DOI:
10.1039/D5FO00435G
(Paper)
Food Funct., 2025,
16, 2656-2668
Effect of long-term moderate red wine consumption in CD1 female mice
Received
22nd January 2025
, Accepted 18th February 2025
First published on 19th February 2025
Abstract
In the early 80s, the so-called “French paradox” was proposed, that is, a correlation between wine consumption, a diet rich in fats, and low mortality from coronary disease. Conversely, it is well established that excessive alcohol consumption increases the risk of cirrhosis and cancer, but few studies have investigated the effects of moderate alcohol consumption. However, all these conclusions were derived from epidemiological population studies that may be subject to distortions due to multiple factors. Here, the effect of moderate consumption of red wine on health throughout life was examined in a murine model. Different variables were evaluated in groups of female animals that were fed a standard or a fat diet throughout their adult life and given water, or wine or alcohol diluted in water in proportions similar to what is considered moderate consumption in humans. Our results showed few differences in most of the analyzed variables (body weight, liver profile and survival rate) between the different female mouse groups. The most remarkable findings were observed in the fat-diet groups that showed more frequent and severe liver lesions and a lower average ovarian weight. Moreover, moderate and prolonged ethanol consumption significantly affected telomere length only when the diet was high in fat, whereas wine consumption showed no difference compared to water, pointing to a possible predominant role of the compounds, particularly polyphenols present in wine. On the other hand, wine-drinking mice fed a fat diet had more oocytes than those in the ethanol-drinking group. Overall, our data suggest that long-term moderate red wine consumption does not substantially influence the health of female mice.
Introduction
After 1979, when it was observed that the French population, which usually consumes a diet rich in saturated fats, had a lower mortality rate due to coronary diseases than other populations studied, a hypothesis was put forward, the so-called French paradox,1 that correlated this lower mortality with wine consumption. Since then, it has been speculated whether this protection could be due to moderate alcohol drinking in general or to the specific ingestion of red wine due to its high polyphenol content,2–5 which plays a crucial role in health due to its antioxidant and anti-inflammatory properties.6,7
However, it is also well known that excessive alcohol consumption increases the risk of cirrhosis, cancer and other diseases.8–11 Furthermore, recently, a population study suggested that even lower levels of drinking could increase the risk of cancer.12
This ongoing debate on alcohol consumption13 has been deepened by a recent publication evaluating the health risks associated with its consumption and the amount of alcohol that could minimize some health risks.14 Setting guidelines and defining an acceptable level of risk will be desirable, but is unlikely to be universal, as several factors influence policymakers’ decisions (easy and widespread availability, wine growers, alcohol industry interests, etc.).
Despite all these considerations, the effect of moderate wine consumption, considered as a limit of 20 g per day and 30 g per day (2 and 3 standard drink units) for women and men, respectively,15 has not yet been analysed in depth. In fact, human population studies carried out on the effects of alcohol are epidemiological studies that could be biased due to multiple confounding factors (geographic location, lifestyle, diet, etc.).16,17
In this context, the mouse model has been used for years to investigate the effects of alcohol on the progression of alcoholic liver disease.18–20 Nevertheless, it should be noted that these studies have been mainly undertaken with a high alcohol intake and over a relatively short period.21–23
The aim of this work was to address, in the murine model, whether moderate consumption of red wine throughout life had any effect on health. To do so, different variables were evaluated in groups of animals that were fed throughout their adult lives (2 to 20 months) with a standard or a fat diet. The animals were given water as a drink, or wine or alcohol diluted in water in proportions similar to what is considered moderate consumption in humans.
Materials and methods
Experimental design
Hsd:ICR(CD1) female mice were housed in an animal facility in a controlled environment of temperature and photoperiod (23 ± 2 °C and 14 h light
:
10 h dark cycle) with ad libitum access to food and drink and daily monitored. Animals were handled in strict accordance with the guidelines of the European Community 86/609/CEE, and the procedures were approved by the Ethical Committee of Animal Experimentation of INIA-CSIC and by the Division of Animal Protection of the Comunidad de Madrid (PROEX 191.8/24).
Animals were weaned one month after birth and, after a four week acclimatization period (equivalent to about 18 years in humans), in which they were fed a standard diet (SAFE®150, Scientific Diet, Rosenberg, Germany) and water, they were randomly assigned to two groups (60 mice per group) (Fig. 1). One group continued to be fed standard food (12.6% energy content from lipids, 21% from proteins, and 66.4% from nitrogen free extract of starch and sugars), and the other with high-Fat food (44.4% energy content from lipids, 15.3% from proteins, and 40.3% from nitrogen free extract of starch and sugars, SAFE®U8955 v. 2, Scientific Diet). Each group was subdivided into three subgroups (20 mice per subgroup) that were given water, or ethanol or wine diluted in water, as a drink for the remainder of the experiment, until they were 20 months old, corresponding to a human age of approximately 70 years.24 Beverage and food consumption was measured weekly and biweekly, respectively, by subtracting the mass (g), or volume (mL), of remaining food and drink from the initial food and drink mass, or volume, provided to each cage. Body weight was measured at 2 weeks and then every 6 weeks until the end of the experiment (72 weeks).
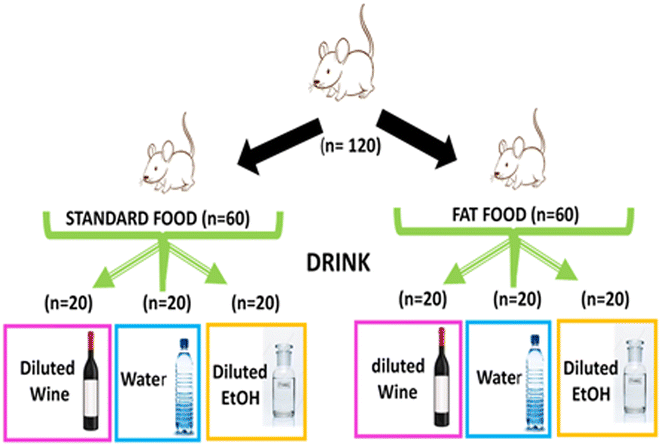 |
| Fig. 1 Schematic representation of the experimental design. | |
The amount of wine (or ethanol) provided to the mice was proportional to what is considered moderate alcohol consumption in humans,15 that is, 20 g per day for an adult female. Here, considering an average weight of 60 kg for an adult female and 30–40 g for a female mouse (which drinks 4–5 ml per day), the animals in the wine and alcohol groups were given, as a drink, water with 25 ml of wine per L (14% alcohol), or water with 3.5 ml of pure ethanol per L (ethanol absolute pharma grade, PanReac Quimica, Barcelona, Spain), respectively.
Three red wines, representing the three main European producing countries (Barbera d'Asti 2020, Italy; Bordeaux 2019, France; and Rioja 2018, Spain) with similar alcohol content (14% vol.) reported on the label and reasonably priced for consumers (6–9 € per bottle of 750 mL), were purchased from local supermarkets. Each month, a bottle of wine from a different origin was opened, aliquoted and, after dilution, used as a drink for the animals of the wine-drinking groups.
At the end of the experiment (18 months after ethanol and wine were added to the diet), surviving mice were anesthetized using isoflurane and humanely sacrificed. Tissue samples (liver, ovaries, hearts, and tails) were collected for histopathological, oocyte and telomere analyses.
Wine analysis
The main chemical–physical parameters and polyphenolic content of the three wines were determined as described,25–27a posteriori, to ensure that the choice of wines for the experiment was not influenced in any way.
Biochemical analyses
Blood from the submandibular vein was collected at 9 and 18 months. Plasma was separated using a micro-sample tube serum CAT (Sarstedt, Nümbrecht, Germany) by centrifugation (10
000g, 5 min at 20 °C) and then frozen at −80 °C until analyses. The levels of alkaline phosphatase, alanine transaminase, aspartate aminotransferase, gamma-glutamyl transferase, creatinine, triglycerides and cholesterol were analyzed using a Cobas® c503 analytical unit (Roche Diagnostics, Basel, Switzerland), following the equipment instructions.
Glucose tolerance test
The glucose tolerance test (GTT), the most commonly used method for assessing glucose homeostasis in rodents,28 was performed (10 mice per group) 6 months after the start of the experiment. Briefly, overnight-fasted mice were given an intraperitoneal glucose dose (1.5 g glucose per kg body weight), and blood glucose levels were measured at different time points: before injection and 15, 30, 60, 90, and 120 min after glucose load. Blood glucose was measured with a standard glucose meter (Glucomen Areo; Menarini, Naples, Italy) on blood samples collected from the tip of the tail vein, and the area under the curve (AUC) was calculated for each group.
Ovary and oocyte analysis
Oocytes were collected from 20-month-old female mice superovulated with 7.5 IU of pregnant mare serum gonadotrophin (Folligon 500, Intervet, Spain). After 50 hours, ovaries were harvested and placed in M2 medium at 37 °C. Cumulus–oocyte complexes (COCs) were retrieved by follicle puncture with a 21G needle and matured in vitro for 17 h in M199 medium (Sigma-Aldrich, Madrid, Spain) supplemented with 10% fetal calf serum under 5% CO2 at 37 °C. Mature COCs were stripped of cumulus cells and treated with Tyrode acid solution (Sigma-Aldrich) to remove the zona pellucida. Oocytes were then washed in PBS, transferred individually to 0.1 ml qPCR tubes (Corbett Research, Sydney, Australia), frozen in liquid nitrogen, and stored at −80 °C for analysis.
Absolute telomere length (ATL) in oocytes
Oocytes were digested overnight at 55 °C in 9 μl of 100 mg ml−1 proteinase K solution, followed by enzyme inactivation at 95 °C for 10 min. Absolute qPCR was performed on the entire digested sample using telomere-specific primers (telomere forward: CGGTTTGTTTGGGTTTGGGTTTGGGTTTGGGTTTGGGTT; reverse GGCTTGCCTTACCCTTACCCTTACCCTTACCCTTACCCT; Rn18S forward AGAAACGGCTACCACATCCAA; reverse CCTGTATTGTTATTTTTCGTCACTACCT).29 Genomic DNA (Rn18S) was quantified in five samples per group to confirm consistent DNA content across oocytes. qPCR was conducted on a Rotor Gene 6000 Real-Time PCR system (Corbett Research) under the following conditions: 94 °C for 3 min, followed by 40 cycles of 94 °C for 10 s, 60 °C for 30 s, and 72 °C for 30 s.30 Due to the limited DNA quantity, absolute qPCR was used instead of relative qPCR. Cycle threshold (Ct) values were compared directly to the group with the highest average Ct (shortest telomere length, TL) without normalization to an internal control. Fold-change in TL was calculated using the ΔΔCt method, with the group having the shortest TL set as the reference (fold-change = 1).
Relative telomere length (RTL) in somatic tissue samples
Somatic tissue samples were collected via tail biopsy and digested overnight at 55 °C with 30 μl of proteinase K (1.25 μg μl−1) in Tris–NaCl–EDTA (STE) buffer. DNA was extracted by digesting samples with 30 μl of proteinase K (1.25 μg μl−1) in STE buffer and 5 μl of 0.1 M dithiothreitol (DTT) at 55 °C overnight. DNA was purified using phenol/chloroform, precipitated with isopropanol, quantified by spectrophotometry (BioPhotometer, Eppendorf, Madrid, Spain), and diluted to 50 ng μl−1. Telomeric length was determined by qPCR using 2 μl of diluted DNA per 20 μl reaction on a Rotor Gene 6000 Real-Time PCR machine (Corbett Research) with the same primers and cycling conditions described above for ATL determination. Relative telomere length (RTL) was calculated using the real-time qPCR method,29 normalizing telomere repeat values to DNA content (quantified using the multicopy gene Rn18S) by the comparative Ct method.31
Histopathological analysis
Samples from the left lobe of the liver of each animal were fixed by immersion in 10% buffered formalin solution for 1 week. After fixation, the samples were routinely processed, embedded in paraffin wax, sectioned at 4 microns, and stained with haematoxylin and eosin for histopathologic evaluations. A single veterinary pathologist performed a blind evaluation of potential histopathologic lesions and their severity. The histopathological parameters were graded following a semi-quantitative scoring system as follows: (0) no lesion; (1) minimal lesion; (2) mild lesion; (3) moderate lesion; and (4) severe lesion.
Statistical analysis
PRISM GraphPad 6.0 was used to determine the statistical significance of the data. Two-way ANOVA and Tukey's multiple comparison tests were performed for comparisons. Kaplan–Meier survival analysis was performed for survival curves. For ovarian and telomere analyses, one-way analysis of variance (followed by multiple pair-wise comparisons using the Holm–Sidak test) was used for the analysis of absolute or relative TL differences between more than two groups. Statistical differences in figures are displayed as *p < 0.05.
Results
Wine composition
The main chemical–physical parameters and polyphenolic content of the three red wines from different geographical origins were analyzed (Table 1). The three wines had similar alcohol content and chemical–physical composition but, as expected due to the different grapes and oenological techniques used, they showed differences both in the total quantity of polyphenols and in the polyphenolic classes present.
Table 1 Chemical–physical and polyphenolic content analysis of the three red wines. Values are presented as means ± SD of three independent determinations
|
|
RIOJA 2018 |
BORDEAUX 2019 |
BARBERA 2020 |
n.d., not detected. |
|
Free SO2 (mg L−1) |
14.02 ± 0.18 |
13.59 ± 0.16 |
18.45 ± 0.09 |
|
Total SO2 (mg L−1) |
37.78 ± 0.34 |
25.17 ± 0.38 |
45.49 ± 1.18 |
|
Alcohol (%) |
13.7 ± 0.01 |
14.24 ± 0.07 |
14.02 ± 0.05 |
|
Glycerol (g L−1) |
9.70 ± 0.08 |
8.37 ± 0.05 |
8.24 ± 0.06 |
|
Glucose (g L−1) |
n.d |
0.54 ± 0.01 |
0.52 ± 0.01 |
|
Fructose (g L−1) |
n.d |
0.91 ± 0.01 |
0.26 ± 0.01 |
|
Total extracts (g L−1) |
25.95 ± 0.15 |
31.40 ± 0.10 |
29.53 ± 0.15 |
|
pH |
3.66 ± 0 |
3.63 ± 0 |
3.55 ± 0 |
|
Total acidity (g L−1) |
4.65 ± 0 |
4.60 ± 0 |
5.22 ± 0 |
|
Color intensity (A420 + A520 + A620) |
0.60 ± 0 |
1.17 ± 0 |
0.60 ± 0 |
|
Color hue (A420/A520) |
0.91 ± 0 |
0.86 ± 0 |
0.83 ± 0 |
|
Total flavonoids (mg L−1) |
1419 ± 2.2 |
3042 ± 0.06 |
1235 ± 3.7 |
|
Total anthocyanins (mg L−1) |
131 ± 0.53 |
192 ± 0.33 |
154 ± 0.31 |
|
Total polyphenols (mg L−1) |
2255 ± 10.1 |
4060 ± 3.40 |
2060 ± 9.75 |
|
Methyl cellulose precipitable tannins (MTC) (mg L−1) (mg L−1) |
1290 ± 10.0 |
3007 ± 52.87 |
1199 ± 8.76 |
Polymeric pigments |
Large polymeric pigments (LPPs) % |
0.38 ± 0.004 |
0.49 ± 0.01 |
0.33 ± 0 |
Small polymeric pigments (SPPs) % |
0.30 ± 0.003 |
0.26 ± 0.01 |
0.32 ± 0 |
|
Gallic acid (mg L−1) |
42.9 ± 1.43 |
115.10 ± 0.35 |
51.02 ± 0.21 |
|
Radical scavenging capacity (DPPH inhibition %) |
73.8 ± 1.90 |
93.43 ± 0.05 |
75.25 ± 2.58 |
HCTA |
t-Caftaric acid (mg L−1) |
21.9 ± 0.27 |
47.44 ± 0.73 |
114.62 ± 2.51 |
cis-Coumaric acid (mg L−1) |
3.7 ± 0.045 |
2.86 ± 0.09 |
5.71 ± 0.48 |
trans-Coumaric acid (mg L−1) |
14.3 ± 0.40 |
11.67 ± 0.29 |
34.99 ± 0.76 |
trans-Fertaric acid (mg L−1) |
2.6 ± 0.03 |
2.14 ± 0.12 |
5.51 ± 0.22 |
Caffeic acid (mg L−1) |
6.6 ± 0.29 |
9.03 ± 0.13 |
8.74 ± 0.22 |
Coumaric acid (mg L−1) |
3.3 ± 0.30 |
6.26 ± 0.16 |
5.19 ± 0.03 |
Flavonols |
Quercetin 3-O-glucoside (mg L−1) |
2.30 ± 0.14 |
4.19 ± 0.07 |
9.44 ± 0.19 |
Kaempferol 3-O-glucoside (mg L−1) |
5.81 ± 0.78 |
7.96 ± 0.24 |
6.44 ± 0.31 |
Quercetin (mg L−1) |
1.97 ± 0.87 |
14.15 ± 0.44 |
15.02 ± 0.23 |
Flavan-3-ols |
Catechin (mg L−1) |
26.0 ± 1.93 |
58.98 ± 0.13 |
53.32 ± 0.88 |
epi-Catechin (mg L−1) |
14.0 ± 1.10 |
62.38 ± 2.43 |
37.03 ± 9.38 |
Dimer B1 (mg L−1) |
22.6 ± 3.76 |
46.53 ± 2.67 |
46.13 ± 1.89 |
Dimer B2 (mg L−1) |
n.d |
29.78 ± 1.97 |
19.41 ± 0.29 |
Dimer B3 (mg L−1) |
37.8 ± 3.06 |
52.97 ± 3.74 |
47.00 ± 1.60 |
Body weight gain
All animals gained weight throughout the experiment, which was greater in the fat diet than in the standard diet groups (Fig. 2). Within the same diet, there were no significant differences between the three groups. However, individual variability was high, as reflected by the standard deviations.
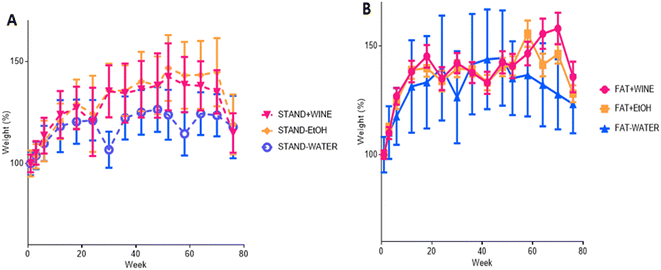 |
| Fig. 2 Weight gain (%) of mice throughout the experiment. (A) Standard diet and (B) fat diet. | |
Food and drink intake
Drink and food consumption was measured weekly and biweekly, respectively. In all cases, consumption was lower in the fat diet groups compared to the standard diet groups, with the water-drinking groups drinking and eating more, regardless of the diet (Table 2).
Table 2 Average weekly food (g) and drink (ml) ingestion of the different groups (n = 20) during the experiment
|
Food ingestion (g per week) |
|
Drink ingestion (mL per week) |
Water |
Wine |
Ethanol |
Water |
Wine |
Ethanol |
Standard diet |
588 |
575 |
578 |
Standard diet |
791 |
713 |
778 |
Fat diet |
402 |
387 |
377 |
Fat diet |
673 |
604 |
571 |
Biochemical analyses
Blood biochemical analyses were conducted at 9 and 18 months. Although the values recorded between individuals in each group were quite variable, they were all within normal limits for female CD1 mice.32
No significant differences were observed between any of the groups with the same diet (standard or fat) in the liver enzymes (alkaline phosphatase, alanine transaminase, aspartate aminotransferase, and gamma-glutamyl transferase) analyzed at 9 or 18 months, nor in the creatinine levels (Table 3). In the groups on a standard diet, the only significant difference was recorded in triglyceride levels between the group that drank water and the group that drank wine at 18 months. In mice on a fat diet, a significant difference was only observed in cholesterol levels between the group that drank water and the group that drank alcohol at 9 months.
Table 3 Serum biochemical values after 9 and 18 months of experimental diets
|
Standard food |
High-fat food |
Wine |
Alcohol |
Water |
Wine |
Alcohol |
Water |
Means ± SD, n = 10 for each group. aP < 0.05 vs. water. bP < 0.05 vs. water. ALP, alkaline phosphatase; ALT, alanine aminotransferase; AST, aspartate aminotransferase; GGT, gamma-glutamyl transferase; TG, triglycerides. |
MONTH 9
|
ALP (UI L−1) |
64.7 ± 9.9 |
90.0 ± 46.8 |
66.5 ± 17.3 |
112.5 ± 20.3 |
121.6 ± 29.2 |
124.0 ± 6.1 |
ALT (UI L−1) |
12.6 ± 7.9 |
15.6 ± 6.3 |
13.1 ± 3.2 |
11.8 ± 5.1 |
7.2 ± 4.8 |
8.4 ± 2.9 |
AST (UI L−1) |
67.0 ± 11.3 |
140.8 ± 71.6 |
281.7 ± 31.9 |
47.0 ± 12.5 |
103.0 ± 55.0 |
112.0 ± 60 |
GGT (UI L−1) |
6.8 ± 3.0 |
9.5 ± 3.2 |
9.6 ± 7.3 |
10.9 ± 8.2 |
6.3 ± 5.8 |
9.7 ± 8.2 |
Creatinine (mg dL−1) |
0.43 ± 0.13 |
0.41 ± 0.27 |
0.47 ± 0.16 |
0.33 ± 0.09 |
0.36 ± 0.10 |
0.44 ± 0.10 |
Cholesterol (mg dL−1) |
60.6 ± 12.8 |
82.7 ± 23.8 |
66.2 ± 19.4 |
110.1 ± 13.8 |
124.7 ± 24.1a |
95.2 ± 14.6 |
TG (mg dL−1) |
187.3 ± 28.7 |
207.8 ± 58.8 |
237.4 ± 77.2 |
86.8 ± 12.2 |
85.6 ± 15.0 |
70.1 ± 6.2 |
MONTH 18
|
ALP (UI L−1) |
75.6 ± 20.7 |
72.5 ± 14.0 |
74.1 ± 7.9 |
61.6 ± 12.4 |
77.9 ± 19.5 |
72.9 ± 18.5 |
ALT (UI L−1) |
25.7 ± 10.6 |
27.5 ± 7.1 |
22.9 ± 9.8 |
17.7 ± 10.4 |
12.2 ± 4.7 |
18.7 ± 12.5 |
AST (UI L−1) |
97.3 ± 26.2 |
83.3 ± 13.1 |
97.5 ± 23.2 |
82.6 ± 32.1 |
106.4 ± 64.5 |
94.6 ± 14.5 |
GGT (UI L−1) |
33.2 ± 13.2 |
24.8 ± 9.6 |
22.4 ± 11.3 |
33.1 ± 21.4 |
27.7 ± 14.3 |
25.2 ± 10.2 |
Creatinine (mg dL−1) |
0.30 ± 0.06 |
0.23 ± 0.17 |
0.35 ± 0.10 |
0.44 ± 0.20 |
0.32 ± 0.08 |
0.34 ± 0.14 |
Cholesterol (mg dL−1) |
62.7 ± 12.5 |
58.9 ± 11.2 |
66.7 ± 20.5 |
103.2 ± 24.9 |
102.9 ± 36.4 |
101.6 ± 55.0 |
TG (mg dL−1) |
128.8 ± 26.9b |
145.7 ± 41.1 |
196.1 ± 68.6 |
118.0 ± 39.0 |
91.0 ± 26.9 |
123.8 ± 38.9 |
Glucose tolerance test
Glucose tolerance tests (GTTs) were conducted at 6 months in 10 mice per group. On the standard diet, the wine group metabolized glucose significantly (95%) better than the water group (Fig. 3). Although not statistically significant, a similar tendency was observed between the three groups on the fat diet.
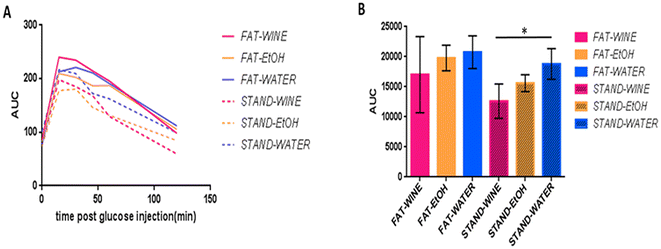 |
| Fig. 3 Glucose tolerance test (GTT) at 6 months. (A) Glucose curves. (B) Area under the curve (AUC) for the different groups. *Significant differences based on the ANOVA test (p ≤ 0.05). | |
Histopathological analysis
The spontaneous non-neoplastic findings observed in the livers of the mice were considered background changes because of age and have not been included in the histopathological evaluation. In mice fed standard food (Fig. 4a–f), no remarkable lesions were observed in those that drank water, and the lesions found in the other two groups were quite similar in both. Centrilobular and periportal hydropic degeneration, accompanied by occasional necrotic hepatocytes, was recorded in five and three wine-drinking mice and in five and one ethanol-drinking mice, respectively. Additionally, focal lipidosis was observed in one ethanol-drinking mouse. On the other hand, among the fat-fed groups (Fig. 4g–l), the most characteristic lesions observed in mice drinking water were the presence of centrilobular hydropic degeneration and focal lipidosis in three and two mice, respectively. In the wine group, centrilobular and periportal hydropic degeneration accompanied by occasional necrotic hepatocytes was observed in five and four mice, and occasional presence of focal lipidosis in one animal, while in the ethanol group, centrilobular hydropic degeneration was recorded in two mice.
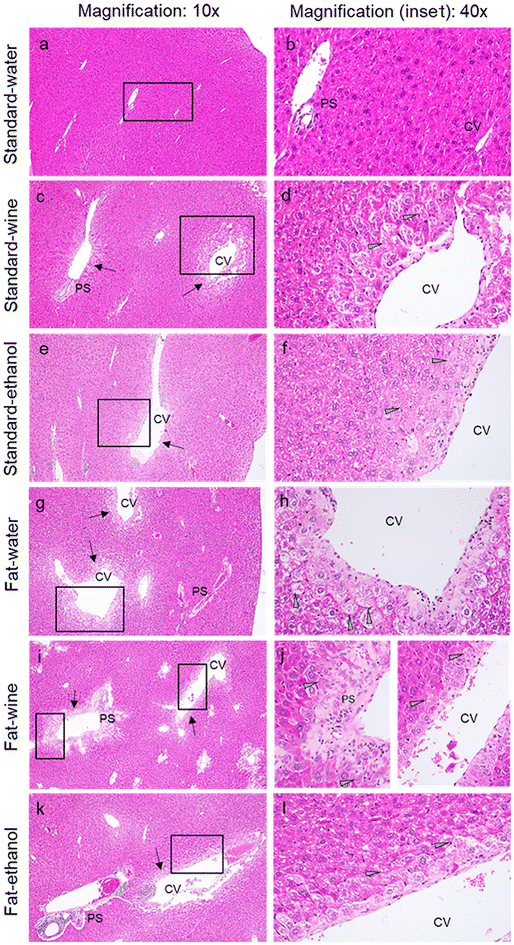 |
| Fig. 4 Representative histopathological sections of the liver of mice from the different groups (haematoxylin and eosin staining). The extent of liver lesions is shown (×10 magnification) along with selected liver areas (black boxes), including details of the most representative histopathological findings (×40 magnification). Standard food-fed mice that drank water (a and b), wine (c and d), or ethanol (e and f). Fat fed mice that drank water (g and h), wine (i and j), or ethanol (k and l). Black arrows: areas with mild to moderate centrilobular and periportal hydropic degeneration; green arrowheads: necrotic hepatocytes within areas with hydropic degeneration; (CV): centrilobular vein; (PS): portal space. | |
Oocyte analysis
When the six experimental groups were analyzed separately, no differences in ovarian weight were observed between groups (Fig. 5A). However, when groups were pooled based on whether the diet was enriched with fat or not, a lower ovarian weight was observed in mice fed the fat diet (Fig. 5B). Similarly, when analyzing relative ovarian weights adjusted for mouse body weight, the results showed no significant differences, except when comparing pooled groups on a standard or fat diet (Fig. 5C and D). Interestingly, when we analyzed the average number of germinal vesicle (GV) oocytes recovered per female, no differences were found among the three groups on the standard diet, but, among the fat diet groups, the ones that consumed wine had more oocytes than the ethanol groups, regardless of whether the diet was standard or fat (Fig. 5E).
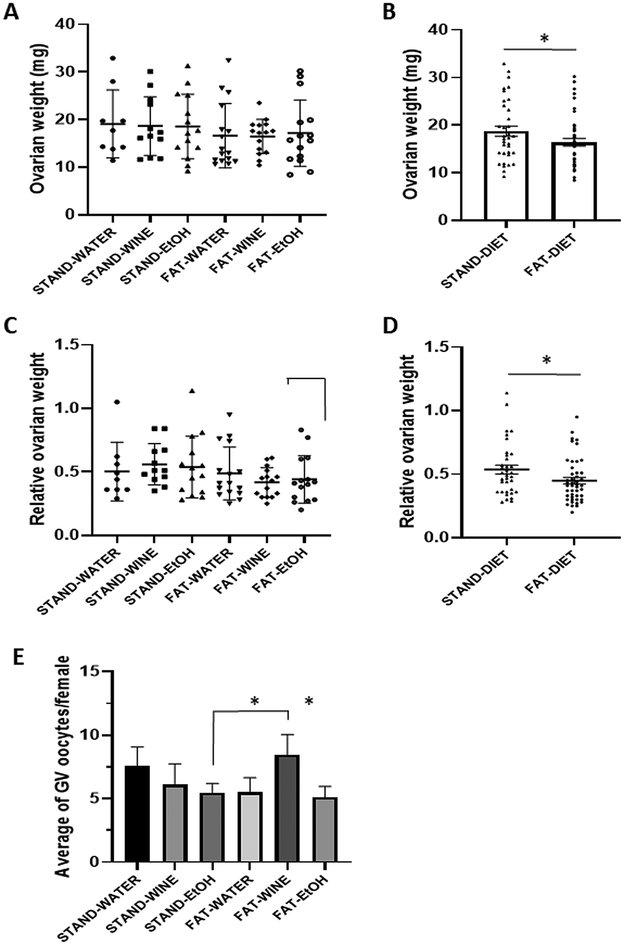 |
| Fig. 5 Ovarian weight and average number of germinal vesicle (GV) oocytes recovered per female from the different groups. Ovarian weight of the individual groups (A) or grouped by the type of diet (B). Relative ovarian weight of the individual groups (C) or grouped by the type of diet (D). Average number of germinal vesicle (GV) oocytes recovered per female from the different groups (E). *Significant differences based on the ANOVA test (p ≤ 0.05). | |
Telomere analysis
The analysis of oocyte telomere length indicates that there was no size difference between the groups of mice fed a standard diet. In contrast, when the diet was fat, the oocytes in the wine group had statistically longer telomeres compared to the ethanol group (Fig. 6A). Regarding the size of heart cell telomeres, again, no differences were observed between the groups fed a standard diet. However, among those on a fat diet, the mice from the wine group had telomeres of similar length to those in the water group and longer than the ethanol group (Fig. 6B). Similarly, no differences were found in the telomeres of tail cells among groups fed a standard diet. In contrast, for groups on a fat diet, the telomeres in the ethanol group were significantly shorter than those in the other two groups (Fig. 6C).
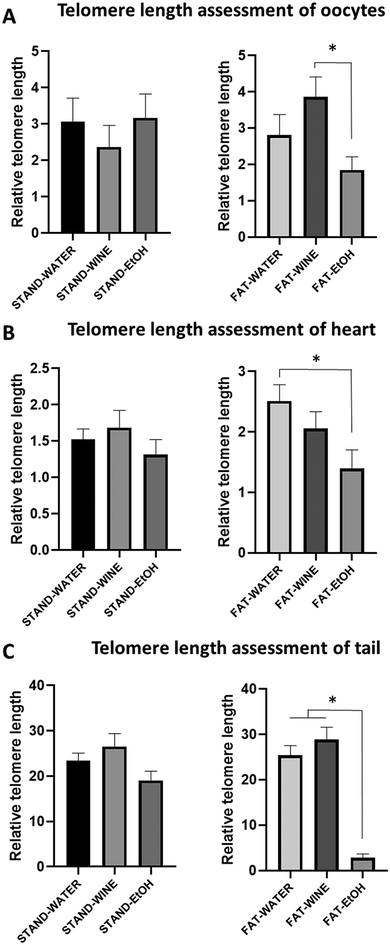 |
| Fig. 6 Changes in telomere length induced by alcohol consumption in oocytes and somatic cells of female mice across different groups. (A) Relative telomere length in oocytes, (B) in somatic cells from the heart, and (C) in somatic cells from the tail. Significant differences based on the ANOVA test (p ≤ 0.05). | |
Survival
The survival rate was higher on the standard diet than on the fat diet (Fig. 7), even though no statistically significant differences were observed.
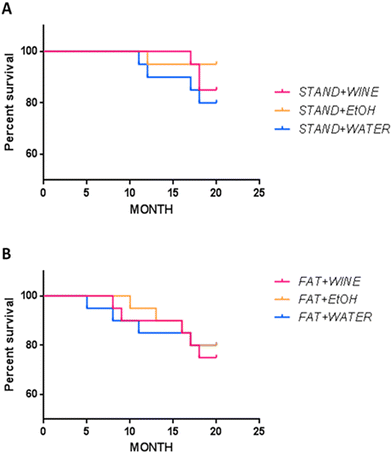 |
| Fig. 7 Survival rate (%) of mice throughout the experiment. (A) Standard diet and (B) fat diet. | |
Discussion
The association between excessive alcohol consumption and an increased risk of various diseases, such as alcoholic liver disease and cancer, is globally accepted.8–11 Despite this, moderate wine consumption is very popular in people's daily lives around the world, and yet, until recently, little attention has been paid to assessing the effects of long-term moderate wine consumption on health.
The observation in the late 1970s that the French population, which tends to maintain a diet rich in saturated fats, showed a lower mortality rate from cardiovascular diseases than other populations studied was associated with wine consumption and led to the so-called French paradox.1 However, there is still debate as to whether this supposed effect is partly attributable to moderate alcohol consumption in general or to the specific ingestion of red wine due to its high polyphenol content,2–5 higher than that found in white wines.33 Subsequent epidemiological studies reported that moderate alcohol consumption was associated with reduced risks of cardiovascular events and a decreased risk of type 2 diabetes and other metabolic-related diseases.34–36 However, it should be noted that studies carried out on the positive or negative effects of alcohol consumption on human populations are epidemiological; as such, their conclusions could be biased due to multiple confounding factors (geographic location, lifestyle, age, diet, etc.).16,17
In this regard, mice are a suitable model to investigate the effects of alcohol on the progression of alcoholic liver disease18–20 and aging,37 including telomere characterization,38 among others. However, studies on the effect of alcohol have been mainly undertaken with a high intake and over a relatively short period.21–23
Here we have addressed, in the murine model, whether moderate consumption of red wine or ethanol throughout life has any effect on the health of female mice. To do this, we have compared groups of animals that were supplied with water, wine or alcohol (in proportions similar to what is considered moderate consumption in humans),15 in a free-choice consumption model, the most widely recommended for these types of studies.19 To avoid bias, three representative red wines, with similar alcohol content, from the three main European producing countries (Italy, France and Spain), were purchased from local groceries and monthly administered sequentially.
Along with the well-established relationship between high alcohol consumption and alcoholic liver disease and cancer, among other complications,8–11 a high-fat diet is also recognized as an important factor in the development of diverse metabolic and cardiovascular diseases.39–41 Therefore, in this study, the drinking groups were fed either a standard diet or a fat diet. The standard food administered had a 12.6% energy content from lipids and the high-Fat food had 44%, similar to the typical American or European diet, which is considered a tolerable fat human diet,42 and lower than what is habitually used (over 65%) in diabetes, obesity and other metabolic disorder studies in rodents.43,44
In our study, all animals gained weight throughout the experiment, this gain being greater in animals fed a fat diet than standard food; no significant differences in weight gain were recorded among the three drinking groups within the same diet. However, food and drink intake was lower in the fat diet groups, with the animals that drank water consuming the most food and drink. A lower intake in mice fed a fat chow compared to those on a standard diet has been previously reported.43
Epidemiological studies in humans have indicated that moderate alcohol consumption may reduce mortality,3,45 whereas diets rich in saturated fats are associated with higher mortality.46 Here, the survival rate did not show significant differences between groups, although it was lower on the fat diet than on the standard diet.
Our analysis showed few noticeable differences in blood biochemical metabolites between the different groups. At 18 months, in the groups on the standard diet, statistically higher triglyceride values were recorded in the female mice that drank water than in those that drank wine. In the fat diet group, cholesterol values were statistically higher in the group that drank ethanol compared to the water group at 9 months. However, all these values were within normal limits for female CD1 mice.32
Glucose homeostasis was assessed by the glucose tolerance test (GTT), the most commonly used method in rodents,28 at 6 months. No significant differences were recorded among any of the three drinking groups under a fat diet. Conversely, in the standard diet group, significantly (95%) better glucose metabolization was observed for the wine group in comparison with the water-drinking group, which may be indicative of a lower risk of diabetes. In this regard, recently, a prospective UK Biobank study found that moderate wine consumption, as part of Mediterranean dietary habits, was independently associated with lower type 2 diabetes incidence.47 Other studies have also found a U-shaped relationship between wine intake and reduced diabetes risk.48,49
Excessive alcohol consumption is also associated with a higher risk of liver cirrhosis.50–52 Here, analyses of serum metabolites at 9 and 18 months showed levels within the normal limits for female CD1 mice,32 and no remarkable differences for any of the liver enzymes analyzed (alkaline phosphatase, alanine transaminase, aspartate aminotransferase, and gamma-glutamyl transferase) were found among any of the drinking groups. Likewise, no differences were recorded for creatinine levels, an increase in which can be a sign of poor kidney function.53,54 Moreover, the histological liver analysis did not show notable differences among the three drinking groups with the same diet, the liver lesions being more frequent in female mice fed the fat diet. Similar results have been described in mice that drank a higher concentration (3.5% v/v) of alcohol than used here and followed a high-Fat diet.55
On the other hand, it is also known that the consumption of high-Fat foods alters the weight of the ovaries and the number of oocytes,56–59 which affects fertility and aging. Likewise, alcohol consumption can shorten the length of telomeres,60,61 which has implications for age-related diseases. Therefore, regarding ovarian weight, our results are consistent with previous studies57 that analyzed the effect of a fat diet on ovarian weight, but we did not find differences between groups, suggesting either no influence or an insufficient number of animals to detect it. However, we observed a significant effect on the number of germinal vesicle (GV) oocytes obtained when comparing animals that consumed wine and a fat diet with those that consumed alcohol on a standard or fat diet. Similarly, when analyzing telomere length in both oocytes and somatic cells obtained from the heart or the tail of mice, our results indicated that consuming the same amount of alcohol as wine or as pure ethanol produced markedly different effects only when the diet was high in fat. Pure ethanol significantly affected telomere length, whereas wine consumption showed no differences compared to the water-drinking group. Again, this effect was observed exclusively in groups subjected to a fat diet. The ability of moderate wine consumption to prevent the deleterious effects of alcohol consumption, specifically in fat diets, without affecting the weight of the mice, suggests that wine could be a key regulator of energy balance. This would also indicate that the effect of wine consumption might arise from a combination of interactions between fat diet effects and wine components. Further studies should investigate the molecular mechanisms underlying these interactions.
In conclusion, our data indicate that female mice in the fat-diet groups showed more frequent and severe liver lesions, as well as a lower average ovarian weight. Moreover, moderate and prolonged ethanol consumption significantly affected telomere length only when the diet included fat, whereas red wine consumption showed no difference compared to water consumption, pointing to a possible predominant role of the polyphenols present in wine. Beneficial effects of wine polyphenols on human health have been widely described, as their ability to produce anti-inflammatory, anti-carcinogenic and cardio-protective effects, among others, is well known.7,62–64 On the other hand, at the end of the experiment, mice on the fat diet that drank wine had more oocytes than those in the ethanol groups, regardless of whether their diet was standard or fat. Finally, a positive effect on glucose metabolism was observed in mice in the wine-drinking group. Thus, overall, our data suggest that long-term moderate red wine consumption does not substantially influence the health of female mice, regardless of whether the diet supplied is standard or high in fat.
Author contributions
Conceptualization: E. G.-M., A. G.-A. and J.-C. S. Methodology: E. G.-M., M. A. M.-A., A. G.-A. and J.-C. S. Investigation and validation: A.-B. B., N. J. O., A. E., S. M., A. C., P. J. S.-C. and E. P. Formal analysis: P. J. S.-C., A.-B. B., M. A. M.-A. and A. G.-A. Visualization: E. G.-M., A.-B. B., A. G.-A. and J.-C. S. Funding acquisition: E. G.-M. Project administration: E. G.-M. and M. A M.-A. Supervision: E. G.-M., A. G.-A. and J.-C. S. Writing – original draft: E. G.-M. and J.-C. S. Writing – review & editing: E. G.-M., A.-B. B., A. G.-A. and J.-C. S.
Data availability
Data are available upon request.
Conflicts of interest
The authors have no competing interest related to the presented results.
Acknowledgements
This work was supported by the Ministero dell'Agricoltura, della Sovranità Alimentare e delle Foreste, MASAF, Italy (D.D MASAF prot. 0338013, 28/06/2023). The funders had no role in the study design, data collection and interpretation, or the decision to submit the work for publication.
References
- J. Ferrieres, The French paradox: lessons for other countries, Heart, 2004, 90, 107–111 CrossRef.
- I. Fernandes, R. Perez-Gregorio, S. Soares, N. Mateus and V. de Freitas, Wine Flavonoids in Health and Disease Prevention, Molecules, 2017, 22(2), 292 CrossRef.
- E. B. Rimm, A. Klatsky, D. Grobbee and M. J. Stampfer, Review of moderate alcohol consumption and reduced risk of coronary heart disease: is the effect due to beer, wine, or spirits, Br. Med. J., 1996, 312, 731–736 CrossRef CAS PubMed.
- L. Snopek, J. Mlcek, L. Sochorova, M. Baron, I. Hlavacova, T. Jurikova, R. Kizek, E. Sedlackova and J. Sochor, Contribution of Red Wine Consumption to Human Health Protection, Molecules, 2018, 23(7), 1684 CrossRef.
- A. S. St Leger, A. L. Cochrane and F. Moore, Factors associated with cardiac mortality in developed countries with particular reference to the consumption of wine, Lancet, 1979, 1, 1017–1020 CrossRef CAS.
- H. Cory, S. Passarelli, J. Szeto, M. Tamez and J. Mattei, The Role of Polyphenols in Human Health and Food Systems: A Mini-Review, Front. Nutr., 2018, 5, 87 CrossRef PubMed.
- I. Buljeta, A. Pichler, J. Simunovic and M. Kopjar, Beneficial Effects of Red Wine Polyphenols on Human Health: Comprehensive Review, Curr. Issues Mol. Biol., 2023, 45, 782–798 CrossRef CAS.
- B. Gao and R. Bataller, Alcoholic liver disease: pathogenesis and new therapeutic targets, Gastroenterology, 2011, 141, 1572–1585 CrossRef CAS PubMed.
- R. Room, T. Babor and J. Rehm, Alcohol and public health, Lancet, 2005, 365, 519–530 Search PubMed.
- D. Sridhar, Health policy: Regulate alcohol for global health, Nature, 2012, 482, 302 CrossRef CAS.
- I. R. Whitman, V. Agarwal, G. Nah, J. W. Dukes, E. Vittinghoff, T. A. Dewland and G. M. Marcus, Alcohol Abuse and Cardiac Disease, J. Am. Coll. Cardiol., 2017, 69, 13–24 CrossRef CAS.
- H. Rumgay, K. Shield, H. Charvat, P. Ferrari, B. Sornpaisarn, I. Obot, F. Islami, V. Lemmens, J. Rehm and I. Soerjomataram, Global burden of cancer in 2020 attributable to alcohol consumption: a population-based study, Lancet Oncol., 2021, 22, 1071–1080 CrossRef.
- R. Burton and N. Sheron, Complex relationship between health and moderate alcohol use, Lancet, 2022, 400, 141–143 CrossRef.
- G. B. D. Alcohol Collaborators, Population-level risks of alcohol consumption by amount, geography, age, sex, and year: a systematic analysis for the Global Burden of Disease Study 2020, Lancet, 2022, 400, 185–235 CrossRef.
-
https://cancer-code-europe.iarc.fr/index.php/en/
, accessed January 2025.
- M. Roerecke, On bias in alcohol epidemiology and the search for the perfect study, Addiction, 2017, 112, 217–218 CrossRef PubMed.
- J. D. Wallach, S. Serghiou, L. Chu, A. C. Egilman, V. Vasiliou, J. S. Ross and J. P. A. Ioannidis, Evaluation of confounding in epidemiologic studies assessing alcohol consumption on the risk of ischemic heart disease, BMC Med. Res. Methodol., 2020, 20, 64 CrossRef PubMed.
- S. Ghosh Dastidar, J. B. Warner, D. R. Warner, C. J. McClain and I. A. Kirpich, Rodent Models of Alcoholic Liver Disease: Role of Binge Ethanol Administration, Biomolecules, 2018, 8(1), 3 CrossRef PubMed.
- W. C. Griffin 3rd, Alcohol dependence and free-choice drinking in mice, Alcohol, 2014, 48, 287–293 CrossRef.
- A. Lamas-Paz, F. Hao, L. J. Nelson, M. T. Vazquez, S. Canals, M. Gomez Del Moral, E. Martinez-Naves, Y. A. Nevzorova and F. J. Cubero, Alcoholic liver disease: Utility of animal models, World J. Gastroenterol., 2018, 24, 5063–5075 CrossRef PubMed.
- Q. He, Y. Diao, T. Zhao, B. Hou, L. D. Ngokana, H. Liang, J. Nie, P. Tan, H. Huang, Y. Li, L. Qi, Y. Zhao, Y. Liu, X. Gao and L. Zhou, SREBP1c mediates the effect of acetaldehyde on Cidea expression in Alcoholic fatty liver Mice, Sci. Rep., 2018, 8, 1200 CrossRef.
- H. Liang, H. Huang, P. Z. Tan, Y. Liu, J. H. Nie, Y. T. Zhang, K. L. Zhang, Y. Diao, Q. He, B. Y. Hou, T. T. Zhao, Y. Z. Li, G. X. Lv, K. Y. Lee, X. Gao and L. Y. Zhou, Effect of iron on cholesterol 7alpha-hydroxylase expression in alcohol-induced hepatic steatosis in mice, J. Lipid Res., 2017, 58, 1548–1560 CrossRef PubMed.
- P. Tan, H. Liang, J. Nie, Y. Diao, Q. He, B. Hou, T. Zhao, H. Huang, Y. Li, X. Gao, L. Zhou and Y. Liu, Establishment of an alcoholic fatty liver disease model in mice, Am. J. Drug Alcohol Abuse, 2017, 43, 61–68 CrossRef PubMed.
- S. Dutta and P. Sengupta, Men and mice: Relating their ages, Life Sci., 2016, 152, 244–248 CrossRef CAS PubMed.
-
https://www.oiv.int/standards/compendium-of-international-methods-of-wine-and-must-analysis
, accessed January 2025.
- Y. Carmona-Jimenez, M. V. Garcia-Moreno, J. M. Igartuburu and C. Garcia Barroso, Simplification of the DPPH assay for estimating the antioxidant activity of wine and wine by-products, Food Chem., 2014, 165, 198–204 CrossRef CAS PubMed.
- S. Motta, M. Guaita, C. Cassino and A. Bosso, Relationship between polyphenolic content, antioxidant properties and oxygen consumption rate of different tannins in a model wine solution, Food Chem., 2020, 313, 126045 CrossRef.
- J. E. Bowe, Z. J. Franklin, A. C. Hauge-Evans, A. J. King, S. J. Persaud and P. M. Jones, Metabolic phenotyping guidelines: assessing glucose homeostasis in rodent models, J. Endocrinol., 2014, 222, G13–G25 CAS.
- P. Ramos-Ibeas, E. Pericuesta, I. Peral-Sanchez, S. Heras, R. Laguna-Barraza, S. Perez-Cerezales and A. Gutierrez-Adan, Longitudinal analysis of somatic and germ-cell telomere dynamics in outbred mice, Mol. Reprod. Dev., 2019, 86, 1033–1043 CrossRef CAS.
- C. de Frutos, A. P. Lopez-Cardona, N. Fonseca Balvis, R. Laguna-Barraza, D. Rizos, A. Gutierrez-Adan and P. Bermejo-Alvarez, Spermatozoa telomeres determine telomere length in early embryos and offspring, Reproduction, 2016, 151, 1–7 Search PubMed.
- T. D. Schmittgen and K. J. Livak, Analyzing real-time PCR data by the comparative C(T) method, Nat. Protoc., 2008, 3, 1101–1108 CrossRef PubMed.
-
https://www.criver.com
, accessed January 2025.
- I. Corkovic, A. Pichler, J. Simunovic and M. Kopjar, A Comprehensive Review on Polyphenols of White Wine: Impact on Wine Quality and Potential Health Benefits, Molecules, 2024, 29(21), 5074 CrossRef PubMed.
- D. O. Baliunas, B. J. Taylor, H. Irving, M. Roerecke, J. Patra, S. Mohapatra and J. Rehm, Alcohol as a risk factor for type 2 diabetes: A systematic review and meta-analysis, Diabetes Care, 2009, 32, 2123–2132 CrossRef PubMed.
- X. H. Li, F. F. Yu, Y. H. Zhou and J. He, Association between alcohol consumption and the risk of incident type 2 diabetes: a systematic review and dose-response meta-analysis, Am. J. Clin. Nutr., 2016, 103, 818–829 CrossRef PubMed.
- M. J. Stampfer, G. A. Colditz, W. C. Willett, J. E. Manson, R. A. Arky, C. H. Hennekens and F. E. Speizer, A prospective study of moderate alcohol drinking and risk of diabetes in women, Am. J. Epidemiol., 1988, 128, 549–558 CrossRef PubMed.
- V. Vanhooren and C. Libert, The mouse as a model organism in aging research: usefulness, pitfalls and possibilities, Ageing Res. Rev., 2013, 12, 8–21 CrossRef PubMed.
- R. P. Barnes, E. Fouquerel and P. L. Opresko, The impact of oxidative DNA damage and stress on telomere homeostasis, Mech. Ageing Dev., 2019, 177, 37–45 CrossRef PubMed.
- M. Dehghan, A. Mente, X. Zhang, S. Swaminathan, W. Li, V. Mohan, R. Iqbal, R. Kumar, E. Wentzel-Viljoen, A. Rosengren, L. I. Amma, A. Avezum, J. Chifamba, R. Diaz, R. Khatib, S. Lear, P. Lopez-Jaramillo, X. Liu, R. Gupta, N. Mohammadifard, N. Gao, A. Oguz, A. S. Ramli, P. Seron, Y. Sun, A. Szuba, L. Tsolekile, A. Wielgosz, R. Yusuf, A. Hussein Yusufali, K. K. Teo, S. Rangarajan, G. Dagenais, S. I. Bangdiwala, S. Islam, S. S. Anand, S. Yusuf and Prospective Urban Rural Epidemiology study investigators, Associations of fats and carbohydrate intake with cardiovascular disease and mortality in 18 countries from five continents (PURE): a prospective cohort study, Lancet, 2017, 390, 2050–2062 CrossRef.
- T. M. Powell-Wiley, P. Poirier, L. E. Burke, J. P. Despres, P. Gordon-Larsen, C. J. Lavie, S. A. Lear, C. E. Ndumele, I. J. Neeland, P. Sanders, M. P. St-Onge and American Heart Association Council on Lifestyle and Cardiometabolic Health; Council on Cardiovascular and Stroke Nursing; Council on Clinical Cardiology; Council on Epidemiology and Prevention; and Stroke Council, Obesity and Cardiovascular Disease: A Scientific Statement From the American Heart Association, Circulation, 2021, 143, e984–e1010 CrossRef.
- F. M. Sacks, A. H. Lichtenstein, J. H. Y. Wu, L. J. Appel, M. A. Creager, P. M. Kris-Etherton, M. Miller, E. B. Rimm, L. L. Rudel, J. G. Robinson, N. J. Stone, L. V. Van Horn and American Heart Association, Dietary Fats and Cardiovascular Disease: A Presidential Advisory From the American Heart Association, Circulation, 2017, 136, e1–e23 CrossRef.
- A. G. Liu, N. A. Ford, F. B. Hu, K. M. Zelman, D. Mozaffarian and P. M. Kris-Etherton, A healthy approach to dietary fats: understanding the science and taking action to reduce consumer confusion, Nutr. J., 2017, 16, 53 CrossRef PubMed.
- M. Piirsalu, E. Taalberg, M. Jayaram, K. Lillevali, M. Zilmer and E. Vasar, Impact of a High-Fat Diet on the Metabolomics Profile of 129S6 and C57BL6 Mouse Strains, Int. J. Mol. Sci., 2022, 23(19), 11682 CrossRef PubMed.
- J. R. Speakman, Use of high-fat diets to study rodent obesity as a model of human obesity, Int. J. Obes., 2019, 43, 1491–1492 CrossRef PubMed.
- A. Di Castelnuovo, S. Costanzo, V. Bagnardi, M. B. Donati, L. Iacoviello and G. de Gaetano, Alcohol dosing and total mortality in men and women: an updated meta-analysis of 34 prospective studies, Arch. Intern. Med., 2006, 166, 2437–2445 CrossRef.
- Y. Kim, Y. Je and E. L. Giovannucci, Association between dietary fat intake and mortality from all-causes, cardiovascular disease, and cancer: A systematic review and meta-analysis of prospective cohort studies, Clin. Nutr., 2021, 40, 1060–1070 CrossRef PubMed.
- J. Maroto-Rodriguez, R. Ortola, A. Carballo-Casla, V. Iriarte-Campo, M. A. Salinero-Fort, F. Rodriguez-Artalejo and M. Sotos-Prieto, Association between a mediterranean lifestyle and Type 2 diabetes incidence: a prospective UK biobank study, Cardiovasc. Diabetol., 2023, 22, 271 CrossRef.
- J. Huang, X. Wang and Y. Zhang, Specific types of alcoholic beverage consumption and risk of type 2 diabetes: A systematic review and meta-analysis, J. Diabetes Invest., 2017, 8, 56–68 CrossRef.
- H. Ma, X. Wang, X. Li, Y. Heianza and L. Qi, Moderate alcohol drinking with meals is related to lower incidence of type 2 diabetes, Am. J. Clin. Nutr., 2022, 116, 1507–1514 CrossRef.
- D. Q. Huang, P. Mathurin, H. Cortez-Pinto and R. Loomba, Global epidemiology of alcohol-associated cirrhosis and HCC: trends, projections and risk factors, Nat. Rev. Gastroenterol. Hepatol., 2023, 20, 37–49 CrossRef PubMed.
- B. Mackowiak, Y. Fu, L. Maccioni and B. Gao, Alcohol-associated liver disease, J. Clin. Invest., 2024, 134(3), 176345 CrossRef.
- M. Roerecke, A. Vafaei, O. S. M. Hasan, B. R. Chrystoja, M. Cruz, R. Lee, M. G. Neuman and J. Rehm, Alcohol Consumption and Risk of Liver Cirrhosis: A Systematic Review and Meta-Analysis, Am. J. Gastroenterol., 2019, 114, 1574–1586 CrossRef.
- Z. V. Varga, C. Matyas, J. Paloczi and P. Pacher, Alcohol Misuse and Kidney Injury: Epidemiological Evidence and Potential Mechanisms, Alcohol Res.: Curr. Rev., 2017, 38, 283–288 Search PubMed.
- H. C. Yuan, Q. T. Yu, H. Bai, H. Z. Xu, P. Gu and L. Y. Chen, Alcohol intake and the risk of chronic kidney disease: results from a systematic review and dose-response meta-analysis, Eur. J. Clin. Nutr., 2021, 75, 1555–1567 CrossRef.
- Y. Diao, J. Nie, P. Tan, Y. Zhao, T. Zhao, J. Tu, H. Ji, Y. Cao, Z. Wu, H. Liang, H. Huang, Y. Li, X. Gao and L. Zhou, Long-term low-dose ethanol intake improves healthspan and resists high-fat diet-induced obesity in mice, Aging, 2020, 12, 13128–13146 CrossRef PubMed.
- F. Gonnella, F. Konstantinidou, C. Di Berardino, G. Capacchietti, A. Peserico, V. Russo, B. Barboni, L. Stuppia and V. Gatta, A Systematic Review of the Effects of High-Fat Diet Exposure on Oocyte and Follicular Quality: A Molecular Point of View, Int. J. Mol. Sci., 2022, 23(16), 8890 CrossRef PubMed.
- G. Hilal, T. Fatma, Y. Ferruh, G. Sabire and A. Yuksel, Effect of high-fat diet on the various morphological parameters of the ovary, Anat. Cell Biol., 2020, 53, 58–67 CrossRef PubMed.
- C. Johnson, L. Zhu, R. Mangalindan, J. Whitson, M. Sweetwyne, A. P. Valencia, D. J. Marcinek, P. Rabinovitch and W. Ladiges, Older-aged C57BL/6 mice fed a diet high in saturated fat and sucrose for ten months show decreased resilience to aging, Aging Pathobiol. Ther., 2023, 5, 101–106 Search PubMed.
- D. G. Le Couteur, D. Raubenheimer, S. Solon-Biet, R. de Cabo and S. J. Simpson, Does diet influence aging? Evidence from animal studies, J. Intern. Med., 2024, 295, 400–415 CrossRef PubMed.
- T. Harpaz, H. Abumock, E. Beery, Y. Edel, M. Lahav, U. Rozovski and O. Uziel, The Effect of Ethanol on Telomere Dynamics and Regulation in Human Cells, Cells, 2018, 7(10), 169 CrossRef.
- A. Topiwala, B. Taschler, K. P. Ebmeier, S. Smith, H. Zhou, D. F. Levey, V. Codd, N. J. Samani, J. Gelernter, T. E. Nichols and S. Burgess, Alcohol consumption and telomere length: Mendelian randomization clarifies alcohol's effects, Mol. Psychiatry, 2022, 27, 4001–4008 CrossRef.
- M. Martinez-Huelamo, J. Rodriguez-Morato, A. Boronat and R. de la Torre, Modulation of Nrf2 by Olive Oil and Wine Polyphenols and Neuroprotection, Antioxidants, 2017, 6(4), 73 CrossRef.
- H. M. Salazar, R. de Deus Mendonca, M. Laclaustra, B. Moreno-Franco, A. Akesson, P. Guallar-Castillon and C. Donat-Vargas, The intake of flavonoids, stilbenes, and tyrosols, mainly consumed through red wine and virgin olive oil, is associated with lower carotid and femoral subclinical atherosclerosis and coronary calcium, Eur. J. Nutr., 2022, 61, 2697–2709 CrossRef.
- I. Tedesco, M. Russo, P. Russo, G. Iacomino, G. L. Russo, A. Carraturo, C. Faruolo, L. Moio and R. Palumbo, Antioxidant effect of red wine polyphenols on red blood cells, J. Nutr. Biochem., 2000, 11, 114–119 CrossRef.
|
This journal is © The Royal Society of Chemistry 2025 |
Click here to see how this site uses Cookies. View our privacy policy here.