Closing the loop: exploring apple pomace as a source of bioactive compounds in the framework of circular economy
Received
7th June 2024
, Accepted 30th September 2024
First published on 29th October 2024
Abstract
Apple pomace (AP), the common designation of bio-residues generated during apple processing, holds a vast potential for alternative added-value solutions, particularly by applying new sustainable technologies in the food sector. This review provides an overview of the scientific validation of AP as a suitable source of starting materials for different competitive applications, compatible with circular economy guidelines and contributing to raising awareness about the impact and advantages of reincorporating bio-residues into the supply chain. The losses of the apple production chain, strategies to recover bio-residues, main nutritional and bioactive components, and innovative and eco-friendly technologies used for their extraction and subsequent use are thoroughly characterized. Additionally, a general perspective on the AP's bioavailability compounds will be presented, focusing on the association among nutrients in food and their physiological use.
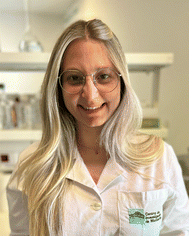 Liege Aguiar Pascoalino | She has a Master degree in Chemical Engineering, integrated with the Dual Diploma project between the Federal Technological University of Paraná, Brazil (UTFPR) and the Polytechnic Institute of Bragança (IPB), Portugal. Currently, she is a PhD student in Pharmaceutical Sciences at the Faculty of Pharmacy of the University of Porto (FFUP) and IPB, with a research grant from the Foundation for Science and Technology (FCT, Portugal). Her research focuses on recovering and valorising bio-residues from the cider industry, specifically apple pomace. |
 Lillian Barros | She is a Principal Researcher at Centro de Investigação de Montanha (CIMO), Instituto Politécnico de Bragança, vice-coordinator of CIMO, and vice-director of the Associate Laboratory SusTEC. She obtained a PhD “Doctor Europeus” in Pharmacy from the University of Salamanca (2008). She has published over 780 indexed papers in the Food Science and Technology area and has an 84 h-index. She has edited several books and book chapters, has registered national and international patents, and is the principal researcher of several national/international projects. She has received awards from different organizations and is a Highly Cited Researcher in Clarivate since 2016. Research targets: identification, separation, and recovery of functional molecules from natural products. |
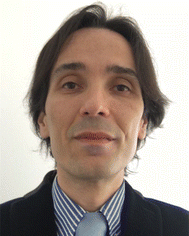 João C. M. Barreira | He has a PhD in Pharmaceutical Sciences, and is a Researcher at Mountain Research Center (CIMO – group: Sustainable Processes and Products), and is author of 139 papers (8734 citations, 219 co-authors, h-index 53), 1 scientific book and 11 scientific book chapters (7 as first author), and 19 pedagogic manuals (first degree and MSc levels). He is a Senior Editor of Food Chemistry X journal, Editor of 5 scientific books, Lead Guest Editor of 4 special issues and 2 topical collections in ISI-indexed peer-reviewed journals, Editorial Board Member in 8 ISI-indexed peer-reviewed journals, and Member of Scientific and Technical Support EFSA panel on Novel Foods & Nutrient Sources. SCOPUS: http://www.scopus.com/authid/detail.uri?authorId=54895546900. |
 M. Beatriz P. P. Oliveira | Beatriz Oliveira is Full Professor from FFUP, Director of Master in Quality Control, a member of the EFSA Scientific Network for Risk Assessment of GMOs, and a member of a specialized committee from ASAE. She is the Head of GRESA/REQUIMTE/LAQV research group. She has large experience in supervision (45 PhD; 108 MSc), has co-authored more than 520 papers in international journals, 56 book chapters, 70 proceedings, and 1450 communications in meetings, and has 2 granted patents by Europe and USA and 7 patents in evaluation. The developed work includes food science, security, environmental sustainability and plant extracts and agro-industrial by-products to add value. |
 Filipa S. Reis | She has a PhD in Pharmacy, with European mention and the highest honours, from the Complutense University of Madrid (UCM; 2017). Currently, she is a Researcher at Centro de Investigação de Montanha (CIMO group: Sustainable Processes and Products), FSR participates(d) in different R&D projects. She has published over 44 documents, including 4 international book chapters, 1 editorial, and 9 publications in proceedings of scientific meetings (h-index of 23). She is an Editorial Board Member of BioMed Research International (section Biochemistry, Hindawi) and Frontiers in Nutrition (section Food Chemistry), and Guest Editor of some Special Issues (Applied Sciences, Processes, and Separations, MDPI). |
Sustainability spotlight
This review highlights the potential of apple pomace (AP), a bio-residue from apple processing, emphasizing its transformation into valuable materials through innovative and eco-friendly technologies. It aims to underscore the importance of reusing AP to reduce the economic and ecological impact its generation entails. Therefore, a solution is presented for companies, allowing the implementation of circular economy measures. The advance lies in validating AP as a source of nutritional and bioactive compounds, contributing to waste reduction and resource efficiency. Reintegrating AP into the supply chain, this work aligns with UN SDGs 2 (Zero Hunger), 3 (Good Health and Well-being), 12 (Responsible Consumption and Production), and 13 (Climate Action), promoting sustainable practices and reducing the biological footprint of food processing industries.
|
Introduction
Roughly a third of the global food production is wasted or lost annually; fruit and vegetable residues concentrate 22% of that volume, resulting in losses of about $490 billion annually.1,2 Unexpectedly, if food leftover constituted a nation, it would rank as the third-largest producer of carbon dioxide, trailing behind the United States and China.3 According to the European Commission, food-related challenges, such as safety concerns and waste management, are among the most critical issues of the era, and food waste and loss were highlighted as one goal of the European Circular Economic Strategy.4 Due to this concern, the United Nations Sustainable Development Goal 12.3 was implemented,5 in which member states are committed to reducing worldwide food waste per person by 2030, diminishing food waste across production and supply chains at both retail and consumer stages.
Diminishing food waste is a pathway to lower production costs, enhance food nutrition and security, and assist in environmental sustainability. It remarkably eases the strain on natural resources and decreases gas emissions.2
Due to expected impacts on the environment, economic losses, and ineffective use of resources, reducing food waste and losses has garnered the attention of manifold latest studies. In this context, the priority is identifying bio-residue production dynamics and developing tools to prevent this generation. In this way, managing inevitable losses by employing strategies such as reuse is possible. Furthermore, a considerable diversity of bio-residues has been demonstrated to be valuable for recovering compounds with high added value, mainly bioactive ones.6–9 It is crucial to discover sustainable solutions so they only end up being considered waste after being entirely utilized.
This review will explore the potential of using bio-residues from the apple production chain through a circular economy idea to extract high-added-value compounds with bioactive potential. It will also consider the issues of applying green and eco-friendly extraction methods and the compound's potential in diverse food applications and health benefits.
Losses and reuses along the apple value chain
Apple (Malus domestica Borkh.) is one of the preferred fruits globally not just for its flavor, but also its nutritional value. Besides being consumed as fresh fruit, apple is an essential raw material in the fruit industry, especially for producing apple juice concentrate and cider.10,11 According to an FAO report, the global apple production in 2022 was estimated to be 113.8 million tons.12 Moreover, the annual apple production in the European Union (EU-27) over the last ten years reached its highest point in 2018/19 at around 14.8 million tons and currently is approximately 12.2 million tons.13
With the development of the apple processing industry, the disposal of residues generated during fruit processing, known as apple pomace (AP), is a fundamental issue of high priority. The demand for green apple production and, simultaneously, the destination of its bio-residues aiming to contribute to the circular economy (CE), and maintaining quality and safety are current challenging responsibilities.14–16 Indeed, the flaws begin in apple orchards, where the lack of sustainable practices generates many bio-residues that are discarded and, therefore, gives rise to several losses, including bioactive compounds that could be found in high concentrations and could be valued.10,14 Consequently, it is fundamental to develop better production management, including the CE system guidelines in apple cultivars, combining economic, environmental, and social issues with fabrication, thus promoting profit for companies and stakeholders.14,16
The typical makeup of apple pomace (AP) consists of 93% to 97% skin/flesh, 2% to 4% seeds, and 1% of stems, and its nutritional composition encompasses 80% to 85% moisture, 3% to 8% fat, 0.6% to 4% ash, 2% to 7% proteins, and 15% to 19% of total fibers (Fig. 1).15,17,18 Mostly, apple peels are an abundant font of dietary fiber, while seeds are generally high in bioactive lipids and polyphenols.19 Therefore, AP encompasses a diverse array of healthy compounds including phenolics, glycosides, alkaloids, volatile compounds, carbohydrates – particularly hemicellulose (4–24%), cellulose (7–44%), and pectin-dietary fibers, lipids, and proteins.8,15 Dietary fiber in AP generally consists of a balanced content of insoluble and soluble fibers and represents high-grade components related to cereals.20 Studies highlight the potential of AP to fortify and enhance the nutritional value of foods, particularly due to its rich fiber composition. The total dietary fiber content in AP ranges from 45% to 51%, with both soluble and insoluble fractions, as previously described.8,18 AP has been successfully incorporated into cookies,21 muffins,22 and even beef burgers23 to boost their fiber content, showcasing its versatility as a fiber-rich ingredient. With the well-documented health benefits of dietary fibers, utilizing AP in food products offers a sustainable and efficient approach to enhancing human health.8 In addition to indigestible fiber, AP also holds a substantial quantity of sugar and starch, rendering it an ideal substrate for fermentation products.24 Queji et al. (2010) assessed the sugar profile in AP from 26 apple varieties with 18–31% fructose, 2.5–12.4% glucose, and 3.4–24% sucrose per dry weight.25 Other studies determined that almost 90% of the total fat in AP consists of unsaturated fatty acids, predominantly oleic and linoleic acids.26,27
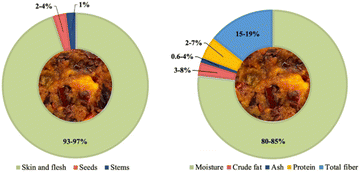 |
| Fig. 1 Graphical representation of (a) the morphological constitution and (b) the nutritional profile of apple pomace. | |
The leading end of AP is being employed in the manufacturing of animal feed. However, due to its small protein content, it does not constitute premium-quality feed, and there is a need to use the various compounds with the high added value present in pomace.28,29 Therefore, extracting nutrients and bioactive compounds from AP is crucial due to their health-related, environmental, and commercial benefits.
The circular economy and bio-residue recycling strategies
The concept of CE suggests a method contrasting to the conventional linear economy, where resources are removed, transformed into products, and then discarded after utilization. The closed systems have been considered the foremost foundation for eco-innovation, targeting at a “zero waste” economy and society where residues are employed as input for novel applications and products, a basis of industrial symbiosis.30,31
Clean and circular production is considered a tactical component in manufacturing technology for current and upcoming products across various industrial sectors. Demand is concentrated on the advancement of alternative methodologies for the reduction of waste, the optimization of processes, and improvement in production effectiveness.28,32 Based on these concerns, the comprehensive idea of food production, exhibited in Fig. 2a, has been formulated. This approach aims to align synergistic objectives, such as the utmost product safety and quality, maximizing production efficiency, and incorporating environmental considerations into both food production and product development. In the concept, all factors and aspects should be considered coherently.32
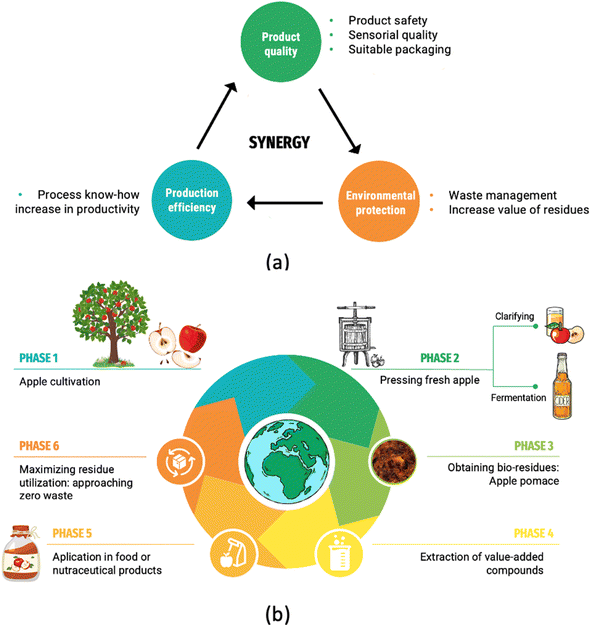 |
| Fig. 2 (a) The comprehensive concept of food production; (b) schematic representation of the apple production chain following the circular economy guidelines. | |
In terms of CE, valorization and recycling of residues allow materials to be returned to the supply chain, thus permitting economic development by reducing food losses.33,34 Implementing a CE model for the apple production chain can lead to greater sustainability since apple juice or cider manufacturing culminates in relatively low nutrient removal, resulting in apple pomace rich in nutrients of interest. Fig. 2b schematically shows an example of applying a CE approach in the apple production chain.
Wani & Mishra (2022b) studied a comprehensive CE model that manages controllable carbon emissions and degradation in an apple orchard, considering principles of a CE35 focused on minimizing the quantity of waste produced, reusing edible fruits, applying its by-products, and mixing closed loops to achieve maximum outputs with minimal inputs, and promoting a more sustainable environment.36
Establishing a closed-loop supply chain could be an ardor and essential portion of a CE aimed at waste reduction since apple orchards are increasing their popularity worldwide, and there has been a scarcity of mathematical modeling of these supply chains.36
Overall, the trends, growths, and impacts of implementing the circular economy vary worldwide. According to Mak et al. (2020), in growing nations, it is noticed that there is a notable increase in food loss during the stages immediately following harvest. On the other hand, developed economies experience a higher overall loss in the form of post-consumer food waste, affected by variables like aesthetics and inaccurate sell-by dates.37 The composition of wastage is affected by specific characteristics of individual countries, such as market access, culture, legislation, and climate. Hence, all these diverse factors must be considered when analyzing different scales, market sizes, and the likelihood of resource reallocations and product replacements connected with the application of circular economy concepts.31
Developing concepts to valorize bio-residues from agribusiness thus contributes to a circular economy by creating economic value from by-products. Apple bio-residues, rich in interesting phytochemical compounds, have the prospective to be applied in food products for the upscale market, making them healthier and adding value to the end product, in addition to increasing the income of the companies that generate these products through their valorization and reduction of the costs associated with their disposal. More than that, products and raw materials are kept in the economic cycle until they reach capacity, as the apple residues themselves are used as a bioactive source, increasing their usefulness. This strategy answers consumers' demands and the industry's search for new, cheaper, and natural ingredients for product improvement, besides promoting industrial competitiveness through the constant search for new ingredients and compounds of interest.
Recovery of compounds of interest from apple bio-residues
Studies have indicated that apple pomace (AP) contains phytonutrients that are valuable to one's health, particularly by decreasing the probability of illnesses including diabetes, cancer, and cardiovascular disease. The protective properties are believed to stem from the presence of nutrients and biologically effective compounds, such as fiber, vitamins, and phytochemicals.38
Nutrients.
Most of the dry weight of AP contains components not digestible by the human enzyme system, namely dietary fibers, which are classified into soluble and insoluble fibers. In AP, two-thirds of fibers are classified as insoluble, which include cellulose, hemicelluloses (galactomannan, xyloglucan, and glucuronarabinoxylan), and lignin (p-coumaryl alcohols, sinapyl, and polymerized coniferyl). It also contains a considerable amount of starch and sugars (fructose, glucose, sucrose), creating an ideal substrate for fermentation products; the remainder is soluble fiber, mainly pectin.15,39 Incorporating dietary fiber into food products is an excellent food improvement technique since it could help to avoid several health conditions, including coronary heart disease, obesity, colon cancer, and diabetes. Dietary fiber is also a valuable ingredient in healthy foods as it lowers calorie and cholesterol levels, besides having a prebiotic influence.40,41 This bio-residue is also abundant in antioxidants, triterpenoids, polyphenolic compounds, malic acid, vitamins C and E, proteins, amino acids, and essential minerals.39
Dry AP also comprises phosphorus, calcium, magnesium, and iron minerals. Additionally, about 90% of the total oil in AP is made up of unsaturated fatty acids, primarily oleic and linoleic acids. Phytochemicals such as chlorogenic acid, protocatechuic, and caffeic acid, as well as antioxidants and other polyphenols like quercetin, or phloridzin, have been extracted from AP.15,42 Thereby, the existence of value-added nutrients and phytochemicals in AP makes it a promising functional ingredient for various foods.
Bioactive compounds.
Thorough research indicated that AP serves as a beneficial origin of phenolic constituents. Likewise, it contains higher bioactive compound concentrations than apple flesh itself.43 The phenolic compounds within AP are predominantly phenolic acids (caffeic acid, hydroxycinnamic acids, and chlorogenic acid), flavonoids (catechins, quercetin, flavonol, epicatechin, dihydrochalcones, and phloridzin) and anthocyanins (cyanidin glycosides).44 Grigoras et al. (2013) assessed the bioactive molecules in AP and the primary phenols recognized were benzoic acids (gallic acid), flavonols (rutin), flavanols (catechin), hydroxycinnamic acids (chlorogenic acid), and chalcones (phloridzin). Of the triterpenes present, oleanolic and ursolic acids were the most prevalent. The authors concluded that for four cultivars (Pink Lady, Granny Smith, Gala, and Golden), crude extracts indicated potential antioxidant activity, validating that AP may be an esteemed font of bioactive molecules comprising not only triterpenes but also polyphenolic compounds.45
Waldbauer et al. (2016) also verified that ursolic acid was the primary triterpene in AP, with lower amounts of oleanolic, maslinic, and betulinic acids. They proposed that the health-promoting properties of bioactive compounds in AP could be attributed to increased vascular nitric oxide bioavailability, mediated by improved endothelial nitric oxide synthase (eNOS) activity. To identify the components of AP responsible for enhancing nitric oxide bioavailability through eNOS activation, the researchers found triterpenoid acids as the predominant constituents in the most active fractions, affirming the capability of AP to serve as a reservoir of bioactive compounds.46
Carpes et al. (2021) investigated the volatile compounds in AP and identified 56 volatiles belonging to different chemical classes: esters, terpenoids, alcohols/phenols, and ketones. α-Farnesene was the greatest common volatile compound, followed by sulcatone, ethyl phenyl propanoate, nonanal, and phenyl ethyl alcohol.47 The Italian AP was studied by Ricci et al. (2019), and they also described α-farnesene as the prevalent sesquiterpene, while sulcatone was the most plentiful ketone. Additionally, phenylethyl alcohol and nonanal were identified at great concentrations in AP of the Italian variety after fermentation with Lactobacillus rhamnosus.48
Madrera & Valles (2011) conducted a study to determine the presence of volatile compounds in AP. They identified various chemical groups, including 48 esters, 30 ketones and aldehydes, 19 norisoprenoids and terpenes, 9 acids, 8 alcohols, 4 lactones, and 6 compounds of further chemical structures. Their analysis of five different cultivars showed that these compounds' total number and proportion varied. However, the leading component within each chemical group remained consistent across all cultivars. For example, benzaldehyde was the dominant compound in the group of ketones and aldehydes, decanoic acid in the acids, a farnesol isomer in the group of terpenes, and ethyldecanoate in the group of lactones and esters. The only exception was the chemical group of alcohols, where the main compound differed among the five cultivars, with four of them having 1-octen-3-ol as the dominant compound, while one cultivar had 1-hexanol as the main compound.49Table 1 condenses the main bioactive compounds identified in apple pomace and discussed above.
Table 1 Comprehensive overview of primary bioactive compounds found in apple pomace
Main classification |
Examples |
Amount |
Ref. |
Insoluble fibers |
Cellulose, hemicelluloses (galactomannan, xyloglucan, and glucuronarabinoxylan), and lignin (p-coumaryl alcohols, sinapyl, and polymerized coniferyl) |
36.5–57.9% of TDF (total dietary fibers) |
15 and 39 |
Soluble fibers |
Pectin |
14.6–19.0% of TDF |
15 and 39 |
Sugars |
Fructose, glucose, and sucrose |
18–31%; 2.5–12.4%; 3.4–24%, respectively |
15 and 39 |
Minerals |
Phosphorus, magnesium, calcium, and iron |
0.07–0.076%; 0.02–0.036%; 0.06–0.1%; 31.8–38.8%, respectively |
15 and 42 |
Unsaturated fatty acids |
Linoleic and oleic acids |
90% of total oil in apple pomace |
15 and 42 |
Phenolic acids |
Caffeic acid, hydroxycinnamic acids, and chlorogenic acid |
In total: 523–1542 mg per kg per dry weight |
44 and 50 |
Flavonoids |
Quercetin, phloridzin, catechins, epicatechin, dihydrochalcones, and flavonol |
In total: 2153–3734 mg per kg per dry weight |
44 and 50 |
Anthocyanins |
Cyanidin glycosides |
In total: 50–130 mg per kg per dry weight |
44 and 50 |
Triterpenes |
Oleanolic acid, maslinic acid, and ursolic acid |
9.6–20.4; 14.6–17.4; 7.9–17.1 mg g−1 respectively |
45 and 46 |
Volatile compounds |
α-Farnesene, sulcatone, ethylphenyl propanoate, and nonanal |
26.6%; 8.3%; 5.7%; 5.5%; 5.4%, respectively |
47 and 48 |
Organic acids |
Malic and ascorbic acids |
4.9–13.2; 0.3–0.5 mg g−1, respectively |
39 and 51 |
Tocopherols |
α-Tocopherol |
4.4–13.9 mg mL−1 |
39 and 52 |
Health effect: bioavailability of apple bioactive compounds
Bioactivity is the action of the absorbed compounds or their metabolites in metabolic pathways, thus exerting biological effects on the body. Nevertheless, bioactive compounds (such as polyphenols) need to be bioavailable to exert their biological effects. Their bioavailability corresponds to normal metabolism's digested, absorbed, and utilized portion. It can change based on their bioaccessibility, which measures the percentage of a consumed compound that is liberated from the solid food matrix and its subsequent availability for uptake within the small intestine or biotransformation via the gut microbiota. Hence, the health effects of apple pomace consumption may be influenced by bioactive compounds' absorption, metabolism, and distribution.41
Bondonno et al. (2017) concluded that alterations in the health advantages of apples as a whole food compared with isolated main polyphenols might be due to variances in their bioavailability. Several elements impact how well a person can absorb and metabolize polyphenols, including the amount consumed, microbial composition, and the occurrence of additional polyphenols and macronutrients in the food matrix. For instance, simultaneous intake of polyphenols and dietary fiber can decrease their absorption in the small intestine but increase the amount that reaches the large intestine. Then, polyphenols could be degraded to phenolic acids, and both dietary fiber and polyphenols may enhance the microbial composition of the colon.41
Pollini et al. (2022) assessed the bioavailability and bioaccessibility in vitro of phenolic compounds extracted from freeze-dried apple pomace (phloridzin, chlorogenic and gallic acids). Their outcome demonstrated that chlorogenic acid had a more significant bioaccessibility (44%) than gallic acid (7%), and phloridzin (17%), with comparable values observed for both freeze-dried and fresh samples. More than that, solely chlorogenic acid showed upper bioavailability in basal compartments after 4 h, i.e., 56% of chlorogenic acid passed the transepithelial barrier.53
Researchers have investigated the bioavailability of epicatechin by utilizing both an apple extract (apple drink) and an apple puree holding varying concentrations of epicatechin. The authors concluded that both extracts are effective sources of bioavailable epicatechin. Nevertheless, epicatechin obtained from whole apples exhibits lower bioavailability compared to when it is consumed in the form of an epicatechin-rich apple extract incorporated into a beverage made with water. This finding supports the idea that the apple matrix diminishes epicatechin bioavailability.54
Innovative processes used to recover bioactive from bio-residues
Numerous extraction procedures have been suggested in the bibliography for the useful recovery of bio-residues, from conventional solid–liquid extraction to non-conventional techniques, to improve the extraction yield of targeted compounds, evading deterioration and reduction in functionality during processing.55 These non-conventional techniques comprise ultrasound-assisted extraction (UAE), microwave-assisted extraction (MAE), supercritical fluid-assisted extraction (SFE), pressurized liquid extraction (PLE), or enzyme-assisted extraction techniques.7,56–58Fig. 3 illustrates a schematic representation of these types of extraction, while Table 2 condenses the various extraction methods applied to recover different compounds of interest from apple bio-residues.
 |
| Fig. 3 Conventional and modern extraction procedures for plant-derived bio-residue recovery. | |
Table 2 Functional compounds recovered from bio-residues of the apple supply chain
Source |
Compounds of interest |
Recovery method |
Potential applications |
Recovery efficiency |
Ref. |
Apple pomace |
Protein enrichment |
Solid-state fermentation (Rhizopus oligosporus) |
Improving the nutritional value in food applications |
30.8% of soluble protein |
59
|
Lactic acid |
Enzymatic digestibility |
Fermentation of food and feed products |
High susceptibility to enzymatic hydrolysis: 80% of the total glucan was converted into glucose |
60
|
Polyphenols |
Supercritical (carbon dioxide + ethanol) extraction |
Natural antioxidants and functional foods |
Total phenolic content (TPC) equal to 0.47 gallic acid equiv. (GAE) per g |
61
|
Antioxidants and polyphenols |
Pressurized liquid extraction (PLE) |
Preservative of food products |
Antioxidant activity 2.4 times higher |
62
|
Flavonoids |
Microwave-assisted extraction (MAE) |
Food fortification |
62.7 mg GAE/100 g dry apple pomace |
63
|
Antioxidants |
Ultrasound-assisted extraction (UAE) |
Substitute of synthetic preservatives |
TPC 30% higher |
64
|
Flavonols |
Pressurized hot water extraction (PHWE) |
Cosmetic, pharmaceutical, and food industries |
Total yield of flavonols of 1.3 μmol g−1 dry AP |
65
|
Fermentable sugars, ethanol, and acetic acid |
Enzymatic hydrolysis and fermentation |
Cosmetic, pharmaceutical, and food industries |
Production of acetic acid at a concentration of 61.4 g/100 g |
66
|
Phenolic and terpenic compounds |
MAE |
Minimizing damage caused by oxidative stress due to radical species |
AP extracts obtained by successive liquid–liquid extraction were able to inhibit the DPPH free radical |
45
|
Pectin |
Subcritical water |
Gelatinization, thickening and stabilization |
Apple pomace pectin equal to 16.7% |
67
|
Phenolic compounds |
MAE |
Substitute to synthetic antioxidants, and dietary supplements |
TPC of 15.8 mg GAE per g |
68
|
Propionic acid |
Fermentation (Propionibacterium freudenreichii) |
Preservative of food products |
Propionic acid biosynthesis equal to 1.771 g L−1 |
69
|
Bound phenolics |
Pulsed electric field |
Enhancing the healthy properties of food products and antioxidant agent |
37.4% of TPC and 86% of antioxidant activity |
70
|
Antioxidants, phenolics and carotenoids |
Supercritical fluid extraction (SFE) |
Bakery, oil-based and cosmetic products |
Antioxidant activity equal to 5.6 mg TEA per g of extract |
71
|
Antioxidants, phenolics and carotenoids |
Warm solvent extraction (WSE) and ultrasound-assisted extraction (UAE) techniques |
Pharmaceutical, cosmetic, and food applications |
TPC equal to 25 mg GAE per g (WSE) and 29 mg GAE per g (UAE) |
72
|
Fermentable sugars and pectin |
Enzymatic hydrolysis |
Conversion in value-added biochemicals |
95.5 g pectin and 110.2 g fermentable sugars were produced from 500 g of AP |
73
|
Xylitol and 2G ethanol |
Acid hydrolysis and fermentation (Candida guilliermondii and Kluyveromyces marxianus) |
Elaboration of a biotechnological platform for the combined use of both the hemicellulosic and cellulosic fraction |
10.47 g L−1 of ethanol and 9.10 g L−1 of xylitol |
74
|
Phenolic compounds |
Pressurized liquids coupled on-line with solid phase extraction |
Food and pharmaceutical industry |
The solvent gradient, static time, and extraction temperature allowed higher mass transfer of the target analytes (furfurals, chlorogenic acids, flavonoids, and phloridzin) |
75
|
Apple pomace ultrafiltration sludge |
Citric acid |
Fermentation (Aspergillus niger) |
Preservative and flavoring in food and beverages |
Citric acid concentration of 44.9 g/100 g |
76
|
Biobutanol |
Fermentation (Clostridium beijerinckii) |
Potential source of renewable energy |
1.4 g L−1 of biobutanol |
77
|
Apple industry waste biomass |
Fumaric acid |
Fermentation (Rhizopus oryzae) |
Chemical building block for coating compounds, synthetic resins, and plasticizers |
25.2 g L−1 of fumaric acid |
78
|
Apple peel and pulp |
Catechins, flavonols (quercetin) and anthocyanins |
PLE |
Improving nutritional and organoleptic properties of foodstuffs |
Confirms the efficiency of PLE to extract polyphenols from AP |
79
|
Apple peels |
Phenolic compounds |
SFE |
Natural antioxidant |
Extractions from 15 g provided the highest phenolics yield of 800 mg/100 g dry peels |
80
|
Among the leading green extraction methodologies that replace conventional methods, supercritical fluid extraction with CO2 (SFE) and high-pressure solvent extraction (HPSE) currently stand out because of their high output and the production of high-quality goods in less processing time and waste.81 These two techniques will be detailed below.
Supercritical fluid extraction with CO2 (SFE)
Supercritical fluid extraction is mostly used in the food domain because it is safe and operated under easily achievable critical circumstances (31.1 °C and 73.8 bar). Furthermore, the lack of air and light through extraction minimizes the likelihood of degradation reactions occurring.61 This technique uses solvents above the critical point, which could be defined as “the specific pressure or temperature point above which gas and liquid do not exist as separate phases”. These fluids present the properties of both liquids in terms of diffusion, viscosity, and surface tension, as well as those of gases, allowing higher yields in a shorter time.82 Besides its lack of acute toxicity, CO2 is also colorless, highly pure, non-flammable, odorless, and easily removed from the extract after decompression stage.71
Nevertheless, the major limitation lies in its small polarity, as CO2 is non-polar and not appropriate as a solvent for polar compounds. The addition of organic co-solvents such as acetone, methanol, ethanol, or dichloromethane improves the solvating power of CO2 and the yield of compound extraction by minimizing the matrix–analyte interactions.61,83
Adil et al. (2007) studied the supercritical (ethanol and carbon dioxide) extraction of polyphenols from peach and apple pomaces and determined the antioxidant activities. The best pressure and temperature ranged between 55–57 MPa and 56–58 °C, respectively, with an ethanol concentration of 20% and an extraction time of 40 minutes for apple pomace. The antiradical efficiencies and the total phenolic contents of the apple pomace extracts obtained were higher than those processed by conventional ethanol extraction, demonstrating that more active polyphenols were selectively extracted.61
Ferrentino et al. (2018) compared the extraction of antioxidants from apple pomace, applying conventional extraction techniques and SFE. Lesser yields were achieved by SFE apple pomace processing. On the other hand, the antioxidant capacity and total phenol recovery were higher than the extracts obtained by conventional extraction techniques. Generally, the outcomes indicate that fewer, but more active polyphenols are selectively extracted, reinforcing the SFE potential as a green technology for recovering high-value compounds from apple pomace.71
Massias et al. (2015) accessed the recuperation of phenolics from apple peels utilizing ethanol and CO2 extraction. They concluded that this technology could recover the phenolics, yielding 120 mg from 15 g of peels using only 1.1 kg of extracting fluid.80
High-pressure solvent extraction (HPSE)
High-pressure solvent extraction is an appealing choice compared to conventional solid–liquid extraction methods, proposing several benefits in what concerns separation conditions, selectivity, and the use of environmentally friendly solvents and technology for food and pharmaceutical applications. This method, also recognized as pressurized solvent extraction (PSE) or pressurized liquid extraction (PLE), comprises the use of H2O or organic solvents at considerably raised pressures (3.3–20.3 MPa), and temperatures (313–473 K), enhancing the ability to conduct beneficial extractions due to its improved attributes related to solvation properties and mass transfer. Elevated temperatures lead to a decrease in liquid solvent viscosity, an enhancement in solvent diffusivity through the matrix, and therefore, an acceleration in extraction kinetics. Furthermore, high pressure can reduce the surface tension of the solvent, forcing it into the matrix pores and hence simplifying the extraction of target compounds.57,84,85 Thereby, this type of extraction exploits the useful mixture of liquids' solvation properties and the beneficial transport characteristics of supercritical fluids.86 The flexibility of pressurized solvents is fundamental due to the physicochemical properties of the solvent (viscosity, diffusivity, dielectric constant, and density), which could be measured by fluctuating the pressure and temperature of the system.87
Nonetheless, a liquid separation step is also mandatory in the post-extraction steps, which is a relative inconvenience to SFE methodology.84
Wijngaard & Brunton (2009) optimized the extraction of antioxidants (polyphenols) from apple pomace by pressurized liquids and concluded that when using this technique, the antioxidant capacity was improved 2.4 times in contrast to conventional extraction.62
da Silva L. C. et al. (2023) studied the simultaneous extraction of apple pomace by gradient PLE coupled in line with solid-phase extraction and online with HPLC. The authors concluded that utilizing an extraction solvent gradient could be an esteemed instrument to enhance the variety of compounds extracted in a single process rather than employing consecutive extractions with diverse solvents, enhancing the extraction yield and achieving quantitative extractions.75
Alonso-Salces et al. (2001) optimized the PLE extraction using diverse process variables (solvent, static time, temperature, and pressure) to increase a substantial recuperation of polyphenols from apple pulp and peel. Optimized conditions for the highest polyphenol recovery were defined: methanol at a temperature of 313 K and 7 MPa pressure for 5 min. Finally, the effectiveness of PLE as an eco-friendly technique for the extraction of polyphenols was similar to conventional solvent extraction, resulting in less solvent volume and shorter sample preparation times.79
Application of bio-residues from the apple supply chain in food and nutraceutical industries
Several efforts have been made to recover bioactive compounds embedded in apples' bio-residues, since they are a prospective source for multiple bioactive compounds, including antioxidants, coloring pigments, phytochemicals, and nutrients, with functional and nutritional values.88 Along these lines, the effective incorporation of these compounds into food and nutraceutical products and proper management of these organic bio-residues could supply economic and environmental advantages by decreasing food loss beyond the elaboration of new products, contributing to the competitiveness of the industries.
Accordingly, a brief overview will be presented on the major applications of apple bio-residues both in the food area and in nutraceuticals. According to different research reports, AP could have an extensive range of applications. Ayar et al. (2018), for instance, investigated the consequences of supplementing with dietary fiber-rich apple pomace to probiotic ice creams. They concluded that it can be implemented in ice cream with enhanced viability of probiotic strains without contrary consequences on the microbiological, physicochemical, and sensory properties. The enriched ice creams presented physicochemical and sensory properties similar to the control, besides having highly satisfactory sensory properties.40
The impact of apple pomace on the quality attributes of crackers was also researched by Mir et al. (2017). It was possible to assume the increased quantity of apple pomace in the flour preparation resulted in superior antioxidant capacities, total dietary fiber, and minerals in the end-product, considering that pomace-based rice crackers have beneficial effects for consumers, especially for coeliac disease patients.89
Rupasinghe et al. (2008) examined the impact of baking on the dietary fiber and phenolics of muffins combined with apple skin powder. They noticed that these muffins were higher in dietary fiber, total phenolic content, and total antioxidant activity than the control. Their results indicate that apple skin powder might serve as an unconventional font of dietary fiber or as a specialty ingredient for bakery items or specific nutraceuticals and functional foods.22 Following this line of reasoning, Wolfe & Liu (2003), in their review paper, stated that valuable food ingredients could be produced from apple peels by drying and grinding without much loss of phytochemicals. Based on fresh weight, these samples' flavonoids and total phenolic contents were comparable to those of fresh apple peels. Thus, the developed ingredient apple peel powder could be a rich and appealing supplement to healthy foods, as a slight amount can significantly enhance the content of phytochemicals and antioxidant capacity of foods.90
de Toledo N. M. V. et al. (2017) explored the sensory and physicochemical effects concerning the partial replacement of wheat flour in cookies with flours derived from apple bio-residues. The use of these bio-residues positively induced the nutritional attributes of the cookies, particularly their fiber content, deducing that they could be a possible alternative to be investigated for technological, nutritional, and sensory aims by the food industry.91 Usman et al. (2020) also investigated enriched cookies quality with apple pomace and based on the sensory and compositional characteristics, it was established that cookies with better-quality organoleptic properties could be made using wheat flour supplemented with 10% of apple pomace powder.21
Bortolini et al. (2020) tested a new approach using apple pomace in cider-making to recover phenolic compounds. The cider enriched with apple pomace was richer in phenolic compounds and, consequently, had higher antioxidant capacity. Also, the sensory analysis revealed lower intensities of sourness and higher intensities of bitterness, whereas the odor and astringency attributes were related to the control cider. The authors hypothesized that apple pomace demonstrated great potential as a source of phenolic compounds to be reintegrated into cider.92
Huber & Rupasinghe (2009) studied apple peel's attributes as natural antioxidants to solve the question of lipid oxidation, particularly the degradation of polyunsaturated fatty acids, a noteworthy problem in the industry affecting both consumers' health and food quality. The authors studied 21 apple genotypes and their profile of phenolic compounds and antioxidant capacities. The extracts were revealed to be potent inhibitors of the oxidation of polyunsaturated fatty acids. They thus could be pondered to develop new natural food antioxidants.93
Henríquez et al. (2010) created an ingredient from Granny Smith apple peel, utilizing a pilot-scale drying method. Every effort to optimize the conservation of dietary fiber and phenolic compounds was determined. Moreover, they scanned the chemical and physical characteristics, sugar and mineral content, and technological functional properties such as solubility index, water retention capacity, and dispersibility. The developed powder ingredient, a font of phenolic compounds and dietary fiber, was considered suitable for food formulations.94
Regarding the pharmaceutical industry, it has been proven that phenolic compounds found in apples hinder fat synthesis in sebaceous cells and also modulate sebum production, thereby diminishing the appearance of acne. In that regard, natural products have a tendency to treat acne, decreasing the inflammation systems.95,96 Moreover, phenolic compounds demonstrated results on different skin syndromes such as rosacea, psoriasis, wounds, atopic dermatitis, and burns.96
Apple pomace has gelling and emulsifying characteristics in the cosmetic, pharmaceutical, and food industries.97,98 Huc-Mathis et al. (2019) studied these apple pomace and oat bran properties. Their insoluble and soluble components stabilized oil-in-water emulsions, but the apple powder showed improved emulsifying potential due to the stabilizing pectins and proteins in the soluble portion. Both raw materials were regarded as renewable sources of stabilizers. Additionally, apple pomace can also serve as a reducing and stabilizing agent in the fabrication of metallic nanoparticles.99
Ren et al. (2019) used apple pomace to synthesize silver nanoparticles. The green production method avoided the use of toxic reducing and dispersing agents. The Ag nanoparticles had great antibacterial activity. Moreover, they exhibited a uniform dispersion, small size, spherical shape, and a high degree of crystallization.100
In that way, the use of apple pomace as a natural additive in the food industry is becoming gradually accepted as it seems to have several effective attributes, such as antioxidant, antimicrobial, and anti-inflammatory properties. Also, apple pomace presents a high potential to be exploited as a competitive and original source of cosmeceuticals, with possible application in developing numerous natural-based preparations while adding value to that industrial bio-residue.11Table 3 recapitulates all the applications cited in this topic.
Table 3 Outline of versatile applications of apple pomace in food and pharmaceutical industries
Area |
Application |
Amount applied |
Results |
Ref. |
Food industry |
Ice cream |
0.5% and 3% of apple pomace |
Improved viability of probiotic strains without any negative consequences |
40
|
Brown rice-based crackers |
3%, 6% and 9% of apple pomace |
Higher antioxidant properties, fibers and minerals |
89
|
Muffins |
4%, 8%, 16%, 24% and 32% of apple skin |
Higher in dietary fiber, total phenolic content, and total antioxidant capacity |
22
|
Apple peel powder as an ingredient to supplement healthy foods |
A small amount could greatly increase the phytochemical content and antioxidant activity of foods |
Increasing the antioxidant activity and content of phytochemicals |
90
|
Cookies |
5%, 10% and 15% of apple by-products; 5%, 10%, 15%, 20% and 25% of dried pomace |
Positively influencing the fiber content and improved organoleptic properties |
21 and 91 |
Cider |
47.3 g L−1 of dried apple pomace |
Higher content of phenolic compounds and antioxidant activity |
92
|
Natural food antioxidants |
Lipid stabilizing ability of 73.8% to 97.2% |
Potential inhibitors of the oxidation of polyunsaturated fatty acids |
93
|
Powder ingredient for food formulations |
Can be used in different concentrations as an ingredient in food formulations |
Source of dietary fibers and phenolic compounds |
94
|
Mayonnaise |
2 to 6% of apple pomace |
Improved antioxidant properties, stability after 30 days, and higher overall acceptability |
101
|
Beef burger fortification |
4% and 8% |
Improved fiber and phenol content, and neutral flavor |
23
|
Treating acne |
50 μg mL−1 of apple polyphenols |
Inhibiting fat production in sebaceous cells and regulating sebum production |
95 and 96 |
Pharmaceutical industry |
Source of stabilizing agent |
The reference particle load was set at 100 mg of powder per g of oil, corresponding to 4.8% wt/wt |
Emulsifying potential due to the stabilizing pectins and proteins in the soluble portion |
99
|
Synthesis of silver nanoparticles |
40 mL of apple pomace extract |
The nanoparticles demonstrated high antibacterial activity, and they exhibited a spherical shape, uniform dispersion, small size, and a high degree of crystallization |
100
|
Concluding remarks and future trends
Apple pomace is an inexpensive, easy to-obtain, and bioactive bio-residue. It has considerably high concentrations of bioactive molecules including dietary fibers, polyphenols, and pentacyclic triterpenes. The practical and sustainable use of apple pomace is an auspicious tendency in apple processing industries to decrease economic loss and environmental contamination. Apple pomace has the potential to diversify industry revenue streams through its conversion into high-value products that do not compromise human food security, besides significantly contributing to the circular economy. To enhance extraction yields, minimize processing time, and diminish the ecological impact of toxic solvents, replacing conventional methods with eco-friendly technologies has been demonstrated to be a promising solution. Despite this, the need to develop industrial processes aligned with a circular economy remains. Considering this, integrating the valorization concept permits the transformation of apple pomace into high-value products with pertinent prospective applications for human ingestion and pharmaceutical industries, such as extracting specific molecules and producing novel functional foods. Such transformation requires the food, pharmaceutical, and nutraceutical industries to promote the development of novel products. Lastly, bioavailability and bioaccessibility should be further studied and understood for applying these compounds of interest more efficiently in human health.
Data availability
No primary research results, software, or code have been included and no new data were generated or analyzed as part of this review.
Conflicts of interest
There are no conflicts to declare.
Acknowledgements
The authors extend their gratefulness to the Foundation for Science and Technology (FCT, Portugal) for financial support through national funds FCT/MCTES (PIDDAC) to CIMO (UIDB/00690/2020 and UIDP/00690/2020) and SusTEC (LA/P/0007/2020). The authors are grateful to FEDER Cooperación Interreg VI A Espanha – Portugal (POCTEP) 2021–2027 for financial support through the project TRANSCoLAB PLUS 0112_TRANSCOLAB_PLUS_2_P. The authors also thank the National funding by FCT, through the institutional scientific employment program-contract with L. Barros, and the individual scientific employment program-contract with F. S. Reis (2021.03728.CEECIND) and J. C. M. Barreira (CEECIND/04479/2017). L. A. Pascoalino thanks FCT for her individual research grant (UI/BD/153745/2022).
References
-
J. F. Ayala Zavala, G. A. González-Aguilar and M. W. Siddiqui, Plant Food By-Products : Industrial Relevance for Food Additives and Nutraceuticals, Apple Academic Press, 2021, Available from: https://www.routledge.com/Plant-Food-By-Products-Industrial-Relevance-for-Food-Additives-and-Nutraceuticals/Ayala-Zavala-Gonzalez-Aguilar-Siddiqui/p/book/9781774631355 Search PubMed.
-
Food and Agriculture Organization of the United Nations, Moving forward on Food Loss and Waste Reduction, The State of Food and Agriculture, 2019, Available from: https://www.fao.org/3/ca6030en/ca6030en.pdf Search PubMed.
-
FAO, Food Wastage Footprint & Climate Change, 2015, Available from: https://www.fao.org/3/bb144e/bb144e.pdf Search PubMed.
-
European Commission, An EU Action Plan for the Circular Economy, 2015 Search PubMed.
-
UN General Assembly, United Nations Transforming Our World: the 2030 Agenda for Suistanable Development, United Nations, New York, NY, USA, 2015 Search PubMed.
- E. C. Gaudino, A. Colletti, G. Grillo, S. Tabasso and G. Cravotto, Emerging Processing Technologies for the Recovery of Valuable Bioactive Compounds from Potato Peels, Foods, 2020, 9(11), 1598 CrossRef CAS PubMed , https://www.mdpi.com/2304-8158/9/11/1598/htm.
- M. G. Leichtweis, M. B. P. P. Oliveira, I. C. F. R. Ferreira, C. Pereira and L. Barros, Sustainable Recovery of Preservative and Bioactive Compounds from Food Industry Bioresidues, Antioxidants, 2021, 10(11), 1827 CrossRef CAS PubMed , https://www.mdpi.com/2076-3921/10/11/1827/htm.
- S. Kauser, M. A. Murtaza, A. Hussain, M. Imran, K. Kabir and A. Najam,
et al., Apple pomace, a bioresource of functional and nutritional components with potential of utilization in different food formulations: A review, Food Chem. Adv., 2024, 4, 100598 CrossRef.
- E. Gołębiewska, M. Kalinowska and G. Yildiz, Sustainable Use of Apple Pomace (AP) in Different Industrial Sectors, Materials, 2022, 15(5), 1788 CrossRef PubMed.
- L. A. Pascoalino, F. S. Reis, M. A. Prieto, J. C. M. Barreira, I. C. F. R. Ferreira and L. Barros, Valorization of Bio-Residues from the Processing of Main Portuguese Fruit Crops: From Discarded Waste to Health Promoting Compounds, Molecules, 2021, 26(9), 2624 CrossRef CAS , https://www.mdpi.com/1420-3049/26/9/2624/htm.
- J. C. M. Barreira, A. A. Arraibi and I. C. F. R. Ferreira, Bioactive and functional compounds in apple pomace from juice and cider manufacturing: Potential use in dermal formulations, Trends Food Sci. Technol., 2019, 90, 76–87 CrossRef CAS.
-
FAOSTAT, FAOSTAT, Available from: https://www.fao.org/faostat/es/#data/QI Search PubMed.
-
Statista, Annual volume of apples produced in the European Union (EU-27) from 2011/12 to 2023/24, Available from: https://www.statista.com/statistics/577753/apples-production-volume-european-union/ Search PubMed.
- N. A. Wani and U. Mishra, An integrated circular economic model with controllable carbon emission and deterioration from an apple orchard, J. Clean. Prod., 2022, 374, 133962 CrossRef CAS.
- A. Iqbal, P. Schulz and S. S. H. Rizvi, Valorization of bioactive compounds in fruit pomace from agro-fruit industries: Present Insights and future challenges, Food Biosci., 2021, 44, 101384 CrossRef CAS.
- E. Gołębiewska, M. Kalinowska and G. Yildiz, Sustainable Use of Apple Pomace (AP) in Different Industrial Sectors, Materials, 2022, 15(5), 1788 CrossRef.
- Z. Kırbaş, S. Kumcuoglu and S. Tavman, Effects of apple, orange and carrot pomace powders on gluten-free batter rheology and cake properties, J. Food Sci. Technol., 2019, 56(2), 914–926 CrossRef , https://link.springer.com/article/10.1007/s13197-018-03554-z.
- M. L. Sudha, V. Baskaran and K. Leelavathi, Apple pomace as a source of dietary fiber and polyphenols and its effect on the rheological characteristics and cake making, Food Chem., 2007, 104(2), 686–692 CrossRef CAS.
- G. Laufenberg, B. Kunz and M. Nystroem, Transformation of vegetable waste into value added products: (A) the upgrading concept; (B) practical implementations, Bioresour. Technol., 2003, 87(2), 167–198 CrossRef CAS.
- M. Kruczek, D. Gumul, M. Kacániová, E. Ivanišhová, J. Marecek and H. Gambus, Industrial apple pomace by-products as a potential source of pro-health compounds in functional food, J. Microbiol. Biotechnol. Food Sci., 2017, 7(1), 22–26 CrossRef CAS , https://office2.jmbfs.org/index.php/JMBFS/article/view/8630.
- M. Usman, S. Ahmed, A. Mehmood, M. Bilal, P. J. Patil and K. Akram,
et al., Effect of apple pomace on nutrition, rheology of dough and cookies quality, J. Food Sci. Technol., 2020, 57(9), 3244–3251 CrossRef CAS , https://link.springer.com/article/10.1007/s13197-020-04355-z.
- H. P. V. Rupasinghe, L. Wang, G. M. Huber and N. L. Pitts, Effect of baking on dietary fibre and phenolics of muffins incorporated with apple skin powder, Food Chem., 2008, 107(3), 1217–1224 CAS.
- L. Pollini, F. Blasi, F. Ianni, L. Grispoldi, S. Moretti and A. Di Veroli,
et al., Ultrasound-Assisted Extraction and Characterization of Polyphenols from Apple Pomace, Functional Ingredients for Beef Burger Fortification, Molecules, 2022, 27(6), 1933 CrossRef CAS.
- L. Liu, Y. You, H. Deng, Y. Guo and Y. Meng, Promoting hydrolysis of apple pomace by pectinase and cellulase to produce microbial oils using engineered Yarrowia lipolytica, Biomass Bioenergy, 2019, 126, 62–69 CrossRef CAS.
- M. D. Queji, G. Wosiacki, G. A. Cordeiro, P. G. Peralta-Zamora and N. Nagata, Determination of simple sugars, malic acid and total phenolic compounds in apple pomace by infrared spectroscopy and PLSR, Int. J. Food Sci. Technol., 2010, 45(3), 602–609 CrossRef CAS , https://onlinelibrary.wiley.com/doi/full/10.1111/j.1365-2621.2010.02173.x.
- P. Górnaś and M. Rudzińska, Seeds recovered from industry by-products of nine fruit species with a high potential utility as a source of unconventional oil for biodiesel and cosmetic and pharmaceutical sectors, Ind. Crops Prod., 2016, 83, 329–338 CrossRef.
- P. Górnaś, M. Rudzińska and D. Segliņa, Lipophilic composition of eleven apple seed oils: A promising source of unconventional oil from industry by-products, Ind. Crops Prod., 2014, 60, 86–91 CrossRef.
- D. A. Campos, R. Gómez-García, A. A. Vilas-Boas, A. R. Madureira and M. M. Pintado, Management of Fruit Industrial By-Products—A Case Study on Circular Economy Approach, Molecules, 2020, 25(2), 320 CrossRef CAS PubMed , https://www.mdpi.com/1420-3049/25/2/320/htm.
- D. R. Kammerer, J. Kammerer, R. Valet and R. Carle, Recovery of polyphenols from the by-products of plant food processing and application as valuable food ingredients, Food Res. Int., 2014, 65(PA), 2–12 CrossRef CAS.
- N. Mirabella, V. Castellani and S. Sala, Current options for the valorization of food manufacturing waste: a review, J. Clean. Prod., 2014, 65, 28–41 CrossRef.
- A. S. Brandão, A. Gonçalves and J. M. R. C. A. Santos, Circular bioeconomy strategies: From scientific research to commercially viable products, J. Clean. Prod., 2021, 295, 126407 CrossRef.
- G. Laufenberg, B. Kunz and M. Nystroem, Transformation of vegetable waste into value added products:: (A) the upgrading concept; (B) practical implementations, Bioresour. Technol., 2003, 87(2), 167–198 CrossRef CAS.
- P. Ghisellini, C. Cialani and S. Ulgiati, A review on circular economy: the expected transition to a balanced interplay of environmental and economic systems, J. Clean. Prod., 2016, 114, 11–32 CrossRef.
- A. P. M. Velenturf and P. Purnell, Principles for a sustainable circular economy, Sustain. Prod. Consum., 2021, 27, 1437–1457 CrossRef.
- N. A. Wani and U. Mishra, An integrated circular economic model with controllable carbon emission and deterioration from an apple orchard, J. Clean. Prod., 2022, 374, 133962 CrossRef CAS.
- B. Rabta, An Economic Order Quantity inventory model for a product with a circular economy indicator, Comput. Ind. Eng., 2020, 140, 106215 CrossRef.
- T. M. W. Mak, X. Xiong, D. C. W. Tsang, I. K. M. Yu and C. S. Poon, Sustainable food waste management towards circular bioeconomy: Policy review, limitations and opportunities, Bioresour. Technol., 2020, 297, 122497 CrossRef CAS PubMed.
- G. Ferretti, I. Turco and T. Bacchetti, Apple as a Source of Dietary Phytonutrients: Bioavai-lability and Evidence of Protective Effects against Human Cardiovascular Disease, Food Nutr. Sci., 2014, 5, 1234–1246, DOI:10.4236/fns.2014.513134 , http://www.scirp.org/journal/fns.
- K. Waldbauer, R. McKinnon and B. Kopp, Apple Pomace as Potential Source of Natural Active Compounds, Planta Med., 2017, 83(12–13), 994–1010 Search PubMed.
- A. Ayar, H. Siçramaz, S. Öztürk and S. Öztürk Yilmaz, Probiotic properties of ice creams produced with dietary fibres from by-products of the food industry, Int. J. Dairy Technol., 2018, 71(1), 174–182 CrossRef CAS , https://onlinelibrary.wiley.com/doi/full/10.1111/1471-0307.12387.
- N. P. Bondonno, C. P. Bondonno, N. C. Ward, J. M. Hodgson and K. D. Croft, The cardiovascular health benefits of apples: Whole fruit vs. isolated compounds, Trends Food Sci. Technol., 2017, 69, 243–256 CrossRef CAS.
- P. A. R. Fernandes, S. S. Ferreira, R. Bastos, I. Ferreira, M. T. Cruz and A. Pinto,
et al., Apple Pomace Extract as a Sustainable Food Ingredient, Antioxidants, 2019, 8(6), 189 CrossRef CAS , https://www.mdpi.com/2076-3921/8/6/189/htm.
- A. Babarikina, V. Nikolajeva, D. Babarykin, A. Babarikina, V. Nikolajeva and D. Babarykin, Anti-Helicobacter Activity of Certain Food Plant Extracts and Juices and Their Composition in Vitro, Food Nutr. Sci., 2011, 2(8), 868–877 CAS , http://www.scirp.org/Html/10-2700184_7889.htm.
- L. Lin, A. Peng, K. Yang and Y. Zou, Monomeric phenolics in different parts of high-acid apple (Malus sieversii f. niedzwetzkyana (Dieck) Langenf): a promising source of antioxidants for application in nutraceuticals, Int. J. Food Sci. Technol., 2018, 53(6), 1503–1509 CrossRef CAS , https://onlinelibrary.wiley.com/doi/full/10.1111/ijfs.13731.
- C. G. Grigoras, E. Destandau, L. Fougère and C. Elfakir, Evaluation of apple pomace extracts as a source of bioactive compounds, Ind. Crops Prod., 2013, 49, 794–804 CrossRef CAS.
- K. Waldbauer, G. Seiringer, D. L. Nguyen, J. Winkler, M. Blaschke and R. McKinnon,
et al., Triterpenoic Acids from Apple Pomace Enhance the Activity of the Endothelial Nitric Oxide Synthase (eNOS), J. Agric. Food Chem., 2016, 64(1), 185–194 CrossRef CAS , https://pubs.acs.org/doi/full/10.1021/acs.jafc.5b05061.
- S. T. Carpes, C. Bertotto, A. P. Bilck, F. Yamashita, O. Anjos and M. A. Bakar Siddique,
et al., Bio-based films prepared with apple pomace: Volatiles compound composition and mechanical, antioxidant and antibacterial properties, Lebensm. Wiss. Technol., 2021, 144, 111241 CrossRef CAS.
- A. Ricci, M. Cirlini, A. Guido, C. M. Liberatore, T. Ganino and C. Lazzi,
et al., From Byproduct to Resource: Fermented Apple Pomace as Beer Flavoring, Foods, 2019, 8(8), 309 CrossRef CAS , https://www.mdpi.com/2304-8158/8/8/309/htm.
- R. R. Madrera and B. S. Valles, Determination of Volatile Compounds in Apple Pomace by Stir Bar Sorptive Extraction and Gas Chromatography-Mass Spectrometry (SBSE-GC-MS), J. Food Sci., 2011, 76(9), C1326–C1334 CrossRef CAS.
- F. Lyu, S. F. Luiz, D. R. P. Azeredo, A. G. Cruz, S. Ajlouni and C. S. Ranadheera, Apple Pomace as a Functional and Healthy Ingredient in Food Products: A Review, Processes, 2020, 8(3), 319 CrossRef CAS.
- G. A. Martău, B. E. Teleky, F. Ranga, I. D. Pop and D. C. Vodnar, Apple Pomace as a Sustainable Substrate in Sourdough Fermentation, Front. Microbiol., 2021, 12, 742020 CrossRef.
- V. Radenkovs, J. Kviesis, K. Juhnevica-Radenkova, A. Valdovska, T. Püssa and M. Klavins,
et al., Valorization of Wild Apple (Malus spp.) By-Products as a Source of Essential Fatty Acids, Tocopherols and Phytosterols with Antimicrobial Activity, Plants, 2018, 7(4), 90 CrossRef CAS.
- L. Pollini, A. Juan-García, F. Blasi, J. Mañes, L. Cossignani and C. Juan, Assessing bioaccessibility and bioavailability in vitro of phenolic compounds from freeze-dried apple pomace by LC-Q-TOF-MS, Food Biosci., 2022, 48, 101799 CrossRef CAS.
- W. J. Hollands, D. J. Hart, J. R. Dainty, O. Hasselwander, K. Tiihonen and R. Wood,
et al., Bioavailability of epicatechin and effects on nitric oxide metabolites of an apple flavanol-rich extract supplemented beverage compared to a whole apple puree: a randomized, placebo-controlled, crossover trial, Mol. Nutr. Food Res., 2013, 57(7), 1209–1217 CrossRef CAS , https://pubmed.ncbi.nlm.nih.gov/23610075/.
- T. Belwal, F. Chemat, P. R. Venskutonis, G. Cravotto, D. K. Jaiswal and I. D. Bhatt,
et al., Recent advances in scaling-up of non-conventional extraction techniques: Learning from successes and failures, Trac. Trends Anal. Chem., 2020, 127, 115895 CrossRef CAS.
- I. Dini and N. Nunes, Bio Discarded from Waste to Resource, Foods, 2021, 10(11), 2652 CrossRef PubMed , https://www.mdpi.com/2304-8158/10/11/2652/htm.
- M. B. Soquetta, L. Terra, M. de and C. P. Bastos, Green technologies for the extraction of bioactive compounds in fruits and vegetables, CyTA – J. Food, 2018, 16(1), 400–412 CrossRef CAS.
- M. Pattnaik, P. Pandey, G. J. O. Martin, H. N. Mishra and M. Ashokkumar, Innovative Technologies for Extraction and Microencapsulation of Bioactives from Plant-Based Food Waste and Their Applications in Functional Food Development, Foods, 2021, 10(2), 279 CrossRef CAS PubMed , https://www.mdpi.com/2304-8158/10/2/279/htm.
- P. M. Albuquerque, F. Koch, T. G. Trossini, E. Esposito and L. N. Ninow, Production of Rhizopus oligosporus protein by solid state fermentation of apple pomace, Braz. Arch. Biol. Technol., 2006, 91–100 CAS , https://www.researchgate.net/publication/279577400_Production_of_Rhizopus_oligosporus_protein_by_solid_state_fermentation_of_apple_pomace.
- B. Gullón, E. Falqué, J. L. Alonso and J. C. Parajó, Evaluation of apple pomace as a raw material for alternative applications in food industries, Food Technol. Biotechnol., 2007, 45(4), 426–433 Search PubMed.
- I. H. Adil, H. I. Çetin, M. E. Yener and A. Bayindirli, Subcritical (carbon dioxide + ethanol) extraction of polyphenols from apple and peach pomaces, and determination of the antioxidant activities of the extracts, J. Supercrit. Fluids, 2007, 43(1), 55–63 CrossRef CAS.
- H. Wijngaard and N. Brunton, The optimization of extraction of antioxidants from apple pomace by pressurized liquids, J. Agric. Food Chem., 2009, 57(22), 10625–10631 CrossRef CAS , https://pubs.acs.org/doi/full/10.1021/jf902498y.
- X. L. Bai, T. L. Yue, Y. H. Yuan and H. W. Zhang, Optimization of microwave-assisted extraction of polyphenols from apple pomace using response surface methodology and HPLC analysis, J. Sep. Sci., 2010, 33(23–24), 3751–3758 CrossRef CAS PubMed , https://pubmed.ncbi.nlm.nih.gov/21077127/.
- D. Pingret, A. S. Fabiano-Tixier, C. l. Bourvellec, C. M. G. C. Renard and F. Chemat, Lab and pilot-scale ultrasound-assisted water extraction of polyphenols from apple pomace, J. Food Eng., 2012, 111(1), 73–81 CrossRef CAS.
- M. Plaza, V. Abrahamsson and C. Turner, Extraction and neoformation of antioxidant compounds by pressurized hot water extraction from apple byproducts, J. Agric. Food Chem., 2013, 61(23), 5500–5510 CrossRef CAS , https://pubs.acs.org/doi/full/10.1021/jf400584f.
- I. Parmar and H. P. V. Rupasinghe, Bio-conversion of apple pomace into ethanol and acetic acid: Enzymatic hydrolysis and fermentation, Bioresour. Technol., 2013, 130, 613–620 CrossRef CAS.
- X. Wang, Q. Chen and X. Lü, Pectin extracted from apple pomace and citrus peel by subcritical water, Food Hydrocolloids, 2014, 38, 129–137 CrossRef CAS.
- V. Chandrasekar, M. F. S. Martín-González, P. Hirst and T. S. Ballard, Optimizing Microwave-Assisted Extraction of Phenolic Antioxidants from Red Delicious and Jonathan Apple Pomace, J. Food Process. Eng., 2015, 38(6), 571–582 CrossRef CAS.
- K. Piwowarek, E. Lipińska and E. Hać-Szymańczuk, Possibility of using apple pomaces in the process of propionic-acetic fermentation, Electron. J. Biotechnol., 2016, 23, 1–6 CrossRef.
- U. C. Lohani and K. Muthukumarappan, Application of the pulsed electric field to release bound phenolics in sorghum flour and apple pomace, Innovat. Food Sci. Emerg. Technol., 2016, 35, 29–35 CrossRef CAS.
- G. Ferrentino, K. Morozova, O. K. Mosibo, M. Ramezani and M. Scampicchio, Biorecovery of antioxidants from apple pomace by supercritical fluid extraction, J. Clean. Prod., 2018, 186, 253–261 CrossRef CAS.
- H. Withouck, A. Boeykens, M. Vanden Broucke, M. M. Moreira, C. Delerue-Matos and L. De Cooman, Evaluation of the impact of pre-treatment and extraction conditions on the polyphenolic profile and antioxidant activity of Belgium apple wood, Eur. Food Res. Technol., 2019, 245(11), 2565–2578 CrossRef CAS , https://link.springer.com/article/10.1007/s00217-019-03373-2.
- J. Luo and Y. Xu, Comparison of Biological and Chemical Pretreatment on Coproduction of Pectin and Fermentable Sugars from Apple Pomace, Appl. Biochem. Biotechnol., 2020, 190(1), 129–137 CrossRef CAS PubMed , https://eurekamag.com/research/069/148/069148006.php.
- L. V. Leonel, L. Sene, M. A. A. da Cunha, K. C. F. Dalanhol and M. d. G. de Almeida Felipe, Valorization of apple pomace using bio-based technology for the production of xylitol and 2G ethanol, Bioprocess Biosyst. Eng., 2020, 43(12), 2153–2163 CrossRef CAS , https://pubmed.ncbi.nlm.nih.gov/32627063/.
- L. C. da Silva, J. Viganó, V. L. Sanches, L. M. de Souza Mesquita, R. Pizani and M. A. Rostagno, Simultaneous extraction and analysis of apple pomace by gradient pressurized liquid extraction coupled in-line with solid-phase extraction and on-line with HPLC, Food Chem., 2023, 407, 135117 CrossRef CAS PubMed.
- G. S. Dhillon, S. K. Brar, M. Verma and R. D. Tyagi, Apple pomace ultrafiltration sludge – A novel substrate for fungal bioproduction of citric acid: Optimisation studies, Food Chem., 2011, 128(4), 864–871 CrossRef CAS.
- S. Maiti, S. J. Sarma, S. K. Brar, Y. le Bihan, P. Drogui and G. Buelna,
et al., Agro-industrial wastes as feedstock for sustainable bio-production of butanol by Clostridium beijerinckii, Food Bioprod. Process., 2016, 98, 217–226 CrossRef CAS.
- R. K. Das, S. K. Brar and M. Verma, A fermentative approach towards optimizing directed biosynthesis of fumaric acid by Rhizopus oryzae 1526 utilizing apple industry waste biomass, Fungal Biol., 2015, 119(12), 1279–1290 CrossRef CAS.
- R. M. Alonso-Salces, E. Korta, A. Barranco, L. A. Berrueta, B. Gallo and F. Vicente, Pressurized liquid extraction for the determination of polyphenols in apple, J. Chromatogr. A, 2001, 933(1–2), 37–43 CrossRef CAS , https://pubmed.ncbi.nlm.nih.gov/11758745/.
- A. Massias, S. Boisard, M. Baccaunaud, F. Leal Calderon and P. Subra-Paternault, Recovery of phenolics from apple peels using CO2 + ethanol extraction: Kinetics and antioxidant activity of extracts, J. Supercrit. Fluids, 2015, 98, 172–182 CrossRef CAS.
- A. Saini, P. S. Panesar and M. B. Bera, Valorization of fruits and vegetables waste through green extraction of bioactive compounds and their nanoemulsions-based delivery system, Bioresour. Bioprocess., 2019, 6(1), 1–12 CrossRef , https://bioresourcesbioprocessing.springeropen.com/articles/10.1186/s40643-019-0261-9.
- K. Ameer, H. M. Shahbaz and J. H. Kwon, Green Extraction Methods for Polyphenols from Plant Matrices and Their Byproducts: A Review, Compr. Rev. Food Sci. Food Saf., 2017, 16(2), 295–315 CrossRef PubMed , https://onlinelibrary.wiley.com/doi/full/10.1111/1541-4337.12253.
- A. Saini, P. S. Panesar and M. B. Bera, Valorization of fruits and vegetables waste through green extraction of bioactive compounds and their nanoemulsions-based delivery system, Bioresour. Bioprocess., 2019, 6(1), 1–12 CrossRef , https://bioresourcesbioprocessing.springeropen.com/articles/10.1186/s40643-019-0261-9.
- I. J. Seabra, A. M. A. Dias, M. E. M. Braga and H. C. de Sousa, High pressure solvent extraction of maritime pine bark: Study of fractionation, solvent flow rate and solvent composition, J. Supercrit. Fluids, 2012, 62, 135–148 CrossRef CAS.
- B. Kaufmann and P. Christen, Recent extraction techniques for natural products: Microwave-assisted extraction and pressurised solvent extraction, Phytochem. Anal., 2002, 13(2), 105–113 CrossRef CAS.
- T. S. Chamblee, R. R. Weikel, S. A. Nolen, C. L. Liotta and C. A. Eckert, Reversible in situ acid formation for β-pinene hydrolysis using CO2 expanded liquid and hot water, Green Chem., 2004, 6(8), 382–386 RSC.
- C. Pronyk and G. Mazza, Design and scale-up of pressurized fluid extractors for food and bioproducts, J. Food Eng., 2009, 95(2), 215–226 CrossRef CAS.
- M. Pattnaik, P. Pandey, G. J. O. Martin, H. N. Mishra and M. Ashokkumar, Innovative Technologies for Extraction and Microencapsulation of Bioactives from Plant-Based Food Waste and Their Applications in Functional Food Development, Foods, 2021, 10(2), 279 CrossRef CAS , https://www.mdpi.com/2304-8158/10/2/279/htm.
- S. A. Mir, S. J. D. Bosco, M. A. Shah, S. Santhalakshmy and M. M. Mir, Effect of apple pomace on quality characteristics of brown rice based cracker, J. Saudi Soc. Agric. Sci., 2017, 16(1), 25–32 Search PubMed.
- K. L. Wolfe and R. H. Liu, Apple peels as a value-added food ingredient, J. Agric. Food Chem., 2003, 51(6), 1676–1683 CrossRef CAS , https://pubmed.ncbi.nlm.nih.gov/12617604/.
- N. M. V. de Toledo, L. P. Nunes, P. P. M. da Silva, M. H. F. Spoto and S. G. Canniatti-Brazaca, Influence of pineapple, apple and melon by-products on cookies: physicochemical and sensory aspects, Int. J. Food Sci. Technol., 2017, 52(5), 1185–1192 CrossRef , https://onlinelibrary.wiley.com/doi/full/10.1111/ijfs.13383.
- D. G. Bortolini, L. Benvenutti, I. M. Demiate, A. Nogueira, A. Alberti and A. A. F. Zielinski, A new approach to the use of apple pomace in cider making for the recovery of phenolic compounds, Lebensm. Wiss. Technol., 2020, 126, 109316 CrossRef CAS.
- G. M. Huber and H. P. V. Rupasinghe, Phenolic Profiles and Antioxidant Properties of Apple Skin Extracts, J. Food Sci., 2009, 74(9), C693–C700 CrossRef CAS PubMed , https://onlinelibrary.wiley.com/doi/full/10.1111/j.1750-3841.2009.01356.x.
- C. Henríquez, H. Speisky, I. Chiffelle, T. Valenzuela, M. Araya and R. Simpson,
et al., Development of an Ingredient Containing Apple Peel, as a Source of Polyphenols and Dietary Fiber, J. Food Sci., 2010, 75(6), H172–H181 CrossRef , https://onlinelibrary.wiley.com/doi/full/10.1111/j.1750-3841.2010.01700.x..
- K. E. Lee, J. K. Youm, W. J. Lee, S. Kang and Y. J. Kim, Polyphenol-rich apple extract inhibits dexamethasone-induced sebaceous lipids production by regulating SREBP1 expression, Exp. Dermatol., 2017, 26(10), 958–960 CrossRef CAS , https://onlinelibrary.wiley.com/doi/full/10.1111/exd.13319.
- M. Działo, J. Mierziak, U. Korzun, M. Preisner, J. Szopa and A. Kulma, The Potential of Plant Phenolics in Prevention and Therapy of Skin Disorders, Int. J. Mol. Sci., 2016, 17(2), 160 CrossRef PubMed , https://www.mdpi.com/1422-0067/17/2/160/htm..
- M. Marić, A. N. Grassino, Z. Zhu, F. J. Barba, M. Brnčić and S. Rimac Brnčić, An overview of the traditional and innovative approaches for pectin extraction from plant food wastes and by-products: Ultrasound-, microwaves-, and enzyme-assisted extraction, Trends Food Sci. Technol., 2018, 76, 28–37 CrossRef.
- L. Skrypnik and A. Novikova, Response Surface Modeling and Optimization of Polyphenols Extraction from Apple Pomace Based on Nonionic Emulsifiers, Agronomy, 2020, 10, 92 CrossRef CAS , https://www.mdpi.com/2073-4395/10/1/92/htm..
- D. Huc-Mathis, C. Journet, N. Fayolle and V. Bosc, Emulsifying properties of food by-products: Valorizing apple pomace and oat bran, Colloids Surf. A, 2019, 568, 84–91 CrossRef CAS.
- Y. y. Ren, H. Yang, T. Wang and C. Wang, Bio-synthesis of silver nanoparticles with antibacterial activity, Mater. Chem. Phys., 2019, 235, 121746 CrossRef CAS.
- L. Mangiapelo, F. Ianni, C. Pagano, L. Grispoldi, F. Blasi and B. Cenci-Goga,
et al., Role of apple pomace in the formulation of a novel healthy mayonnaise, Eur. Food Res. Technol., 2023, 249(11), 2835–2847 CrossRef CAS.
|
This journal is © The Royal Society of Chemistry 2025 |
Click here to see how this site uses Cookies. View our privacy policy here.