DOI:
10.1039/D4RA08604J
(Review Article)
RSC Adv., 2025,
15, 12402-12442
Herbal bioactive-loaded biopolymeric formulations for wound healing applications
Received
6th December 2024
, Accepted 1st April 2025
First published on 17th April 2025
Abstract
Recent advancements in wound healing technologies focus on incorporating herbal bioactives into biopolymeric formulations. A biocompatible matrix that promotes healing is provided by biopolymeric wound dressings. These dressings use components such as ulvan, hyaluronic acid, starch, cellulose, chitosan, alginate, gelatin, and pectin. These natural polymers assist in three crucial processes, namely, cell adhesion, proliferation, and moisture retention, all of which are necessary for effective wound repair. Curcumin, quercetin, Aloe vera, Vinca alkaloids, and Centella asiatica are some of the herbal bioactives that are included in biopolymeric formulations. They have powerful anti-inflammatory, antibacterial, and antioxidant activities. Chitosan, cellulose, collagen, alginate, and hyaluronic acid are some of the biopolymers that have shown promise in clinical trials for wound healing. These trials have also confirmed the safety and functional performance of these materials. Their recent advancements in wound care can be understood by the increasing number of patents linked to these formulations. These innovative dressings improve healing outcomes in acute and chronic wounds while minimizing adverse effects by incorporating biopolymers with herbal bioactives in an efficient manner. This review emphasizes that the development of next-generation wound care products can be facilitated via the integration of natural materials and bioactive substances.
1. Introduction
There are different types of wounds, which are described as breaches in the skin or underlying tissues, and they can range from open to closed, surgical to traumatic, and acute to chronic.1 In contrast to chronic wounds, which are often linked to underlying diseases, such as diabetes or venous insufficiency, acute wounds caused by abrasions or lacerations heal predictably; chronic wounds can take longer to heal because of chronic inflammation or poor perfusion.2 The biological reaction to injury or wound healing is intricate and multi-staged, including the stages of hemostasis, inflammation, proliferation, and remodeling. Factors such as the patient's age, nutritional status, comorbidities, and genetic predispositions play major roles in wound healing.3 These factors impact cellular responses and tissue regeneration. Important extrinsic factors that might help or hinder healing include the local wound environment, moisture balance, infection control, and the use of proper dressings. Stress and mental health status are two examples of psychological elements that can have an indirect impact on healing through their effects on immunological function and general physiological resilience. Healthcare providers cannot improve wound care, increase patient satisfaction, and decrease healthcare expenditures related to chronic wound management without having a thorough comprehension of the complex relationship between various wound types and different factors impacting healing.4
Wound healing is a multifaceted biological process that occurs in response to injury to the body's structure and physiology. It encompasses several cellular and molecular mechanisms, both intracellular and extracellular, working together to accelerate the recovery of the damage.5,6 It generally occurs in four major phases: hemostasis, inflammation (0 to 3 days), proliferation (2 to 12 days) and remodeling (3 to 6 months) (Fig. 1).7 The two important types of wounds are acute (surgical, mechanical, thermal or chemical wounds) and chronic (bed-sores, ischemia, venous stasis disorder, pressure or diabetic ulcers).8,9 In the wound healing process, wound dressings have crucial roles, mainly in the chronic wounds that heal slowly.10 Various properties such as non-toxic, protective, good absorption, and oxygen permeability properties are essential for wound healing as they prevent contamination and trauma and hasten the healing process.11 Various medicinal systems (Ayurveda, Unani, Chinese, Siddha, etc.) are useful to treat skin damage.12 For example, the seeds of Moringa oleifera are very popular due to their pharmacological and nutritional constituents, which contain all the vital phytochemical constituents necessary for wound healing.13 Traditionally, wound dressing with herbal products (extract of plant/animal) plays an essential role in the development of modern wound healing.14 Modern dressings consist of both synthetic and natural polymers (alginate, chitosan, starch, silicone and hydrocolloid gels, etc.) to boost the healing process because of the great anti-microbial-, anti-bacterial-, and growth factor (GF) properties.15–17 Smart wound dressings (biopolymers + nanoparticles) provide numerous opportunities such as enhancing the wound healing by the drug delivery system (DDS), mimicking the lost natural intrinsic environment.18 Active dressings are one of the trending modern wound healings that shows non-toxic, biodegradable, and biocompatible behaviours to fight against infections.19 They have anti-oxidant properties that prevent extreme oxidation and regulate inflammation to support wound healing.20 Hydrogels are promising wound dressing materials with inter-connected porous structures that transfer oxygen and moisture vapor to simulate the physical and chemical properties of tissues and absorb liquid fluids.21
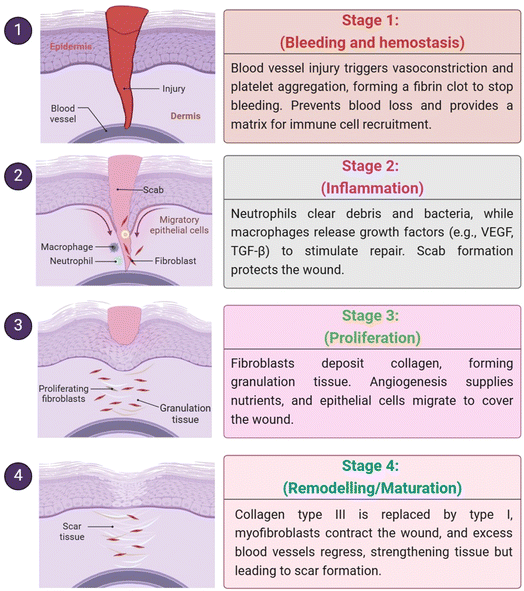 |
| Fig. 1 Four major phases of wound healing. | |
In addition to the capacity to develop gels when exposed to fluid, the greater the number of pores in porous dressings, the greater the rate of cell proliferation and tissue regeneration.22 In underdeveloped nations, primarily in Africa and Asia, 70–80% of the population relies entirely on herbal therapy for various ailments, including wounds, metabolic disorders, and infectious infections. Consequently, traditional remedies primarily sourced from natural items (flora, fauna, sea organisms, and microorganisms) constitute a significant component of wound care for millions worldwide.23,24 Natural polymers are increasingly significant because of their resource availability, compatibility, cost-effectiveness, and biodegradable properties. Nanofibers (NFs) are optimal materials for wound healing because of their exceptional properties, including porosity, surface-volume ratio, mechanical characteristics, and permeability.25,26
The management of chronic wounds, especially diabetic foot ulcers (DFUs), requires a thorough, multidisciplinary strategy because of their intricate characteristics and extended healing durations.27 The initial assessment involves measuring wound size, depth, and exudate levels, succeeded by essential debridement methods, including sharp, enzymatic, or autolytic debridement to eliminate non-viable tissue and foster an optimal healing environment.28,29 The use of advanced dressings, such as foam dressings, hydrocolloids, and alginates, is essential for preserving moisture balance, absorbing exudate, and preventing infection.30 Offloading treatments are crucial for diabetic foot ulcers, employing customized footwear or whole contact casting to reduce pressure on ulcerated regions. Infection therapy may necessitate the use of local or systemic antibiotics, particularly in instances of osteomyelitis.31 Furthermore, managing underlying comorbidities via glycemic regulation and nutritional enhancement is essential for improving healing results. Interdisciplinary teamwork among healthcare professionals, such as endocrinologists, podiatrists, and nutritionists, facilitates comprehensive management, while patient education on foot care and self-monitoring contributes to the prevention of recurrence.32 This methodical, evidence-based methodology is essential for efficient chronic wound treatment and enhancing patient's quality of life. In this review article, various herbal bioactives loaded with biopolymers and its formulation are discussed for wound healing applications.
2. Biopolymeric wound dressing
Biopolymers are polymers produced by living organisms that are made up of long chains of monomers (joined by polymerization), e.g., alginate (ALG), chitosan (Cs), collagen (Col), and silk fibroin (SF).33 These polymers have advantages over synthetic materials due to their biocompatibility, biodegradability, low antigenicity and reproducibility. They can exert antibacterial, anti-inflammatory, and proliferative effects or other targeted effects on specific cells to play important roles in the healing process.34 Wound dressings (bandages) are used to cover a wound by adhering to the surrounding skin with wound dressing tape or glue. Wound dressings can be gel (hydrogel), foam, gauze or any other type of wound dressing patch. They aid in the prevention of infection, the promotion of healing, and the alleviation of pain.35,36 The formulation of wound healing products entails the amalgamation of biocompatible materials to produce hydrogels, scaffolds, or nanogels that facilitate tissue regeneration, inhibit infection, and improve healing. Hydrogels are often synthesized from natural polymers like collagen, chitosan, or alginate, which are crosslinked by physical or chemical techniques to form water-saturated networks.37 These hydrogels can be infused with antibacterial drugs, growth factors, or stem cells to promote healing and manage infections. Scaffolds are constructed from polymers such as chitosan or polycaprolactone, frequently integrated with nanoparticles and bioactive substances to enhance mechanical strength, porosity, and bioactivity, and are generally produced using methods like 3D bioprinting or electrospinning. Nanogels are diminutive, crosslinked polymer networks that are utilized to encapsulate pharmaceuticals or bioactive substances for regulated distribution, facilitating enhanced penetration into wound areas for targeted treatment (Fig. 2). These formulations can be augmented with supplementary bioactive agents, such growth factors, antibacterial agents, or anti-inflammatory chemicals, facilitating expedited healing and minimizing problems. The ultimate wound healing products undergo testing for mechanical characteristics, biocompatibility, and therapeutic efficacy before clinical application.38 The summarized forms of various biopolymeric materials and their efficacy in wound healing applications, including their formulation, are provided in Table 1.
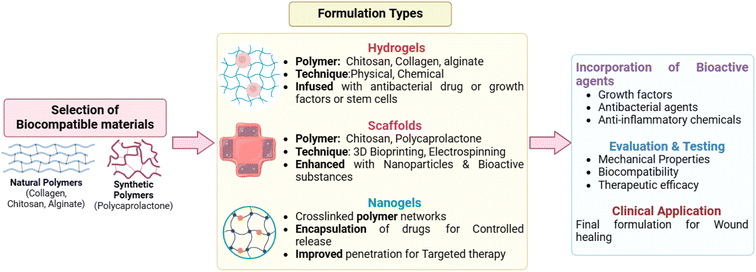 |
| Fig. 2 Bioactive polymer-based formulations for wound repair. | |
Table 1 Summary of biopolymeric materials, and their efficacy in wound healing applications
Biopolymer |
Formulation |
Discussion |
Reference |
Chitosan (Ch) + acid soluble collagen (ASC) |
Collagen–chitosan films (CChF) |
CChF-treated rats showed a 95.75% ± 2.28% decrease in wound diameter, significantly higher than the control (22.25% ± 2.45%), CF (63.25% ± 2.08%), and ChF (52.67 ± 1.58%) groups. Higher hydroxyproline content (48.82 ± 1.25 mg g−1) further supported wound healing efficacy |
39 |
Ch + zein-methyl cellulose + curcumin (ZeinMCNPs) |
Ch/ZeinMCNPs nanocomposite 1–3 films |
Ch/ZeinMCNPs2 and Ch/ZeinMCNPs3 films showed 96% and 98% wound contraction, with reduced inflammation, improved re-epithelization, neovascularization, and increased collagen deposition. Higher SOD and lower MDA levels confirmed enhanced wound healing |
40 |
Ulvan + chitosan + dopamine (DPA), silver nanoparticles (Ag NPs), human umbilical cord mesenchymal stem cell lyophilized powder (hUC-MSCs) |
UC–DPA–Ag@hUC-MSC hydrogel |
The UC–DPA–Ag@hUC-MSC hydrogel significantly accelerated wound healing in a type II diabetic mouse model, promoting cell proliferation, migration, and effective wound closure. It demonstrated strong antioxidant and antibacterial activity, and enhanced mechanical properties, making it a promising material for chronic diabetic wound management |
41 |
Pectin (TFP), polyethylene glycol (PEG), montmorillonite (MMT), neomycin sulfate |
Optimized nanocomposite film (ONCF) |
The nanocomposite film showed no cytotoxicity (90% + C6 glioma cell survival), significant antioxidant activity, and enhanced in vitro wound healing. In vivo studies confirmed its efficacy in wound healing, making it a promising biomedical material |
42 |
Polyvinyl alcohol (PVA) + tapioca pearl starch + α-terpineol |
Electron beam crosslinked PVA/tapioca starch hydrogel |
The α-terpineol-loaded PVA/tapioca starch hydrogel accelerated wound closure, promoted re-epithelialization, collagen and keratin deposition, and stimulated angiogenesis in full-thickness acid burn wounds. Histological analysis showed significant healing, including partial restoration of skin appendages, over 30 days. The hydrogel demonstrated good biocompatibility with 90% fibroblast viability and no inflammatory response in vivo |
43 |
Komagataeibacter xylinus (K1G4) or K. rhaeticus (K2G46), Lacticaseibacillus casei UMCC 2535 (HA-producer), BNC (bacterial nanocellulose) |
BNC–HA nanocomposites (C1–K1 and C2–K2) |
The BNC–HA nanocomposites exhibited enhanced crystallinity, increased mechanical strength, higher moisture uptake, and water absorption compared to pure BNC. They were non-cytotoxic with >90% cell viability and promoted complete wound closure within 48 hours in scratch assays |
44 |
Chitosan + polyherbal extracts (Aloe vera, Azadirachta indica, Alternanthera brasiliana), silver nanoparticles (AgNPs) |
Polyherbal hydrogel integrated with AgNPs |
The hydrogel significantly reduced wound size within 12 days, exhibited higher angiogenic potential, and showed strong antimicrobial activity against E. coli and S. aureus. It also demonstrated anti-inflammatory effects with reduced IL-6 and TNF-α levels, supporting its potential for enhanced wound healing |
45 |
Sodium alginate + allantoin, calcium chloride, citric acid |
Enhanced alginate dressing |
The S2 alginate dressing showed improved water absorption (363–442%), tensile strength (44.90–55.19 MPa), 52.71% cell migration, and 86.6% wound healing rate in mice, with low cytotoxicity and good biocompatibility |
46 |
Chitosan + gum kondagogu (GKG), zinc oxide nanoparticles (ZnO NP), barbaloin (BB) |
CS/GKG/BB-3 biocomposite film |
The CS/GKG/BB-3 biocomposite film exhibited excellent mechanical strength (8.9 ± 0.30 MPa), high water absorption (451.1% ± 6.02%), strong antioxidant and antimicrobial activity, and facilitated rapid re-epithelialization in vivo. It promoted tissue regeneration with minimal scarring, offering a superior alternative to traditional wound dressings for effective wound care and tissue repair |
47 |
Gelatin + oxidized lignosulfonate (OLS) |
Gelatin/OLS wound dressing |
The gelatin/OLS wound dressing significantly enhanced wound healing in vivo, as evidenced by improved re-epithelialization, collagen formation, reduced inflammation, and increased blood vessel density, outperforming untreated wounds |
48 |
Oxidized carboxymethyl cellulose (OCMC), gelatin, polyvinyl alcohol (PVA) |
Hybrid nanofibers |
The OCMC/PVA–gelatin nanofibers showed 99.9% antibacterial activity, excellent biocompatibility (91–92% cell viability), and full degradation in 21 days, with promising applications for advanced wound healing |
49 |
Sodium alginate + Mentha aquatica (MA) methanol extract |
Hydrogel |
The SA/MA hydrogel exhibited effective antibacterial activity with an MIC of 12.5 mg mL−1. In vivo studies showed faster tissue regeneration, enhanced collagen recovery, and bacterial infection eradication in deep third-degree burn wounds, highlighting its potential as a promising wound dressing for infected and injured skin tissue |
50 |
Bioactive properties such as antimicrobial, immune modulatory and angiogenic of the biopolymers create a microenvironment favourable for the healing process and contribute in the development of new systems based on nanotechnology for successful skin creation in chronic wounds.51,52 The functional and structural characteristics of biopolymers can be improved to meet current wound care demands such as tissue repair, restoration of lost tissue integrity, and scarless healing due to technological advances in material science, regenerative medicine and bioengineering (scaffolds).53,54
2.1 Collagen
Collagen (mammalian protein) is a triple helix of collagen fibrils, with each fibril being a repeating polymer of amino acids linked by peptide bonds.55 It can be extracted from cultures of Clostridium histolyticum and is commonly used in therapies to remove cellular debris and extracellular tissue necrosis.56 MicroRNA-mediated MMP-2 (matrix metallopeptidase 2) upregulation creates a collagenolytic environment within the wound, significantly reducing the ratio of collagen I to collagen III, comprising the biomechanical properties of the repaired skin and repairing it. It may leave the affected skin vulnerable to wound recurrence and increase fibroblast production.57 Because of its chemotactic properties, it attracts fibroblasts to the wound site. Collagen promotes the creation of new blood vessels, granulation tissue, wound debridement, and the ability of the wound to re-epithelize.58 An injectable hydrogel was developed by Kim et al. (2022) by enzymatically cross-linking tyramine, alginate, and collagen using hydrogen peroxide and horseradish peroxidase. The potential for tissue regeneration was demonstrated by the 3D-bioprinted structures, which exhibited considerably greater cell proliferation and vitality (92.13% ± 0.70%) compared with ALG-TYR alone (68.18% ± 3.73%).59 Similar to this, Wang et al. (2023) successfully eliminated bacterial infections by conjugating antimicrobial peptides with cypates and recombinant collagen-III to form a multifunctional hydrogel dressing. This dressing exemplifies the multipurpose properties of advanced hydrogels in tissue repair and infection management; it combines collagen, oxygen-carrying liposomes, and antimicrobial peptides to improve wound healing and fight multidrug-resistant infections in chronic wounds.59,60 Yang et al. (2022), developed composite bioinks made of gelatin methacryloyl-recombinant human type III collagen (rhCol3). rhCol3-free bioinks fail to improve cell proliferation or more confluent spreading of epidermal keratinocytes compared with rhCol3-containing bioinks. Additionally, rhCol3 sped up skin wound healing in in vivo testing, which means it could be a useful bioink component for skin tissue engineering.61 For 3D bioprinting bi-layer skin structures with fibroblasts and keratinocytes, Jiao et al. (2023) also investigated the biological properties of collagen/sodium alginate (Col/SA) and 1% collagen hydrogels. Their findings revealed improved cell spreading and proliferation, with Col/SA hydrogels showing the essential tunability for skin bioprinting with wound healing applications as bioinks.61,62 The application of collagen-based formulations has been the primary focus of recent biomaterials research with the aim of enhancing wound healing and tissue regeneration. A non-denatured type I collagen (YCI) with a melting point of 42.7 °C was isolated from yak hide by Fu et al. (2023). The capacity of YCI to restore collagen integrity and promote skin regeneration was proven in a sunburn mouse model, where therapy considerably reduces the presence of denatured collagen in the skin. In addition to improving wound healing in burnt tissue, the non-denatured YCI shows enhanced biocompatibility and bioactivity.63 At the same time, Kumar et al. (2024) synthesized a variety of NPs based on food-grade biopolymers, such as polylactic acid, collagen, chitosan, and alginate, which have antibacterial and wound-healing properties. This biopolymer therapy accelerates the healing process by stimulating tissue regeneration and reducing the amount of time it takes for wounds to close. These studies highlight the potential of collagen-based materials to enhance wound healing by repairing and regenerating tissues, whether in their native form or as composites.63,64
2.2 Cellulose
Cellulose (insoluble dietary fibre) found in plant cell walls is composed of repeating units of β-D-glucose linked by β-1,4-glycosidic bonds and is produced by bacteria belonging to Acetobacter, Sarcina ventriculi and Agrobacterium genera.65,66 Cellulose has the chemical formula (C6H10O5)n, where n denotes the degree of polymerization and the quantity of glucose groups.67 It acts as a primary matrix because of its strong structure and mechanical stability, it moisturizes the area around the wound, absorbs excess exudate, tissue repair, prevention of microbial infection, with the ability to stop bleeding and can be used to generate elastic gels with properties such as biocompatibility, low toxicity, and biodegradability to promote wound debridement.68–70 Multifunctional hydrogels and scaffolds with improved therapeutic potential have been developed as a result of recent advances in biomaterials for wound healing. A hydrogel containing mesenchymal stem cell-derived exosomes (MSC-Exos) was developed by Geng et al. (2022) and used to treat chronic diabetic wounds. The hydrogel is composed of carboxyethyl chitosan (CEC) and dialdehyde carboxymethyl cellulose (DCMC). Hydrogels composed of CEC and DCMC possess antibacterial and self-healing characteristics. These hydrogels were produced using Schiff base reactions. The type 1 diabetic rats wound inflammatory microenvironment was much improved and wound healing was expedited by the MSC-Exos@CEC–DCMC hydrogel.71 Similarly, Biranje et al. (2022) used bioprinting technology to develop a three-dimensional (3D) composite scaffold that included cellulose nanofibrils (TCNFs), chitosan, and casein to control bleeding and promote wound healing. The scaffold encourages the development and proliferation of NIH 3T3 fibroblasts, which are important for wound healing. When treated with cellulase, the scaffold shows considerable degeneration, with a weight loss of 80% ± 5%, suggesting that it might be used for fast wound closure.72 Using NIR-responsive CNC, pH-responsive chitosan oligosaccharide, dynamic imine linkages, and temperature-responsive poly(N-isopropylacrylamide), He et al. (2022) developed an intelligent wound dressing based on cellulose nanocrystals. This versatile dressing demonstrates strong anticancer effects, effectiveness against methicillin-resistant Staphylococcus aureus (MRSA) and biofilm, and the ability to improve wound healing by photodynamic and photothermal therapies; thus, it can potentially treat drug-resistant infections. Taken as a whole, these studies highlight the potential of multifunctional biomaterials for improving long-term wound healing, infection prevention, and tissue regeneration.71–73 According to Wang et al. (2025), a hydrogel made of hydroxyapatite (HA) and collagen considerably improves the rate of wound healing and bone regeneration. In vivo rat model experiments show that the hydrogel considerably prevails over control groups, accelerating wound closure to 93.5% in 14 days. Histological examination revealed enhanced skin regeneration and re-epithelialization at the wound location. New bone tissue was regenerated in bone defect models as a result of the HA–collagen hydrogel's stimulation of osteoblast proliferation and bone formation. Cells, especially fibroblasts and osteoblasts, were able to migrate and proliferate in the hydrogel, which helped with cutaneous and skeletal tissue regeneration and repair. The hydrogel could be used for wound healing and bone regeneration at the same time.74 Munhoz et al., 2023 fabricated nanoscale silver compounds (AgNO3), which are incorporated into CMs (bacterial cellulose membrane) for antimicrobial activity in wound healing. AgCM exhibits antibacterial effects in vitro without toxicity. Furthermore, AgCM offers balanced oxidative action, regulated inflammatory profile by reducing IL-1 and increasing IL-10 as well as improved angiogenesis and collagen synthesis in vivo. The results suggest that silver nanoparticles improve CM properties, antibacterial effects, modulate the inflammatory phase and promote healing in skin lesions to treat injuries.75
Novel materials have been developed to improve the therapeutic properties of biopolymeric wound healing formulations and to optimize the distribution of active chemical compounds. Patil and Wairkar (2024) formulated a mupirocin film-forming spray (MUP-FFS) utilizing chitosan and α-cellulose, optimized by the Box–Behnken design to enhance the sprayability and drying time. The MUP-FFS exhibits rapid application and releases 98.066% of mupirocin, indicating enhanced efficacy relative to commercial ointments and mupirocin API. In rat models, the spray markedly improves wound contraction and healing, while efficiently targeting S. aureus and Escherichia coli. This mixture offers a viable therapy for chronic wounds.76 Similarly, Abaza et al. (2024) developed an innovative wound dressing by incorporating curcumin-loaded zein-methylcellulose (ZeinMCNPs) nanofillers into a chitosan matrix. The resultant Ch/ZeinMCNP nanocomposite film displays superior mechanical properties (Young's modulus, elongation, tensile strength) and enhanced antioxidant and antibacterial activity. This nanocomposite film may work as a versatile and effective wound dressing, facilitating wound healing due to its multifunctional characteristics. Collectively, these investigations highlight the future potential of biopolymer-based formulations, ranging from film-forming sprays to nanocomposite films, in improving wound healing therapies through enhanced drug delivery, antibacterial efficacy, and tissue regeneration.77
2.3 Chitosan
Chitosan (fibrous compound) is found in crustaceans, mollusks, and insects and is synthesized by some fungi, composed of N-acetylglucosamine held by β-1,4 bonds.78,79 Chitosan promotes the wound healing process by stimulating inflammatory cells, macrophages, and fibroblasts reduces the inflammation phase, and initiates the proliferative phase earlier in wound healing.80 Chitosan is a promising hemostatic agent for red blood cells and platelets, and it has various applications in medicine, drug delivery, and moisture permeability and in hydrogels and adhesives owing to its antioxidant, antifungal and antimicrobial activities.81,82 Recent research has focused on developing advanced biopolymeric formulations for diabetic wound healing, integrating natural compounds with biocompatible polymers to improve therapeutic effectiveness. Zeng et al. (2023) developed a chitosan-based injectable hydrogel loaded with puerarin (C@P), a traditional Chinese medication, that enhances angiogenesis and suppresses the inflammatory response in diabetic wounds. C@P hydrogel controls the expression of miR-29a and miR-29b1, which in turn controls M1 polarization and pro-inflammatory cytokines (IL-1β and TNF-α) to facilitate wound healing.83 In the same direction, Anushree et al. (2023) synthesized phosphorylated chitosan (PC) and investigated its antioxidant properties, revealing that PC-treated wounds display superior wound contraction (91.11%), elevated superoxide dismutase (SOD) activity, reduced lipid peroxidation, and enhanced tissue morphology, characterized by increased fibroblast activity, collagen deposition, and angiogenesis. These data indicate that PC may serve as a potential agent for the repair of diabetic wounds.84 Le et al. (2023) examined chitosan-based hydrocolloid patches for wound healing, proving that these patches significantly reduce inflammation, inhibit pro-inflammatory cytokines, and facilitate skin regeneration by enhancing fibroblast proliferation and the expression of essential biomarkers (e.g., vimentin, α-SMA, collagen I, and TGF-β1). Collectively, these investigations emphasize the efficacy of chitosan-based formulations independently and in conjunction with bioactive compounds such as puerarin and phosphorylated chitosan in expediting wound healing, mitigating inflammation, and promoting tissue regeneration in diabetic wounds.85 Linju et al., 2023 synthesized and characterized scaffolds of amino acid L-proline conjugated onto chitosan by FTIR and NMR that are characterized by swelling, dissolution, porosity and healing properties. The scaffold shows no cytotoxicity against L929 and HaCaT cells and improves wound healing potential in the L929 cell line, showing 53.35% ± 2.3%, 72.96% ± 2.2%, and 50.89% ± 0.3% wound closure with CS-P 200, 400, and 600, respectively compared to the native CS scaffold. Hence, the modified scaffold promotes collagen deposition, remodeling wound microenvironment and has potential as a wound dressing.86 Moreira et al., 2023 fabricated chitosan (CSF) and pentoxifylline films (PTX) for cutaneous wound healing, evaluating interactions, structural characteristics, in vitro release and morphometric aspects in vivo at two concentrations: F1 (2.0 mg mL−1) and F2 (4.0 mg mL−1). As a result, the release of films was proportional to concentration, with two phases: fast ≤2 h and slow >2 h. After 72 h, F2 shows faster healing, with wound reductions up to 60% on day 2 compared to CSF, F1, and the positive control. Therefore, CSF and PTX effectively form and incorporate, accelerating skin-wound reduction.87
Innovative hydrogels and nanogels possessing antibacterial and wound-healing capabilities have recently been the focus of biomaterial research and development. To aid in the healing of bladder wounds and to prevent urinary tract infections (UTIs), Yang et al. (2024) synthesized a nanogel (NA@CS) out of nalidixic acid and chitosan. The nanogel shows promising inhibitory effects on bacterial strains, lowering their pathogenicity and virulence, and it is well-tolerated by L929 fibroblast cells and an animal model of Artemia salina. The nanogel has potential as a treatment for urinary tract infections and for mending bladder wounds.88 A biomimetic composite bioink was developed for 3D bioprinting by Khoshmaram et al. (2024) using a similar strategy, combining gelatin methacrylate (GelMA) with chitosan nanoparticles (CSNPs). Nanoparticles with curcumin infused into them have better antibacterial and skin cell proliferation capabilities. Applying the composite hydrogel to Wistar rats shows potential for skin tissue engineering and wound healing since it promotes cell division and effectively blocks bacterial infections. Both studies show that advanced biomaterials can heal wounds in the skin and the urinary system, and that they are effective in managing infections and repairing tissues.88,89
2.4 Alginate
Alginate (linear compound) is composed of repeating units of β-D-mannuronic acid and α-L-guluronic acid by α-1,4 glycosidic bonds derived from the sea and is extracted from brown algae such as Laminaria, Macrocystis and Ascophyllum species.90,91 Alginate dressings are light, nonwoven textiles that are developed for moderately to heavily exuding wounds and possess highly absorbent properties, have modest hemostatic qualities, help to minimize bacterial infections, and can be left on the wound bed for days.92 Various properties like nontoxicity, high mechanical strength, abundance, and high adsorption capacity have made the alginate-based polymeric systems a promising material.93 Recent research shows favorable outcomes in the development of improved wound healing solutions utilizing biopolymers and bioactive compounds. Saraiva et al. (2023) reported that sodium alginate (SA) and polyvinyl alcohol (PVA) films, when crosslinked with Ca2+, enhanced their physicochemical and biological properties, thereby augmenting their potential for wound healing through improved drug incorporation.94 By crosslinking alginate, chitosan, and arginine–glycine–aspartate (RGD) with tannic acid (TA), Mndlovu et al. (2023) developed scaffolds that significantly increase the viability of mouse embryonic fibroblast cells and achieve an 86% encapsulation efficiency with a 57% burst release in 24 hours, showing the scaffolds' potential for acute and chronic wound healing.95 Chen et al. (2023) synthesized SCTF cryogels by crosslinking sodium alginate with tannic acid and Fe3+ ions, showing significant hemostatic properties and improved bactericidal effectiveness via synergistic photothermal and chemodynamic mechanisms, thereby facilitating wound healing.96 Cruz Sánchez et al. (2023) formulated chitosan and alginate membranes infused with lavender essential oil (LEO), revealing that the CHT/ALG + LEO membranes display a significant water absorption capacity (638% after 48 hours) with low cytotoxicity and regulated LEO release, rendering them appropriate for wound healing applications.97 Ndlovu et al. (2024) developed SA/PVA/PLGA nanofibers loaded with Capparis sepiaria extract, exhibiting significant antibacterial properties against multiple bacterial species and displaying hemostatic potential, suggesting their possible application as burn wound dressings.98 Sellappan et al. (2024) developed keratin–sodium alginate dressings infused with zinc oxide nanoparticles and herbal products, exhibiting superior antibacterial efficacy against E. coli and Bacillus subtilis, enhances biocompatibility, and increases collagen deposition, emphasizing their potential as antimicrobial wound dressings for enhanced healing. These studies collectively highlight the efficacy of biopolymer-based formulations in promoting wound healing by increasing antibacterial characteristics, biocompatibility, and regenerative potential.99
2.5 Starch
Starch (polysaccharide) is a soft, tasteless powder produced by green plants. It is a granular, organic chemical comprising glucose monomers joined in α-1,4 linkages. The linear polymer amylose is the most basic type of starch, and amylopectin is the branched form.100,101 Starch hydrophilicity absorbs wound exudates, potentially causing bacterial infection, aiding in antimicrobial treatment for chronic wounds with the process of proliferation, differentiation and regeneration of cells.102,103 Starch is an appealing polymer for wound healing applications because of its vast availability, low cost, biocompatibility, and biodegradability.104 Wound dressing polysaccharides such as sago starch help facilitate and promote healing.105 Recent studies have investigated numerous starch-based formulations and composites to improve wound healing capabilities using advanced biopolymeric scaffolds and hydrogels. Guo et al. (2023) engineered microcapsules comprising waxy maize starch (WMS) and modified waxy maize starch (EWMS), which were subjected to α-amylase and transglucosidase treatment to augment their self-healing capabilities. EWMS-16 shows a high degree of branching (21.88%), enhanced healing efficiency (58.33%), and self-healing abilities, exhibiting superior characteristics relative to WMS microcapsules.106 Huang et al. (2023) developed a starch/natural rubber composite hydrogel that has tunable mechanical properties, significant elasticity, fatigue resistance, and self-healing abilities, along with exceptional durability and stability for tracking human movement, indicating its potential for wearable health monitoring applications.107 Lopes et al. (2023) integrated the bioactive compound, porphyrin tetraiodide (TMPyP), into starch-based films, markedly enhancing their antibacterial efficacy and wound healing capacity. These films efficiently photoinactivate E. coli (>99.99%) and accelerate wound healing without light, highlighting TMPyP's potential in developing water-resistant, photosensitive biomaterials for wound care.108 Finally, Joseph et al. (2024) utilized 3D bioprinting to develop scaffolds from gellan gum and starch derived from Maranta arundinacea, exhibiting superior cell survivability and improved performance relative to conventional monolayer cultures. This biocompatible and biodegradable scaffold exhibits potential for long-term wound healing by obstructing environmental pollutants. Collectively, these investigations highlight the adaptability and efficacy of starch-based formulations and composites in enhancing wound healing via superior antibacterial-, self-healing-, and biocompatible characteristics.109 Khalid et al., 2024 investigated the application of hydrogels as a possible remedy for full-thickness acid burn wounds when loaded with functional substances like α-terpineol and starch. The hydrogels encourage angiogenesis, re-epithelialization, the deposition of keratin which results in the partial restoration of skin appendages and the repair of thick dermal and epidermal tissues. As a result, the hydrogels have potential as an economical and effective wound dressing, increasing the usefulness of the sheet hydrogel dressing made of natural polymers.110
2.6 Hyaluronic acid
Hyaluronic acid (hyaluronate) is a naturally produced gooey substance found in the body, particularly in eyes, joints, and skin. It acts as a cushion and lubricant, composed of polymeric disaccharides linked by a glucuronic β (1→3) bond.111,112 It modulates specific HA receptors, inflammation (signaling the body to build more blood vessels in the damaged area), re-epithelization, scar tissue formation (promotes collagen and elastin production), cellular migration and angiogenesis, all of which are important phases of wound healing.113 It has many unique properties including excellent biocompatibility, high viscoelasticity, biodegradability, hydrophilicity, moisture retention capacity and non-immunoreactivity.114
Modern research has focused on developing novel formulations for wound healing that make use of biodegradable substances and bioactive compounds. AlSalem et al. (2023) developed three biodegradable wound dressings utilizing collagen, hyaluronic acid (HA), silver nanoparticles (AgNPs), and gentamicin (GENT) by freeze-drying and assessment of physical properties. The findings revealed enhanced antibacterial efficiency against Gram-positive and Gram-negative bacteria, yeast, and fungi, with COL/HA/AgNPs/GENT displaying the greatest effectiveness in wound healing and antibacterial attributes. The membranes exhibit outstanding swelling characteristics, quick degradability, and cytocompatibility, with the exception of one formulation (COL/HA/AgNPs/GENT), which was considered unsafe for cellular application.115 A multifunctional hydrogel made of oxidized hyaluronic acid (OHA) and gallic acid-grafted quaternized chitosan (GA-QCS) crosslinked by Schiff base chemistry was developed by Bai et al. in 2023. The GA-QCS/OHA hydrogels have injectable characteristics, efficient hemostasis, and regulated drug release, in addition to antioxidant and migration-enhancing activities. These hydrogels accelerate wound healing by suppressing TNF-α and enhancing CD31 expression, demonstrating their potential as an efficient, multifunctional approach for wound management, especially in cases of infection complications. Collectively, these studies illustrate the potential of bioactive and biodegradable wound dressings in improving wound healing via antibacterial-, anti-inflammatory-, and regenerative properties.116 Lin et al., 2023 developed a drug free hybrid hydrogel combining chitosan (CS) and hyaluronic acid (HA) for synergistic healing in MRSA-infected diabetic wounds. The CS/HA hydrogel exhibits broad-spectrum antibacterial activity, fibroblast proliferation, ROS scavenging, and cell-protection and promotes wound healing in diabetic mouse wounds, eliminating MRSA infection and enhancing epidermal regeneration, collagen deposition, and angiogenesis and has potential for clinical use in managing chronic diabetic wounds.117 Eeckhout et al., 2022 established hyaluronic acid gel after alveolar ridge preservation (ARP) in healthy patients showed changes in wound dimensions over time (patients with at least one neighboring tooth and >50% buccal bone were included). Three sites in the control group and six in the test group showed complete wound resolution at T2 (p = 0.259). HA did not affect analgesics, patient-reported outcomes, alveolitis, socket healing, soft tissue changes or mucosal scarring, while horizontal bone loss was significantly higher in the test group (p ≤ 0.025). Thus, the hyaluronic acid gel trial evaluates wound healing and preservation.118 Hyaluronic acid (HA) and other biopolymeric formulations have been investigated in recent studies for their potential to improve wound healing through a variety of mechanisms, including anti-inflammatory-, antimicrobial-, and regenerative characteristics. Lee et al. (2022) investigated the impact of HA films on oral wound healing in a rat model, revealing that the HA gel (84.4% ± 9.2%) and film (74.0% ± 15.0%) groups display significantly enhanced healing rates relative to controls, along with reduced inflammation, increased re-epithelialization, and reduced COL1α1 expression levels, thereby substantiating the efficacy of HA film in facilitating oral wound healing.119 Mosawi et al. (2024) investigated the role of hyaluronic acid (HA) in drug delivery systems, emphasizing its biological functions that are dependent on molecular weight, including anti-angiogenic, wound-healing, and angiogenic properties, with potential applications in micro and nano-formulations containing antibacterial and anticancer agents.120 Katiyar et al. (2024) formulated a hemocompatible hybrid material consisting of chitosan, gelatin, and hyaluronic acid infused with graphene oxide–silymarin (CGH–SGO), demonstrating improved biocompatibility, with antibacterial and antioxidant characteristics. The hybrid structures exhibit rapid blood coagulation and expedited healing of full-thickness burn injuries in vivo, positioning them as potential options for burn wound therapy. Collectively, these investigations underscore the adaptability and efficacy of HA-based formulations in enhancing wound healing via increased cellular responses, antimicrobial properties, and tissue regeneration.121
2.7 Gelatin
Gelatin (gelatine) is a translucent, colorless ingredient derived from collagen from animal body parts and used as a gelling agent in beverages, medications, drug or vitamin capsules, photographic films, etc. It is brittle when dry and rubbery when moist, also known as hydrolyzed collagen.122 It is a protein with 98–99% protein content, with hydrolyzed collagen containing 19 amino acids, primarily glycine, proline, and hydroxyproline, affecting gelation properties.123 Porous gelatin matrices absorb wound exudates and maintain moisture, cell migration, and support tissue development to promote the wound healing process.124 Its water-binding, gel-, film-, and foam forming abilities, water vapour barrier, and emulsification propensity make it a promising material for wound healing.125
Mirjalili et al., 2023 developed platelet-rich fibrin–chitosan (CH–PRF) nanoparticles integrated into gelatin–chitosan hydrogel (Gel–CH/CH–PRF) for improved hydrogel dressing properties. Chitosan-containing hydrogels have the lowest scavenging capacity (83%) and highest DPPH radical scavenging activity and excellent cell viability and proliferation, with wound closure significantly higher on the Gel–CH/CH–PRF hydrogel which accelerates wound healing.126
Razack et al., 2023 fabricated Oregano essential oil nanoemulsion and low-level laser therapy for diabetic wound healing using hydrogel-based patch by polymers (chitosan, gelatin, and polyvinyl pyrrolidone) with cellulose nanofibrils for enhanced stability. The drug concentration of 128 μg mL−1 showed viability of NIH/3 T3 fibroblasts after 24 hours. Hence, the combination of nanoemulgel and low-level laser therapy is a regimen for managing diabetic foot ulcers, resulting in rapid healing and minimal scar formation.127
Lu et al., 2022 synthesized tilapia fish skin gelatin–fucose gum–tannic acid (Gel&Fuc–TA) hydrogel that promotes wound healing by combining with tannic acid, gelatin and fucoidan, offering excellent antibacterial-, antioxidant-, and hemostatic properties. As a result, Gel&Fuc–TA hydrogel is a green cross-linking reaction-based hydrogel that promotes the expression of VEGF, CD-31, and α-SMA, collagen deposition, wound repair, microbiome changes and regulates macrophage conversion.128
Asada et al., 2022 showed that a dermal defect graft, Terudermis® Artificial Dermis (AD-T), was used as dressings on 100 mm2 wounds with exposed bone in rats. The wound-healing efficacy of the treatment was compared between AD-T and GS (gelatin sponge) groups at 1, 2 and 4 weeks after surgery. AD-T achieves faster wound healing, accelerates bone remodeling, and increases the production of blood vessels, fibroblasts, and osteoblasts. This suggests that AD-T is better as a wound dressing material.129
Khoshmaram et al., 2024 employed chitosan nanoparticles (CSNPs) and gelatin methacrylate (GelMA) to generate a biomimetic composite bioink for 3D bioprinting. Nanoparticles infused with curcumin enhance antibacterial activity and skin proliferation. The CSNPs demonstrate an efficient barrier against germs and regulate medication release. The biocomposite aids in wound healing, encourages cell division and lessens microbial infections in Wistar rats.130
Bessalah et al., 2024 investigated the potential of gelatin–chitosan–Moringa leaf extract (G–CH–M) as a novel biomaterial for biomedical applications. Blood hemolysis, anti-inflammatory-, antioxidant- and antibacterial-properties against Gram-positive and Gram-negative bacterial isolates were evaluated for the wound-dressing G–CH–M biopolymer. Additionally, the biopolymer shields plasmid DNA from oxidative damage.131
The eco-friendly production of silver nanoparticles (AgNPs) using Dactyloctenium aegyptium extract as a capping and reducing agent can be used in wound healing.132 Nanoparticles were subsequently integrated into PVA, Na-alginate, and gelatin-based hydrogel dressings to examine their in vivo wound healing efficacy in rats. The change in color of the reaction mixture and the surface plasmon resonance at 400 nm validated the synthesis of AgNPs. FT-IR research demonstrated the participation of phytochemicals from the plant extract in the capping and stability of nanoparticles. The nanoparticles display a crystalline structure, with an average crystal size of 28.03 nm, and show antibacterial efficacy against S. aureus, Pseudomonas aeruginosa, Klebsiella pneumoniae, and E. coli, with zones of inhibition of 19 ± 0.0, 9 ± 0.0, 13 ± 0.0, and 13 ± 0.0 mm, respectively. Moreover, silver nanoparticle-embedded hydrogels demonstrate enhanced wound healing in rats relative to untreated animals and those administered a commercial product (Fig. 3). Consequently, the formulated hydrogel dressing exhibits promise for practical use in wound healing and infection management.
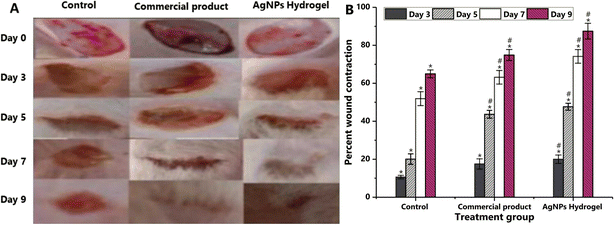 |
| Fig. 3 Assessment of wound healing efficacy of AgNPs-loaded hydrogel compared with a control and a commercial product (A) Photographs of injuries of different animal groups on distinct measuring days. The control group received no therapy, the experimental group received commercial treatment (1% silver sulfadiazine), and another group received AgNPs–DA-loaded (1%) hydrogel dressing. (B) Percentage of wound contraction. Data are presented as mean ± standard deviation (SD). * signifies statistical comparison between the control group and the standard group, as well as the AgNP–DA hydrogel group, whereas # indicates a comparison between the standard group and the AgNPs–DA hydrogel group. Reproduced from ref. 132 with permission from [Elsevier], copyright [2024]. | |
2.8 Pectin
Pectin (structural fiber) or gelatinous polysaccharide found in fruit is primarily present in the peel portion and becomes water-soluble during fruit ripening. It is a linear polymer with a backbone of galacturonic acid monomer units linked via α-(1→4)-glycosidic bonds.133 Pectin is a hydrophilic substance that combines with wound fluid to generate a soft gel over the wound bed, which aids in the removal or management of exudates.134 During pectin solubilization, the acidity of the resultant pectin solution improves the system's bacterial or virus barrier characteristics.135 Pectin binds to intestines, adds bulk to stools, and reduces cholesterol absorption, aiding in mitigating high cholesterol, diabetes, heartburn, and diarrhea and functions in cell adhesion and wall hydration.136
Chanmontri et al., 2023 used quaternized chitosan (QCS) and oxidized pectin (OPEC) to improve antibacterial activity and enhance solubility, with self-healing hydrogels enhancing ionic interactions through co-injection. The hydrogel displays self-healing, fast gelation, storage modulus and compressibility and has no cytotoxicity to NCTC clone 929 cells. The extraction media lacks antibacterial characteristics, while QCS has a MIC50 of 0.04 mg mL−1 against E. coli and S. aureus. Hence, injectable self-healing QCS/OPEC hydrogel has potential for wound management.137
Song et al., 2023 designed a pectin–chitosan (PEC–CS) hydrogel with a bioadhesive micelle containing ciprofloxacin for wound healing. PEC–CS hydrogels have high water content (>95%), strong absorption, good water retention, and no cytotoxicity, making them suitable for wounds. Thus, dopamine-modified carriers improve solubility, retention time, and antibacterial activity. In vitro and in vivo pharmacodynamics experiments show that they resist bacteria, promote wound healing and possesses anti-infective properties.138
Saucedo-Acuña et al., 2023 synthesized allantoin-enriched pectin hydrogel and showed that it improves surgical wound healing in rat models with hydrophilic behavior and healing efficacy. Hydrophilic behavior (11.37°) and functional groups like carboxylic acid and amine groups are found in the amorphous pectin hydrogel. As a result, allantoin promotes wound drying and interaction with cells, reducing healing time by 71.43% and achieving total closure in 15 days in female Wistar rats.139
Phonrachom et al., 2023 investigated quaternized chitosan (QCS) and pectin (Pec) blended to enhance water solubility and antibacterial activity in hydrogel films. Propolis was loaded for wound healing, mechanical properties, adhesiveness, and biological activities. Blending QCS and Pec enhances hydrogel films, tensile strength, stability, and controls propolis release. These films show antioxidant activity (∼21–36%), bacterial growth inhibition, non-toxicity to mouse fibroblast cells, and support wound closure. Thus, propolis-loaded QCS/Pec hydrogel films are promising wound dressing materials.140
Alsakhawy et al., 2022 encapsulated NAR in Arabic gum (AG)/pectin hydrogel which showed excellent EE% (99.88% ± 0.096%) and DL% (16.64% ± 0.013%) characterized using FTIR, DSC, SEM and EDS%. NAR-loaded AG/pectin hydrogel accelerates wound healing by enhancing angiogenesis, re-epithelialization and collagen deposition. It significantly down-regulates inflammatory mediators and apoptosis (P < 0.001) and has potent antioxidant activity, enhancing SOD and GSH levels.141
Elsherif et al., 2024 evaluates how nebivolol hydrochloride and pectin affect wound healing. The formulation containing pectin nanoparticles loaded with NBV demonstrated a particle size of 572 nm and an encapsulation effectiveness of 70.68%. The formulation encourages collagen deposition, tissue proliferation and wound healing in vivo. This implies that it is a potentially useful substance for tissue regeneration and wound healing.142
Kapoor et al. examined formulation tactics and crosslinking approaches to improve drug entrapment and controlled release, 2024 s. Pectin hydrogels could deliver medicinal drugs in clinical trials, wound healing, tissue engineering and oral and transdermal administration. Thus, pectin hydrogels appears to have a bright future, notwithstanding issues with standardization and regulatory compliance.143
2.9 Ulvan
Ulvan are sulfated heteropolysaccharides from marine macroalgae (sea lettuce) used in food, medicine, and agriculture. They consist of L-rhamnose, D-xylose, D-glucose, and D-glucuronic acid, with aldobiuronic acid (4-O-β-D-glucuronosyl-L-rhamnose) being a unique component.144,145 It is a biopolymer with antioxidant-, antiviral-, anti-inflammatory-, and anticoagulant properties, and it is valuable for wound dressing development. It reduces cholesterol, LDL, and triglycerides, which are risk factors for coronary disease.146,147 Adding uncommon carbohydrates into its backbone structure, such as iduronic acid and sulfated rhamnose, keeping the wound wet, and increasing its ability to absorb wound exudate can improve wound healing activity.148,149 Ulvan protects cells from free radical damage and boosts our immunity.150
Kikionis et al., 2022 fabricated nanofibrous patches made from ulvan and polyethylene oxide (PEO) for anti-inflammatory and antioxidant properties for use in keloid (fibroproliferative disorder) treatment and cryosurgery (treatment for keloids, causes skin traumas). The ulvan/PEO patch demonstrates significant wound healing, skin inflammation elimination and biophysical restoration after 21 days of cryosurgery. It is the first wound dressing that heals skin trauma after cryosurgical treatment of keloids without discomfort.151
Ren et al., 2022 prepared Ulva fenestrata green macroalgae polysaccharide to create a hydrogel for chronic diabetic wound healing (UC–DPA–Ag hydrogel) containing hUC-MSCs for enhanced healing. The hydrogel with UC–DPA–Ag exhibits mechanical properties, swelling capability, adhesiveness, antioxidant, antibacterial ability and promotes cell proliferation and migration. Thus, it accelerates wound healing in diabetic mellitus mice and offers a new route for Ulva biomaterial production.152
Don et al., 2022 developed crosslinked ulvan/chitosan complex films with or without the addition of glycerol and chlorophyll. The ulvan/chitosan complex films shows high tensile strength and elongation at break (2.23–2.48 MPa), with water vapor transmission rates of 1791–2029 g per m2 per day. Biocompatibility studies show that glycerol and chlorophyll-added films promote migration, protection, wound healing, regeneration and collagen production in NIH 3T3 and HaCaT cells.153
Sulastri et al., 2023 successfully fabricated a novel hydrogel film wound dressing combining ulvan and silver nanoparticles, with silver nitrate concentrations of 0.5 mM and 1 mM to produce ulvan–silver nanoparticle hydrogels. Physicochemical characteristics were evaluated using various techniques. Ulvan–silver nanoparticle hydrogel films show potential as wound dressings for second-degree burn healing, with UHF–AgNP0.5 showing the highest antimicrobial activity and accelerated healing in Wistar rats.154
Mariia et al., 2021 incorporated chitosan–ulvan hydrogel with cellulose nanocrystals (CNCs) loaded with epidermal growth factor for improved morphological features, mechanical stress curve and swelling behavior through a freeze-drying process. As a result, nanocomposites exhibit non-toxic behavior, cell proliferation, and enhanced epidermal growth factor delivery (15 days from 100% wound contraction) with CS–U–CNC–EGF hydrogels showing faster wound healing efficiency, faster tissue formation and collagen deposition, potentially enhancing wound dressing applications.155
Foroughi et al., 2024 described a novel technique for employing ulvan hydrogel to create 3D biomaterials for wound healing applications. Wet-spinning and additive manufacturing were used to create 3D printed hydrogel structures and wet-spun ulvan fibers. The ulvan solution improves mechanical characteristics and cell survival with a viscosity of 110 Pa s and surpasses 180 Pa s on day four. Because ulvan fibers are naturally biocompatible, they act as a potential remedy for wound healing.156
Statha et al., 2024 examined the marine sulfated polysaccharide carrageenan's and ulvan's capacity to promote wound healing in gels. The 10% w/w carrageenan gel considerably accelerates wound healing in female SKH-hr2 mice without hair and with burn-inflamed skin, particularly in the initial phases. The 5% w/w ulvan gel shows effectiveness in accelerating the healing process, particularly in the later phases. These results imply that ulvan and carrageenan gels may be able to increase the effectiveness of wound healing in second-degree burn injuries.157
Wound dressings were synthesized from alginate and pectin, integrated with mangosteen extract (ME), and encapsulated in niosomes (ME-loaded niosomes).158 Researchers subsequently analyzed the in vitro release and physical properties of ME-loaded niosomes. The agar diffusion method quantifies the extent of a substance's antibacterial inhibition. The size of the zone of inhibition increased with antibacterial efficacy. The NCs contained identical components to the tested samples, except ME. MEs at doses of less than 0.15 mg and 0.3 mg did not inhibit S. aureus and Staphylococcus epidermidis, respectively. Meanwhile, 20 mg of ME suppressed S. aureus and S. epidermidis, yielding zones of inhibition (ZOIs) of 17 ± 1.1 mm and 16 ± 1.2 mm, respectively (Fig. 4). The findings indicated that the ME concentration rose in conjunction with antibacterial activity. Excessive levels of ME adversely affect fibroblasts (L929). Reduced concentrations of ME were most effective for inhibiting bacterial growth. Furthermore, researchers analyzed the swelling ratio and biological properties of the hydrogel film. The maximum swelling ratios of patches with 0.5% and 1% Ca2+ crosslinking were 867 wt% and 1025 wt%, respectively, after 30 min. A medium dose (15 mg) of niosomal ME incorporated in a hydrogel film provides better bacterial inhibition, cell migration, and cell adhesion in an in vitro model. Additionally, no toxicity is observed in the fibroblasts and red blood cells. Consequently, this product may serve as a viable option for wound dressing applications.
 |
| Fig. 4 Results of disk diffusion (zone of inhibition) for S. epidermidis (left) and S. aureus (right) vancomycin as the positive control (PC), normal saline solution as the negative control (NC), and various doses of ME were used. Reproduced from ref. 158 with permission from [CellPress], copyright [2024]. | |
3. Herbal bioactive loaded formulations
An abundant number of medicinal plants are being therapeutically used for skin and wound treatment (Fig. 5 and 6).159 The natural products promote the healing process as a result of the active chemical constituent's existence, e.g., flavonoids, alkaloids, triterpenes, and other biomolecules.160
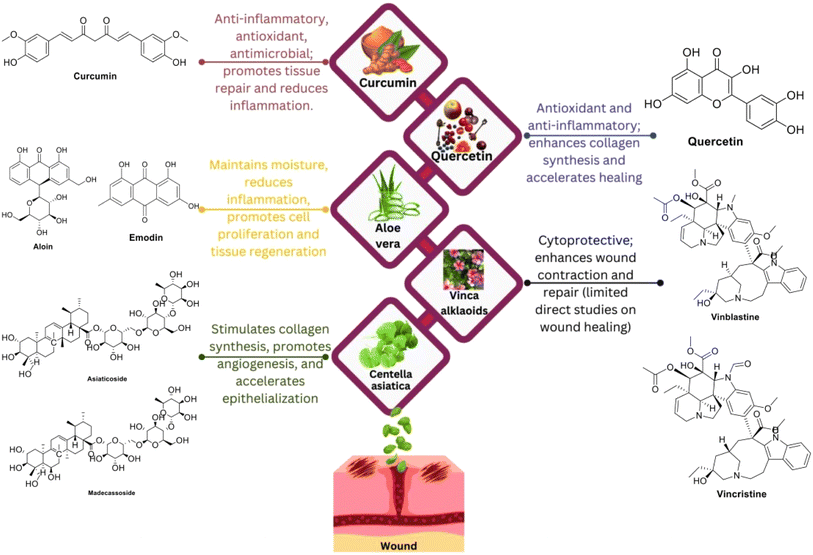 |
| Fig. 5 Medicinal plants, with their chemical structures and bioactivities, utilized in wound treatment. | |
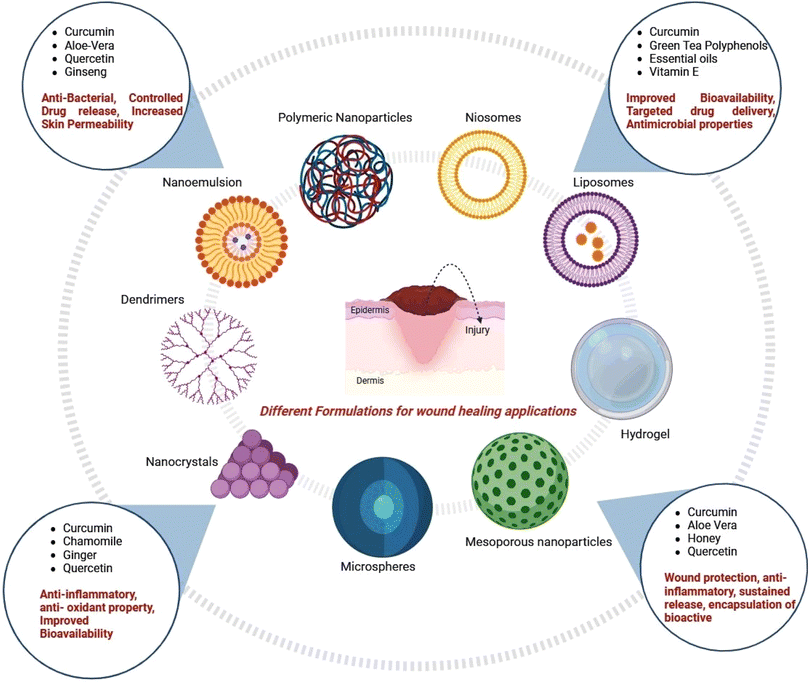 |
| Fig. 6 Nano-formulations loaded with herbal bioactives for enhanced therapeutic efficacy. | |
3.1 Curcumin
Curcumin (Cur or diferuloylmethane) is an herbal plant with a low molecular weight poly-phenolic flavonoid, i.e., extracted from the rhizomes of Curcumin longa, family Zingiberaceae.161 The molecular formula of Cur is C21H20O6.162 Curcumin (77%) is a bio-active constituent of turmeric rhizomes, with 17% demethoxycurcumin, 3% cyclocurcumin, and 3% bisdemethoxycurcumin.163 It is an ideal therapeutic biomolecule for the treatment of various inflammatory diseases (Alzheimer's, rheumatoid arthritis, diabetes, multiple sclerosis, inflammatory bowel, atherosclerosis, etc.) and wound healing due to its strong anti-infective, anti-inflammatory, antibacterial, and antioxidant properties.164 Various in vitro and in vivo studies prove that it can accelerate skin, excision and chronic wound healing.165,166 Novel drug delivery systems (nano DDS) are required to increase its restricted therapeutic efficacy, e.g., poor oral absorption bioavailability, water solubility, chemically unstable, rapid metabolism and elimination, short shelf life, etc.167,168 Cur has also gained attention as a local drug due to its pharmacological properties: non-toxic, good tolerance, etc.169 It also helps forms anti-scars by reducing inflammatory factors secretion and inducing apoptosis.170 Curcumin hydrogels are promising tools for drug delivery to the epidermis and dermis to enhance the drug concentration at the site of treatment and they also reduce adverse reactions in systemic circulation.171,172 WHO confirmed that the daily consumption of Cur as a food preservative has extraordinary wound healing properties by stimulating proliferation and remodeling phases of the skin regeneration process.173,174 It enhances the contraction rate of wounds, boosting the wound healing process.175 Cur also binds with COX-2 protein, which ultimately reduces its expression, as well as prostaglandin and thromboxane synthesis. It increases the wound area by up to 20%.176 During inflammatory reactions, Cur blocks the activity of the two crucial cytokines i.e., tumor necrosis factor-alpha (TNF-α) and interleukin-1 (IL-1), which are generated by macrophages and monocytes that regulate inflammatory responses.177 It enhances PPAR-γ activity, which ultimately inhibits vascular smooth muscle cell proliferation and decreases angiotensin-II induced inflammatory reactions.178 Curcumin has an effective protective function against oxidative stress, a complex element which limits tissue regeneration in the process of wound healing through modulating lipoxygenases (LPx) by scavenging free radicals.179 Curcumin treatment causes fibroblast infiltration into wound sites.180 It enhances granulation tissue formation and ultimately facilitates re-epithelialization by providing a stable foundation for epithelial cells for migration and healing of wound gaps.181 Cur enhances collagen and synthesis of the extracellular matrix to accelerate the wound healing process.182 Gels, films, sponges, synthetic polymers (polyurethane and polyester) and natural biopolymers membranes (e.g., chitosan, hyaluronic acid, and collagen) are used for wound treatment.183 Curcumin with CNP results in enhanced maturation of the wound, collagen content, cell proliferation and granulation tissue development.184,185 The applications of curcumin-loaded formulations are summarized in Table 2, highlighting their applications in various wound healing scenarios.
Table 2 Applications of curcumin-loaded formulations
Components |
Formulation |
Applications |
Reference |
Curcumin + chitosan |
Nanoparticles, nanofibers, nanotubes |
Bacterial infection |
186 |
Curcumin + succinyl chitosan-fish |
Hydrogel |
Subcutaneous wounds |
187 |
Cur + chitosan + carboxy methyl cellulose (CMC) |
Injectable hydrogels |
Diabetic wound regeneration |
188 |
Curcumin + pectin + chitosan |
Nanofilms |
Antibacterial, wound dressing material |
189 |
Curcumin + fenugreek essential oil (FEO) + polylactic acid (PLA) |
Films |
Antibacterial, antioxidant |
190 |
Cur + dextran + sodium alginate |
Wafer dressings |
Enhances the healing rate |
191 |
Curcumin + alginate + carrageenan + poloxamer |
Hydrogel films |
Transdermal wound healing |
192 |
Curcumin + alginate + carrageenan + poloxamer + diclofenac |
Films |
Antibacterial, transdermal dressings |
193 |
Cur + chitosan + methylcellulose |
3D-biocomposite scaffolds |
Diabetic wound healing |
194 |
Cur + hyaluronic acid + pullulan |
Injectable hydrogel |
Diabetic wound repair |
195 |
Curcumin + silk fibroin + HA |
Hydrogels |
Cell therapy, scaffolding |
196 |
Indole curcumin analogue (ICA) + folic acid conjugated chitosan |
Nanoparticles |
Antibacterial, anti-inflammatory, anticancer |
197 |
Liposomal CUR + hyaluronic acid (HA) + polyvinyl alcohol (PVA) |
Hydrogel |
Skin recovery, wound dressings |
198 |
Al-Arjan et al., 2022 fabricated pH-responsive hydrogels by blending bacterial cellulose (BC) with polyvinyl alcohol (PVA) and graphene-oxide (GO) dressing materials by crosslinking with tetraethyl orthosilicate (TEOS). The formulated hydrogels show good, controlled curcumin release (at pH 6.4, 7.4, and 8.4) in a controlled form. As curcumin loaded-BSG-4 shows anti-bacterial (p < 0.05, p < 0.01, and p < 0.001) and anti-tumor properties (against U87 cell lines), these hydrogels act as a potent biomaterial for chronic wound healing.199
Velmurugan et al., 2022 formulated chitosan-based curcumin loaded carbon nanospheres (CNS) on polypropylene (PP) non-woven fabric support. CNS and Cur both contribute to feasible water and moisture absorption by scaffolds and the wound dressing shows maximum wettability (475.37% ± 8.98%). About 96.5% of wound contraction was measured, hence it is effective in skin regeneration.200
Singh et al., 2022 developed sustainable extracellular matrices (ECMs) containing Cur and decellularized goat small intestine submucosa (DG-SIS). The scaffolds scavenge free radicals (DG-SIS: 8.6%, DG-SIS/C1: 65.8%, DG-SIS/C2: 71.7%, DG-SIS/C3: 79.9%) and exhibit antioxidant and antibacterial properties. The porosity% and large pore size are 87–94% and 50–357 μm, respectively, which results in enhanced water uptake. DG-SIS/C3 containing 1 wt% Cur shows free radical scavenging of about 80%, which is beneficial for wound healing. Therefore, the system is a promising biomaterial for skin tissue engineering and wound healing.201
Wu et al., 2023 encapsulated Cur and Cur-chitosan nanoparticles (CCNP) into chitosan collagen vanillin scaffold by the freeze-drying method. The CCNP + VC and Cur NP + VC nanoscaffolds have particle sizes of 110.6 nm and 195.9 nm, respectively. These nanoscaffolds also have release profiles >60%, improved anti-oxidant properties (>80%) and enhanced wound healing capacity (85.62% and 77.05%, respectively) in a murine cell line. Hence, they are effective and biodegradable drug delivery system for topical use to heal wounds and stop bacterial infection.202
Kenawy et al., 2023 prepared cross-linked antimicrobial membrane comprising PVA–Aloe vera hydrogels by propanol to transform PVA into a highly crystalline structure. Cur and gentamycin incorporation enhances biological and antimicrobial activities. Cur/gentamicin-loaded hydrogel membranes show the highest angle of contact values (78.2° decreased to 27.7°) after encapsulating the Aloe vera to 80% in membranes. In vivo studies and the wound dressed histological test reveals the reduced size of the fully thickened wound and re-epithelialization. Therefore, it can be a preferred biomaterial for skin regeneration and wound healing.203
Li et al. (2024) investigated the use of decellularized caprine small intestine submucosa (D-CIS) encapsulated with nanoformulations of cerium oxide and curcumin for enhancing burn wound healing. The study highlighted the bioactive gel's properties, including antimicrobial-, antioxidant-, and anti-inflammatory effects, along with the sustained release of active components. The combination of cerium oxide and curcumin in the gel accelerates burn wound healing by mitigating oxidative stress, reducing inflammation, and supporting cell recruitment for epithelial and vascular regeneration. These findings underscore curcumin's role as a key component in promoting tissue repair through its antioxidant properties, establishing it as a promising candidate in wound healing applications.204
Miele et al. 2024 conducted a comparative study on electrospun nanofibers made of collagen and polycaprolactone (PCL) loaded with curcumin (Cur) or resveratrol (Rsv) to evaluate their biocompatibility and effects on wound healing and angiogenesis. Curcumin-loaded fibers exhibit hydrophobic properties, support cell adhesion, and maintain structural integrity, indicating potential for wound dressing applications. Both Cur and Rsv display anti-angiogenic effects in the chick embryo chorioallantoic membrane assay, suggesting suitability for anticancer uses but posing challenges for wound healing where angiogenesis is critical. This highlights the importance of optimizing curcumin concentration to balance its antioxidant and anti-inflammatory benefits while avoiding reduced vascularization in healing tissues.205
3.2 Quercetin
Quercetin (3,3′,4′,5,7-pentahydroxyflavone) is an endogenous antioxidant from the flavonoid group of polyphenols found in many fruits, vegetables, leaves, seeds, red wines, onions and capers (Liliaceae).206 It exists as a glycoside, glycone (sugar attached) or aglycone (no sugar attached). It takes many forms in nature, but the form found in plant is quercetin-3-O-glucoside (iso-quercetin).207 The molecular formula of quercetin is C15H10O7 (302.236 g mol−1).208 Quercetin minimizes fibrosis and scar formation during wound healing, boosts fibroblast cell proliferation, limits immune cell infiltration, and cause changes in fibrosis-related signaling pathway.209 It has potent antibacterial, anticancer, anti-inflammatory and antioxidant effects, which benefits inflammatory diseases like asthma, arthritis, lung damage, and diabetic angiopathy. In addition, quercetin can reduce inflammation in the acute and chronic phases.210,211 QCN protects skin from dehydration and inhibits collagen deterioration during inflammatory feedback.212 It is a great reactive oxygen and nitrogen species and reactive nitrogen species scavenger, making it a suitable option to reduce oxidative stress.213 QCN is crucial for wound healing due to its free radical scavenging and histamine inhibition, reducing skin swelling.214 Quercetin has been produced in a variety of dermatological preparations including gel, emulgel, and microemulsion gel.215 Nanoformulations offer advantages like increased bioavailability, targeted tissue targeting, and controlled release behavior, but also face long-term instability, complex preparation, and high costs, making them less accessible to patients.216 The application of QCN-loaded gels leads to a significant improvement in wound healing compared with other groups, highlighted by enhancement and reduction of fibroblasts and inflammatory cells, respectively.217 The levels of inflammatory factors such as tumour necrosis factor, interleukin-1, and interleukin-6 were dramatically lowered.218 Prodrugs and liposomes are some of the ways to facilitate topical/transdermal delivery of Qu through the skin. The production of quercetin-3-O-acyl esters results in a potential topical prodrug.219,220 QCN has antiaging properties on middle-aged keratinocytes and supports regeneration of terminally senescent cells. It promotes wound recovery, regulates VEGF, TGF-β1, IL-10, slow-modulates TNF-α, and improves wound care by inhibiting the MAPK pathway.221,222 The wound healing capacity of quercetin and curcuminoid combinations were tested using a scratch experiment, and show faster wound closure and reduced immune cell infiltration (cell migration).223 In vitro studies reveal that quercetin enhances keratinocyte proliferation and migration by increasing β-catenin expression.224,225 Activation of the ER accelerates re-epithelialization during wound healing in vivo. As a result, we believe that quercetin may promote EpSC proliferation via the ER.226 Polymeric drug delivery systems, including nanoparticles, offer promising strategies for disease prevention and treatment.227,228 Nanoparticles can enhance tumor-targeted delivery by regulating surface ligands, transport various agents via antitumor mechanisms, and accumulate higher tumor-encapsulated drugs through permeability and retention.229 Quercetin's effect on cancer cells includes cell growth inhibition, apoptosis induction, and metastasis inhibition (activates caspase-3, inhibits Akt, mTOR, ERK, reduces β-catenin, and stabilizes HIF-1α).230 Quercetin stability is affected by antioxidant concentrations, pH, temperature, and metal ions. Encapsulating it in a strong carrier protects it from oxidation, isomerization, and degradation, increasing shelf stability and enabling effective administration.231 Hence, quercetin therapy enhances wound healing by modulating cytokines and growth factors.232 Table 3 provides a comprehensive summary of the formulations and applications of quercetin-loaded systems, emphasizing their therapeutic potential in various wound healing scenarios.
Table 3 Applications of quercetin-loaded formulations
Components |
Formulation |
Applications |
Reference |
Sodium alginate + poly(vinyl) alcohol (PLA) + quercetin |
Hydrogel |
Skin ageing and inflammation |
233 |
Quercetin + oleic acid |
Nano-hydrogel |
Diabetic foot ulcer |
234 |
Quercetin + silver nanoparticles (AgNPs) |
Hydrogel matrices |
Diabetic wounds |
235 |
Pectin + chitosan NP + quercetin |
Beads |
Antibacterial, antioxidant |
236 |
Quercetin + chitosan |
Nanotube |
Promote moist environment |
237 |
Asparagus cellulose + quercetin |
Films |
Antimicrobial |
238 |
Quercetin + H2S |
Conjugates |
Diabetic wounds |
239 |
Quercetin + lactoferrin + chitosan nanofiber |
Nanofiber |
Biodegradability, antioxidant |
240 |
Chitosan + PLGA + quercetin |
Scaffolds |
Oral lesions |
241 |
Quercetin + ZnO + CuO + chitosan |
Nanocomposite |
Wound recovery |
242 |
Quercetin + PLA + graphene oxide (GO) |
Scaffold matrices |
Anti-infection |
243 |
Quercetin + Prunus armeniaca gum exudate (PAGE) |
Green nanoparticles |
Antibacterial |
244 |
Quercetin + carboxymethyl cellulose (CMC) |
Nanogel |
Anticancer |
245 |
Nalini et al., 2023 created gel formulations of quercetin-loaded alginate (Q-ALG)/chitosan nanoparticles (CSNP) incorporated into carbopol encoded as Q-ALG/CSNP-G1 and Q-ALG/CSNP-G2. Q-ALG/CSNP-G2 gel effectively releases quercetin (62.51% ± 0.72%) for 24 h, promotes wound healing, enhances antioxidant and antibacterial effects, and improves the healing quality in Wistar albino rats.246
Chaturvedi et al., 2023 explored nano-encapsulated quercetin formulations (lipid-based nanocarriers, nanocrystals, polymeric, mesoporous silica nanoparticles, nanofibers, and scaffolds) to overcome the shortcomings of quercetin. Owing to low aqueous solubility (0.48 g mL−1), membrane permeability (1.8), and high gut wall metabolism (93.3%), a 5% bioavailability enhances quercetin permeability and retention for diabetic foot ulcer treatment efficacy.247
Saleh et al., 2023 synthesized quercetin/selenium nanoparticles (Qu/SeNPs) mixed in various ratios into the produced hydrogel to enhance the biological performance and revealed antibacterial-, cell viability-, and anti-inflammatory properties. Qu is used as a reducing agent in SeNP manufacturing since it reduces cytotoxicity against HFB4 and enhances erythrocyte protection, suggesting potential tissue engineering applications with low harmful effects.248
Li et al. 2024 developed an SF/SPI-Q hydrogel by incorporating quercetin into a silk fibroin and soybean protein isolate hydrogel. The SF/SPI-Q hydrogel combines excellent biocompatibility, biodegradability, and cell migration promotion with the antibacterial and antioxidant properties of quercetin. It accelerates wound healing in an infected burn wound model by promoting epidermal regeneration, collagen deposition, angiogenesis, and reducing inflammation, making it a promising treatment for wound care.249
Kumar et al. 2024 synthesized a CaCO3/SiO2 nanocomposite using the sol–gel method and characterized it using XRD, FTIR, and TEM. They combined this nanocomposite with quercetin and incorporated it into a PLGA/gelatin patch to enhance wound healing. The patch promotes cell proliferation, exhibits antibacterial efficacy against four major wound-associated bacterial strains, and demonstrates excellent water retention, making it an ideal material for diabetic wound healing. In vivo trials confirm the enhanced wound healing potential of the patch, highlighting its effectiveness in treating diabetic foot ulcers.250
3.3 Aloe vera
Aloe vera (Aloe barbadensis miller, family-Liliaceae) is a perennial, stem-less, xerophytic and succulent green herb, which is found in the desertic region of Asia, Europe, America, Africa and is also cultivated in India.251 Aloe vera (AV) is a plant extract that is widely used for therapeutic purposes as it consists of polysaccharides (pectic acid and glucomannans) and enzymes (bradykinase, carboxypeptidase, peroxidase, lipase, cellulase, and catalase).252 AV leaves consist of three layers: inner (transparent gel containing 99% water + 1% of lipids, glucomannans, vitamins, amino acids, and sterols), middle (bitter yellow juice with anthraquinones and glycosides) and outer (contains carbohydrates and proteins) layers.253 It is known for its anti-oxidant-, anti-diabetic-, immunostimulatory-, neuro-protective-, anti-bacterial-, anti-viral-, wound healing-, anti-tumor-, antiseptic-, analgesic-, anti-fungal-, anti-hyperlipidemic-, anti-rheumatoid-, anti-arthritis-, and skin protectant properties.254 Also, AV leaves contain various chemical constituents such as anthraquinones (treat burns + wound healing), minerals (essential in wound healing), amino acids (reconstruct damage tissues), hormones (wound healing), salicylic acid (analgesic), steroids (anti-inflammatory), saponins (antiseptic), sugars (antiviral), vitamins (anti-oxidant), and protein (cell proliferation).255 It promotes healing by affecting different factors involved in the process of wound healing.256 AV is widely used for chronic wounds, e.g., psoriasis, surgical, burn wounds, pressure ulcers and genital herpes.257 Various polymeric wound dressing materials including non-fibers, hydrogels, foams, transdermal patches, membranes, films, and wafers are used to improve the wound healing properties.258 They prevents the formation of scar during skin injury by promoting and stimulating the regeneration process and production of cells respectively at hypodermis layer.259 AV enhances the migration of epithelial cells, maturation of collagen and reduces swelling by keeping the wound moist.260 The active constituent in the AV gel is a polymer (acemannan), which helps activate macrophages and T-cells to stimulate cytokine production and accelerates the process of wound healing by inducing pro-inflammatory mRNA transcription.261,262 Additionally, when acemannan is applied topically, it acts through Cyclin-D1 and AKT/mTOR signaling pathways, resulting in reducing the time for wound closure.263 Also, magnesium lactate as its constituent helps to stop histamine production, which ultimately results in skin itching and irritation.264 Aloe vera enhances the wound healing process due to its inhibiting activity on thromboxane, IL-6 and IL-8 and reduces the levels of TNF-α.265 AV is responsible for enhancing the cross-linkage of collagen and its composition.266 It also consists of mannose, which enhances macrophage activity by increasing collagen production.267 Table 4 provides a summary of the formulations and applications of Aloe vera-loaded systems, emphasizing their therapeutic effectiveness in enhancing wound healing in diverse clinical contexts.
Table 4 Applications of Aloe vera-loaded formulations
Components |
Formulation |
Applications |
Reference |
Arabic gum + Aloe vera + gelatin powder + polyurethane |
Bio-based wounds |
Bedsore |
268 |
Aloe vera-acetate/polyvinylpyrrolidone (AVAc/PVP) |
Electrospun fibres |
Anti-bacterial and anti-inflammatory |
269 |
Alginate + Aloe vera + honey + cellulose |
Nanocrystals |
Scratch wound and anti-bacterial |
270 |
Thymol + Aloe vera + chitosan |
Films |
Anti-oxidant |
271 |
Tetracycline hydrochloride (TCH) + poly(caprolactone) + gelatin + Aloe vera |
Hybrid nanofibers |
Anti-microbial |
272 |
Chitosan + Aloe vera |
Hydrogels |
Cell adhesion, reduce inflammation, better re-epithelization |
273 |
Aloe vera + honey + sodium alginate + chitosan |
Wound dressings |
Repair tissue |
274 |
Xanthan gum (XG) + Aloe vera extract (AVE) + bimetallic Ag/MgO NPs |
Nanocomposite |
Anti-bacterial |
275 |
AV + gum Arabic (GA) + silver (Ag) |
Nanocomposites |
Anti-biofilm |
276 |
Chitosan + Aloe vera + mesenchymal stem cells (MSCs) |
Gel |
Grade-II burn injuries |
277 |
Aloe vera + copaiba + chitosan |
Nanofilms |
Wound closure |
278 |
Alginate/gelatin + nanosilver + AV + CUR + plantain peel + Calendula flower petal |
Hydrogel |
Antioxidant, antimicrobial, anti-inflammatory |
279 |
Zinc oxide (ZnO) + Aloe vera + cellulose |
Nanoparticles |
Antimicrobial, antioxidant |
280 |
Chakraborty et al., 2021 synthesized Aloe vera topical gel + nanoemulsion loaded with insulin using polyethylene glycol-400 (PEG), oleic acid, and Tween-80, and evaluated wound healing in diabetic rats. The anti-diabetic effect was found to be P < 0.0001 and the biochemical shows greater wound contraction, i.e., about 75 percent in 15 days. Hence, the nanoemulsion acts as a promising tool in rounds of treatment in diabetic patients.281
Valizadeh et al., 2022 developed a nanoemulsion gel based on Aloe vera loaded erythromycin (AVNE) to improve the process of wound healing. The size of the nanoemulsion particles were 21.2 ± 5.7 nm. There was great anti-bacterial activity and a reduction in the epithelization period, wound contraction and inflammatory cells. Therefore, AVNE acts as a potential candidate in healing wounds as it enhances collagen synthesis.282
Wang et al., 2021 designed a hydrogel wound dressing using AV, dopamine (DA), sodium hyaluronate (SH) and chitosan with the help of deep eutectic solvent (DES). The hydrogel shows great cell compatibility and promotes the regeneration of skin tissues within 12 days. The developed hydrogels has potential as a potent wound dressing.283
Ijaz et al., 2022 formulated herbal cream containing AV-gel and tomato powder. The amount of AV-gel was 2.0 mL, 4.0 mL, 6.0 mL, 8.0 mL and of tomato powder was 0.2 g, 0.4 g, 0.6 g, and 0.8 g in four different formulations (F1 to F4, respectively). The pH of the creams is 7.3 to 7.6, so it is safe for human skin and the spreadability is between 9 to 13. Hence, the herbal creams are effective against any skin injury due to the economical and moisturizing behaviour.284
Ghorbani et al., 2020 synthesized the nano-fibers (NFs)-zein/polycaprolactone (PCL)/collagen loaded with zinc oxide NPs + AV (ZnO NPs AV) for tissue engineering. The ZnO (1 wt%), AV (8 wt%) and zein/PCL (70
:
30) show mechanical properties and thermal stability. Also, the water contact angle of NFs is enhanced by reducing the zein/PCL. The formulated NFs inhibited E. coli and S. aureus bacteria with ZOIs of 15.38 ± 1.12 mm and 19.23 ± 1.35 mm, respectively. Therefore, NFs act as a promising active scaffold for the process of wound healing.285
Vajrabhaya et al., 2022 synthesized toothpaste containing Aloe vera + sodium chloride for enhancing healing as well as oral hygiene. The cytotoxicity of 0.2% (96.44%) or 0.02% (99.07%) toothpaste solution was checked: of the human fibroblast cell line shows good cell migration for the 0.2% concentration. The herbal formulation also enhances cell migration compared with control medium (p = 0.02). Also, the anti-microbial effect on Porphyromonas gingivalis planktonic form is lower than 0.12% chlorhexidine (38.22 + 9.13%) and P. gingivalis biofilm inhibition is enhanced. Therefore, it is an effective and promising formulation for oral health purposes.286
Kenawy et al. 2024 developed physically crosslinked PVA membranes blended with Aloe vera extract using a solution-casting method. These membranes were loaded with caffeine and vitamin C and evaluated for their wound healing potential in Wistar albino rats. In vitro results show improved wound healing through enhanced tissue platelet aggregation and appropriate release behavior. In vivo studies reveal that PVA/Aloe vera/vitamin C membranes significantly reduce the wound area, while the PVA/Aloe vera/caffeine membranes show faster wound closure. Membranes containing both caffeine and vitamin C result in the most significant healing, including hair re-growth. Histological analyses confirm effective re-epithelialization, demonstrating the strong wound healing and skin regeneration properties of these membranes.287
Aizaz et al. 2024 developed a biocompatible, biodegradable biopolymeric gel based on Agar–agar (AA) and gelatin (G) infused with hyaluronic acid (HA) and Aloe vera (AV) for burn treatment and wound healing. The gel exhibits therapeutic effects due to its hydrating-, antibacterial-, and angiogenic properties. Characterization through SEM shows a porous structure, allowing the release of AV and HA, while FTIR analysis confirms crosslinking between AA and G. The gels display rapid release of AV and HA, with high antibacterial activity against E. coli and S. aureus. In vivo studies using the chorioallantoic membrane assay confirmed enhanced angiogenic potential, with a significant increase in the number of branched vessels in the AA/G/HA/AV gel. The results suggest that the AA/G biopolymeric gels infused with AV and HA are effective for burn treatment and wound healing.288
3.4 Vinca alkaloids
Vinca alkaloids (Cape periwinkle) are organic compounds derived from the Madagascar periwinkle plant Catharanthus roseus G, containing carbon, hydrogen, nitrogen, and oxygen with hypoglycemic and cytotoxic effects.289 Vinca alkaloids including vinblastine (VBL), vinorelbine (VRL), vincristine (VCR) and vindesine (VDS), which prevent mitosis of cancerous cells by inhibiting microtubule formation.290,291 Vinca alkaloids have dimeric structures with an indole nucleus (catharanthine) and dihydroindole nucleus (vindoline) which are linked together with complex systems.292 Vinca alkaloid treats various diseases, including leukemia, lymphoma, Hodgkin's disease, neuroblastoma, and breast and testicular cancer.293 It possesses various activities, like antioxidant, antimicrobial, antitumor, antidiabetic, antineoplastic, etc.294 Vinca alkaloids prevent spindle formation during the M-phase of the cell cycle. At low doses, they disrupt microtubular function, but cell cycle arrest and apoptosis occur at higher doses.295 Cell cycle arrest is caused by B-cell lymphoma BCL-2 protein phosphorylation and increased BAX protein levels. Microtubules are involved in transcellular transport, motility, and cytoskeleton stability.296 C. roseus wet flowers and leaf extracts are used as a paste for wounds and tea for Ayurvedic treatment of skin issues, dermatitis, eczema, and acne.297 The juice of Vinca rosea leaves is more successful in lowering total cholesterol, LDL-C, and VLDL in serum levels, triglycerides, and histology of the liver, kidney, and atherosclerotic activity.298,299 Bioactive compounds are heat-, light-, and oxygen sensitive, and drying procedures have a substantial impact on the retention of bioactive components in C. roseus material.300 The investigation of ex vivo applications of the nanoformulation to assess the increased deliverability of Vinca alkaloids via nano-vectors creates a novel drug delivery system based on nanosized particulate particles, known as niosomes, to improve the bioavailability.301,302 Vinca alkaloids and anti-microtubule agents affect nonmalignant and malignant cells in the nonmitotic cell cycle, making them commonly used in combination chemotherapy regimens without DNA cross-resistance.303,304 Cancer remains a significant challenge, with vincristine and vinblastine being highly beneficial agents but their exorbitant and inefficient production persists.305,306 Due to the diverse qualities and applications, Vinca alkaloids are an effective herbal bioactive in the treatment of a variety of ailments.307 The therapeutic potential of Vinca alkaloid-loaded systems to enhance wound healing across various clinical scenarios is highlighted in Table 5.
Table 5 Applications of Vinca alkaloid-loaded formulations
Components |
Formulation |
Applications |
Reference |
Polyesteramide (PEA) + Vinca alkaloids + polyvinylpyrrolidone, polyvinyl alcohol + surfactants |
Nanoparticles |
Cytotoxic activity |
308 |
VNR + propylene glycol + melanoma cell |
Hydrogel |
Treatment of skin cancer |
309 |
Vinca alkaloid + niosomes |
Nano niosomal |
Cell growth and strong cytotoxic activity |
310 |
Vinpocetine (VIN) tablets + poloxamer–chitosan + carbopol–HPMC–alginate |
Hydrogel |
Chronic disorders |
311 |
Ag–MnO2 + Vinca minor extracts + Chelidonium majus |
Nanoparticles |
Chemotherapeutic and cytotoxicity |
312 |
Curcumin + Vinca alkaloids, + taxanes + camptothecin + anthracyclins |
Nanocarriers |
Cancer therapy and improved bioavailability |
313 |
Monoterpene indole alkaloids (Vinca) + MS + HRMS + acetylcholinesterase |
Scaffolds |
Antioxidant |
314 |
Vincristine + vinorelbine + cyclopropane ring |
Conjugates |
Antitumor, chemotherapeutic agent |
315 |
Uracil + paclitaxel + Vinca alkaloids + doxorubicin (DOX) |
Nanotool |
Enhanced permeation and retention (cancer) |
316 |
Manganese-doped copper NPs + Vinca rosea leaf |
Nanoparticles |
Antioxidant, antibacterial |
317 |
ZnO + V-doped ZnO + Vinca plant leaf |
Nanoparticles |
Antidiabetic, antioxidant |
318 |
Aldawsari et al., 2022 synthesized vinpocetine (VNP) to improve cerebrovascular disease management but it faces limitations due to reduced bioavailability and brain levels. The study evaluated the drug delivery effectiveness of surface-tailored intranasal emulsomes using 3221 factorial design and optimization methods. Rat pharmacokinetic assessment improves bioavailability and higher brain levels in surface-tailored VNP emulsomal formulations. Thus, surface-tailored emulsomes are promising for generating high VNP levels in the brain.319
Barnett et al., 2023 used photochemical internalization (PCI) to improve the anticancer drug therapeutic index and develop novel nanoformulations against breast and pancreatic cancer cells. Frontline drugs, Vinca alkaloids, taxanes, antimetabolites, and nano-sized formulations were tested in a 3D PCI in vitro model. PCI technology enhances therapeutic activity in drug molecules (up to 5000–170
000-fold) with Vinca alkaloids and nanoformulations showing potency, efficacy, and synergy.320
Bluette et al., 2021 developed a nanoparticle vincristine (VCR) formulation to alleviate chronic ischemic neuropathy (CIPN) in nonclinical animals. Nano-VCR and empty nanoparticles do not affect gait parameters, while solution-based VCR reduces run speed and increases step cycle (P < 0.05), with no sciatic nerve degeneration. Hence, nano-VCR formulation alleviates behavioral changes in the peripheral nerve without structural improvements; hence optimization is needed for better observation.321
Wang et al., 2021 fabricated liposome polymeric micelles (PMs) loaded with vinorelbine (NVB) and cisplatin (CDDP) for NSCLC treatment, addressing high mortality and poor prognosis. CoNP-lips, with a spherical shape and uniform size distribution (162.97 ± 9.06 nm), show synergistic cytotoxicity in NSCLC cell lines, a higher plasma half-life than NP solution, antitumor efficacy in C57BL/6 mice and enhanced permeability and a retention effect in tumors.322
Mohsen et al. (2024) developed a polyelectrolyte complex (PEC) carrier system for vinorelbine tartrate used in cancer treatment. The PEC was prepared by entrapping vinorelbine tartrate using gum kondagogu and chitosan as polymers. Various formulations were optimized by adjusting the ratios of these polymers. The highest PEC output was achieved with gum kondagogu concentrations exceeding 80%. The PEC demonstrates reduced drug release in 0.1 N HCl compared to phosphate buffer (pH 6.8), with increased drug release and swelling as the gum kondagogu concentration rose. The formulation with a higher chitosan content (PEC 1
:
3) shows 98% drug release in 4.5 h. These findings suggest that the gum kondagogu/chitosan-based hydrogels offer significant potential for controlled drug delivery by reducing the dosing frequency for drugs like vinorelbine tartrate.323
3.5 Centella asiatica
Centella asiatica (CA) or Gotu Kola or Jalbrahmi or Indian pennywort or tiger grass is a medicinal herbal perennial plant and a culinary vegetable belonging to the Umbelliferae family which is available in Southeast Asian countries.324–326 Traditionally, it is popular for skin condition therapy such as leprosy, ulcers, eczema, lupus, etc., due to its anti-hepatotoxic, anti-oxidant, anti-inflammatory, anti-microbial, neuroprotective and wound-healing properties.327,328 CA extracts have positive action on the wound healing process as it enhances the synthesis of collagen as well as micro-circulatory functions.329,330 Also, it contains asiatic acid (AA) which has a great potential for keloid (occurs due to collagen deposition and high proliferation, i.e., fibro-proliferative lesions) management, because of abnormal healing beyond wound margins.331 CV induces collagen formation, plays an important role in Transforming Growth Factor-β1 (TGF-β1) and prevents plasminogen activators action by Plasminogen Activator Inhibitor-1 (PAI-1).332 CV is responsible for preventing blood loss and is important for clot dissolution by sealing the blood vessels and protecting them.333 Various triterpenes/centelloids are present in CA, i.e., asiaticoside (AS), madecassoside (MS), AA, madecassic acid (MA), brahmoside, brahminoside, brahmic acid, madasiatic acid, centic acid, cenellic acid, centelloside, isothankuniside, thankiniside, etc.334,335 C. asiatica reduces inflammation by bringing up the lessening proteinase and inhibiting lipoxygenase (LOX) activity to inhibit protein denaturation, which can improve rheumatoid arthritis.336 Other phytoconstituents in CA include flavonoids, saponins, sesquiterpenes, eugenol, catechin, epicatechin, kaempferol, quercetin and plant sterols.337 AA is one of the most important chemical constituents with a pivotal role in the wound healing process.338 CA has potential therapeutics in respiratory, endocrine, digestive, neurological, cardiovascular and dermatological diseases.339 AS promotes cell migration, attachment and growth of normal human skin.340 CA is very effective for the treatment of wound burns, wound infections and hyper-trophic scars.341 It accounts for the rapid epithelization and induces angiogenesis that promotes the wound healing process.342 It is also used as a brain-tonic.343 It is rich in niacin, carotene, and vitamins (C, B1, B2, and A).344 Table 6 provides a comprehensive analysis of Centella asiatica-loaded formulations, highlighting their therapeutic efficacy in promoting wound healing across multiple clinical applications.
Table 6 Applications of Centella asiatica-loaded formulations
Components |
Formulation |
Applications |
Reference |
Chitosan-sodium tripolyphosphate + polyvinyl alcohol (PVA) + Centella asiatica |
Nanocomposite |
Bacterial penetration |
345 |
PVA + Centella asiatica |
Nanofibers |
Antibacterial |
346 |
Gelatin/chitosan + Centella asiatica + Phellodendron amurense |
Patches |
Accelerate skin fibroblast cell viability |
347 |
PVA + Centella asiatica (CA) + NH4I |
Polymer electrolytes |
Wound healing |
348 |
Chitosan + Centella asiatica + ethanol extract |
Microneedles |
Wound healing |
349 |
Poly(hydroxybutyrate-co-hydroxyvalerate) and carboxymethylcellulose (CMC) + Centella asiatica |
Nanocomposite |
Anti-proliferative |
350 |
Centella asiatica + hyaluronic acid |
Hydrogel |
Self-healing |
351 |
Collagen + gelatin + Centella asiatica |
Nanocomposite |
Tissue repair |
352 |
CMC + gelatin + Centella asiatica |
Nanofilm |
Antioxidant |
353 |
CA + polyurethane + asiaticoside + silver nanoparticles + CMC |
Dressings |
Traumatic dermal wound |
354 |
CA + CMC + polyethylene oxide + cellulose |
Nanofiber |
Anti-bacterial |
355 |
CA + chitosan + PVA |
Nanogel |
Anti-inflammatory, antioxidant, antibacterial |
356 |
C. asiatica (pegagan) + chitosan |
Nanoparticles |
Diabetic wounds |
357 |
Sukmawan et al., 2021 determined wound healing activity of Ageratum conyzoides L. leaf ethanolic extract + CA + astaxanthin gel. The wound healing activity are 69.36%, 72.51%, 70.14%, 81.70%, 86.54% and 80.21% for the six-treatments: positive (bioplacenton) control, negative (placebo) control, BP5, BU5, BU10 and BP10, respectively. Therefore, BU10 acts as a promising tool that provides the best and highest wound healing activity (p < 0.05).358
Camacho-Alonso et al., 2019 evaluated the effect of porcine acellular urinary bladder matrix (AUBM) + CA extract for healing tongue wounds. For this, four groups were created: Group 1 (Control with no product), Group 2 (CAE), Group 3 (AUBM + orabase), and Group 4 (Orabase). Based on CD31 and histological wound repair, they were arranged as Group 3 > 2 > 4 > 1 and tongue wound % were arranged as least to greatest: 3 < 2 < 4 < 1. Group3 containing AUBM shows a vast difference compared with the other groups. Therefore, CAE and AUBM are effective for the oral tissues and oral mucosa regeneration, respectively.359
Bozkaya et al., 2022 produced antimicrobial nanofiber wound dressing using CA coated silver NPs. The size and zeta potential of NPs are 14.8 ± 7.3 nm and −30.4 mV, respectively. The 12% polycaprolactone (PCL) and 3.5% polyethylene oxide (PEO) solutions were also prepared. In vitro and silver release profile shows that nanofibers show great potential, with cytotoxic and anti-microbial studies indicating that the nanofibers are effective against Staphylococcus, E. coli and Candida albicans, with good biocompatibility for L929 fibroblast cells. Hence, the nanofibers have a promising potential for wound healing.360
Wang et al., 2021 designed sandwich like nanocomposite hydrogel dressing using non-woven fabrics (NF) in the middle, with gelatin + chitosan hydrogel + CA as the base. The hydrogels showed a uniform microporous structure with high water absorbency, and in vitro study also showed sustained release with goof anti-bacterial activity against S. aureus and E. coli. Therefore, the hydrogels show excellent biocompatibility and have a potential in wound healing.361
Liu et al., 2022 fabricated a topical gel by combining CA, nitric oxide (NO), and hydroxyethyl cellulose for diabetic cutaneous ulcers (DCU) for promoting wound healing. This combination accelerates the healing speed of the DCU wounds. The 8% CA total glycoside nitric oxide gel (CATGNOG) shows the best headlining effect on ulcers by inhibiting the bacterial growth on the surface of wounds, thereby relieving the inflammation reaction and promoting DCU healing.362
Tanga et al., 2022 evaluated the efficacy and biocompatibility of CA using CMC on methanol based extract for wound healing progression. The concentrations were prepared as: 0.0%, 0.25%, 0.5% and 1% of CA extract; out of which 0.5% extract showed the highest wound contraction (p < 0.05) in terms of tissue deposition and polymorphonuclear cell infiltration. Therefore, 0.5% CA extract in CMC shows great wound closure progression by enhancing collagen-II and III expression and reducing TGFβ1 expression.363
Wang et al. 2024 developed a PLX/ZnO nanocomposite incorporated with Centella asiatica extract (CAE) for diabetic wound healing. The ZnO nanoparticles are evenly distributed within the PLX framework, as confirmed by XRD, FTIR, and TEM analyses. The nanocomposite is biocompatible with mouse fibroblast (L929) cells and exhibits key properties, such as rapid self-healing and effective antibacterial activity against Gram-positive and Gram-negative bacteria. The formulation significantly improves cell proliferation, migration, and tube formation in skin fibroblast and HUVEC cell lines. This CAE@PLX/ZnO nanoformulation offers a promising approach for multi-functional diabetic wound healing treatment.364
4. Clinical trials
4.1 Chitosan
Abdollahimajd et al., 2022 compared the clinical safety and efficacy of chitosan dressing and nano-silver (Anticoat™) dressings for the treatment of refractory diabetic wounds. The dressings were applied on wounds and examined every week. The mean% reduction of the 10-item-Diabetic-Foot-infection (DFI) score were 74.2% and 78.1% in nano-silver and chitosan, respectively; with no toxic events. The above data proves that the chitosan dressing as more effective and safer for diabetic wounds than nano-silver.365
Radhakrishna et al., 2023 evaluated wound healing and hemostatic efficacy of chitosan dressing compared with cotton pressure pack after extraction of tooth in anti-thrombotic patients. Out of 54 subjects, 36 patients were on single anti-thrombotics whereas 19 patients were on dual anti-thrombotics. The time taken to achieve homeostasis by the chitosan dressing was shorter compared with cotton pressure packs (96 ± 4 and 797 ± 23 seconds; P < 0.001). The chitosan group took a similar time to achieve homeostasis of single (90 ± 6 seconds)/dual therapy (109 ± 8 seconds). On the other hand, patients on single therapy in the cotton pressure pack group have less time to achieve homeostasis (726 ± 26 seconds; P < 0.001) compared with the time taken by dual therapy (940 ± 20 seconds). Also, the alveolar healing index was better in chitosan (88.9%) compared with the cotton pressure pack (3.7%). Hence, the chitosan dressing has a future potential in patients with post-operative bleeding either with single or dual anti-thrombotic therapy.366
Slivnik et al., 2024 assessed the efficacy of a chitosan-based gel (ChitoCare) for treating non-healing diabetic foot ulcers (DFUs). Forty-two patients with chronic DFUs were randomized to receive either ChitoCare or a placebo gel for 10 weeks, followed by a 4-week follow-up. At week 10, the ChitoCare group showed 16.7% complete wound closure compared to 4.2% in the placebo group (p = 0.297). The ChitoCare group also had a significantly greater median relative reduction in wound surface area (92% vs. 37%, p = 0.008) and a 4.62-fold higher likelihood of achieving 75% wound closure (p = 0.012). Additionally, the Bates-Jensen Wound Assessment Tool revealed significantly better wound conditions in the ChitoCare group. These findings suggest that the ChitoCare gel significantly enhances the healing of DFUs and offers promising results for chronic wound management.367
4.2 Cellulose
Oliveira et al., 2021 investigated the effectiveness of human recombinant-Epidermal Growth Factor (h-EGF) compared with 2% carboxymethyl cellulose (CMC) in diabetic wound healing. For this, 25 subjects (14: rh-EGF and 11: CMC) were taken with Type-II diabetes. The %reduction in wound area was higher in the intervention group (reduce slough) compared with the control group (p = 0.049). The observations were enhanced granulation and epithelial tissues with reduction in exudate levels in both groups. h-EGF is considered to be effective and feasible as no toxic effects are observed, it causes significant reduction in the wound area and is the safest therapy for chronic wounds.368
Koivuniemi et al., 2020 evaluated the performance of nanofibrillar cellulose (NFC) wound dressing (FibDex®) for donor site treatment against polylactide-based copolymer dressing. About 24 patients were enrolled for skin grafting with mean age of 49 ± 18, and the results showed enhanced skin elasticity properties; therefore, it has potential for the treatment of wounds.369
4.3 Collagen
Miyab et al., 2020 assessed the effect of cheaper, oral collagen-based supplement for the healing process in 31 patients with about 30% body surface area burn. The patients were assigned to receive a collagen supplement or isocaloric placebo. After changes, the concentrations were higher in the collagen group at week 2 (from 29.7 ± 13.6 vs. 17.8 ± 7.5 mg dL−1, P = 0.006 to 13.9 ± 9.8 vs. −1.9 ± 10.3 mg dL−1, P < 0.001) than that of week 4 (from 35.1 ± 7.6 vs. 28.3 ± 8.2 mg dL−1, P = 0.023 to 19.2 ± 7.5 vs. 8.5 ± 10.1 mg dL−1, P = 0.002). Also, the ratio of wound healing was 3.7 times in collagen as that of control group (95% CI: 1.434–9.519, P = 0.007). Therefore, hydrolysed collagen supplement improves the healing of wounds and clinically reduces 20–30% of burns in patients.370
Chandler et al., 2020 evaluated the comparison of diabetic foot ulcer (DFUs) outcomes with a daily saline-moistened gauze dressing: standard of care (SOC) or collagen wound conforming matrix (WCM), made up of 2.6% fibrillar bovine dermal collagen. There is a faster healing rate of the WCM compared with the SOC. Over 4 weeks, the wound area decreased to ≥75% in about 50% patients with WCM compared with 13% for the SOC treatment. Due to the non-toxic, well-tolerated and feasible nature of WCM, it has a potential to treat modality to accelerate the healing rates of DFU.371
Beinz et al., 2024 evaluated the progression of wound healing using three different collagen-based wound dressings—Mucograft® (MG), Mucoderm® (MD), and Fibro-Gide® (FG)—compared to a control (C) group for palatal defects. The study involved 20 participants, each with four palatal defects randomly assigned to the treatment groups. All groups achieved complete wound closure by day 14. At day 7, the control group showed the least remaining open wound (49.3%) compared to FG (70.1%), MD (56.8%), and MG (62.2%), with statistically significant differences between FG and C (p = 0.01) and MD and FG (p = 0.04). Pain levels remained low across all groups, with no participant rating pain higher than 4 out of 10. The results indicate that collagen-based dressings aid in covering open defects but do not significantly accelerate wound closure or reduce pain. FG exhibits slower wound closure than C and MD, despite not being designed for open oral wounds.372
4.4 Alginate
Zhao et al., 2022 explored the timing of change in first dressing with alginate dressing, used after peripherally inserted central catheter (PICC) line insertion in patients with a tumor. About 286 patients with tumors and alginate dressing after PICC were then divided into the control (10) and observation group 1 or OG1 (9) and 2 or OG2 (10); out of which 29 have +ve bacterial cultures. The condition of each patient after 96 h of PICC placement promotes wound healing.373
Loera-Valencia et al., 2022 developed calcium-alginate dressings with ZnO nanoparticle dressings (CAZnODs) for DFU therapy and it was tested on 26 Type 2 diabetes patients for its efficacy. Patients were grouped as G1 (CA NPs), n = 16 and G2 (without NPs), n = 10. In G1, about 75% of wound closure was achieved whereas it was about 71% in G2 (p = 0.011). The average time of healing is 48 days in G1 and 72 days in G2. Hence, the NP dressings avoid the secondary or T2D infection and induce better tissue regeneration.374
Wang et al. (2024) conducted a randomized clinical trial to compare the effectiveness of electrospun poly(L-lactide-co-caprolactone) and formulated the porcine fibrinogen (PLCL/Fg) dressing with alginate dressing for treating diabetic foot ulcers (DFUs). The study included 52 patients with DFUs of 1–20 cm2 and Wagner grade 1 or 2. The participants were randomized to receive PLCL/Fg (n = 26) or alginate dressing (n = 26) for 12 weeks. The results show that 91.7% of patients in the PLCL/Fg group achieve complete healing, compared with 63.6% in the alginate group (p = 0.003). Both groups experience some treatment-related adverse events (20.8% in the PLCL/Fg group and 18.1% in the alginate group). The PLCL/Fg dressing is more effective at promoting wound healing and regulating the wound microenvironment in DFUs.375
4.5 Hyaluronic acid (HA)
Kartika et al., 2021 evaluated autologous platelet rich fibrin (A-PRF) + HA, A-PRF + NaCl 0.9% as a control for the treatment of DFU. Patients with DFU with a wound time of 3 months were randomly arranged into A-PRF + HA, A-PRF and Control groups and examined on day 0, 3 and 7. ARF + HA has an enhanced VEGF from day 0 (232.8 pg mg−1) vs. day 7 (544.5 pg mg−1) and day 3 (p = 0.022) vs. day 7 (p = 0.001). Also, there is decrease in IL-6 in this group from day 0 (106.4 pg mg−1) vs. day 7 (88.7 pg mg−1) compared with the other groups, where it was enhanced. Therefore, the A-PRF + HA group enhances angiogenesis and reduces inflammation in patients with DFU.376
Ibraheem et al., 2022 evaluated the efficacy of 0.01% HA spray Gengigel® and 0.2% HA gel Gengigel® in the extraction wound healing through ruler and digital planimetry method. About 30 healthy females (20–60 years old) were grouped into Test Group A (gel), test Group B (spray) and a control group (without HA). The results in the ruler method in terms of wound closure in the control group, gel group and spray group were 43.01%, 67.01% and 65.82%, respectively. On the other hand, it was 47.97%, 69.08% and 66.94% for control, gel and spray group respectively with the digital planimetry method. Therefore, HA gel has greater potential than the spray due to its better wound closure properties.377
Graziani et al. (2024) conducted a single-center, randomized clinical trial to evaluate the effect of chlorhexidine (CHX)-based mouth rinses on periodontal surgical wound healing. Patients were randomly assigned to one of three groups: (i) CHX + anti-discoloration system (ADS) + hyaluronic acid (HA), (ii) CHX + ADS, or (iii) no treatment (control). Wound healing was assessed using the plaque score, gingival inflammation, and the early healing index (EHI) at 3, 7, and 14 days post-surgery. At 3 days, the CHX + ADS group has significantly improved healing, with lower EHI scores compared to the control (p < 0.01). The CHX + ADS + HA group exhibits enhanced healing, with improved EHI, plaque containment, and reduced gingival inflammation at all-time points (p < 0.01). The study concluded that CHX–ADS mouth rinses improve early wound healing and the addition of HA further promotes soft tissue closure following periodontal surgery.378
4.6 Pectin
Sabando et al., 2020 evaluated and assessed hydrocolloid films by crosslinking pectin/starch blend-loaded with bioactive extracts of Ugni molinae and Gunnera tinctoria leaves to check the healing property on pressure ulcers. The formed films show good water-uptake capacity (100–160%) and inhibit about 50% of topical edematous responses due to the incorporation of leaf extract. The topical application of films shows complete closure of pressure ulcers after 17 days without any toxic effect; therefore, the hydrocolloid matrix exhibits physiochemical properties, which can be used as plant extract carriers with wound healing properties.379
Alsakhawy et al., 2022 utilized Arabic gum (AG)/pectin hydrogel to encapsulate naringin (NAR) for wound healing potential. The hydrogel shows 99.88% ± 0.096% encapsulation efficiency with 16.64% ± 0.013% drug loading%. NAR-loaded hydrogel accelerates the healing of wounds, and increases angiogenesis and collagen deposition due to its potent anti-oxidant property. Also, the hydrogel down regulates (P < 0.001) TNF-α and apoptosis mRNA expression. Hence, the NAR-loaded AG/pectin hydrogel is effective and potent for wound healing purposes.380
Gou et al., 2024 developed a multifunctional carboxymethyl chitosan-based hydrogel dressing (EGF@PDA–CMCS–PE) for diabetic wound healing. The hydrogel incorporates pectin (PE) and polydopamine (PDA) to enhance its mechanical properties, tissue adherence, and water retention. Loaded with recombinant human epidermal growth factor (rhEGF), it exhibits significant antioxidant capacity, scavenging harmful radicals and increasing antioxidant enzyme levels in vivo. The hydrogel demonstrates good biocompatibility, antimicrobial properties, and a sustained EGF release over 120 h. In vivo studies in diabetic mice show a 97.84% wound contraction rate by day 14, with histopathology confirming fibroblast proliferation, neovascularization, and improved wound healing. The EGF@PDA–CMCS–PE hydrogel holds promise as a therapeutic tool for chronic diabetic wounds and future clinical applications.381
4.7 Gelatin
Ehab et al., 2020 compared the effects of Alvogyl vs. an absorbable gelatin sponge for the first time as palatal dressings on post-surgical bleeding, post-operative pain and wound re-epithelization. For this, 36 healthy patients were randomized to receive Alvogyl (18 patients, intervention group) or absorbable gelatin sponge (18 patients, control group). Higher visual analogue scale (VAS) pain scores were reported in the control compared with the intervention group up to 12 days after surgery (from (median [range]) 8.5 [2–10] to 1 [0–2] and from 6 [0–10] to 0 [0–2] respectively), with higher analgesics consumption (from 2 [1–3] to 1 [0–3] and from 1 [0–3] to 0 [0–2] tablets, respectively). At 4 weeks, about 22.2% patients in the intervention group vs. 11.1% patients in the control group showed complete re-epithelization and no post-surgical bleeding of the palatal engraftment site. Therefore, absorbable gelatin sponge has more potential due to its low cost, haemostasis, pain-reduction and re-epithelization properties.382
Li et al., 2022 compared the healing and its outcomes of gelatin sponge patch, ofloxacin otic solution for large traumatic tympanic membrane perforation and healing. About 136 patients with the perforation were included and were divided into three groups: G1 (ofloxacin otic solution), G2 (gelatin sponge patch) and G3 (spontaneous healing). The following closure rates were found: G1-97.6% with a mean time of 13.12 ± 4.61 days, G2-87.2% with a mean time of 16.47 ± 6.24 days and G3-79.2% (P = 0.041) with a mean time of 49.51 ± 18.22 days (P < 0.001). Therefore, G1 and G2 are effective methods for traumatic large tympanic membrane peroration.383
Hussein et al., 2024 evaluated the effect of a melatonin-loaded gelatin sponge on palatal wound healing after graft harvesting. Twenty-six patients were divided into two groups: the test group received the melatonin-loaded sponge, while the control group received a placebo-loaded sponge. Wound healing was assessed clinically using photo-digital planimetry at 7 and 14 days, and histological specimens analyzed. At 7 days, the test group shows significantly reduced raw wound area and increased immature epithelial area compared to the control group. Histologically, melatonin treatment accelerates healing and improves maturation. Pain scores show no significant differences according to the VAS. The study concluded that melatonin-loaded gelatin sponges enhance wound healing, offering a promising new approach to improve palatal wound recovery and reduce morbidity.384
4.8 Ulvan
Mariia et al., 2021 evaluated chitosan–ulvan hydrogel encapsulated with cellulose nanocrystals + Epidermal Growth Factor (CS–U–CNC–EGF) by the freeze drying method for wound healing. The NC encapsulation modifies the porous microstructure (size: 237 ± 59 μm to 53 ± 16 μm) and enhances the curve of mechanical stress (0.57 MPa to 1.2 MPa), and swelling behaviour. The nanocomposites show the best cell proliferation and non-toxic effect and the hydrogel shows sustained release of EGF. Hence the hydrogel enhances the EGF delivery at the site of the wound for 15 days from the 100% wound contraction treated group. Therefore, the study proved that the hydrogel has faster wound healing efficiency.385
Kikionis et al., 2022 evaluated and investigated the non-woven nanofibrous patches with a ulvan + polyethylene oxide (PEO) patch for anti-inflammatory properties for keloid treatment. Twenty-four patients volunteered for cryosurgery or to apply the ulvan/PEO patch for about 21 days. The results showed significant wound healing, skin inflammation elimination, and biological–physical parameter restoration with no side effects. Hence, the designed patches potentially heal skin trauma after keloid cryo-surgical treatment.386
5. Patents
S. no. |
Patent number |
Biopolymer |
Goal |
Reference |
1 |
CN113372585A (2021) |
Hyaluronic acid + chitosan |
Hydrogel having self-healing capacity |
387 |
2 |
EP3801654A1 (2021) |
Chitosan + sodium alginate |
Film showing tissue adhesive and healing property |
388 |
3 |
CN118718059A (2024) |
Chitosan |
Chronic wounds, anti-inflammatory effects |
389 |
4 |
DE102018009781A1 (2020) |
Chitosan + gelatin |
Aerosol-forming bubble for high absorption used for wound healing treatment and other skin conditions |
390 |
5 |
CN118236541A (2024) |
Hyaluronic acid |
Accelerates healing of diabetic wounds, manages bacterial infection, reduces inflammation, promotes angiogenesis and collagen deposition |
391 |
6 |
CN112957521A (2022) |
Alginate–silk fibroin |
Hydrogel carrying artemisinin liposome that promotes wound tissue healing |
392 |
7 |
US11571492B2 (2023) |
Starch, chitosan + alginate |
Gel used to enhance adhesive properties |
393 |
8 |
JP2023090744A (2023) |
Alginate + hyaluronate + collagen |
Hydrogel preventing scarring after surgery |
394 |
9 |
CN112741929B (2022) |
Chitosan + sodium alginate |
Composite dressing used as base material and a vacuumizing mode |
395 |
10 |
BR122021015641B1 (2023) |
Cellulose + hyaluronic acid |
Composition used for chronic wound treatment |
396 |
6. Conclusion
The integration of herbal bioactives into biopolymeric formulations has marked a significant progress in wound healing, merging the advantages of natural substances with modern biomedical engineering methods. Biopolymers, including ulvan, hyaluronic acid, collagen, chitosan, cellulose, alginate, starch, gelatin, and pectin, have inherent qualities that facilitate wound healing by maintaining moisture, protecting cells from microbial intrusion, and enhancing cell adhesion and proliferation. The incorporation of herbal bioactives such as curcumin, quercetin, Aloe vera, Vinca alkaloids, and Centella asiatica significantly improves the therapeutic efficacy of these formulations, providing anti-inflammatory, antibacterial, and antioxidant properties that mitigate numerous limitations of traditional wound care treatments. Biopolymeric dressings infused with herbal bioactives can expedite wound healing, reduce infection risk, and lessen consequences such as delayed wound closure. Nonetheless, significant research gaps and limitations persist despite these encouraging developments. A challenge is the inconsistency in the efficacy of herbal bioactives, as their biological activity can be affected by factors such as extraction techniques, doses, and formulation stability. Furthermore, the scalability of production and the standardization of these biopolymeric formulations for extensive clinical application necessitate additional research. A further challenge pertains to the necessity for more extensive clinical research to determine long-term safety and efficacy profiles, especially with chronic wounds. Notwithstanding these limitations, the rising quantity of patents pertaining to these advanced formulations reflects the escalating interest in the commercialization of biopolymeric dressings infused with herbal bioactives. These advances possess significant potential to enhance patient care and quality of life by providing a comprehensive approach to wound healing, integrating ancient herbal remedies with advanced biomedical engineering to meet acute and chronic wound healing requirements.
7. Future perspective
Ongoing research and innovative technology are driving the future of herbal bioactive-loaded biopolymeric formulations in wound healing, which is expected to see transformational advancements. One promising approach is to develop multifunctional dressings that can adapt to different wound microenvironments in real time, considering factors like temperature, pH, and the presence of particular biomarkers. By allowing the controlled release of bioactive chemicals suitable to the particular healing phase of the wound, such responsive materials would improve the therapeutic efficacy. Furthermore, careful investigation of synergistic interactions among various herbal bioactives within single formulations may produce improved therapeutic results. Research endeavors must focus on determining the ideal mixtures and concentrations that enhance bioactive characteristics while reducing potential cytotoxicity or adverse effects. The incorporation of nanotechnology offers a significant opportunity for enhancing the mechanical characteristics and bioactivity of these biopolymeric dressings. Utilizing nanoparticles could lead to improved drug delivery methods or the development of coatings with supplementary antibacterial properties. Longitudinal clinical trials are necessary for these novel formulations to be proven as safe, effective, and standardized across diverse patient demographics and wound types. Artificial intelligence (AI) and machine learning (ML) are progressively employed to develop more efficient wound healing therapies by facilitating the analysis of extensive datasets to forecast ideal biomaterial combinations, formulation methodologies, and healing outcomes. These technologies enhance the optimization of drug delivery systems, personalized treatments, and the discovery of novel bioactive compounds by examining patterns in cellular behavior, inflammatory responses, and tissue regeneration processes, thereby expediting the advancement of more targeted and efficient wound care solutions. Additionally, regulating the development of protocols for the clinical application of these sophisticated dressings will require coordinated efforts among regulatory authorities, academia, and industry. Future developments anticipate more emphasis on sustainability. In line with worldwide sustainability efforts and patient care needs, biopolymeric dressings that prioritize the use of biodegradable and eco-friendly materials are being developed. Overall, next-generation wound care solutions that improve healing trajectories and the patient's quality of life will be made possible by the convergence of advanced materials science, herbal pharmacology, and clinical practice.
Data availability
No new data sets were generated during the study.
Conflicts of interest
There are no conflicts to declare.
References
- A. Sharma, V. Puri, P. Kumar and I. Singh, Rifampicin-loaded alginate-gelatin fibers incorporated within transdermal films as a fiber-in-film system for wound healing applications, Membranes, 2020, 11(1), 7 CrossRef PubMed.
- H. Chopra, S. Kumar and I. Singh, Strategies and therapies for wound healing: a review, Curr. Drug Targets, 2022, 23(1), 87–98 CrossRef CAS PubMed.
- A. Sharma, A. Mittal, V. Puri, P. Kumar and I. Singh, Curcumin-loaded, alginate–gelatin composite fibers for wound healing applications, 3 Biotech, 2020, 10(11), 464 CrossRef PubMed.
- A. Sharma, V. Puri, P. Kumar and I. Singh, Biopolymeric, nanopatterned, fibrous carriers for wound healing applications, Curr. Pharm. Des., 2020, 26(38), 4894–4908 CrossRef CAS PubMed.
- N. Zulkefli, C. N. Che Zahari, N. H. Sayuti, A. A. Kamarudin, N. Saad, H. S. Hamezah, H. Bunawan, S. N. Baharum, A. Mediani, Q. U. Ahmed and A. F. Ismail, Flavonoids as potential wound-healing molecules: Emphasis on pathways perspective, Int. J. Mol. Sci., 2023, 24(5), 4607 CrossRef CAS PubMed.
- L. Fan, C. Xiao, P. Guan, Y. Zou, H. Wen, C. Liu, Y. Luo, G. Tan, Q. Wang, Y. Li and P. Yu, Extracellular matrix-based conductive interpenetrating network hydrogels with enhanced neurovascular regeneration properties for diabetic wounds repair, Adv. Healthcare Mater., 2022, 11(1), 2101556 CrossRef CAS PubMed.
- M. Abazari, A. Ghaffari, H. Rashidzadeh, S. M. Badeleh and Y. Maleki, A systematic review on classification, identification, and healing process of burn wound healing, Int. J. Lower Extremity Wounds, 2022, 21(1), 18–30 CrossRef PubMed.
- M. Li, H. Tang, X. Geng, J. Zhou, S. Mou, C. Li, J. Chang, M. Xu, C. Wang, R. Fu and Y. Wang, All-natural hydrogel composed of carboxymethyl chitosan and oxidized dextran for promoting wound healing by immune-microenvironment regulation, Carbohydr. Polym., 2025, 347, 122731 CrossRef CAS PubMed.
- M. Mirhaj, S. Labbaf, M. Tavakoli and A. M. Seifalian, Emerging treatment strategies in wound care, Int. Wound J., 2022, 19(7), 1934–1954 CrossRef PubMed.
- D. Mijaljica, F. Spada, D. J. Klionsky and I. P. Harrison, Autophagy is the key to making chronic wounds acute in skin wound healing, Autophagy, 2023, 19(9), 2578–2584 CrossRef CAS PubMed.
- L. Qi, C. Zhang, B. Wang, J. Yin and S. Yan, Progress in hydrogels for skin wound repair, Macromol. Biosci., 2022, 22(7), 2100475 CrossRef CAS PubMed.
- S. A. Polshettiwar, D. H. Sawant, N. B. Abhale, N. B. Chavan, A. M. Baheti, M. S. Wani, A. A. Tagalpallewar, C. D. Deshmukh and A. P. Polshettiwar, Review on Regulation of Herbal Products Used as a Medicine Across the Globe: A Case Study on Turmeric—Golden Medicine, Biomed. Pharmacol. J., 2022, 15, 1227–1237 CAS.
- N. Chhikara, A. Kaur, S. Mann, M. K. Garg, S. A. Sofi and A. Panghal, Bioactive compounds, associated health benefits and safety considerations of Moringa oleifera L.: An updated review, Nutr. Food Sci., 2021, 51(2), 255–277 CrossRef.
- B. Patel, V. Kothari and N. Acharya, Mainstreaming traditional practices for wound management, Wound Healing Research: Current Trends and Future Directions, 2021, pp. 219–245 Search PubMed.
- I. A. Duceac and S. Coseri, Biopolymers and their derivatives: Key components of advanced biomedical technologies, Biotechnol. Adv., 2022, 61, 108056 CrossRef CAS PubMed.
- M. Firoozbahr, P. Kingshott, E. A. Palombo and B. Zaferanloo, Recent advances in using natural antibacterial additives in bioactive wound dressings, Pharmaceutics, 2023, 15(2), 644 CrossRef CAS PubMed.
- A. Indurkar, A. Pandit, R. Jain and P. Dandekar, Plant-based biomaterials in tissue engineering, Bioprinting, 2021, 21, e00127 CrossRef.
- M. A. Abourehab, R. R. Rajendran, A. Singh, S. Pramanik, P. Shrivastav, M. J. Ansari, R. Manne, L. S. Amaral and A. Deepak, Alginate as a promising biopolymer in drug delivery and wound healing: A review of the state-of-the-art, Int. J. Mol. Sci., 2022, 23(16), 9035 CrossRef CAS PubMed.
- P. O. Stanescu, I. C. Radu, R. Leu Alexa, A. Hudita, E. Tanasa, J. Ghitman, O. Stoian, A. Tsatsakis, O. Ginghina, C. Zaharia and M. Shtilman, Novel chitosan and bacterial cellulose biocomposites tailored with polymeric nanoparticles for modern wound dressing development, Drug Delivery, 2021, 28(1), 1932–1950 CrossRef CAS PubMed.
- N. Tiwari, D. Kumar, A. Priyadarshani, G. K. Jain, G. Mittal, P. Kesharwani and G. Aggarwal, Recent progress in polymeric biomaterials and their potential applications in skin regeneration and wound care management, J. Drug Delivery Sci. Technol., 2023, 82, 104319 CrossRef CAS.
- T. Khaliq, M. Sohail, M. U. Minhas, S. A. Shah, N. Jabeen, S. Khan, Z. Hussain, A. Mahmood, M. Kousar and H. Rashid, Self-crosslinked chitosan/κ-carrageenan-based biomimetic membranes to combat diabetic burn wound infections, Int. J. Biol. Macromol., 2022, 197, 157–168 CrossRef CAS PubMed.
- R. Yu, H. Zhang and B. Guo, Conductive biomaterials as bioactive wound dressing for wound healing and skin tissue engineering, Nano-Micro Lett., 2022, 14, 1–46 CrossRef CAS PubMed.
- F. Patel, A. Doshi Ankita and D. Baxi, Role of medicinal plants in wound healing: an ethnopharmacological approach, Wound Healing Research: Current Trends and Future Directions, 2021, pp. 177–217 Search PubMed.
- S. El-Ashram, L. M. El-Samad, A. A. Basha and A. El Wakil, Naturally-derived targeted therapy for wound healing: Beyond classical strategies, Pharmacol. Res., 2021, 170, 105749 CrossRef CAS PubMed.
- H. S. Kusuma, G. I. Al Lantip and X. Mutiara, A detail account of natural nanofibers (as chitin/chitosan, cellulose, gelatin, alginate, hyaluronic acid, fibrin, collagen, etc.), in Polymeric Nanofibers and Their Composites, Elsevier Science Ltd, 2025, pp. 91–111 Search PubMed.
- S. Kailasa, M. S. Reddy, M. R. Maurya, B. G. Rani, K. V. Rao and K. K. Sadasivuni, Electrospun nanofibers: materials, synthesis parameters, and their role in sensing applications, Macromol. Mater. Eng., 2021, 306(11), 2100410 CrossRef CAS.
- A. Sharma, D. Dheer, V. Puri, A. Alsayari, S. Wahab and P. Kesharwani, Insights of biopolymeric blended formulations for diabetic wound healing, Int. J. Pharm., 2024, 656, 124099 CrossRef CAS PubMed.
- H. Chopra, S. Bibi, S. Kumar, M. S. Khan, P. Kumar and I. Singh, Preparation and evaluation of chitosan/PVA based hydrogel films loaded with honey for wound healing application, Gels, 2022, 8(2), 111 CrossRef CAS PubMed.
- H. Chopra, S. Bibi, Y. K. Mohanta, T. Kumar Mohanta, S. Kumar, I. Singh, M. Saad Khan, P. Ranjan Rauta, A. Alshammari, M. Alharbi and A. F. Alasmari, In vitro and in Silico characterization of curcumin-loaded chitosan–PVA hydrogels: antimicrobial and potential wound healing activity, Gels, 2023, 9(5), 394 CrossRef CAS PubMed.
- J. Chhabra, H. Chopra, R. Pahwa, N. Raina, K. Wadhwa, S. Saini, P. Negi, M. Gupta, I. Singh, H. Dureja and T. B. Emran, Potential of nanoemulsions for accelerated wound healing: innovative strategies, Int. J. Surg., 2023, 109(8), 2365–2377 CrossRef PubMed.
- A. Sharma, V. Puri, I. S. Bakshi and P. Kumar, Herbal bioactive-incorporated scaffolds for wound healing applications, in Herbal Bioactive-Based Drug Delivery Systems, Academic Press, 2022, pp. 311–330 Search PubMed.
- A. Sharma, H. Chopra, I. Singh and T. B. Emran, Physically and chemically crosslinked hydrogels for wound healing applications, Int. J. Surg., 2022, 106, 106915 CrossRef PubMed.
- R. Singh, S. Gautam, B. Sharma, P. Jain and K. D. Chauhan, Biopolymers and their classifications, in Biopolymers and Their Industrial Applications, Elsevier, 2021, pp. 21–44 Search PubMed.
- S. S. Cai, T. Li, T. Akinade, Y. Zhu and K. W. Leong, Drug delivery carriers with therapeutic functions, Adv. Drug Delivery Rev., 2021, 176, 113884 CrossRef CAS PubMed.
- M. Chen, Y. Wu, B. Chen, A. M. Tucker, A. Jagota and S. Yang, Fast, strong, and reversible adhesives with dynamic covalent bonds for potential use in wound dressing, Proc. Natl. Acad. Sci. U. S. A., 2022, 119(29), e2203074119 CrossRef CAS PubMed.
- G. Divyashri, R. V. Badhe, B. Sadanandan, V. Vijayalakshmi, M. Kumari, P. Ashrit, D. Bijukumar, M. T. Mathew, K. Shetty and A. V. Raghu, Applications of hydrogel-based delivery systems in wound care and treatment: an up-to-date review, Polym. Adv. Technol., 2022, 33(7), 2025–2043 CrossRef CAS.
- N. Mukherjee, S. Ghosh, R. Roy, D. Mukherjee, S. Sen, D. Nandi, J. Sarkar and S. Ghosh, Extracellular matrix mimicking wound microenvironment responsive amyloid–heparin@ TAAgNP co-assembled hydrogel: an effective conductive antibacterial wound healing material, ACS Appl. Mater. Interfaces, 2024, 16(24), 30929–30957 CrossRef CAS PubMed.
- S. Sen, S. Ghosh, A. Jana, M. Jash, S. Ghosh, N. Mukherjee, D. Mukherjee, J. Sarkar and S. Ghosh, Multi-faceted antimicrobial efficacy of a quinoline-derived bidentate copper (II) ligand complex and its hydrogel encapsulated formulation in methicillin-resistant Staphylococcus aureus inhibition and wound management, ACS Appl. Bio Mater., 2024, 7(6), 4142–4161 CrossRef CAS PubMed.
- J. Zhou, K. Li and P. Ramasamy, Chitosan-collagen biopolymer biofilm derived from cephalopod gladius; Evaluation of osteogenesis, angiogenesis and wound healing for tissue engineering application, Int. J. Biol. Macromol., 2024, 279, 135078 CrossRef CAS PubMed.
- S. F. Abaza, N. S. Elbialy, R. Darwesh and N. Mohamed, Reinforcing chitosan film with a natural nanofiller “Zein-methyl cellulose loaded curcumin” for improving its physicochemical properties and wound healing activity, Carbohydr. Polym. Technol. Appl., 2024, 8, 100593 CAS.
- Y. Ren, A. Aierken, L. Zhao, Z. Lin, J. Jiang, B. Li, J. Wang, J. Hua and Q. Tu, hUC-MSCs lyophilized powder loaded polysaccharide ulvan driven functional hydrogel for chronic diabetic wound healing, Carbohydr. Polym., 2022, 288, 119404 CrossRef CAS PubMed.
- N. R. Naveen, P. Anitha, B. Gowthami, P. Goudanavar and S. Fattepur, QbD assisted formulation design and optimization of thiol pectin based Polyethyleneglycol and Montmorillonite (PEG/MMT) nanocomposite films of neomycin sulphate for wound healing, J. Drug Delivery Sci. Technol., 2023, 82, 104348 CrossRef.
- M. Khalid, F. Jameel, T. Jabri, A. Jabbar, A. Salim, I. Khan and M. R. Shah, α-Terpineol loaded, electron beam crosslinked polyvinyl alcohol/tapioca starch hydrogel sheets; fabrication, characterization and evaluation of wound healing potential on a full thickness acid burn wound, RSC Adv., 2024, 14(38), 28058–28076 RSC.
- M. Brugnoli, J. P. Carvalho, M. P. Arena, H. Oliveira, C. Vilela, C. S. Freire and M. Gullo, Co-cultivation of Komagataeibacter sp. and Lacticaseibacillus sp. strains to produce bacterial nanocellulose-hyaluronic acid nanocomposite membranes for skin wound healing applications, Int. J. Biol. Macromol., 2025, 21, 140208 CrossRef PubMed.
- D. Zahra, Z. Shokat, M. Sufyan, Z. Chaudhary and U. A. Ashfaq, Exploring the potential of chitosan polyherbal hydrogel loaded with AgNPs to enhance wound healing A triangular study, Int. J. Biol. Macromol., 2024, 281, 135896 CrossRef CAS PubMed.
- H. Zhu, H. T. Ao, Y. Fu, C. Zou, Z. Chen, Z. Jin, H. Zhou, B. Sun and S. Guo, Optimizing alginate dressings with allantoin and chemical modifiers to promote wound healing, Int. J. Biol. Macromol., 2024, 275, 133524 CrossRef CAS PubMed.
- S. Borse, S. Nangare, P. Bafna, P. Jain and L. Zawar, Barbaloin loaded chitosan-gum kondagogu polyelectrolyte complex based biocomposites film for enhanced antibacterial, antioxidant and wound healing activity, Surf. Interfaces, 2025, 56, 105506 CrossRef CAS.
- R. Najafloo, P. B. Milan, A. Karimi, Z. Bagher, R. R. Kalmer, M. Ghasemian and R. Faridi-Majidi, Crosslinking gelatin with robust inherent antibacterial natural polymer for wound healing, Int. J. Biol. Macromol., 2024, 280, 136144 CrossRef CAS PubMed.
- A. Hivechi, H. Yousefmoumji, S. H. Bahrami and P. B. Milan, Fabrication and characterization of in situ gelling oxidized carboxymethyl cellulose/gelatin nanofibers for wound healing applications, Int. J. Biol. Macromol., 2025, 17, 140033 CrossRef PubMed.
- E. Solhtalab, I. Nikokar, A. Mojtahedi, R. Shokri, P. Karimian, E. Mahdavi and S. Faezi, Encapsulation of Mentha aquatica methanol extract in alginate hydrogel promotes wound healing in a murine model of Pseudomonas aeruginosa burn infection, Int. J. Biol. Macromol., 2024, 280, 135920 CrossRef CAS PubMed.
- B. Azimi, H. Maleki, L. Zavagna, J. G. De la Ossa, S. Linari, A. Lazzeri and S. Danti, Bio-based electrospun fibers for wound healing, J. Funct. Biomater., 2020, 11(3), 67 CrossRef CAS PubMed.
- N. Raina, R. Rani, R. Pahwa and M. Gupta, Biopolymers and treatment strategies for wound healing: an insight view, Int. J. Polym. Mater. Polym. Biomater., 2022, 71(5), 359–375 CrossRef CAS.
- R. Gobi, P. Ravichandiran, R. S. Babu and D. J. Yoo, Biopolymer and synthetic polymer-based nanocomposites in wound dressing applications: a review, Polymers, 2021, 13(12), 1962 CrossRef CAS PubMed.
- C. Kalirajan and T. Palanisamy, Bioengineered Hybrid Collagen Scaffold Tethered with Silver-Catechin Nanocomposite Modulates Angiogenesis and TGF-β Toward Scarless Healing in Chronic Deep Second Degree Infected Burns, Adv. Healthcare Mater., 2020, 9(12), 2000247 CrossRef CAS PubMed.
- Fibrous Proteins: Design, Synthesis, and Assembly, ed. S. Ling, Humana Press, 2021 Search PubMed.
- G. Singh, S. Senapati, S. Satpathi, P. K. Behera, B. Das and B. Nayak, Establishment of decellularized extracellular matrix scaffold derived from caprine pancreas as a novel alternative template over porcine pancreatic scaffold for prospective biomedical application, FASEB J., 2022, 36(10), e22574 CrossRef CAS PubMed.
- A. Hajialiasgary Najafabadi, M. H. Soheilifar and N. Masoudi-Khoram, Exosomes in skin photoaging: biological functions and therapeutic opportunity, Cell Commun. Signaling, 2024, 22, 32 CrossRef CAS PubMed.
- H. Zhang, S. Wang, C. Lei, G. Li and B. Wang, Experimental study of negative pressure wound therapy combined with platelet-rich fibrin for bone-exposed wounds, Regener. Med., 2022, 17(1), 23–35 CrossRef CAS PubMed.
- S. D. Kim, S. Jin, S. Kim, D. Son and M. Shin, Tyramine-functionalized alginate-collagen hybrid hydrogel inks for 3D-bioprinting, Polymers, 2022, 14(15), 3173 Search PubMed.
- X. Wang, D. Zhao, Y. Li, X. Zhou, Z. Hui, X. Lei, L. Qiu, Y. Bai, C. Wang, J. Xia and Y. Xuan, Collagen hydrogel with multiple antimicrobial mechanisms as anti-bacterial wound dressing, Int. J. Biol. Macromol., 2023, 232, 123413 CrossRef CAS PubMed.
- Y. Yang, R. Xu, C. Wang, Y. Guo, W. Sun and L. Ouyang, Recombinant human collagen-based bioinks for the 3D bioprinting of full-thickness human skin equivalent, Int. J. Bioprint., 2022, 8(4), 611 CrossRef CAS PubMed.
- T. Jiao, Q. Lian, W. Lian, Y. Wang, D. Li, R. L. Reis and J. M. Oliveira, Properties of collagen/sodium alginate hydrogels for bioprinting of skin models, J. Bionic Eng., 2023, 20(1), 105–118 CrossRef.
- C. Fu, S. Shi, J. Tian, H. Gu, L. Yao and J. Xiao, Non-denatured yak type I collagen accelerates sunburned skin healing by stimulating and replenishing dermal collagen, Biotechnol. Rep, 2023, 37, e00778 CrossRef CAS PubMed.
- M. Kumar, S. Mahmood, S. Chopra and A. Bhatia, Biopolymer based nanoparticles and their therapeutic potential in wound healing–A review, Int. J. Biol. Macromol., 2024, 10, 131335 CrossRef PubMed.
- R. Khan, M. D. Shah, L. Shah, P. C. Lee and I. Khan, Bacterial polysaccharides—A big source for prebiotics and therapeutics, Front. Nutr., 2022, 9, 1031935 CrossRef PubMed.
- S. O. Serna Saldívar and F. E. Ayala Soto, Chemical composition and biosynthesis of dietary fiber components. Science and Technology of Fibers in Food Systems, 2020, pp. 15–43 Search PubMed.
- M. Butnariu and A. I. Flavius, General information about cellulose, J. Biotechnol. Bioprocess, 2022, 3, 1–5 Search PubMed.
- F. Fahma, A. Firmanda, J. Cabral, D. Pletzer, J. Fisher, B. Mahadik, I. W. Arnata, D. Sartika and A. Wulandari, Three-dimensional printed cellulose for wound dressing applications, 3D printing and additive manufacturing, 2023, 10(5), 1015–1035 CrossRef PubMed.
- M. Gruppuso, G. Turco, E. Marsich and D. Porrelli, Polymeric wound dressings, an insight into polysaccharide-based electrospun membranes, Appl. Mater. Today, 2021, 24, 101148 CrossRef.
- L. Qi, C. Zhang, B. Wang, J. Yin and S. Yan, Progress in hydrogels for skin wound repair, Macromol. Biosci., 2022, 22(7), 2100475 CrossRef CAS PubMed.
- X. Geng, Y. Qi, X. Liu, Y. Shi, H. Li and L. Zhao, A multifunctional antibacterial and self-healing hydrogel laden with bone marrow mesenchymal stem cell-derived exosomes for accelerating diabetic wound healing, Biomater. Adv., 2022, 133, 112613 CrossRef CAS PubMed.
- S. S. Biranje, J. Sun, L. Cheng, Y. Cheng, Y. Shi, S. Yu, H. Jiao, M. Zhang, X. Lu, W. Han and Q. Wang, Development of cellulose nanofibril/casein-based 3D composite hemostasis scaffold for potential wound-healing application, ACS Appl. Mater. Interfaces, 2022, 14(3), 3792–3808 CrossRef CAS PubMed.
- Y. He, R. Chen, C. Zhao, Q. Lu, Z. Chen, H. Zhu, Q. Bu, L. Wang and H. He, Design of near-infrared-triggered cellulose nanocrystal-based in situ intelligent wound dressings for drug-resistant bacteria-infected wound healing, ACS Appl. Mater. Interfaces, 2022, 14(46), 51630–51644 CrossRef CAS PubMed.
- S. Wang, Z. Zhao, S. Yang and X. Zhang, Development of a functional hydrogel incorporating hydroxyapatite nanoparticles for enhanced wound healing and bone tissue regeneration, Adv. Funct. Mater., 2025, 35, 2200–2215 Search PubMed.
- L. L. Munhoz, M. T. Alves, B. C. Alves, M. G. Nascimento, R.
M. Sábio, K. F. Manieri, H. S. Barud, M. A. Esquisatto, A. A. Aro, L. de Roch Casagrande and P. C. Silveira, Bacterial cellulose membrane incorporated with silver nanoparticles for wound healing in animal model, Biochem. Biophys. Res. Commun., 2023, 654, 47–54 CrossRef CAS PubMed.
- N. Patil and S. Wairkar, Chitosan and α-cellulose-based mupirocin topical film-forming spray: Optimization, in vitro characterization, antimicrobial studies and wound healing activity, Int. J. Biol. Macromol., 2024, 254, 127622 CrossRef CAS PubMed.
- S. F. Abaza, N. S. Elbialy, R. Darwesh and N. Mohamed, Reinforcing chitosan film with a natural nanofiller “Zein-methyl cellulose loaded curcumin” for improving its physicochemical properties and wound healing activity, Carbohydr. Polym. Technol. Appl., 2024, 8, 100593 CAS.
- T. Huq, A. Khan, D. Brown, N. Dhayagude, Z. He and Y. Ni, Sources, production and commercial applications of fungal chitosan: A review, J. Bioresour. Bioprod., 2022, 7(2), 85–98 CAS.
- M. Butnariu, Biological and chemical aspects of chitosan, in Chitosan Nanocomposites: Bionanomechanical Applications, Springer Nature Singapore, Singapore, 2023, pp. 27–54 Search PubMed.
- J. Mao, L. Chen, Z. Cai, S. Qian, Z. Liu, B. Zhao, Y. Zhang, X. Sun and W. Cui, Advanced biomaterials for regulating polarization of macrophages in wound healing, Adv. Funct. Mater., 2022, 32(12), 2111003 CrossRef CAS.
- R. Eivazzadeh-Keihan, E. B. Noruzi, S. F. Mehrban, H. A. Aliabadi, M. Karimi, A. Mohammadi, A. Maleki, M. Mahdavi, B. Larijani and A. E. Shalan, The latest advances in biomedical applications of chitosan hydrogel as a powerful natural structure with eye-catching biological properties, J. Mater. Sci., 2022, 1, 1–37 Search PubMed.
- D. Gheorghiţă, H. Moldovan, A. Robu, A. I. Biţa, E. Grosu, A. Antoniac, I. Corneschi, I. Antoniac, A. D. Bodog and C. I. Băcilă, Chitosan-based biomaterials for hemostatic applications: a review of recent advances, Int. J. Mol. Sci., 2023, 24(13), 10540 CrossRef PubMed.
- X. Zeng, B. Chen, L. Wang, Y. Sun, Z. Jin, X. Liu, L. Ouyang and Y. Liao, Chitosan@ Puerarin hydrogel for accelerated wound healing in diabetic subjects by miR-29ab1 mediated inflammatory axis suppression, Bioact. Mater., 2023, 19, 653–665 CAS.
- U. Anushree, P. Punj and B. S. Vasumathi, Phosphorylated chitosan accelerates dermal wound healing in diabetic wistar rats, Glycoconjugate J., 2023, 40(1), 19–31 CrossRef CAS PubMed.
- L. T. Le, N. N. Giang, P. N. Chien, X. T. Trinh, N. V. Long, L. T. Van Anh, P. T. Nga, X. R. Zhang, S. Y. Nam and C. Y. Heo, Enhancement of wound healing efficacy by chitosan-based hydrocolloid on Sprague Dawley rats, In Vivo, 2023, 37(3), 1052–1064 CrossRef CAS PubMed.
- M. C. Linju and M. R. Rekha, Proline conjugated chitosan as wound healing material: In vitro studies on the influence of the scaffold on collagen production and wound healing, Int. J. Biol. Macromol., 2023, 242, 124688 CrossRef CAS PubMed.
- V. M. Moreira, J. M. Leite, K. D. Medeiros, K. M. Assis, J. C. Borges, L. M. Santana, L. M. Moreira, L. P. Alves, T. K. Oliveira, J. W. Silveira and D. T. Silva, Pentoxifylline/chitosan films on wound healing: In vitro/in vivo evaluation, Pharmaceutics, 2023, 15(4), 1122 CrossRef CAS PubMed.
- H. Yang, J. Meng and L. Shi, Novel Fabrication of Bio-polymeric Nanogel Loaded with Nalidixic Acid Formulations for the Effective Prevention of Bacterial Pathogens Leading Urinary Tract Infection and Its Biosafety Evaluation, Curr. Microbiol., 2024, 81(10), 347 CrossRef CAS PubMed.
- K. Khoshmaram, F. Yazdian, Z. Pazhouhnia and N. Lotfibakhshaiesh, Preparation and characterization of 3D bioprinted gelatin methacrylate hydrogel incorporated with curcumin loaded chitosan nanoparticles for in vivo wound healing application, Biomater. Adv., 2024, 156, 213677 CrossRef CAS PubMed.
- J. O. Jin, D. Yadav, K. Madhwani, N. Puranik, V. Chavda and M. Song, Seaweeds in the oncology arena: Anti-cancer potential of fucoidan as a drug—A review, Molecules, 2022, 27(18), 6032 CrossRef CAS PubMed.
- M. Gisbert, D. Franco, J. Sineiro and R. Moreira, Antioxidant and antidiabetic properties of phlorotannins from Ascophyllum nodosum seaweed extracts, Molecules, 2023, 28(13), 4937 CrossRef CAS PubMed.
- M. Minsart, S. Van Vlierberghe, P. Dubruel and A. Mignon, Commercial wound dressings for the treatment of exuding wounds: an in-depth physico-chemical comparative study, Burns Trauma, 2022, 10, tkac024 CrossRef PubMed.
- V. Hegde, U. T. Uthappa, T. Altalhi, H. Y. Jung, S. S. Han and M. D. Kurkuri, Alginate based polymeric systems for drug delivery, antibacterial/microbial, and wound dressing applications, Mater. Today Commun., 2022, 33, 104813 CrossRef CAS.
- M. M. Saraiva, M. D. Campelo, J. F. Camara Neto, A. B. Lima, G. D. Silva, A. T. Dias, N. M. Ricardo, D. L. Kaplan and M. E. Ribeiro, Alginate/polyvinyl alcohol films for wound healing: Advantages and challenges, J. Biomed. Mater. Res., Part B, 2023, 111(1), 220–233 CrossRef CAS PubMed.
- H. Mndlovu, L. C. du Toit, P. Kumar and Y. E. Choonara, Tannic acid-loaded chitosan-RGD-alginate scaffolds for wound healing and skin regeneration, Biomed. Mater., 2023, 18(4), 045009 CrossRef CAS PubMed.
- J. Chen, Y. Xia, Q. Lan, M. Hu, Y. Xu, Q. Wu, X. Liu and Y. Liu, Alginate based photothermal cryogels boost ferrous-supply for enhanced antibacterial chemodynamic therapy and accelerated wound healing, Int. J. Biol. Macromol., 2023, 232, 123473 CrossRef CAS PubMed.
- E. Cruz Sánchez, M. T. García, J. Pereira, F. Oliveira, R. Craveiro, A. Paiva, I. Gracia, J. M. García-Vargas and A. R. Duarte, Alginate–chitosan membranes for the encapsulation of lavender essential oil and development of biomedical applications related to wound healing, Molecules, 2023, 28(9), 3689 CrossRef PubMed.
- S. P. Ndlovu, K. S. Motaung, S. A. Adeyemi, P. Ubanako, L. Ngema, T. Y. Fonkui, D. T. Ndinteh, P. Kumar, Y. E. Choonara and B. A. Aderibigbe, Sodium alginate-based nanofibers loaded with Capparis sepiaria plant extract for wound healing, J. Biomater. Sci., Polym. Ed., 2024, 35(15), 2380–2401 CrossRef CAS PubMed.
- L. K. Sellappan and S. Manoharan, Fabrication of bioinspired keratin/sodium alginate based biopolymeric mat loaded with herbal drug and green synthesized zinc oxide nanoparticles as a dual drug antimicrobial wound dressing, Int. J. Biol. Macromol., 2024, 259, 129162 CrossRef CAS PubMed.
- S. F. Kabir, A. Rahman, F. Yeasmin, S. Sultana, R. A. Masud, N. A. Kanak and P. Haque, Occurrence, distribution, and structure of natural polysaccharides, in Radiation-processed Polysaccharides, Academic Press, 2022, pp. 1–27 Search PubMed.
- S. H. Musa and B. S. Sagagi, Extraction and some characteristics of mango seed kernel starch for industrial applications, Sci. Lett., 2023, 17(2), 81–90 Search PubMed.
- A. O. Ijaola, D. O. Akamo, F. Damiri, C. J. Akisin, E. A. Bamidele, E. G. Ajiboye, M. Berrada, V. O. Onyenokwe, S. Y. Yang and E. Asmatulu, Polymeric biomaterials for wound healing applications: A comprehensive review, J. Biomater. Sci., Polym. Ed., 2022, 33(15), 1998–2050 CrossRef CAS PubMed.
- S. Hasan, M. A. Hasan, M. U. Hassan, M. Amin, T. Javed and L. Fatima, Biopolymers in diabetic wound care management: A potential substitute to traditional dressings, Eur. Polym. J., 2023, 189, 111979 CrossRef CAS.
- M. A. Shalaby, M. M. Anwar and H. Saeed, Nanomaterials for application in wound Healing: Current state-of-the-art and future perspectives, J. Polym. Res., 2022, 29(3), 91 CrossRef CAS.
- N. Abuhamed, Z. Ahmad and N. Sarifuddin, Thermoplastic sago starch nanocomposites wound dressing fortified with antibiotic-modified HNT, in IOP Conference Series: Materials Science and Engineering, IOP Publishing, 2021, vol. 1192, No. 1, p. 012030 Search PubMed.
- L. Guo, N. Yang, W. Gao, H. Tao, B. Cui, P. Liu, F. Zou, L. Lu, Y. Fang and Z. Wu, Self-healing properties of retrograded starch films with enzyme-treated waxy maize starch as healing agent, Carbohydr. Polym., 2023, 299, 120238 CrossRef CAS PubMed.
- B. Huang, L. Zhu, S. Wei, Y. Li, Y. Nie and W. Zhao, Starch-Based Ion-Conductive Organo-Hydrogels with Self-Healing, Anti-Freezing, and High Mechanical Properties toward Strain Sensors, Macromol. Rapid Commun., 2023, 44(7), 2200890 CrossRef CAS PubMed.
- P. Lopes, A. S. Joaquinito, A. Ribeiro, N. M. Moura, A. T. Gomes, S. G. Guerreiro, M. A. Faustino, A. Almeida, P. Ferreira, M. A. Coimbra and M. G. Neves, Starch-based films doped with porphyrinoid photosensitizers for active skin wound healing, Carbohydr. Polym., 2023, 313, 120894 CrossRef CAS PubMed.
- A. Joseph, A. S. Vijayan, J. Xavier, M. KB, A. Karthikeyan, N. Gopinath, M. PV and B. G. Nair, 3D printed arrowroot starch-gellan scaffolds for wound healing applications, Int. J. Biol. Macromol., 2024, 264, 130604 CrossRef CAS PubMed.
- M. Khalid, F. Jameel, T. Jabri, A. Jabbar, A. Salim, I. Khan and M. R. Shah, α-Terpineol loaded, electron beam crosslinked polyvinyl alcohol/tapioca starch hydrogel sheets; fabrication, characterization and evaluation of wound healing potential on a full thickness acid burn wound, RSC Adv., 2024, 14(38), 28058–28076 RSC.
- C. Buckley, E. J. Murphy, T. R. Montgomery and I. Major, Hyaluronic acid: A review of the drug delivery capabilities of this naturally occurring polysaccharide, Polymers, 2022, 14(17), 3442 CrossRef CAS PubMed.
- X. Lin, C. T. Tsao, M. Kyomoto and M. Zhang, Injectable natural polymer hydrogels for treatment of knee osteoarthritis, Adv. Healthcare Mater., 2022, 11(9), 2101479 CrossRef CAS PubMed.
- Q. Liu, L. Hu, C. Wang, M. Cheng, M. Liu, L. Wang, P. Pan and J. Chen, Renewable marine polysaccharides for microenvironment-responsive wound healing, Int. J. Biol. Macromol., 2023, 225, 526–543 Search PubMed.
- Y. W. Ding, Z. Y. Wang, Z. W. Ren, X. W. Zhang and D. X. Wei, Advances in modified hyaluronic acid-based hydrogels for skin wound healing, Biomater. Sci., 2022, 10(13), 3393–3409 Search PubMed.
- H. S. AlSalem and A. A. Bukhari, Biodegradable wound dressing-based collagen/hyaluronic acid loaded antibacterial agents for wound healing application, Int. J. Biol. Macromol., 2023, 242, 124700 Search PubMed.
- Q. Bai, Q. Gao, F. Hu, C. Zheng, W. Chen, N. Sun, J. Liu, Y. Zhang, X. Wu and T. Lu, Chitosan and hyaluronic-based hydrogels could promote the infected wound healing, Int. J. Biol. Macromol., 2023, 232, 123271 CrossRef CAS PubMed.
- Y. Lin, J. Xu, Y. Dong, Y. Wang, C. Yu, Y. Li, C. Zhang, Q. Chen, S. Chen and Q. Peng, Drug-free and non-crosslinked chitosan/hyaluronic acid
hybrid hydrogel for synergistic healing of infected diabetic wounds, Carbohydr. Polym., 2023, 314, 120962 CrossRef CAS PubMed.
- C. Eeckhout, J. Ackerman, M. Glibert and J. Cosyn, A randomized controlled trial evaluating hyaluronic acid gel as wound healing agent in alveolar ridge preservation, J. Clin. Periodontol., 2022, 49(3), 280–291 Search PubMed.
- J. H. Lee, K. E. Lee, O. H. Nam, Y. K. Chae, M. H. Lee, D. K. Kweon, M. S. Kim, H. S. Lee and S. C. Choi, Orodispersible hyaluronic acid film delivery for oral wound healing in rats, J. Dent. Sci., 2022, 17(4), 1595–1603 Search PubMed.
- S. H. Mosawi and E. Rezaei Niaraki, Advanced formulations of hyaluronic acid and its derivatives for antimicrobial, wound healing, tissue engineering, and anticancer applications, Micro Nano Bio Aspects, 2024, 3(2), 7–13 Search PubMed.
- S. Katiyar, A. D. Tripathi, R. K. Singh, A. K. Chaurasia, P. K. Srivastava and A. Mishra, Graphene-silymarin-loaded chitosan/gelatin/hyaluronic acid hybrid constructs for advanced full-thickness burn wound management, Int. J. Pharm., 2024, 659, 124238 Search PubMed.
- F. Alam, S. Das, P. Kalita, B. J. Pathak, M. I. Judder, D. Dasgupta and D. Sarkar, Development and Evaluation of Natural Anti-dandruff Shampoo, J. Nat. Rem., 2023, 31, 1125–1134 Search PubMed.
- N. S. Said and N. M. Sarbon, Physical and mechanical characteristics of gelatin-based films as a potential food packaging material: A review, Membranes, 2022, 12(5), 442 Search PubMed.
- R. Yu, H. Zhang and B. Guo, Conductive biomaterials as bioactive wound dressing for wound healing and skin tissue engineering, Nano-Micro Lett., 2022, 14, 1–46 CrossRef CAS PubMed.
- Q. Luo, M. A. Hossen, Y. Zeng, J. Dai, S. Li, W. Qin and Y. Liu, Gelatin-based composite films and their application in food packaging: A review, J. Food Eng., 2022, 313, 110762 CrossRef CAS.
- F. Mirjalili and M. Mahmoodi, Controlled release of protein from gelatin/chitosan hydrogel containing platelet-rich fibrin encapsulated in chitosan nanoparticles for accelerated wound healing in an animal model, Int. J. Biol. Macromol., 2023, 225, 588–604 CrossRef CAS PubMed.
- S. A. Razack, Y. Lee, H. Shin, S. Duraiarasan, B. S. Chun and H. W. Kang, Cellulose nanofibrils reinforced chitosan-gelatin based hydrogel loaded with nanoemulsion of oregano essential oil for diabetic wound healing assisted by low level laser therapy, Int. J. Biol. Macromol., 2023, 226, 220–239 Search PubMed.
- Y. Lu, X. Zhu, C. Hu, P. Li, M. Zhao, J. Lu and G. Xia, A fucoidan-gelatin wound dressing accelerates wound healing by enhancing antibacterial and anti-inflammatory activities, Int. J. Biol. Macromol., 2022, 223, 36–48 Search PubMed.
- Y. Asada, S. Koshinuma, M. Mikami, Y. Shirai, Y. Machida, T. Nakayama, R. Kushima, G. Yamamoto and K. I. Mukaisho, Comparison of the wound-healing efficacy of gelatin sponge dressings and that of artificial dermis using atelocollagen in a rat cranial periosteal defect model, Exp. Anim., 2022, 71(2), 161–172 CrossRef CAS PubMed.
- K. Khoshmaram, F. Yazdian, Z. Pazhouhnia and N. Lotfibakhshaiesh, Preparation and characterization of 3D bioprinted gelatin methacrylate hydrogel incorporated with curcumin loaded chitosan nanoparticles for in vivo wound healing application, Biomater. Adv., 2024, 156, 213677 CrossRef CAS PubMed.
- S. Bessalah, A. Faraz, M. Dbara, T. Khorcheni, M. Hammadi, D. J. Ajose and S. I. Saeed, Antibacterial, Anti-Biofilm, and Anti-Inflammatory Properties of Gelatin–Chitosan–Moringa-Biopolymer-Based Wound Dressings towards Staphylococcus aureus and Escherichia coli, Pharmaceuticals, 2024, 17(5), 545 CrossRef CAS PubMed.
- M. Zain, S. Nayab, Z. Rashid, A. Aleem, H. Raza and M. D. Yousif, Biosynthesis and in vivo wound healing abilities of Dactyloctenium aegyptium-mediated silver nanoparticles
used as hydrogel dressing, Process Biochem., 2024, 147, 31–38 Search PubMed.
- A. Lewis, Identification and Characterisation of an Enzyme from Monoglobus Pectinilyticus Associated with Degradation of Pectin from Kiwiberry: a Thesis Presented in Partial Fulfilment of the Requirements for the Degree of Master of Science in Biological Sciences at Massey University, Doctoral dissertation, Massey University, Palmerston North, New Zealand, 2022 Search PubMed.
- B. Neamtu, A. Barbu, M. O. Negrea, C. Ş. Berghea-Neamţu, D. Popescu, M. Zăhan and V. Mireşan, Carrageenan-based compounds as wound healing materials, Int. J. Mol. Sci., 2022, 23(16), 9117 CrossRef CAS PubMed.
- B. E. Teleky, L. Mitrea, D. Plamada, S. A. Nemes, L. F. Călinoiu, M. S. Pascuta, R. A. Varvara, K. Szabo, P. Vajda, C. Szekely and G. A. Martău, Development of pectin and poly (vinyl alcohol)-based active packaging enriched with itaconic acid and apple pomace-derived antioxidants, Antioxidants, 2022, 11(9), 1729 CrossRef CAS PubMed.
- W. Khalid, M. S. Arshad, A. Jabeen, F. Muhammad Anjum, T. B. Qaisrani and H. A. Suleria, Fiber-enriched botanicals: A therapeutic tool against certain metabolic ailments, Food Sci. Nutr., 2022, 10(10), 3203–3218 CrossRef CAS PubMed.
- M. Chanmontri, A. E. Swilem, A. L. Mutch, L. Grøndahl and O. Suwantong, Physicochemical and in vitro biological evaluation of an injectable self-healing quaternized chitosan/oxidized pectin hydrogel for potential use as a wound dressing material, Int. J. Biol. Macromol., 2023, 242, 124984 CrossRef CAS PubMed.
- K. Song, Y. Hao, Y. Liu, R. Cao, X. Zhang, S. He, J. Wen, W. Zheng, L. Wang and Y. Zhang, Preparation of pectin-chitosan hydrogels based on bioadhesive-design micelle to prompt bacterial infection wound healing, Carbohydr. Polym., 2023, 300, 120272 CrossRef CAS PubMed.
- R. A. Saucedo-Acuña, K. Z. Meza-Valle, J. C. Cuevas-González, E. G. Ordoñez-Casanova, M. I. Castellanos-García, E. A. Zaragoza-Contreras and G. F. Tamayo-Pérez, Characterization and in vivo assay of allantoin-enriched pectin hydrogel for the treatment of skin wounds, Int. J. Mol. Sci., 2023, 24(8), 7377 CrossRef PubMed.
- O. Phonrachom, P. Charoensuk, K. Kiti, N. Saichana, P. Kakumyan and O. Suwantong, Potential use of propolis-loaded quaternized chitosan/pectin hydrogel films as wound dressings: Preparation, characterization, antibacterial evaluation, and in vitro healing assay, Int. J. Biol. Macromol., 2023, 241, 124633 CrossRef CAS PubMed.
- M. A. Alsakhawy, D. A. Abdelmonsif, M. Haroun and S. A. Sabra, Naringin-loaded Arabic gum/pectin hydrogel as a potential wound healing material, Int. J. Biol. Macromol., 2022, 222, 701–714 CrossRef CAS PubMed.
- N. I. Elsherif, A. M. Al-Mahallawi, I. S. Ahmed and R. N. Shamma, Pectin nanoparticles loaded with nitric oxide donor drug: A potential approach for tissue regeneration, Int. J. Pharm.: X, 2024, 7, 100244 CAS.
- D. U. Kapoor, R. Garg, M. Gaur, A. Pareek, B. G. Prajapati, G. R. Castro, S. Suttiruengwong and P. Sriamornsak, Pectin hydrogels for controlled drug release: Recent developments and future prospects, Saudi Pharm. J., 2024, 102002 CrossRef CAS PubMed.
- S. Madhusudan and R. Baskaran, The sea lettuce Ulva sensu lato: Future food with health-promoting bioactives, Algal Res., 2023, 71, 103069 CrossRef.
- B. S. Negreanu-Pirjol, T. Negreanu-Pirjol, D. R. Popoviciu, R. E. Anton and A. M. Prelipcean, Marine bioactive compounds derived from macroalgae as new potential players in drug delivery systems: a review, Pharmaceutics, 2022, 14(9), 1781 CrossRef CAS PubMed.
- S. Ghosh, T. Sarkar, S. Pati, Z. A. Kari, H. A. Edinur and R. Chakraborty, Novel bioactive compounds from marine sources as a tool for functional food development, Front. Mar. Sci., 2022, 9, 832957 CrossRef.
- N. L. Dashputre, R. R. Sable, M. Sawant, S. J. Khairnar, E. D. Ahire, S. B. Patil and J. D. Kadam, Marine-Derived Sources of Nutritional Vitamins, Vitamins as Nutraceuticals: Recent Advances and Applications, 2023, pp. 129–166 Search PubMed.
- P. Otero, M. Carpena, P. Garcia-Oliveira, J. Echave, A. Soria-Lopez, P. García-Pérez, M. Fraga-Corral, H. Cao, S. Nie, J. Xiao and J. Simal-Gandara, Seaweed polysaccharides: Emerging extraction technologies, chemical modifications and bioactive properties, Crit. Rev. Food Sci. Nutr., 2023, 63(13), 1901–1929 CrossRef CAS PubMed.
- S. G. Jin, Production and application of biomaterials based on polyvinyl alcohol (PVA) as wound dressing, Chem.–Asian J., 2022, 17(21), e202200595 CrossRef CAS PubMed.
- S. Basak and J. Gokhale, Immunity boosting nutraceuticals: Current trends and challenges, J. Food Biochem., 2022, 46(3), e13902 CrossRef CAS PubMed.
- S. Kikionis, M. Koromvoki, A. Tagka, E. Polichronaki, A. Stratigos, A. Panagiotopoulos, A. Kyritsi, V. Karalis, A. Vitsos, M. Rallis and E. Ioannou, Ulvan-based nanofibrous patches enhance wound healing of skin trauma resulting from cryosurgical treatment of keloids, Mar. Drugs, 2022, 20(9), 551 CrossRef CAS PubMed.
- Y. Ren, A. Aierken, L. Zhao, Z. Lin, J. Jiang, B. Li, J. Wang, J. Hua and Q. Tu, hUC-MSCs lyophilized powder loaded polysaccharide ulvan driven functional hydrogel for chronic diabetic wound healing, Carbohydr. Polym., 2022, 288, 119404 CrossRef CAS PubMed.
- T. M. Don, C. H. Ma and Y. C. Huang, In situ release of ulvan from crosslinked ulvan/chitosan complex films and their evaluation as wound dressings, Polymers, 2022, 14(24), 5382 CrossRef CAS PubMed.
- E. Sulastri, R. Lesmana, M. S. Zubair, A. F. Mohammed, K. M. Elamin and N. Wathoni, Ulvan/Silver nanoparticle hydrogel films for burn wound dressing, Heliyon, 2023, 9(7), e18044 CrossRef CAS PubMed.
- K. Mariia, M. Arif, J. Shi, F. Song, Z. Chi and C. Liu, Novel chitosan-ulvan hydrogel reinforcement by cellulose nanocrystals with epidermal growth factor for enhanced wound healing: In vitro and in vivo analysis, Int. J. Biol. Macromol., 2021, 183, 435–446 CrossRef CAS PubMed.
- J. Foroughi, A. Ruhparwar, S. Aloko and C. H. Wang, Manufacturing ulvan biopolymer for wound dressings, Macromol. Mater. Eng., 2024, 309(2), 2300268 CrossRef CAS.
- D. Statha, A. Papaioannou, S. Kikionis, M. Kostaki, I. Sfiniadakis, A. Vitsos, J. Anastassopoulou, E. Ioannou, V. Roussis and M. C. Rallis, Healing Potential of the Marine Polysaccharides Carrageenan and Ulvan on Second-Degree Burns, J. Funct. Biomater., 2024, 15(9), 257 CrossRef CAS PubMed.
- P. Pooprommin, C. Manaspon, A. Dwivedi, A. Mazumder, S. Sangkaew, S. Wanmasae, J. Tangpong, T. Ongtanasup and K. Eawsakul, Alginate/pectin dressing with niosomal mangosteen extract for enhanced wound healing: evaluating skin irritation by structure-activity relationship, Heliyon, 2022, 8(12), e12032 CrossRef CAS PubMed.
- A. Wendimu, W. Tekalign and B. Asfaw, A survey of traditional medicinal plants used to treat common human and livestock ailments from Diguna Fango district, Wolaita, southern Ethiopia, Nord. J. Bot., 2021, 39(5), 1–20 Search PubMed.
- M. Kumar, S. Changan, M. Tomar, U. Prajapati, V. Saurabh, M. Hasan, M. Sasi, C. Maheshwari, S. Singh and S. Dhumal, Radha. Custard apple (Annona squamosa L.) leaves: Nutritional composition, phytochemical profile, and health-promoting biological activities, Biomolecules, 2021, 11(5), 614 CrossRef CAS PubMed.
- W. Sun and M. H. Shahrajabian, Therapeutic potential of phenolic compounds in medicinal plants—Natural health products for human health, Molecules, 2023, 28(4), 1845 CrossRef CAS PubMed.
- C. Porro and M. A. Panaro, Recent progress in understanding the health benefits of curcumin, Molecules, 2023, 28(5), 2418 CrossRef CAS PubMed.
- D. Canistro, A. Chiavaroli, D. Cicia, F. Cimino, D. Curro, M. Dell'Agli, C. Ferrante, L. Giovannelli, S. Leone, G. Martinelli and L. Milella, The pharmacological basis of the curcumin nutraceutical uses: An update, Pharmadvances, 2021, 3(2), 421–466 Search PubMed.
- K. Duszka, Versatile triad alliance: bile acid, taurine and microbiota, Cells, 2022, 11(15), 2337 CrossRef CAS PubMed.
- J. Zhang, K. Chen, C. Ding, S. Sun, Y. Zheng, Q. Ding, B. Hong and W. Liu, Fabrication of chitosan/PVP/dihydroquercetin nanocomposite film for in vitro and in vivo evaluation of wound healing, Int. J. Biol. Macromol., 2022, 206, 591–604 CrossRef CAS PubMed.
- Y. Yang, X. Zhao, J. Yu, X. Chen, R. Wang, M. Zhang, Q. Zhang, Y. Zhang, S. Wang and Y. Cheng, Bioactive skin-mimicking hydrogel band-aids for diabetic wound healing and infectious skin incision treatment, Bioact. Mater., 2021, 6(11), 3962 CAS.
- M. E. Abd El-Hack, M. T. El-Saadony, A. A. Swelum, M. Arif, M. M. Abo Ghanima, M. Shukry, A. Noreldin, A. E. Taha and K. A. El-Tarabily, Curcumin, the active substance of turmeric: its effects on health and ways to improve its bioavailability, J. Sci. Food Agric., 2021, 101(14), 5747–5762 CrossRef CAS PubMed.
- R. Chang, L. Chen, M. Qamar, Y. Wen, L. Li, J. Zhang, X. Li, E. Assadpour, T. Esatbeyoglu, M. S. Kharazmi and Y. Li, The bioavailability, metabolism and microbial modulation of curcumin-loaded nanodelivery systems, Adv. Colloid Interface Sci., 2023, 318, 102933 CrossRef CAS PubMed.
- J. Sharifi-Rad, C. Quispe, M. Butnariu, L. S. Rotariu, O. Sytar, S. Sestito, S. Rapposelli, M. Akram, M. Iqbal, A. Krishna and N. V. Kumar, Chitosan nanoparticles as a promising tool in nanomedicine with particular emphasis on oncological treatment, Cancer Cell Int., 2021, 21(1), 318 CrossRef CAS PubMed.
- Y. L. Ti, F. Song, Z. Fang and P. Zhang, Plants and phytochemicals inhibit scar formation: A systematic review, Ind. Crops Prod., 2022, 185, 115113 CrossRef CAS.
- S. A. Shah, M. Sohail, S. A. Khan and M. Kousar, Improved drug delivery and accelerated diabetic wound healing by chondroitin sulfate grafted alginate-based thermoreversible hydrogels, Mater. Sci. Eng., C, 2021, 126, 112169 CrossRef CAS PubMed.
- D. Ramadon, M. T. McCrudden, A. J. Courtenay and R. F. Donnelly, Enhancement strategies for transdermal drug delivery systems: Current trends and applications, Drug Delivery Transl. Res., 2022, 1, 1–34 Search PubMed.
- S. Vitale, S. Colanero, M. Placidi, G. Di Emidio, C. Tatone, F. Amicarelli and A. M. D'Alessandro, Phytochemistry and biological activity of medicinal plants in wound healing: an overview of current research, Molecules, 2022, 27(11), 3566 CrossRef CAS PubMed.
- A. Amini, H. Soleimani, F. Rezaei, S. K. Ghoreishi, S. Chien and M. Bayat, The combined effect of photobiomodulation and curcumin on acute skin wound healing in rats, Int. J. Lower Extremity Wounds, 2021, 12, e9 Search PubMed.
- B. Gorain, M. Pandey, N. H. Leng, C. W. Yan, K. W. Nie, S. J. Kaur, V. Marshall, S. P. Sisinthy, J. Panneerselvam, N. Molugulu and P. Kesharwani, Advanced drug delivery systems containing herbal components for wound healing, Int. J. Pharm., 2022, 617, 121617 CrossRef CAS PubMed.
- M. Rudrapal, W. A. Eltayeb, G. Rakshit, A. A. El-Arabey, J. Khan, S. M. Aldosari, B. Alshehri and M. Abdalla, Dual synergistic inhibition of COX and LOX by potential chemicals from Indian daily spices investigated through detailed computational studies, Sci. Rep., 2023, 13(1), 8656 CrossRef CAS PubMed.
- A. R. Afshari, M. Sanati, H. Mollazadeh, P. Kesharwani, T. P. Johnston and A. Sahebkar, Nanoparticle-based drug delivery systems in cancer: A focus on inflammatory pathways, in Seminars in Cancer Biology, Academic Press, 2022, vol. 86, pp. 860–872 Search PubMed.
- X. Yang, Y. Yang, K. Liu and C. Zhang, Traditional Chinese medicine monomers: targeting pulmonary artery smooth muscle cells proliferation to treat pulmonary hypertension, Heliyon, 2023, 9(4), e14916 CrossRef CAS PubMed.
- S. V. Raghu, S. Rao, V. Kini, A. K. Kudva, T. George and M. S. Baliga, Fruits and their phytochemicals in mitigating the ill effects of ionizing radiation: review on the existing scientific evidence and way forward, Food Funct., 2023, 14(3), 1290–1319 RSC.
- L. Ansari, H. Mashayekhi-Sardoo, V. Baradaran Rahimi, R. Yahyazadeh, M. Ghayour-Mobarhan and V. R. Askari, Curcumin-based nanoformulations alleviate wounds and related disorders: A comprehensive review, Biofactors, 2023, 49(4), 736–781 CrossRef CAS PubMed.
- H. M. Abu-Hijleh, R. M. Al-Zoubi, A. Zarour, A. Al-Ansari and H. Bawadi, The Therapeutic Role of Curcumin in Inflammation and Post-Surgical Outcomes, Food Rev. Int., 2024, 40(1), 293–308 CrossRef CAS.
- P. Viaña-Mendieta, M. L. Sánchez and J. Benavides, Rational selection of bioactive principles for wound healing applications: Growth factors and antioxidants, Int. Wound J., 2022, 19(1), 100–113 CrossRef PubMed.
- P. K. Sasmal and S. Ganguly, Polymer in hemostasis and follow-up wound healing, J. Appl. Polym. Sci., 2023, 140(9), e53559 CrossRef CAS.
- S. Taghavifar, F. Afroughi and M. Saadati Keyvan, Curcumin nanoparticles improved diabetic wounds infected with methicillin-resistant Staphylococcus aureus sensitized with HAMLET, J. Laser Med. Sci., 2022, 21(2), 141–153 CAS.
- L. Ansari, H. Mashayekhi-Sardoo, V. Baradaran Rahimi, R. Yahyazadeh, M. Ghayour-Mobarhan and V. R. Askari, Curcumin-based nanoformulations alleviate wounds and related disorders: A comprehensive review, Biofactors, 2023, 49(4), 736–781 CrossRef CAS PubMed.
- Q. Hu and Y. Luo, Chitosan-based nanocarriers for encapsulation and delivery of curcumin: A review, Int. J. Biol. Macromol., 2021, 179, 125–135 CrossRef CAS PubMed.
- N. S. Chatterjee, H. G. Sukumaran, P. K. Dara, B. Ganesan, M. Ashraf, R. Anandan, S. Mathew and R. C. Nagarajarao, Nano-encapsulation of curcumin in fish collagen grafted succinyl chitosan hydrogel accelerates wound healing process in experimental rats, Food Hydrocolloids Health, 2022, 2, 100061 CrossRef.
- S. A. Shah, M. Sohail, M. Karperien, C. Johnbosco, A. Mahmood and M. Kousar, Chitosan and carboxymethyl cellulose-based 3D multifunctional bioactive hydrogels loaded with nano-curcumin for synergistic diabetic wound repair, Int. J. Biol. Macromol., 2023, 227, 1203–1220 CrossRef CAS PubMed.
- V. Muthulakshmi and G. R. Rajarajeswari, In vivo wound healing efficiency of curcumin-incorporated pectin-chitosan biodegradable films, New J. Chem., 2021, 45(38), 17918–17929 RSC.
- M. Subbuvel and P. Kavan, Preparation and characterization of polylactic acid/fenugreek essential oil/curcumin composite films for food packaging applications, Int. J. Biol. Macromol., 2022, 194, 470–483 CrossRef CAS PubMed.
- A. Al Fatease, M. A. Abourehab, A. M. Alqahtani, K. Chidambaram, A. A. Qureshi, K. Venkatesan, S. M. Alshahrani and H. Abdelkader, Polymeric/Dextran Wafer Dressings as Promising Long-Acting Delivery Systems for Curcumin Topical Delivery and Enhancing Wound Healing in Male Wistar Albino Rats, Pharmaceuticals, 2022, 16(1), 38 CrossRef PubMed.
- K. Postolović, B. Ljujić, M. M. Kovačević, S. Đorđević, S. Nikolić, S. Živanović and Z. Stanić, Optimization, characterization, and evaluation of carrageenan/alginate/poloxamer/curcumin hydrogel film as a functional wound dressing material, Mater. Today Commun., 2022, 31, 103528 CrossRef.
- K. S. Postolović, M. D. Antonijević, B. Ljujić, S. Radenković, M. Miletić Kovačević, Z. Hiezl, S. Pavlović, I. Radojević and Z. Stanić, Curcumin and diclofenac therapeutic efficacy enhancement applying transdermal hydrogel polymer films, based on carrageenan, alginate and poloxamer, Polymers, 2022, 14(19), 4091 CrossRef PubMed.
- M. Gupta, A. Sharma, C. S. Beniwal and P. Tyagi, Curcumin coated 3D biocomposite scaffolds based on chitosan and cellulose for diabetic wound healing, Heliyon, 2022, 8(11), e11442 CrossRef CAS PubMed.
- S. A. Shah, M. Sohail, M. U. Minhas, S. Khan, Z. Hussain, A. Mahmood, M. Kousar, H. E. Thu and M. Abbasi, Curcumin-laden hyaluronic acid-co-Pullulan-based biomaterials as a potential platform to synergistically enhance the diabetic wound repair, Int. J. Biol. Macromol., 2021, 185, 350–368 CrossRef CAS PubMed.
- M. Chaala, F. Z. Sebba, M. G. Fuster, I. Moulefera, M. G. Montalbán, G. Carissimi and G. Víllora, Accelerated simple preparation of curcumin-loaded silk fibroin/hyaluronic acid hydrogels for biomedical applications, Polymers, 2023, 15(3), 504 CrossRef CAS PubMed.
- D. Dutta, S. Pajaniradje, A. S. Nair, S. Chandramohan, S. A. Bhat, E. Manikandan and R. Rajagopalan, An in-vitro study of active targeting & anti-cancer effect of folic acid conjugated chitosan encapsulated indole curcumin analogue nanoparticles, Int. J. Biol. Macromol., 2024, 282, 136990 CrossRef CAS PubMed.
- C. Pornpitchanarong, K. C. Aye, K. Arunprasert, P. Opanasopit and P. Patrojanasophon, Computational designed and optimized liposomal curcumin-embedded bifunctional cross-linked hydrogels for Wound Healing, Gels, 2024, 10(9), 598 CrossRef CAS PubMed.
- W. S. Al-Arjan, M. U. Khan, H. H. Almutairi, S. M. Alharbi and S. I. Razak, pH-Responsive PVA/BC-f-GO dressing materials for burn and chronic wound healing with curcumin release kinetics, Polymers, 2022, 14(10), 1949 CrossRef CAS PubMed.
- R. Velmurugan, G. Hegde, K. Soontarapa and R. S. Keri, Evaluation of wound healing effect of curcumin loaded OPL carbon nanospheres embedded chitosan membranes, J. Polym. Environ., 2022, 30(12), 5190–5201 CrossRef.
- H. Singh, S. D. Purohit, R. Bhaskar, I. Yadav, S. Bhushan, M. K. Gupta and N. C. Mishra, Curcumin in decellularized goat small intestine submucosa for wound healing and skin tissue engineering, J. Biomed. Mater. Res., Part B, 2022, 110(1), 210–219 CrossRef CAS PubMed.
- Y. Y. Wu, R. Kumar, C. C. Wong, D. N. Reddy and F. Y. Huang, Synthesis and characterization of curcumin incorporated multi component nano-scaffold with enhanced anti-bacterial and wound healing properties, Curr. Drug Delivery, 2023, 20(4), 400–413 CrossRef CAS PubMed.
- E. R. Kenawy, E. A. Kamoun, Z. S. Ghaly, A. B. Shokr, M. A. El-Meligy and Y. A. Mahmoud, Novel physically cross-linked curcumin-loaded PVA/aloe vera hydrogel membranes for acceleration of topical wound healing: In vitro and in vivo experiments, Arabian J. Sci. Eng., 2023, 48(1), 497–514 CrossRef CAS.
- J. Li, H. Li, K. Wang and H. Chou, Evaluation of decellularized caprine small intestine submucosa encapsulated with nano-formulations of cerium oxide and curcumin in the management of burn wound, Regener. Ther., 2024, 26, 578–589 CrossRef CAS PubMed.
- D. Miele, L. Catenacci, M. Sorrenti, S. Perteghella, S. Filiberti, D. Mandracchia, R. Ronca and M. C. Bonferoni, Collagen/PCL electrospun fibers loaded with polyphenols: Curcumin and resveratrol comparison, Int. J. Biol. Macromol., 2024, 279, 135333 CrossRef CAS PubMed.
- K. Wadhwa, V. Kadian, V. Puri, B. Y. Bhardwaj, A. Sharma, R. Pahwa, R. Rao, M. Gupta and I. Singh, New insights into quercetin nanoformulations for topical delivery, Phytomed. Plus, 2022, 2(2), 100257 CrossRef.
- H. G. Ulusoy and N. Sanlier, A minireview of quercetin: from its metabolism to possible mechanisms of its biological activities, Crit. Rev. Food Sci. Nutr., 2020, 60(19), 3290–3303 CrossRef CAS PubMed.
- Q. Aljelehawy, O. R. Mal Allah and G. Sourazur, Physicochemical properties, medicinal chemistry, toxicity, and absorption of quercetin and its interaction with spike glycoprotein of SARS-CoV-2: Molecular docking, Nano Micro Biosyst., 2022, 1(1), 32–39 Search PubMed.
- N. Zulkefli, C. N. Che Zahari, N. H. Sayuti, A. A. Kamarudin, N. Saad, H. S. Hamezah, H. Bunawan, S. N. Baharum, A. Mediani, Q. U. Ahmed and A. F. Ismail, Flavonoids as potential wound-healing molecules: Emphasis on pathways perspective, Int. J. Mol. Sci., 2023, 24(5), 4607 CrossRef CAS PubMed.
- B. T. Tung, T. V. Linh, T. P. Thao and N. D. Thuan, Anti-inflammatory Agents from Medicinal Plants, Phytochemical Drug Discovery for Central Nervous System Disorders: Biochemistry and Therapeutic Effects, 2023, vol. 14, pp. 219–250 Search PubMed.
- F. Di Pierro, A. Khan, S. Iqtadar, S. U. Mumtaz, M. N. Chaudhry, A. Bertuccioli, G. Derosa, P. Maffioli, S. Togni, A. Riva and P. Allegrini, Quercetin as a possible complementary agent for early-stage COVID-19: Concluding results of a randomized clinical trial, Front. Pharmacol., 2023, 13, 1096853 CrossRef PubMed.
- V. K. Panthi, M. Imran, A. Chaudhary, K. R. Paudel and Y. Mohammed, The significance of quercetin-loaded advanced nanoformulations for the management of diabetic wounds, Nanomedicine, 2023, 18(4), 391–411 CrossRef CAS PubMed.
- R. Apak, A. Calokerinos, S. Gorinstein, M. A. Segundo, D. B. Hibbert, İ. Gülçin, S. Demirci Çekiç, K. Güçlü, M. Özyürek, S. E. Çelik and L. M. Magalhães, Methods to evaluate the scavenging activity of antioxidants toward reactive oxygen and nitrogen species (IUPAC Technical Report), Pure Appl. Chem., 2022, 94(1), 87–144 CrossRef CAS.
- W. Qin, Y. Wu, J. Liu, X. Yuan and J. Gao, A comprehensive review of the application of nanoparticles in diabetic wound healing: therapeutic potential and future perspectives, Int. J. Nanomed., 2022, 17, 6007 CrossRef PubMed.
- S. B. Mishra, S. Singh, A. K. Singh, A. K. Singh and D. R. Sharma, Emulgels: a novel approach for enhanced topical drug delivery systems, Advances in novel formulations for drug delivery, 2023, pp. 231–262 Search PubMed.
- D. Paramanick, V. D. Singh and V. K. Singh, Neuroprotective effect of phytoconstituents via nanotechnology for treatment of Alzheimer diseases, J. Controlled Release, 2022, 351, 638–655 CrossRef CAS PubMed.
- Y. Wang, X. Wei, L. Wang, Z. Qian, H. Liu and Y. Fan, Quercetin-based composite hydrogel promotes muscle tissue regeneration through macrophage polarization and oxidative stress attenuation, Composites, Part B, 2022, 247, 110311 CrossRef CAS.
- K. S. El-Said, A. Atta, M. A. Mobasher, M. O. Germoush, T. M. Mohamed and M. M. Salem, Quercetin mitigates rheumatoid arthritis by inhibiting adenosine deaminase in rats, Mol. Med., 2022, 28(1), 24 CAS.
- T. C. De Oliveira, M. E. Tavares, J. L. Soares-Sobrinho and L. L. Chaves, The role of nanocarriers for transdermal application targeted to lymphatic drug delivery: Opportunities and challenges, J. Drug Delivery Sci. Technol., 2022, 68, 103110 CrossRef CAS.
- H. Peng and F. Shahidi, Quercetin fatty acid monoesters (C2: 0–C18: 0): Enzymatic preparation and antioxidant activity, J. Agric. Food Chem., 2022, 70(43), 14073–14083 CrossRef CAS PubMed.
- S. Cherian, K. M. Hacisayidli, R. Kurian and A. Mathews, Therapeutically important bioactive compounds of the genus Polygonum L. and their possible interventions in clinical medicine, J. Pharm. Pharmacol., 2023, 75(3), 301–327 CrossRef PubMed.
- V. K. Panthi, M. Imran, A. Chaudhary, K. R. Paudel and Y. Mohammed, The significance of quercetin-loaded advanced nanoformulations for the management of diabetic wounds, Nanomedicine, 2023, 18(4), 391–411 CrossRef CAS PubMed.
- M. Prasathkumar and S. Sadhasivam, Chitosan/Hyaluronic acid/Alginate and an assorted polymers loaded with honey, plant, and marine compounds for progressive wound healing—Know-how, Int. J. Biol. Macromol., 2021, 186, 656–685 CrossRef CAS PubMed.
- S. Mazumdar, A. K. Ghosh, M. Dinda, A. K. Das, S. Das, K. Jana and P. Karmakar, Evaluation of wound healing activity of ethanol extract of Annona reticulata L. leaf both in vitro and in diabetic mice model, J. Tradit. Complementary Med., 2021, 11(1), 27–37 CrossRef CAS PubMed.
- N. Sutthammikorn, V. Supajatura, H. Yue, M. Takahashi, S. Chansakaow, N. Nakano, P. Song, T. Ogawa, S. Ikeda, K. Okumura and H. Ogawa, Topical Gynura procumbens as a novel therapeutic improves wound healing in diabetic mice, Plants, 2021, 10(6), 1122 CrossRef CAS PubMed.
- J. Y. Park, A. King, V. Björk, B. W. English, A. Fedintsev and C. Y. Ewald, Strategic outline of interventions targeting extracellular matrix for promoting healthy longevity, Am. J. Physiol. Cell Physiol., 2023, 325(1), C90–C128 CrossRef CAS PubMed.
- G. Rivera-Hernández, M. Antunes-Ricardo, P. Martínez-Morales and M. L. Sanchez, Polyvinyl alcohol based-drug delivery systems for cancer treatment, Int. J. Pharm., 2021, 600, 120478 CrossRef PubMed.
- N. Osman, N. Devnarain, C. A. Omolo, V. Fasiku, Y. Jaglal and T. Govender, Surface modification of nano-drug delivery systems for enhancing antibiotic delivery and activity, Wiley Interdiscip. Rev. Nanomed. Nanobiotechnol., 2022, 14(1), e1758 CrossRef CAS PubMed.
- M. L. Regenold, A Thermosensitive Liposome Formulation of Vinorelbine for the Treatment of Recurrent Rhabdomyosarcoma, Doctoral dissertation, University of Toronto Canada, 2023 Search PubMed.
- P. Asgharian, A. P. Tazekand, K. Hosseini, H. Forouhandeh, T. Ghasemnejad, M. Ranjbar, M. Hasan, M. Kumar, S. M. Beirami, V. Tarhriz and S. R. Soofiyani, Potential mechanisms of quercetin in cancer prevention: focus on cellular and molecular targets, Cancer Cell Int., 2022, 22(1), 257 CrossRef CAS PubMed.
- I. Shabir, V. Kumar Pandey, R. Shams, A. H. Dar, K. K. Dash, S. A. Khan, I. Bashir, G. Jeevarathinam, A. V. Rusu, T. Esatbeyoglu and R. Pandiselvam, Promising bioactive properties of quercetin for potential food applications and health benefits: A review, Front. Nutr., 2022, 9, 999752 CrossRef PubMed.
- P. Hagde, P. Pingle, A. Mourya, C. B. Katta, S. Srivastava, R. Sharma, K. K. Singh, R. K. Sodhi and J. Madan, Therapeutic potential of quercetin in diabetic foot ulcer: Mechanistic insight, challenges, nanotechnology driven strategies and future prospects, J. Drug Delivery Sci. Technol., 2022, 74, 103575 CrossRef CAS.
- L. Esposito, A. I. Barbosa, T. Moniz, S. Costa Lima, P. Costa, C. Celia and S. Reis, Design and characterization of sodium alginate and poly (vinyl) alcohol hydrogels for enhanced skin delivery of quercetin, Pharmaceutics, 2020, 12(12), 1149 CrossRef CAS PubMed.
- G. Gallelli, E. Cione, R. Serra, A. Leo, R. Citraro, P. Matricardi, C. Di Meo, F. Bisceglia, M. C. Caroleo, S. Basile and L. Gallelli, Nano-hydrogel embedded with quercetin and oleic acid as a new formulation in the treatment of diabetic foot ulcer: A pilot study, Int. Wound J., 2020, 17(2), 485–490 CrossRef PubMed.
- R. Badhwar, B. Mangla, Y. R. Neupane, K. Khanna and H. Popli, Quercetin loaded silver nanoparticles in hydrogel matrices for diabetic wound healing, Nanotechnology, 2021, 32(50), 505102 CrossRef CAS PubMed.
- T. Nalini, S. K. Basha, A. M. Sadiq and V. S. Kumari, Pectin/chitosan nanoparticle beads as potential carriers for quercetin release, Mater. Today Commun., 2022, 33, 104172 CrossRef CAS.
- N. H. Baktehir, M. A. Arbi, T. Selvaras and N. I. Ismail, Blend of multi-walled carbon nanotubes and quercetin improves physicochemical properties of chitosan membrane for wound dressing application, Malays. J. Fundam. Appl. Sci., 2023, 19(2), 202–214 CrossRef.
- T. Jin, L. Yan, W. Liu, S. Liu, C. Liu and L. Zheng, Preparation and physicochemical/antimicrobial characteristics of asparagus cellulose films containing quercetin, Food Sci. Hum. Wellness, 2021, 10(2), 251–257 CrossRef CAS.
- S. He, J. Liu, L. Hu, Y. Zhan, H. Tong, H. Zhu, H. Guo, H. Sun and M. Liu, Design, Synthesis, Biological Evaluation and Molecular Docking Studies of Quercetin-Linker-H2S Donor Conjugates for the Treatment of Diabetes and Wound Healing, Chem. Biodiversity, 2023, 20(7), e202300513 CrossRef CAS PubMed.
- M. Tavassoli, M. A. Sani, A. Khezerlou, A. Ehsani and D. J. McClements, Multifunctional nanocomposite active packaging materials: Immobilization of quercetin, lactoferrin, and chitosan nanofiber particles in gelatin films, Food Hydrocolloids, 2021, 118, 106747 CrossRef CAS.
- N. M. Munot, Y. D. Shinde, P. Shah, A. Patil, S. B. Patil and S. D. Bhinge, Formulation and evaluation of chitosan-PLGA biocomposite scaffolds incorporated with quercetin liposomes made by QbD approach for improved healing of oral lesions, AAPS PharmSciTech, 2023, 24(6), 147 CrossRef CAS PubMed.
- G. A. Govindasamy, R. B. SMN Mydin, N. K. Gadaime and S. Sreekantan, Phytochemicals, biodegradation, cytocompatibility and wound healing profiles of chitosan film embedded green synthesized antibacterial ZnO/CuO nanocomposite, J. Polym. Environ., 2023, 31(10), 4393–4409 CrossRef CAS.
- A. M. Croitoru, Y. Karaçelebi, E. Saatcioglu, E. Altan, S. Ulag, H. K. Aydoğan, A. Sahin, L. Motelica, O. Oprea, B. M. Tihauan and R. C. Popescu, Electrically triggered drug delivery from novel electrospun poly (lactic acid)/graphene oxide/quercetin fibrous scaffolds for wound dressing applications, Pharmaceutics, 2021, 13(7), 957 CrossRef CAS PubMed.
- E. A. Mazyed, G. Magdy, E. Elekhnawy, M. Yammine, C. Rolando and M. H. ElNaggar, Formulation and characterization of quercetin-loaded Prunus armeniaca gum nanoparticles with enhanced anti-bacterial effect, J. Drug Delivery Sci. Technol., 2024, 94, 105485 CrossRef CAS.
- S. A. El-Kholy, S. S. Osman, R. Abdel-Sattar and I. E. El Sayed, Synthesis of quercetin-loaded carboxymethyl cellulose nanogel: morphological structure and in vitro release, Biomass Convers. Biorefin., 2025, 15(5), 7495–7507 CrossRef CAS.
- T. Nalini, S. Khaleel Basha, A. Mohamed Sadiq and V. Sugantha Kumari, Fabrication and evaluation of nanoencapsulated quercetin for wound
healing application, Polym. Bull., 2023, 80(1), 515–540 CrossRef CAS.
- S. Chaturvedi, S. Agrawal, A. Garg and V. Rastogi, Potential of nanoencapsulated quercetin topical formulations in the management of diabetic foot ulcer, Rev. Bras. Farmacogn., 2023, 33(3), 484–501 CrossRef CAS.
- S. Saleh, A. E. Omar, H. S. Zayed and E. Tolba, Quercetin/selenium functional nanoparticle for enhancing of antimicrobial activity and anti-inflammatory potential of chitosan/polyvinyl alcohol cryogel, J. Inorg. Organomet. Polym. Mater., 2023, 33(4), 1037–1051 CrossRef CAS.
- J. Li, Y. Li, C. Guo and X. Wu, Development of quercetin loaded silk fibroin/soybean protein isolate hydrogels for burn wound healing, Chem. Eng. J., 2024, 481, 148458 CrossRef CAS.
- A. S. Kumar, D. Prema, R. G. Rao, J. Prakash, P. Balashanmugam, T. Devasena and G. D. Venkatasubbu, Fabrication of poly (lactic-co-glycolic acid)/gelatin electro spun nanofiber patch containing CaCO3/SiO2 nanocomposite and quercetin for accelerated diabetic wound healing, Int. J. Biol. Macromol., 2024, 254, 128060 CrossRef CAS PubMed.
- K. Adlakha, B. Koul and A. Kumar, Value-added products of Aloe species: Panacea to several maladies, S. Afr. J. Bot., 2022, 147(1), 1124–1135 CrossRef.
- C. Altinkaynak, E. Haciosmanoglu, M. Ekremoglu, M. Hacioglu and N. Özdemir, Anti-microbial, anti-oxidant and wound healing capabilities of Aloe vera-incorporated hybrid nanoflowers, J. Biosci. Bioeng., 2023, 135(4), 321–330 CrossRef CAS PubMed.
- D. Adom, P. A. Sekyere and M. K. Krishnappa, The chemical constituents, anti-inflammatory, anti-oxidant, and ethnomedicinal properties of aloe barbadensis, in Research Anthology on Recent Advancements in Ethnopharmacology and Nutraceuticals, IGI Global Scientific Publishing, 2022, pp. 454–471 Search PubMed.
- K. Adlakha, B. Koul and A. Kumar, Value-added products of Aloe species: Panacea to several maladies, S. Afr. J. Bot., 2022, 147, 1124–1135 CrossRef.
- O. Yazarlu, M. Iranshahi, H. R. Kashani, S. Reshadat, S. Habtemariam, M. Iranshahy and M. Hasanpour, Perspective on the application of medicinal plants and natural products in wound healing: A mechanistic review, Pharmacol. Res., 2021, 174, 105841 CrossRef CAS PubMed.
- S. Alven, V. Khwaza, O. O. Oyedeji and B. A. Aderibigbe, Polymer-based scaffolds loaded with aloe vera extract for the treatment of wounds, Pharmaceutics, 2021, 13(7), 961 CrossRef CAS PubMed.
- D. Hekmatpou, F. Mehrabi, K. Rahzani and A. Aminiyan, The effect of aloe vera clinical trials on prevention and healing of skin wound: a systematic review, Iran. J. Med. Sci., 2019, 44(1), 1 Search PubMed.
- J. Liang, L. Cui, J. Li, S. Guan, K. Zhang and J. Li, Aloe vera: a medicinal plant used in skin wound healing, Tissue Eng., Part B, 2021, 27(5), 455–474 CrossRef PubMed.
- A. Hernández-Rangel and E. S. Martin-Martinez, Collagen based electrospun materials for skin wounds treatment, J. Biomed. Mater. Res. Part A, 2021, 109(9), 1751–1764 CrossRef PubMed.
- Y. Pilehvar-Soltanahmadi, M. Dadashpour, A. Mohajeri, A. Fattahi, R. Sheervalilou and N. Zarghami, An overview on application of natural substances incorporated with electrospun nanofibrous scaffolds to development of innovative wound dressings, Mini-Rev. Med. Chem., 2018, 18(5), 414–427 CrossRef CAS PubMed.
- M. Sánchez, E. González-Burgos, I. Iglesias and M. P. Gómez-Serranillos, Pharmacological update properties of Aloe vera and its major active constituents, Molecules, 2020, 25(6), 1324 CrossRef PubMed.
- S. Pattnaik, S. Mohanty, S. K. Sahoo and C. Mohanty, A mechanistic perspective on the role of phytoconstituents-based pharmacotherapeutics and their topical formulations in chronic wound management, J. Drug Delivery Sci. Technol., 2023, 84, 104546 CrossRef CAS.
- A. Shedoeva, D. Leavesley, Z. Upton and C. Fan, Wound healing and the use of medicinal plants, Evid. base Compl. Alternative Med., 2019, 2019(1), 2684108 Search PubMed.
- O. Comas-Basté, S. Sánchez-Pérez, M. T. Veciana-Nogués, M. Latorre-Moratalla and M. D. Vidal-Carou, Histamine intolerance: The current state of the art, Biomolecules, 2020, 10(8), 1181 CrossRef PubMed.
- B. Alikiaii, M. Bagherniya, G. Askari, T. P. Johnston and A. Sahebkar, The role of phytochemicals in sepsis: A mechanistic and therapeutic perspective, BioFactors, 2021, 47(1), 19–40 CrossRef CAS PubMed.
- M. I. Mondal, J. Saha and M. A. Rahman, Functional applications of aloe vera on textiles: A review, J. Polym. Environ., 2021, 29, 993–1009 CrossRef CAS.
- C. Liu, Y. Cui, F. Pi, Y. Cheng, Y. Guo and H. Qian, Extraction, purification, structural characteristics, biological activities and pharmacological applications of acemannan, a polysaccharide from aloe vera: A review, Molecules, 2019, 24(8), 1554 CrossRef CAS PubMed.
- Z. Doozandeh, S. Saber-Samandari and A. Khandan, Preparation of novel Arabic gum-C6H9NO biopolymer as a bedsore for wound care application, Acta Med. Iran., 2020, 8, 520–530 Search PubMed.
- N. Aghamohamadi, N. S. Sanjani, R. F. Majidi and S. A. Nasrollahi, Preparation and characterization of Aloe vera acetate and electrospinning fibers as promising antibacterial properties materials, Mater. Sci. Eng., C, 2019, 94, 445–452 CrossRef CAS PubMed.
- D. Thomas, M. S. Nath, N. Mathew, E. Philip and M. S. Latha, Alginate film modified with aloevera gel and cellulose nanocrystals for wound dressing application: Preparation, characterization and in vitro evaluation, J. Drug Delivery Sci. Technol., 2020, 59, 101894 CrossRef CAS.
- K. Sharma, M. Munjal, R. K. Sharma and M. Sharma, Thymol encapsulated chitosan-Aloe vera films for antimicrobial infection, Int. J. Biol. Macromol., 2023, 235, 123897 CrossRef CAS PubMed.
- S. Baghersad, A. Hivechi, S. H. Bahrami, P. B. Milan, R. A. Siegel and M. Amoupour, Optimal Aloe vera encapsulated PCL/Gel nanofiber design for skin substitute application and the evaluation of its in vivo implantation, J. Drug Delivery Sci. Technol., 2022, 74, 103536 CrossRef CAS.
- J. Movaffagh, B. S. Bazzaz, Z. Taherzadeh, M. Hashemi, A. S. Moghaddam, S. abbas Tabatabaee, M. Azizzadeh and N. Jirofti, Evaluation of wound-healing efficiency of a functional Chitosan/Aloe vera hydrogel on the improvement of re-epithelialization in full thickness wound model of rat, J. Tissue Viability, 2022, 31(4), 649–656 CrossRef PubMed.
- M. Saberian, E. Seyedjafari, S. J. Zargar, F. S. Mahdavi and P. Sanaei-rad, Fabrication and characterization of alginate/chitosan hydrogel combined with honey and aloe vera for wound dressing applications, J. Appl. Polym. Sci., 2021, 138(47), 51398 CrossRef CAS.
- K. Saravanakumar, A. Sathiyaseelan, X. Zhang, M. Choi and M. H. Wang, Bimetallic (Ag and MgO) nanoparticles, Aloe vera extracts loaded xanthan gum nanocomposite for enhanced antibacterial and in-vitro wound healing activity, Int. J. Biol. Macromol., 2023, 242, 124813 CrossRef CAS PubMed.
- K. V. Naveen, K. Saravanakumar, A. Sathiyaseelan and M. H. Wang, Eco-friendly synthesis and characterization of Aloe vera/Gum Arabic/silver nanocomposites and their antibacterial, antibiofilm, and wound healing properties, Colloid Interface Sci. Commun., 2022, 46, 100566 CrossRef CAS.
- E. Sharifi, M. Chehelgerdi, A. Fatahian-Kelishadrokhi, F. Yazdani-Nafchi and K. Ashrafi-Dehkordi, Comparison of therapeutic effects of encapsulated Mesenchymal stem cells in Aloe vera gel and Chitosan-based gel in healing of grade-II burn injuries, Regener. Ther., 2021, 18, 30–37 CrossRef CAS PubMed.
- B. P. Genesi, R. de Melo Barbosa, P. Severino, A. C. Rodas, C. M. Yoshida, M. B. Mathor, P. S. Lopes, C. Viseras, E. B. Souto and C. F. da Silva, Aloe vera and copaiba oleoresin-loaded chitosan films for wound dressings: Microbial permeation, cytotoxicity, and in vivo proof of concept, Int. J. Pharm., 2023, 634, 122648 CrossRef CAS PubMed.
- D. Jessy Mercy, A. Thirumalai, S. Udayakumar, B. Deepika, G. Janani, A. Girigoswami and K. Girigoswami, Enhancing Wound Healing with Nanohydrogel-Entrapped Plant Extracts and Nanosilver: An In Vitro Investigation, Molecules, 2024, 29(21), 5004 CrossRef CAS PubMed.
- C. Wu, T. Zhang, B. Ji, Y. Chou and X. Du, Green synthesis of zinc oxide nanoparticles using Aloe vera leaf extract and evaluation of the antimicrobial and antioxidant properties of the ZnO/regenerated cellulose film, Cellulose, 2024, 31(8), 4849–4864 CrossRef CAS.
- T. Chakraborty, S. Gupta, A. Nair, S. Chauhan and V. Saini, Wound healing potential of insulin-loaded nanoemulsion with Aloe vera gel in diabetic rats, J. Drug Delivery Sci. Technol., 2021, 64, 102601 CrossRef CAS.
- A. Valizadeh, M. H. Darvishi, A. Amani and A. A. Zarchi, Design and development of novel formulation of Aloe vera nanoemulsion gel contained erythromycin for topical antibacterial therapy: In vitro and in vivo assessment, J. Drug Delivery Sci. Technol., 2022, 74, 103519 CrossRef CAS.
- Y. Wang, Y. Zhang, Z. Lin, T. Huang, W. Li, W. Gong, Y. Guo, J. Su, J. Wang and Q. Tu, A green method of preparing a natural and degradable wound dressing containing aloe vera as an active ingredient, Composites, Part B, 2021, 222, 109047 CrossRef CAS.
- N. Ijaz, A. I. Durrani, S. Rubab and S. Bahadur, Formulation and characterization of Aloe vera gel and tomato powder containing cream, Acta Ecol. Sin., 2022, 42(2), 34–42 CrossRef.
- M. Ghorbani, P. Nezhad-Mokhtari and S. Ramazani, Aloe vera-loaded nanofibrous scaffold based on Zein/Polycaprolactone/Collagen for wound healing, Int. J. Biol. Macromol., 2020, 153, 921–930 CrossRef CAS PubMed.
- L. O. Vajrabhaya, S. Korsuwannawong, N. Ruangsawasdi, C. Phruksaniyom and R. Srichan, The efficiency of natural wound healing and bacterial biofilm inhibition of Aloe vera and Sodium Chloride toothpaste preparation, BMC Complementary Med. Ther., 2022, 22(1), 66 CrossRef CAS PubMed.
- E. R. Kenawy, M. A. El-Meligy, Z. S. Ghaly, M. E. Kenawy and E. A. Kamoun, Novel Physically-Crosslinked Caffeine and Vitamin C-Loaded PVA/Aloe Vera Hydrogel Membranes for Topical Wound Healing: Synthesis, Characterization and In-Vivo Wound Healing Tests, J. Polym. Environ., 2024, 32(5), 2140–2157 CrossRef CAS.
- A. Aizaz, M. H. Nawaz, H. Shafique, M. H. Rehman, M. E. Khan, M. Abbas, T. Vayalpurayil and M. A. Rehman, Synthesis and characterization of aloe vera and hyaluronic acid-infused agar-agar/gelatin-based biopolymeric gel for potential skincare applications, J. Drug Delivery Sci. Technol., 2024, 100, 106017 CrossRef CAS.
- K. Mazumder, A. Aktar, P. Roy, B. Biswas, M. E. Hossain, K. K. Sarkar, S. C. Bachar, F. Ahmed, A. S. Monjur-Al-Hossain and K. Fukase, A review on mechanistic insight of plant derived anticancer bioactive phytocompounds and their structure activity relationship, Molecules, 2022, 3036 Search PubMed.
- S. Bhatnagar, R. Srivastava and R. Saxena, Mode of action of vinca alkaloids against cancer using Insilco analysis technique, Int. J. Sci. Res. Arch., 2022, 7(2), 181–188 CrossRef.
- A. Banyal, S. Tiwari, A. Sharma, I. Chanana, S. K. Patel, S. Kulshrestha and P. Kumar, Vinca alkaloids as a potential cancer therapeutics: recent update and future challenges, 3 Biotech., 2023, 13(6), 211 CrossRef PubMed.
- E. González-Burgos and M. P. Gómez-Serranillos, Vinca alkaloids as chemotherapeutic agents against breast cancer, Discovery and Development of Anti-breast Cancer Agents from Natural Products, 2021, pp. 69–101 Search PubMed.
- P. Dhyani, C. Quispe, E. Sharma, A. Bahukhandi, P. Sati, D. C. Attri, A. Szopa, J. Sharifi-Rad, A. O. Docea, I. Mardare and D. Calina, Anticancer potential of alkaloids: a key emphasis to colchicine, vinblastine, vincristine, vindesine, vinorelbine and vincamine, Cancer Cell Int., 2022, 22(1), 206 CrossRef CAS PubMed.
- U. R. Shwetha, M. S. Latha, C. R. Kumar, M. S. Kiran, H. S. Onkarappa and V. S. Betageri, Potential antidiabetic and anticancer activity of copper oxide nanoparticles synthesised using Areca catechu leaf extract, Adv. Nat. Sci.: Nanosci. Nanotechnol., 2021, 12(2), 025008 CAS.
- M. A. Albahde, B. Abdrakhimov, G. Q. Li, X. Zhou, D. Zhou, H. Xu, H. Qian and W. Wang, The role of microtubules in pancreatic cancer: therapeutic progress, Front. Oncol., 2021, 11, 640863 CrossRef CAS PubMed.
- G. E. Choi and H. J. Han, Glucocorticoid impairs mitochondrial quality control in neurons, Neurobiol. Dis., 2021, 152, 105301 CrossRef CAS PubMed.
- K. Meyanungsang, Documentation and Biological and Phytochemical Analysis of Chungtia Medicinal Plants of Nagaland, Doctoral dissertation, Macquarie University, India, 2022 Search PubMed.
- R. P. Lahare, H. S. Yadav, Y. K. Bisen and A. K. Dashahre, Estimation of total phenol, flavonoid, tannin and alkaloid content in different extracts of Catharanthus roseus from Durg district, Chhattisgarh, India, Sch. Bull., 2021, 7(1), 1–6 CrossRef.
- G. Kumar, R. Kumar, G. K. Gautam and H. Rana, The phytochemical and pharmacological properties of Catharanthus roseus (Vinca), Sci. Prog. Res., 2021, 2(1), 379–384 Search PubMed.
- W. Pan, F. Gu, X. Yan, J. Huang, H. Liao and F. Niu, Biomacromolecular carriers based hydrophobic natural products for potential cancer therapy, Int. J. Biol. Macromol., 2024, 269, 132274 CrossRef CAS PubMed.
- S. Goswami, A. Ali, M. E. Prasad and P. Singh, Pharmacological significance of Catharanthus roseus in cancer management: A review, Pharmacological Research-Modern Chinese Medicine, 2024, 11, 100444 CrossRef.
- A. Upaganlawar, S. Polshettiwar, S. Raut, A. Tagalpallewar and V. Pande, Effective cancer management: Inimitable role of phytochemical based nano-formulations, Curr. Drug Metab., 2022, 23(11), 869–881 CrossRef CAS PubMed.
- D. Ganapathy and S. KalaivaniNatarajan, Awareness About Vinca Alkaloids Among Dental Students, Int. J. Dent. Oral Sci., 2021, 8(04), 2337–2341 CrossRef.
- P. Dhyani, C. Quispe, E. Sharma, A. Bahukhandi, P. Sati, D. C. Attri, A. Szopa, J. Sharifi-Rad, A. O. Docea, I. Mardare and D. Calina, Anticancer potential of alkaloids: a key emphasis to colchicine, vinblastine, vincristine, vindesine, vinorelbine and vincamine, Cancer Cell Int., 2022, 22(1), 206 CrossRef CAS PubMed.
- W. Jiang, G. Cai, P. Hu and Y. Wang, Personalized medicine of non-gene-specific chemotherapies for non-small cell lung cancer, Acta Pharm. Sin. B, 2021, 11(11), 3406–3416 CrossRef CAS PubMed.
- A. Banyal, S. Tiwari, A. Sharma, I. Chanana, S. K. Patel, S. Kulshrestha and P. Kumar, Vinca alkaloids as a potential cancer therapeutics: recent update and future challenges, 3 Biotech., 2023, 13(6), 211 CrossRef PubMed.
- S. Srivastava, A. Verma and A. Giri, Therapeutic Applications and Diverse Uses of Vinca rosea in Treating Various Disease Conditions: A Comprehensive Review, J. Drug Discovery Health Sci., 2024, 1(01), 11–20 Search PubMed.
- L. Ebralidze, A. Tsertsvadze, E. Sanaia, D. Berashvili and A. Bakuridze, Formulation Of Biodegradable Polymeric Nanoparticles Containing Cytotoxic Substance Of Plant Origin, Georgian Medical News, 2020, 1(299), 137–142 Search PubMed.
- A. de Melo Fonseca, C. D. Araújo, J. H. da Silva, T. da Silva Honório, L. E. Nasciutti, L. M. Cabral, F. A. do Carmo and V. P. de Sousa, Development of transdermal based hydrogel formulations of vinorelbine with an evaluation of their in vitro profiles and activity against melanoma cells and in silico prediction of drug absorption, J. Drug Delivery Sci. Technol., 2021, 63, 102449 CrossRef.
- B. Amiri, H. Ahmadvand, A. Farhadi, A. Najmafshar, M. Chiani and D. Norouzian, Delivery of vinblastine-containing niosomes results in potent in vitro/in vivo cytotoxicity on tumor cells, Drug Dev. Ind. Pharm., 2018, 44(8), 1371–1376 CrossRef CAS PubMed.
- N. Nafee, A. E. Ameen and O. Y. Abdallah, Patient-friendly, olfactory-targeted, stimuli-responsive hydrogels for cerebral degenerative disorders ensured> 400% brain targeting efficiency in rats, AAPS PharmSciTech, 2020, 22(1), 6 CrossRef PubMed.
- A. Ciorîţă, M. Suciu, S. Macavei, I. Kacso, I. Lung, M. L. Soran and M. Parvu, Green synthesis of Ag-MnO2 nanoparticles using chelidonium majus and vinca minor extracts and their in vitro cytotoxicity, Molecules, 2020, 25(4), 819 CrossRef PubMed.
- R. Mohammadinejad, V. S. Madamsetty, A. Kumar, M. Varzandeh, A. Dehshahri, A. Zarrabi, F. Sharififar, M. Mohammadi, A. Fahimipour and S. Ramakrishna, Electrospun nanocarriers for delivering natural products for cancer therapy, Trends Food Sci. Technol., 2021, 118, 887–904 CrossRef CAS.
- R. Vrabec, J. Maříková, M. Ločárek, J. Korábečný, D. Hulcová, A. Hošťálková, J. Kuneš, J. Chlebek, T. Kučera, M. Hrabinová and D. Jun, Monoterpene indole alkaloids from Vinca minor L.(Apocynaceae): Identification of new structural scaffold for treatment of Alzheimer's disease, Phytochemistry, 2022, 194, 113017 CrossRef CAS PubMed.
- S. Mayer, A. Keglevich, C. Sepsey Für, H. Bölcskei, V. Ilkei, P. Keglevich and L. Hazai, Results in chemistry of natural organic compounds. synthesis of new anticancer vinca alkaloids and flavone alkaloids, Chemistry, 2020, 2(3), 714–726 CrossRef CAS.
- R. Arshad, M. S. Arshad, A. Rahdar, D. Hassan, R. Behzadmehr, S. Ghotekar, D. I. Medina and S. Pandey, Nanomaterials as an advanced nano-tool for the Doxorubicin delivery/Co-Delivery—A Comprehensive Review, J. Drug Delivery Sci. Technol., 2023, 83, 104432 CrossRef CAS.
- M. M. Ahmad, H. M. Kotb, S. Mushtaq, M. Waheed-Ur-Rehman, C. M. Maghanga and M. W. Alam, Green synthesis of Mn+ Cu bimetallic nanoparticles using Vinca rosea extract and their antioxidant, antibacterial, and catalytic activities, Crystals, 2022, 12(1), 72 CrossRef CAS.
- S. Chandrasekaran and V. Anbazhagan, Green synthesis of ZnO and V-doped ZnO nanoparticles using vinca rosea plant leaf for biomedical applications, Appl. Biochem. Biotechnol., 2024, 196(1), 50–67 CrossRef CAS PubMed.
- H. M. Aldawsari, S. M. Badr-Eldin, N. Y. Assiri, N. A. Alhakamy, A. Privitera, F. Caraci and G. Caruso, Surface-tailoring of emulsomes for boosting brain delivery of vinpocetine via intranasal route: in vitro optimization and in vivo pharmacokinetic assessment, Drug Delivery, 2022, 29(1), 2671–2684 CrossRef CAS PubMed.
- C. Barnett, F. Joubert, A. Iliopoulou, R. S. Álvarez and G. Pasparakis, Photochemical internalization using natural anticancer drugs, antimetabolites, and Nanoformulations: a systematic study against breast and pancreatic cancer cell lines, Mol. Pharm., 2023, 20(3), 1818–1841 CrossRef CAS PubMed.
- C. T. Bluette, A. M. Shoieb, Q. Peng, B. Manickam, W. Huang, E. Shin, W. Zhang, Y. H. Song and C. N. Liu, Behavioral, histopathologic, and molecular biological responses of nanoparticle-and solution-based formulations of vincristine in mice, Int. J. Toxicol., 2021, 40(1), 40–51 CrossRef CAS PubMed.
- S. Wang, J. Gou, Y. Wang, X. Tan, L. Zhao, X. Jin and X. Tang, Synergistic antitumor efficacy mediated by liposomal co-delivery of polymeric micelles of vinorelbine and cisplatin in non-small cell lung cancer, Int. J. Nanomed., 2021, 22, 2357–2372 CrossRef PubMed.
- M. M. Mohsen, K. Nagasree and A. B. Patil, The Development, Formulation, and Assessment of Polyelectrolytic Complexes of an Anticancer Compound (Vinorelbine Tartarate), Indian J. Pharm. Educ. Res., 2024, 58(3), 760–770 CrossRef.
- E. Arribas-López, N. Zand, O. Ojo, M. J. Snowden and T. Kochhar, A systematic review of the effect of Centella asiatica on wound healing, Int. J. Environ. Res. Public Health, 2022, 19(6), 3266 CrossRef PubMed.
- A. Kotnala, K. Verma, A. Sharma, S. Parashar, B. Rathi, R. Kumar, B. S. Chhikara and J. Singh, Indian Medicinal Plants for skin care and cosmeceuticals: A review, J. Biomed. Ther. Sci., 2019, 6(2), 24–60 Search PubMed.
- S. S. Mitra, A. Mukherjee, P. Nongdam, D. K. Pandey and A. Dey, Endophytes producing active constituents in Centella asiatica with a special emphasis on asiaticoside and madecassoside: a review update, Volatiles and Metabolites of Microbes, 2021, pp. 409–427 Search PubMed.
- S. Chandra, M. Gahlot, A. N. Choudhary, S. Palai, R. S. de Almeida, J. E. de Vasconcelos, F. A. dos Santos, P. A. de Farias and H. D. Coutinho, Scientific evidences of anticancer potential of medicinal plants, Food Chem. Adv., 2023, 2, 100239 CrossRef.
- S. Sen, R. Samat, M. Jash, S. Ghosh, R. Roy, N. Mukherjee, S. Ghosh, J. Sarkar and S. Ghosh, Potential broad-spectrum antimicrobial, wound healing, and disinfectant cationic peptide crafted from snake venom, J. Med. Chem., 2023, 66(16), 11555–11572 CrossRef CAS PubMed.
- M. Jamil, M. Mansoor, N. Latif, R. Naz, F. Anwar, M. Arshad, J. Gul, S. Ullah and M. Saddam, Review effect of aloe vera on wound healing: Review: Effect of aloe vera on wound healing, Pak. J. Sci. Ind. Res., Ser. B, 2020, 63(1), 48–61 CrossRef CAS.
- G. Pompilio, A. Nicolaides, S. K. Kakkos and D. Integlia, Systematic literature review and network Meta-analysis of sulodexide and other drugs in chronic venous disease, Phlebology, 2021, 36(9), 695–709 CrossRef PubMed.
- S. Bandopadhyay, S. Mandal, M. Ghorai, N. K. Jha, M. Kumar, G. A. Radha, J. Proćków, J. M. Perez de la Lastra and A. Dey, Therapeutic properties and pharmacological activities of asiaticoside and madecassoside: A review, J. Cell. Mol. Med., 2023, 27(5), 593–608 CrossRef CAS PubMed.
- Y. L. Ti, F. Song, Z. Fang and P. Zhang, Plants and phytochemicals inhibit scar formation: A systematic review, Ind. Crops Prod., 2022, 185, 115113 CrossRef CAS.
- S. Pattnaik, S. Mohanty, S. K. Sahoo and C. Mohanty, A mechanistic perspective on the role of phytoconstituents-based pharmacotherapeutics and their topical formulations in chronic wound management, J. Drug Delivery Sci. Technol., 2023, 84, 104546 CrossRef CAS.
- K. S. Park, Pharmacological effects of Centella asiatica on skin diseases: Evidence and possible mechanisms, Evid. base Compl. Alternative Med., 2021, 2021(1), 5462633 Search PubMed.
- N. N. Razali, C. T. Ng and L. Y. Fong, Cardiovascular protective effects of Centella asiatica and its triterpenes: a review, Planta Med., 2019, 85(16), 1203–1215 CrossRef CAS PubMed.
- P. Chaudhary, P. Janmeda, A. O. Docea, B. Yeskaliyeva, A. F. Abdull Razis, B. Modu, D. Calina and J. Sharifi-Rad, Oxidative stress, free radicals and antioxidants: Potential crosstalk in the pathophysiology of human diseases, Front. Chem., 2023, 11, 1158198 CrossRef CAS PubMed.
- K. Yeshi, D. Crayn, E. Ritmejerytė and P. Wangchuk, Plant secondary metabolites produced in response to abiotic stresses has potential application in pharmaceutical product development, Molecules, 2022, 27(1), 313 CrossRef CAS PubMed.
- L. R. Diniz, L. L. Calado, A. B. Duarte and D. P. de Sousa, Centella asiatica and its metabolite asiatic acid: wound healing effects and therapeutic potential, Metabolites, 2023, 13(2), 276 CrossRef CAS PubMed.
- B. Sun, L. Wu, Y. Wu, C. Zhang, L. Qin, M. Hayashi, M. Kudo, M. Gao and T. Liu, Therapeutic potential of Centella asiatica and its triterpenes: a review, Front. Pharmacol., 2020, 11, 568032 CrossRef CAS PubMed.
- S. Sen and S. Dutta, A comprehensive review on Thankuni (Centella asiatica) as an herbal remedy in diabetes mellitus and wound healing, J. Pharmacogn. Phytochem., 2020, 110085, 120129 Search PubMed.
- C. M. Sherwin and S. Heidari-Soureshjani, Effects and mechanisms of medicinal plants on healing scars: a systematic review, Curr. Tradit. Med., 2022, 8(1), 69–80 Search PubMed.
- M. L. Hossain, M. A. Rahman, A. Siddika, M. H. Adnan, H. Rahman, F. Diba, M. Z. Hasan and S. M. Asaduzzaman, Burn and wound healing using radiation sterilized human amniotic membrane and centella asiatica derived gel: a review, Regener. Eng. Transl. Med., 2020, 6, 347–357 CrossRef CAS.
- D. Biswas, S. Mandal, S. Chatterjee Saha, C. K. Tudu, S. Nandy, G. E. Batiha, M. S. Shekhawat, D. K. Pandey and A. Dey, Ethnobotany, phytochemistry, pharmacology, and toxicity of Centella asiatica (L.) Urban: A comprehensive review, Phytother. Res., 2021, 35(12), 6624–6654 CrossRef PubMed.
- L. P. Tirta and A. G. Yadnya-Putra, A narrative review of apiaceae family plants in usada netra for eye disease treatment, J. Pharmaceut. Sci. Appl., 2020, 2(2), 49–65 Search PubMed.
- C. Mouro, R. Fangueiro and I. C. Gouveia, Preparation and characterization of electrospun double-layered nanocomposites membranes as a carrier for Centella asiatica (L.), Polymers, 2020, 12(11), 2653 CrossRef CAS PubMed.
- S. Manotham, M. Khieokae and P. Butnoi, Electrospun biopolymer polyvinyl alcohol/Centella asiatica extract nanofibers for antibacterial activity, Mater. Today: Proc., 2023 Search PubMed.
- C. W. Kuo, Y. F. Chiu, M. H. Wu, M. H. Li, C. N. Wu, W. S. Chen and C. H. Huang, Gelatin/chitosan bilayer patches loaded with cortex Phellodendron amurense/Centella asiatica extracts for anti-acne application, Polymers, 2021, 13(4), 579 CrossRef CAS PubMed.
- R. Hemalatha, M. Alagar, P. Rameshbabu, A. A. Parvathi and R. H. Devamani, Development of medicinal plant (Centella Asiatica–Gotu Kola) based proton conducting polymer electrolytes for electrochemical device applications, Mater. Today: Proc., 2023, 81, 330–335 CAS.
- C. Ryall, S. Chen, S. Duarah and J. Wen, Chitosan-based microneedle arrays for dermal delivery of Centella asiatica, Int. J. Pharm., 2022, 627, 122221 CrossRef CAS PubMed.
- S. Aizad, S. I. Zubairi, B. H. Yahaya and A. M. Lazim, Centella asiatica extract potentiates anticancer activity in an improved 3-D PHBV-composite-CMC A549 lung cancer microenvironment scaffold, Arabian J. Sci. Eng., 2021, 46, 5313–5325 CrossRef CAS.
- S. Chen, Dermal Delivery of Centella Asiatica Using Hyaluronic Acid Niosomal System for Wound Healing,Doctoral dissertation, University of Auckland, 2022 Search PubMed.
- A. Gaspar-Pintiliescu, A. M. Stanciuc and O. Craciunescu, Natural composite dressings based on collagen, gelatin and plant bioactive compounds for wound healing: A review, Int. J. Biol. Macromol., 2019, 138, 854–865 CrossRef CAS PubMed.
- N. N. Nazmi and N. M. Sarbon, Characterization on antioxidant and physical properties of gelatin based composite films with incorporation of Centella asiatica (pegaga) extract, Food Res., 2020, 4(1), 224–233 Search PubMed.
- N. Namviriyachote, P. Muangman, K. Chinaroonchai, C. Chuntrasakul and G. C. Ritthidej, Polyurethane-biomacromolecule combined foam dressing containing asiaticoside: fabrication, characterization and clinical efficacy for traumatic dermal wound treatment, Int. J. Biol. Macromol., 2020, 143, 510–520 CrossRef CAS PubMed.
- T. Maver, M. Kurečič, T. Pivec, U. Maver, L. Gradišnik, P. Gašparič, B. Kaker, A. Bratuša, S. Hribernik and K. Stana Kleinschek, Needleless electrospun carboxymethyl cellulose/polyethylene oxide mats with medicinal plant extracts for advanced wound care applications, Cellulose, 2020, 27, 4487–4508 CrossRef CAS.
- N. Q. Thanh, D. H. Mai, T. P. Le, N. H. Do and P. K. Le, Novel chitosan/polyvinyl alcohol gel encapsulating ethanolic Centella asiatica extract for cosmeceutical applications, Polym. Bull., 2025, 82(2), 523–541 CrossRef CAS.
- B. Muchtaromah, A. M. Firdaus, A. N. Ansori, M. R. Duhita, E. B. Minarno, A. Hayati, M. Ahmad and I. Analisa, Effect of pegagan (Centella asiatica) nanoparticle coated with chitosan on the cytokine profile of chronic diabetic mice, Narra J, 2024, 4(1), e697 CrossRef CAS PubMed.
- Y. P. Sukmawan, I. Alifiar, L. Nurdianti and W. R. Ningsih, Wound healing effectivity of the ethanolic extracts of Ageratum conyzoides L. Leaf (white and purple flower type) and Centella asiatica and astaxanthin combination gel preparation in animal model, Turk. J. Pharm. Sci., 2021, 18(5), 609 CrossRef CAS PubMed.
- F. Camacho-Alonso, M. R. Torralba-Ruiz, N. García-Carrillo, J. Lacal-Luján, F. Martínez-Díaz and M. Sánchez-Siles, Effects of topical applications of porcine acellular urinary bladder matrix and Centella asiatica extract on oral wound healing in a rat model, Clin. Oral Invest., 2019, 23, 2083–2095 CrossRef PubMed.
- O. Bozkaya, E. Arat, Z. G. Gök, M. Yiğitoğlu and I. Vargel, Production and characterization of hybrid nanofiber wound dressing containing Centella asiatica coated silver nanoparticles by mutual electrospinning method, Eur. Polym. J., 2022, 166, 111023 CrossRef CAS.
- L. Wang, D. Li, Y. Shen, F. Liu, Y. Zhou, H. Wu, Q. Liu and B. Deng, Preparation of Centella asiatica loaded gelatin/chitosan/nonwoven fabric composite hydrogel wound dressing with antibacterial property, Int. J. Biol. Macromol., 2021, 192, 350–359 CrossRef CAS PubMed.
- Y. Q. Liu, D. Zhang, J. Deng, Y. Liu, W. Li and X. Nie, Preparation and safety evaluation of Centella asiatica total glycosides nitric oxide gel and its therapeutic effect on diabetic cutaneous ulcers, Evid. base Compl. Alternative Med., 2022, 2022(1), 1419146 Search PubMed.
- B. M. Tanga, S. Bang, X. Fang, C. Seo, M. De Zoysa, I. M. Saadeldin, S. Lee, S. U. Park, S. O. Chung, G. J. Lee and J. Cho, RETRACTED: Centella asiatica extract in carboxymethyl cellulose at its optimal concentration improved wound healing in mice models, Heliyon, 2022, 8(12) CrossRef CAS PubMed.
- L. Wang, Y. Yang, W. Han and H. Ding, Novel design and development of Centella Asiatica extract-loaded poloxamer/ZnO nanocomposite wound closure material to improve anti-bacterial action and enhanced wound healing efficacy in diabetic foot ulcer, Regener. Ther., 2024, 27, 92–103 CrossRef CAS PubMed.
- F. Abdollahimajd, M. R. Pourani, H. Mahdavi, H. Mirzadeh, S. Younespour and H. Moravvej, Efficacy and safety of chitosan-based bio-compatible dressing versus nanosilver (ActicoatTM) dressing in treatment of recalcitrant diabetic wounds: A randomized clinical trial, Dermatol. Ther., 2022, 35(9), e15682 CrossRef CAS PubMed.
- S. Radhakrishna, V. Shukla and S. K. Shetty, Is chitosan dental dressing better than cotton gauze in achieving hemostasis in patients on antithrombotics?, J. Oral Maxillofac. Surg., 2023, 81(2), 224–231 CrossRef PubMed.
- M. Slivnik, M. N. Preložnik, M. Fir, J. Jazbar, N. Č. Lipovec, I. Locatelli, H. L. Lauzon and V. U. Rovan, A randomized, placebo-controlled study of chitosan gel for the treatment of chronic diabetic foot ulcers (the CHITOWOUND study), BMJ Open Diabetes Res. Care, 2024, 12(3), e004195 CrossRef PubMed.
- B. C. Oliveira, B. G. de Oliveira, G. Deutsch, F. S. Pessanha and S. R. de Castilho, Effectiveness of a synthetic human recombinant epidermal growth factor in diabetic patients wound healing: pilot, double-blind, randomized clinical controlled trial, Wound Repair Regen., 2021, 29(6), 920–926 CrossRef PubMed.
- R. Koivuniemi, T. Hakkarainen, J. Kiiskinen, M. Kosonen, J. Vuola, J. Valtonen, K. Luukko, H. Kavola and M. Yliperttula, Clinical study of nanofibrillar cellulose hydrogel dressing for skin graft donor site treatment, Adv. Wound Care, 2020, 9(4), 199–210 CrossRef PubMed.
- K. B. Miyab, E. Alipoor, R. Vaghardoost, M. S. Isfeedvajani, M. Yaseri, K. Djafarian and M. J. Hosseinzadeh-Attar, The effect of a hydrolyzed collagen-based supplement on wound healing in patients with burn: A randomized double-blind pilot clinical trial, Burns, 2020, 46(1), 156–163 CrossRef PubMed.
- L. A. Chandler, O. M. Alvarez, P. A. Blume, P. J. Kim, R. S. Kirsner, J. C. Lantis and W. A. Marston, Wound conforming matrix containing purified homogenate of dermal collagen promotes healing of diabetic neuropathic foot ulcers: Comparative analysis versus standard of care, Adv. Wound Care, 2020, 9(2), 61–67 CrossRef PubMed.
- S. P. Bienz, N. Gadzo, A. N. Zuercher, D. Wiedemeier, R. E. Jung and D. S. Thoma, Clinical and histological wound healing patterns of collagen-based substitutes: An experimental randomized controlled trial in standardized palatal defects in humans, J. Clin. Periodontol., 2024, 51(3), 319–329 CrossRef CAS PubMed.
- J. Zhao, Z. Ruan, J. Zhao, Y. Yang, S. Xiao and H. Ji, Study on the timing of first dressing change with alginate dressing application in PICC placement among tumor patients, J. Cancer Res. Ther., 2022, 18(7), 2013–2020 CrossRef PubMed.
- R. Loera-Valencia, R. E. Neira, B. P. Urbina, A. Camacho and R. B. Galindo, Evaluation of the therapeutic efficacy of dressings with ZnO nanoparticles in the treatment of diabetic foot ulcers, Biomed. Pharmacother., 2022, 155, 113708 CrossRef CAS PubMed.
- G. Wang, X. Li, S. Ju, Y. Li, W. Li, H. He, Y. Cai, Z. Dong and W. Fu, Effect of electrospun poly (L-lactide-co-caprolactone) and formulated porcine fibrinogen for diabetic foot ulcers, Eur. J. Pharm. Sci., 2024, 198, 106800 CrossRef CAS PubMed.
- R. W. Kartika, I. Alwi, F. D. Suyatna, E. Yunir, S. Waspadji, S. Immanuel, S. Bardosono, S. Sungkar, J. Rachmat, M. Hediyati and T. Silalahi, Wound healing in diabetic foot ulcer patients using combined use of platelet rich fibrin and hyaluronic acid, platelet rich fibrin and placebo: an open label, randomized controlled trial, Acta Med. Indones., 2021, 53(3), 268 Search PubMed.
- W. Ibraheem, W. H. Jedaiba, A. M. Alnami, L. A. Hussain Baiti, S. M. Ali Manqari, A. Bhati, A. Almarghlani and M. Assaggaf, Efficacy of hyaluronic acid gel and spray in healing of extraction wound: A randomized controlled study, Eur. Rev. Med. Pharmacol. Sci., 2022, 26(10), 3444–3449 CAS.
- F. Graziani, R. Izzetti, M. Perić, U. Marhl, M. Nisi and S. Gennai, Early periodontal wound healing after chlorhexidine rinsing: a randomized clinical trial, Clin. Oral Invest., 2024, 28(6), 354 CrossRef PubMed.
- C. Sabando, W. Ide, M. Rodríguez-Díaz, G. Cabrera-Barjas, J. Castaño, R. Bouza, N. Müller, C. Gutiérrez, L. Barral, J. Rojas and F. Martínez, A novel hydrocolloid film based on pectin, starch and Gunnera tinctoria and Ugni molinae plant extracts for wound
dressing applications, Curr. Top. Med. Chem., 2020, 20(4), 280–292 CrossRef CAS PubMed.
- M. A. Alsakhawy, D. A. Abdelmonsif, M. Haroun and S. A. Sabra, Naringin-loaded Arabic gum/pectin hydrogel as a potential wound healing material, Int. J. Biol. Macromol., 2022, 222, 701–714 CrossRef CAS PubMed.
- D. Gou, P. Qiu, F. Hong, Y. Wang, P. Ren, X. Cheng, L. Wang, T. Liu, J. Liu and J. Zhao, Polydopamine modified multifunctional carboxymethyl chitosan/pectin hydrogel loaded with recombinant human epidermal growth factor for diabetic wound healing, Int. J. Biol. Macromol., 2024, 274, 132917 CrossRef CAS PubMed.
- K. Ehab, O. Abouldahab, A. Hassan and K. M. Fawzy El-Sayed, Alvogyl and absorbable gelatin sponge as palatal wound dressings following epithelialized free gingival graft harvest: a randomized clinical trial, Clin. Oral Invest., 2020, 24, 1517–1525 CrossRef PubMed.
- X. Li, H. Zhang and Y. Zhang, Repair of large traumatic tympanic membrane perforation using ofloxacin otic solution and gelatin sponge, Braz. J. Otorhinolaryngol., 2022, 88, 9–14 CrossRef PubMed.
- S. N. Hussein, G. S. Kotry, A. S. Eissa, L. A. Heikal and Y. Y. Gaweesh, Efficacy of a Novel Melatonin-Loaded Gelatin Sponge in Palatal Wound Healing, Int. Dent. J., 2024, 74(6), 1350–1361 CrossRef PubMed.
- K. Mariia, M. Arif, J. Shi, F. Song, Z. Chi and C. Liu, Novel chitosan-ulvan hydrogel reinforcement by cellulose nanocrystals with epidermal growth factor for enhanced wound healing: In vitro and in vivo analysis, Int. J. Biol. Macromol., 2021, 183, 435–446 CrossRef CAS PubMed.
- S. Kikionis, M. Koromvoki, A. Tagka, E. Polichronaki, A. Stratigos, A. Panagiotopoulos, A. Kyritsi, V. Karalis, A. Vitsos, M. Rallis and E. Ioannou, Ulvan-based nanofibrous patches enhance wound healing of skin trauma resulting from cryosurgical treatment of keloids, Mar. Drugs, 2022, 20(9), 551 CrossRef CAS PubMed.
- Z. Li, W. Xu, X. Wang, W. Jiang, X. Ma, F. Wang, C. Zhang and C. Ren, Fabrication of PVA/PAAm IPN hydrogel with high adhesion and enhanced mechanical properties for body sensors and antibacterial activity, Eur. Polym. J., 2021, 146, 110253 CrossRef CAS.
- R. M. Hanes and A. L. Hanes, Inventors; HCS Innovation LLC, assignee. Natural polymer based tissue adhesive with healing promoting properties, US Pat., US10835635, 2020 Search PubMed.
- S. Liu, J. Wang, X. Wang, L. Tan, T. Liu, Y. Wang, Y. Shi, Z. Zhang, S. Ding, K. Hou and W. Zhang, Smart chitosan-based nanofibers for real-time monitoring and promotion of wound healing, Int. J. Biol. Macromol., 2024, 282, 136670 CrossRef CAS PubMed.
- Q. Tang, Z. Huang, B. Wang and H. Lu, Surfactant-free aqueous foams stabilized with synergy of xanthan-based amphiphilic biopolymer and nanoparticle as potential hydraulic fracturing fluids, Colloids Surf., A, 2020, 603, 125215 CrossRef CAS.
- R. Yadav, R. Kumar, M. Kathpalia, B. Ahmed, K. Dua, M. Gulati, S. Singh, P. J. Singh, S. Kumar, R. M. Shah and P. K. Deol, Innovative approaches to wound healing: insights into interactive dressings and future directions, J. Mater. Chem. B, 2024, 12(33), 7977–8006 RSC.
- K. S. Leung, S. Shirazi, L. F. Cooper and S. Ravindran, Biomaterials and extracellular vesicle delivery: current status, applications and challenges, Cells, 2022, 11(18), 2851 CrossRef CAS PubMed.
- R. M. Hanes and A. L. Hanes, Inventors; HCS Innovation LLC, assignee. Natural polymer-based tissue adhesive with healing-promoting properties, US Pat., US11571492, 2023 Search PubMed.
- J. Yang and S. Wang, Polysaccharide-based multifunctional hydrogel bio-adhesives for wound healing: A review, Gels, 2023, 9(2), 138 CrossRef CAS PubMed.
- Y. Feng, Y. He, X. Lin, M. Xie, M. Liu and Y. Lvov, Assembly of clay nanotubes on cotton fibers mediated by biopolymer for robust and high-performance hemostatic dressing, Adv. Healthcare Mater., 2023, 12(1), 2202265 CrossRef CAS PubMed.
- F. Diban, S. Di Lodovico, P. Di Fermo, S. D'Ercole, S. D'Arcangelo, M. Di Giulio and L. Cellini, Biofilms in chronic wound infections: innovative antimicrobial approaches using the in vitro Lubbock chronic wound biofilm model, Int. J. Mol. Sci., 2023, 24(2), 1004 CrossRef PubMed.
|
This journal is © The Royal Society of Chemistry 2025 |
Click here to see how this site uses Cookies. View our privacy policy here.