity against drug-resistant Plasmodium falciparum, Med. Res. Rev., 2020, 40, 931–971 CrossRef CAS PubMed.
- B. Meunier, Hybrid Molecules with a Dual Mode of Action: Dream or Reality?, Acc. Chem. Res., 2008, 41, 69–77 CrossRef CAS PubMed.
- F. Gao, G. Hung and J. Xiao, Chalcone hybrids as potential anticancer agents: Current development, mechanism of action, and structure-activity relationship, Med. Res. Rev., 2020, 40, 2049–2084 CrossRef CAS PubMed.
- P. Sharma, C. LaRosa, J. Antwi, R. Govindarajan and K. Werbovetz, Imidazoles as Potential Anticancer Agents: An Update on Recent Studies, Molecules, 2021, 26, 4213 CrossRef CAS PubMed.
- A. Siwach and P. K. Verma, Synthesis, therapeutic potential of imidazole containing compounds, BMC Chem., 2021, 15, 12 CrossRef CAS PubMed.
- M. Hossain and A. K. Nanda, A Review on Heterocyclic: Synthesis and Their Application in Medicinal Chemistry of Imidazole Moiety, Sci. J. Chem., 2018, 6, 83–94 CrossRef CAS.
- S. R. Oskuei, S. Mirzaei, M. R. Jafari-Nik, F. Hadizadeh, F. Eisvand, F. Mosaffa and R. Ghodsi, Design, synthesis and biological evaluation of novel imidazole-chalcone derivatives as potential anticancer agents and tubulin polymerization inhibitors, Bioorg. Chem., 2021, 112, 104904 CrossRef PubMed.
- Y. Wang, S. Xue, R. Li, Z. Zheng, H. Yi and Z. Li, Synthesis and biological evaluation of novel synthetic chalcone derivatives as anti-tumor agents targeting Cat L and Cat K, Bioorg. Med. Chem., 2018, 26, 8–16 CrossRef CAS PubMed.
- Y. Zou, L. Liu, J. Liu and G. Liu, Bioisosteres in Drug Discovery: Focus on Tetrazole, Future Med. Chem., 2020, 12, 91–93 CrossRef CAS PubMed.
- H. Abd ElMonaem, N. Abdel-Aziz, M. Morsy, F. Badria, F. ElSenduny, M. El-Ashmawy and M. Moustafa, Synthesis, in vitro antiproliferative evaluation and molecular docking of new tetrazole-chalcone, tetrazole-pyrazoline hybrids, J. Appl. Pharm. Sci., 2018, 8, 75–87 CAS.
- S. Ke, Z. Zhang, L. Shi, M. Liu, W. Fang, Y. Zhang, Z. Wu, Z. Wan, T. Long and K. Wang, An efficient synthesis and bioactivity evaluation of oxazole-containing natural hinduchelins A–D and their derivatives, Org. Biomol. Chem., 2019, 17, 3635–3639 RSC.
- Y. Li, Y. Sun, Y. Zhou, X. Li, H. Zhang and G. Zhang, Discovery of orally active chalcones as histone lysine specific demethylase 1 inhibitors for the treatment of leukaemia, J. Enzyme Inhib. Med. Chem., 2021, 36, 207–217 CrossRef CAS PubMed.
- M. J. Naim, O. Alam, F. Nawaz, M. J. Alam and P. Alam, Current status of pyrazole and its biological activities, J. Pharm. BioAllied Sci., 2016, 8, 2–17 CrossRef CAS PubMed.
- N. Abdelgawad, M. Ismail, M. Hekal and M. J. Marzouk, Design, synthesis, and evaluation of some novel heterocycles bearing pyrazole moiety as potential anticancer agents, Heterocycl. Chem., 2019, 56, 1771–1779 CrossRef CAS.
- M. Ahmed, E. Santali and R. El-Haggar, Novel piperazine-chalcone hybrids and related pyrazoline analogues targeting VEGFR-2 kinase; design, synthesis, molecular docking studies, and anticancer evaluation, J. Enzyme Inhib. Med. Chem., 2021, 36, 307–318 Search PubMed.
- C. C. Prasad and P. V. S. Machiraju, Synthesis and characterization of some novel aryl and heteroaryl chalcone derivatives of 3-(3,4,5-trimethoxyphenyl)-1-phenyl-1H-pyrazole-4-carbaldehyde for assessing their potentials as anticancer agents, Russ. J. Gen. Chem., 2017, 87, 2056–2066 CrossRef.
- M. M. A. Hawash, D. C. Kahraman, F. Eren, R. C. Atalay and S. N. Baytas, Synthesis and biological evaluation of novel pyrazolic chalcone derivatives as novel hepatocellular carcinoma therapeutics, Eur. J. Med. Chem., 2017, 129, 12–26 CrossRef CAS PubMed.
- A. Ayati, S. Emami, A. Asadipour, A. Shafiee and A. Foroumadi, Recent applications of 1,3-thiazole core structure in the identification of new lead compounds and drug discovery, Eur. J. Med. Chem., 2015, 97, 699–718 CrossRef CAS PubMed.
- T. Italo da Santana, M. Barbosa, P. A. T. Gomes, A. C. Nascimento da Cruz, T. Gonçalves da Silva and A. C. T. Leite, Synthesis, anticancer activity and mechanism of action of new thiazole derivatives, Eur. J. Med. Chem., 2018, 144, 874–886 CrossRef PubMed.
- Anuradha, S. Patel, R. Patle, P. Parameswaran, A. Jain and A. Shard, Design, computational studies, synthesis and biological evaluation of thiazole-based molecules as anticancer agents, Eur. J. Pharm. Sci., 2019, 134, 20–30 CrossRef CAS PubMed.
- V. R. Suma, R. Sreenivasulu, M. V. B. Rao, M. Subramayam, M. J. Ahsan, R. Alluri and K. R. M. Rao, Design, synthesis, and biological evaluation of chalcone-linked thiazole-imidazopyridine derivatives as anticancer agents, Med. Chem. Res., 2020, 29, 1643–1654 CrossRef CAS.
- T. Farghaly, G. Masaret, Z. Muhammad and M. Harras, Discovery of thiazole-based-chalcones and 4-hetarylthiazoles as potent anticancer agents: Synthesis, docking study and anticancer activity, Bioorg. Chem., 2020, 98, 103761 CrossRef CAS PubMed.
- N. Gurrapu, E. P. Kumar, P. K. Kolluri, S. Putta, S. K. Sivan and N. J. P. Subhashini, Synthesis, biological evaluation and molecular docking studies of novel 1,2,3-triazole tethered chalcone hybrids as potential anticancer agents, J. Mol. Struct., 2020, 1217, 128356 CrossRef CAS.
- F. Ahmed, A. A. A. El-Hafeez, S. H. Abbas, D. Abdelhamid and M. Abdel-Aziz, New 1,2,4-triazole-Chalcone hybrids induce Caspase-3 dependent apoptosis in A549 human lung adenocarcinoma cells, Eur. J. Med. Chem., 2018, 151, 705–722 CrossRef CAS PubMed.
- A. Mohassab, H. Hassan, D. Abdelhamid, B. Youssif, H. Tateishi, M. Fujita, M. Otsuka and M. Abdel-Aziz, Design and synthesis of novel quinoline/chalcone/1,2,4-triazole hybrids as potent antiproliferative agent targeting EGFR and BRAFV600E kinases, Bioorg. Chem., 2021, 106, 104510 CrossRef CAS PubMed.
- A. M. Alsina-Sánchez, S. Montalvo-Vázquez, N. Grafals-Ruiz, C. Acosta, E. M. Ormé, I. Rodríguez, S. M. Delgado-Rivera, A. D. Tinoco, S. Dharmawardhane and I. C. Montes-González, Synthesis of Novel Heterocyclic Ferrocenyl Chalcones and Their Biological Evaluation, ACS Omega, 2023, 38, 34377–34387 Search PubMed.
- H. V. Chavan, S. D. Ganapure, N. N. Mali and P. S. Bhale, Synthesis, characterization and biological evaluation of N-substituted indolyl chalcones as anticancer, anti-inflammatory and antioxidant agents, Mater. Today: Proc., 2023, 73, 396–402 Search PubMed.
- E. Erturk, G. Tuna, D. Coskun and F. Ari, Investigation of Anti-Cancer Activity of Newly Synthesized 2,4-pentadien-1-one Derivative Containing Benzofuran in Human Lung and Colon Cancer Cells, EJMO, 2023, 7, 24–33 Search PubMed.
- M. H. El-Wakil, S. N. Khattab, A. F. El-Yazbi, N. El-Nikhely, A. Soffar and H. H. Khalil, New chalcone-tethered 1,3,5-triazines potentiate the anticancer effect of cisplatin against human lung adenocarcinoma A549 cells by enhancing DNA damage and cell apoptosis, Bioorg. Chem., 2020, 105, 104393 CrossRef CAS PubMed.
- Y. Ouyang, J. Li, X. Chen, X. Fu, S. Sun and Q. Wu, Chalcone Derivatives: Role in Anticancer Therapy, Biomolecules, 2021, 11, 894 CrossRef CAS PubMed.
Footnote |
† Equal contribution. |
|
This journal is © The Royal Society of Chemistry 2025 |
Click here to see how this site uses Cookies. View our privacy policy here.