Sulfur-linked cyanoterphenyl-based liquid crystal dimers and the twist-bend nematic phase†
Received
1st November 2024
, Accepted 25th February 2025
First published on 26th February 2025
Abstract
The synthesis and characterisation of two series of cyanoterphenyl-based liquid crystal dimers containing sulfur links between the spacer and mesogenic units, the 34-{ω-[(4′-cyano-[1,1′-biphenyl]-4-yl)thio]alkyl}-[11,21:24,31-terphenyl]-14-carbonitriles (CBSnCT), and the 34-({ω-[(4′-cyano-[1,1′-biphenyl]-4-yl)thio]alkyl}oxy)-[11,21:24,31-terphenyl]-14-carbonitriles (CBSnOCT) are described. The odd members of both series show twist-bend nematic and nematic phases, whereas the even members exhibit only the nematic phase. This is consistent with the widely held view that molecular curvature is a prerequisite for the observation of the twist-bend nematic phase. The nematic–isotropic and twist-bend nematic–nematic transition temperatures are higher for the dimers containing cyanoterphenyl groups than for the corresponding cyanobiphenyl-based dimers. This change is more pronounced for the nematic–isotropic transition temperatures and is attributed to the enhanced interaction strength parameter associated with the cyanoterphenyl fragment whereas the molecular shapes, as governed by the spacer, are rather similar. The behaviour of CBS2CT appears somewhat anomalous and exhibits a higher value of the twist-bend nematic–nematic transition temperature than expected, and this is attributed to the presence of highly bent molecular conformations.
Introduction
The twist-bend nematic (NTB) phase continues to be the focus of considerable research activity following its experimental discovery in 2011, perhaps largely because it provides a rare example of spontaneous chiral symmetry breaking in a system composed of achiral molecules.1–4 The NTB phase was first predicted in 1976 by Meyer5 and later independently by Dozov in 2001.6 Dozov suggested that bent molecules have a strong natural tendency to pack into bent structures, however, pure uniform bend is not allowed in nature and thus must be accompanied by other deformations in the local director, such as splay or twist. These deformations give rise to the splay-bend or twist-bend nematic phases.6 In the twist-bend nematic phase the director forms a helix, and is tilted with respect to the helical axis at a constant angle, see Fig. 1.7 The formation of chirality is spontaneous and hence, equal numbers of left- and right-handed helices would be expected.8 The pitch of the helix in the NTB phase was found to be surprisingly short, of the order of 10 nm, which corresponds to just 3 to 4 molecular lengths.7,9
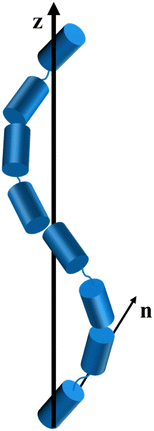 |
| Fig. 1 A schematic representation of the twist-bend nematic (NTB) phase with z denoting the helical axis, and n, the local director. | |
The NTB phase was first reported in the compound, 1,7-bis-4-(4′-cyanobiphenyl)heptane, commonly referred to as CB7CB.1 CB7CB is a liquid crystal dimer in which two cyanobiphenyl mesogenic units are connected by a flexible spacer. The properties of a dimer are strongly dependent on the parity of the spacer, and this is attributed to the role played by the spacer in determining the molecular shape. Thus, if we consider the spacer in an all-trans conformation then, for an even-membered spacer, the mesogenic units are more or less parallel, and the molecule is essentially linear. In contrast, for an odd-membered spacer the two mesogenic units are inclined with respect to each other and the molecule is bent. CB7CB is a bent, odd-membered dimer and it quickly became apparent that molecular bend is a prerequisite for the observation of the NTB phase.6,10 This is wholly consistent with both the predictions made by Dozov6 and those made using a Maier–Saupe theory developed for V-shaped molecules.11 Within the framework of this model, for bend angles less than 130°, the direct twist-bend nematic to isotropic liquid transition is expected, for angles between 130° and 150° the twist-bend nematic phase will be preceded by a nematic phase and for angles above 150° the twist-bend nematic to nematic transition is predicted only at very low temperatures.11 The overwhelming majority of twist-bend nematogens may be described as odd-membered symmetric12–21 or non-symmetric dimers,22–35 but other molecular architectures also support the NTB phase including trimers,15,27,36,37 tetramers,38 higher oligomers,39–42 bent-core mesogens,43–45 hydrogen-bonded systems46–50 and chiral mesogens.22,48,51–53 Recently, an example of a dimer with an overall even-membered spacer was shown to exhibit the NTB phase.54 Despite this large collection of twist-bend nematogens, direct NTB–I transitions are still very rare either in pure materials or in mixtures.16,21,55–59
The inclusion of sulfur in compounds designed to show liquid crystalline behaviour is highly topical and not least because it increases the optical birefringence due to its high polarisability.60–63 Sulfur also tends to reduce the liquid crystal transition temperatures although increases the melting point.64–66 Highly birefringent mesogenic materials are of significant interest because of potential applications in liquid crystal displays,67,68 liquid crystal lenses,69–71 lasers,72,73 plasmonic devices74 and holographic materials.75 Synthetically, there are a wide range of options for incorporating sulfur into mesogenic structures including terminal alkylthio chains,25,64,65,76–80 thiophene moieties,81–84 thiocyanate terminal groups,16,60,85,86 and thioester linking groups.87–89 In terms of twist-bend nematogens, and more specifically dimers and trimers, sulfur has most often been included within the spacer.12,13,15,31,36,57,90 If we consider the link between the mesogenic unit and the spacer then replacing either an oxygen or carbon atom by sulfur reduces the molecular bend angle because the C–S–C bond angle, 100.5°, is lower than either the C–C–C, 113.5°, or C–O–C, 119°, bond angles.12 As we have seen, the formation of the NTB phase is thought to be driven by molecular curvature, and the expectation is that a more bent dimer may show direct NTB–I transitions. Increasing molecular curvature, however, will decrease the liquid crystal transition temperatures, and very monotropic behaviour is a likely outcome. To offset this possibility, the structural anisotropy of the mesogenic units can be increased. Thus, here we describe the transitional properties of two new series of sulfur-containing liquid crystalline dimers containing cyanobiphenyl and cyanoterphenyl moieties. The cyanoterphenyl moiety has an additional phenyl ring compared to cyanobiphenyl, and this greater shape anisotropy will significantly increase the transition temperatures. Studies of twist-bend nematogens containing a cyanoterphenyl mesogenic unit are limited12,32,91–94 due to the tendency of such compounds to instead exhibit smectic phases.26,95 In the first series of compounds, the 34-({ω-[(4′-cyano-[1,1′-biphenyl]-4-yl)thio]alkyl}oxy)-[11,21:24,31-terphenyl]-14-carbonitriles, Fig. 2, the focus was on odd-membered spacers, and these ensured the prerequisite molecular curvature for the observation of the NTB phase. For comparative purposes, however, we also report the behaviour of the even members with n = 4 and 6. This series is referred to using the acronym CBSnOCT in which CB and CT refer to cyanobiphenyl and cyanoterphenyl, respectively, n the number of methylene units in the flexible spacer, and S and O to thioether and ether linkages, respectively.
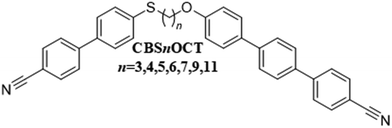 |
| Fig. 2 The molecular structure of the CBSnOCT series. | |
To further compare the effects of changing the nature of the linking groups between the spacer and mesogenic units, and hence the molecular shape, a second cyanoterphenyl-based series was synthesised, the 34-{ω-[(4′-cyano-[1,1′-biphenyl]-4-yl)thio]alkyl}-[11,21:24,31-terphenyl]-14-carbonitriles, see Fig. 3. The acronym used to refer to this series is CBSnCT, in which n now denotes the number of methylene units in the spacer including the methylene link to the cyanoterphenyl unit. Again, our focus has been on dimers having an overall odd-membered spacer noting that now when n is even and sulfur is included, an odd number of atoms connect the two mesogenic units. For comparative purposes, we also report the properties of an even-member of the series with n = 5.
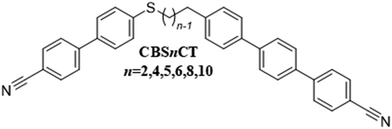 |
| Fig. 3 The molecular structure of the CBSnCT series. | |
Experimental
Synthesis
The synthesis of the CBSnOCT series (3) follows the steps outlined in Scheme 1. 4-Bromo-4′-hydroxybiphenyl was reacted with the appropriate dibromoalkane in a Williamson ether reaction96 to obtain 1 which was reacted with 4-bromothiophenol in a modified Williamson ether reaction to obtain 2. Finally, 2 and 4-cyanophenylboronic acid were reacted in a Suzuki–Miyaura cross-coupling reaction to form the desired product 3. The synthesis of the CBSnCT (8) series followed the steps outlined in Scheme 2. Thus, the appropriate bromoalkanoic acid was converted to the corresponding acid chloride, used immediately in a Friedel–Crafts acylation with 4-bromobiphenyl, and the product subsequently underwent a hydrosilane reduction. 6 was reacted with 4-bromothiophenol in a modified Williamson ether reaction and a subsequent Suzuki–Miyaura cross-coupling reaction of 7 with 4-cyanophenylboronic acid was used to form the desired product 8. A detailed description of the preparation of these compounds, including the structural characterisation data for all intermediates and final products, is provided in the ESI.†
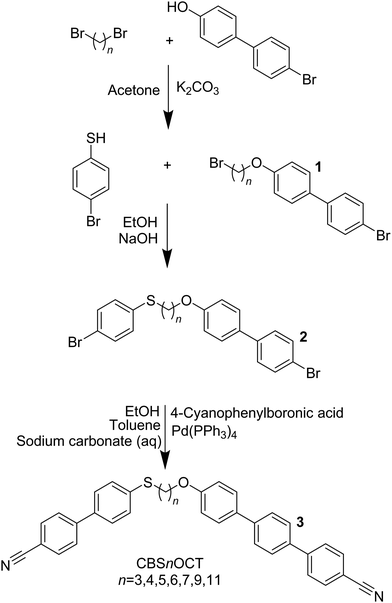 |
| Scheme 1 Synthesis of the CBSnOCT series. | |
 |
| Scheme 2 Synthesis of the CBSnCT series. | |
Optical studies
Phase characterisation was performed by polarised light microscopy, using an Olympus BH2 polarising light microscope equipped with a Linkam TMS 92 hot stage.
Differential scanning calorimetry
The phase behaviour of the materials was studied by differential scanning calorimetry performed using a Mettler Toledo DSC1 or DSC3 differential scanning calorimeter equipped with TSO 801RO sample robots and calibrated using indium and zinc standards. Heating and cooling rates were 10 °C min−1, with a 3-min isotherm between either heating or cooling, and all samples were measured under a nitrogen atmosphere. Transition temperatures and associated enthalpy changes were extracted from the heating traces unless otherwise noted. For each sample, two aliquots were measured, and the data listed are the average of the two sets of data.
Molecular modelling
The geometric parameters of the reported compounds were obtained using quantum mechanical DFT calculations with Gaussian09 software.97 Optimisation of the structures was carried out at the B3LYP/6-311G(d,p) level of theory. Visualisations of the space-filling models were produced post-optimisation using the QuteMol package.98
Conformational modelling
Conformational preferences of compounds CBS2CT and CBS6CT were studied by generating 1000 conformers for each system using the ETKDGv3 (Experimental-Torsion with Knowledge Distance Geometry version 3) as implemented in RDkit.99–102 The geometry of each conformer was then optimized with the MMFF (Merck molecular force field) method. After removing duplicate entries through pruning, the bend-angle of each unique conformer was calculated as the angle between the vectors defined by the two nitrile C–N bonds. From the energy of each conformer, the probability weighted bend-angle calculated at a temperature of 298 K was obtained.
Birefringence measurements
Optical birefringence was measured with a setup based on a photoelastic modulator (PEM-90, Hinds) working at a modulation frequency f = 50 kHz; as a light source, a halogen lamp (Hamamatsu LC8) was used equipped with narrow bandpass filter (532 nm). The signal from a photodiode (FLC Electronics PIN-20) was deconvoluted with a lock-in amplifier (EG&G 7265) into 1f and 2f components to yield a retardation induced by the sample. Knowing the sample thickness, the retardation was recalculated into optical birefringence. Samples were prepared in 1.8-μm-thick cells with planar anchoring. The alignment quality was checked prior to measurement by inspection under the polarised light optical microscope.
X-ray diffraction
2D X-ray diffraction patterns were obtained with the Bruker D8 GADDS system (micro-focus-type X-ray source with Cu anode, Goebel mirror monochromator, 0.5
mm point beam collimator, VANTEC2000 area detector), equipped with a modified Linkam heating stage. Samples were prepared as droplets on a heated surface.
Results
The transitional properties of the CBSnOCT series are listed in Table 1. The homologues with an odd-membered spacer showed a conventional enantiotropic nematic phase, N, and a monotropic twist-bend nematic phase, NTB. The nematic phase was assigned by the observation of a characteristic schlieren texture containing both two- and four-point brush defects when sandwiched between two untreated glass slides, Fig. 4(a). In addition, the values of the scaled entropy change associated with the nematic–isotropic transition, ΔSNI/R, listed in Table 1 are consistent with this phase assignment. As the nematic phase was cooled, the optical flickering associated with director fluctuations ceased and a blocky schlieren texture formed along with regions of focal conic textures coexisting with a polygonal texture characteristic of the NTB phase, Fig. 4(b). The even homologues showed exclusively a conventional enantiotropic nematic phase that crystallized on cooling without the formation of another liquid crystal phase. As would be expected, the even members showed higher values of both TNI, and ΔSNI/R compared to those of the odd members.
Table 1 Transition temperatures and associated entropy changes for the CBSnOCT series
n
|
T
CrN/°C |
T
NTBN/°C |
T
NI/°C |
ΔSCrN/R |
ΔSNTBN/R |
ΔSNI/R |
Values extracted from DSC cooling traces.
|
3 |
168 |
100a |
277 |
6.84 |
≈0a |
0.46 |
4 |
210 |
— |
316 |
7.77 |
— |
1.12 |
5 |
187 |
129a |
271 |
7.95 |
≈0a |
0.48 |
6 |
207 |
— |
296 |
9.41 |
— |
1.37 |
7 |
147 |
130a |
265 |
5.69 |
≈0a |
0.52 |
9 |
120 |
119a |
245 |
7.16 |
≈0a |
0.60 |
11 |
128 |
115a |
236 |
8.48 |
≈0a |
0.62 |
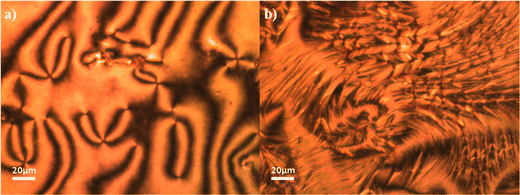 |
| Fig. 4 Textures observed for CBS7OCT; (a) schlieren texture of the nematic phase (T = 205 °C) and (b) focal conic regions with blocky schlieren texture of the twist-bend nematic phase (T = 111 °C). | |
The transitional properties of the CBSnCT series are listed in Table 2. All the homologues with an overall odd-membered central spacer, i.e., an even value of n, exhibited the conventional nematic phase, N, and the twist-bend nematic phase, NTB. We reported the transition temperatures for CBS6CT previously.12 These phase assignments were based on the observation of characteristic optical textures and representative examples are shown in Fig. 5, and these have been described earlier. The values of ΔSNI/R listed in Table 2 are consistent with this assignment. The NTB phase assignment for CBS6CT has been confirmed previously using resonant soft X-ray scattering (RSoXS).12 The values of ΔSNTBN/R listed in Table 2 for n = 2 and 4 are particularly small, and these are consistent with the small associated values of TNTBN/TNI.12 The dimer with an overall even-membered spacer, CBS5CT, showed only a conventional enantiotropic nematic phase, and again as would be expected, the associated values of both TNI and ΔSNI/R are significantly higher than those observed for the odd members.
Table 2 Transition temperatures and associated entropy changes for the CBSnCT series
n
|
T
CrN/°C |
T
NTBN/°C |
T
NI/°C |
ΔSCrN/R |
ΔSNTBN/R |
ΔSNI/R |
Values extracted from DSC cooling traces.
|
2 |
165 |
149a |
201 |
4.99 |
0.070a |
0.089 |
4 |
169 |
153a |
237 |
7.54 |
0.011a |
0.20 |
5 |
225 |
— |
297 |
8.12 |
— |
1.29 |
6 |
131 |
145 |
239 |
5.57 |
≈0 |
0.35 |
8 |
113 |
146 |
235 |
7.53 |
≈0 |
0.46 |
10 |
101 |
138 |
224 |
4.33 |
≈0 |
0.56 |
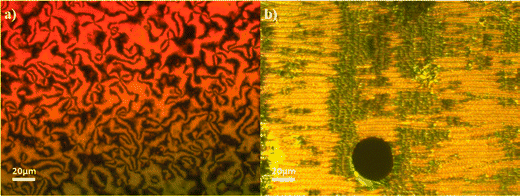 |
| Fig. 5 Textures observed for CBS6CT: (a) schlieren texture of the nematic phase (T = 157 °C) and (b) rope-like textures coexisting with a polygonal texture of the twist-bend nematic phase (T = 95 °C). | |
The temperature dependence of the optical birefringence was measured for the homologues of the CBSnCT series, with n = 2, 4, 8 and 10 (Fig. 6 and Fig. S1, ESI†). Similar behaviour was found for all the homologues, with the birefringence in the nematic phase following a power law increase,
, below the Iso-N phase transition (Tc). The parameter Δn0, which reflects the extrapolated birefringence of the perfectly ordered nematic phase, systematically decreased with elongation of the spacer length, from 0.48 to 0.43 for CBS2CT and CBS10CT, respectively. The trend can be ascribed to progressive dilution of the highly anisotropic biphenyl and terphenyl cores with less anisotropic aliphatic chains of increasing length. The critical exponent β was found to be close to 0.2, and similar values were determined previously, e.g., for bent core nematogens.103 A chosen homologue of the CBSnOCT series, namely CBS9OCT, was also measured as a direct comparison with CBS10CT, both these dimers having the same total length of the spacer, s = 11, Fig. 6. The similar power law dependence of birefringence was found, however with considerably higher value of Δn0 = 0.50. This can be attributed to the greater linearity of the ether-linked dimer. The relatively high birefringence of all the sulfur linked dimers studied here is thought to be linked to the high polarizability of the sulfur atom.104 On the approach to the NTB phase, the measured birefringence departed from the critical, power law dependence due to the formation of instantaneous, local heliconical states.105
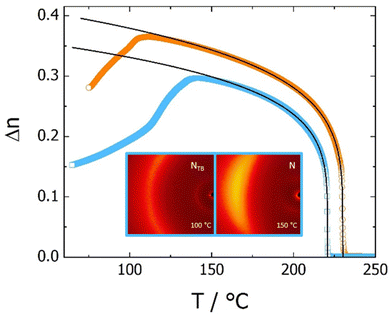 |
| Fig. 6 Temperature dependence of the optical birefringence for CBS9OCT (orange circles) and CBS10CT (blue squares) measured with green light (λ = 532 nm) on cooling. Black lines show fitted power-law dependencies. Inset: 2D X-ray diffraction patterns for CBS9OCT in the: (right) N phase (T = 150 °C) and (left) NTB phase (T = 100 °C). | |
Both CBS9OCT and CBS10CT were also characterised using conventional non-resonance X-ray diffraction (Fig. 6 and Fig. S2, S3, ESI†). For both compounds, only diffuse X-ray diffraction signals were detected in the low and wide-angle regions of the N phase, indicative of a lack of long-range positional ordering of the molecules. From the analysis of the signal width a correlation length for molecular positions was estimated to be ∼10 Å and ∼5 Å, along and perpendicular to the director, respectively, indicating local positional order extending only to the nearest neighbours. There was no qualitative change in the signal positions, nor widths, upon entering the NTB phase, the correlation length along director increased slightly to ∼15 Å. The local periodicities in the N and NTB phases, for both CBS9OCT and CBS10CT compounds, deduced from the peak measured in the low-angle region corresponded to about half the molecular length, l, suggesting an intercalated packing arrangement of the molecules. This matches our previous reporting on similar sulfur dimers12 and a schematic showing how this packing arrangement would fit into the NTB helix is shown in Fig. 7.
 |
| Fig. 7 Schematic showing the intercalated packing arrangement in the NTB phase. | |
Discussion
The dependence of the nematic–isotropic, TNI, and twist-bend nematic–nematic, TNTBN, transition temperatures on the total spacer length, s, for the odd-membered dimers of the CBSnOCT and CBSnCT series are compared in Fig. 8. The CBSnOCT series shows higher values of TNI than the corresponding members of the CBSnCT series and this may be accounted for in terms of their molecular shapes, see Fig. 9. Using the trapezium method that we have reported previously,21 the bend angle of CBS6CT is estimated to be 124.7°, some 14° smaller than that of CBS5OCT. The smaller bend angle seen for the CBSnCT dimers may be attributed to the difference in bond angles associated with the methylene and ether linkages as described earlier. This trend in TNI is wholly consistent with the work of Ferrarini et al.106 who used a molecular field theory to estimate the values of TNI in dimers by exclusively varying the magnitude of the bond angle between the spacer and the mesogenic units. The difference in the values of TNI between corresponding members of the two series decreases on increasing s and this is associated with the greater number of conformations available to the spacer which reduces the difference in their shapes. The greater structural anisotropy of the CBSnOCT dimers is also manifested in higher values of ΔSNI/R than seen for the corresponding members of the CBSnCT series. As we noted earlier, the dimers with overall even-membered spacers show higher values of both TNI and ΔSNI/R than those of the adjacent odd members in both series, and this is a consequence of the more linear nature of the even-membered dimers.
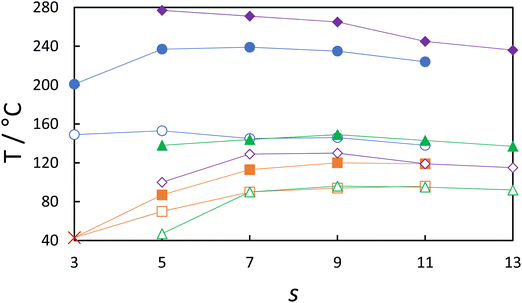 |
| Fig. 8 Dependence of transition temperatures on the total spacer length, s, for the CBSnOCT (purple diamonds) CBSnCT (blue circles), CBSnOCB (green triangles) and CBSnCB (orange squares and red cross) series, where s is the total number of atoms linking the cyanobiphenyl and cyanoterphenyl units, i.e. s = n + 2 for the CBSnOCT and CBSnOCB series and s = n + 1 for the CBSnCT and CBSnCB series. The filled symbols denote TNI and the open symbols TNTBN and the cross denoting TNTBI. | |
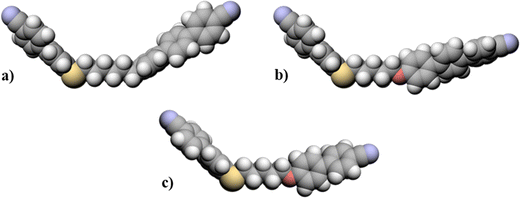 |
| Fig. 9 Space-filling models comparing the molecular shapes of (a) CBS6CT, (b) CBS5OCT and (c) CBS5OCB. | |
In contrast to the behaviour seen for TNI, the values of TNTBN shown by the odd members of the CBSnCT series are higher than those of the corresponding members of the CBSnOCT series. This reflects the more bent shape of the former dimers, and that the N–NTB phase transition is predominantly shape driven but also that both series have a similar degree of biaxiality endowed by the sulfur in the spacer.
Also shown in Fig. 8 are the values of TNI, TNTBN and TNTBI for the corresponding cyanobiphenyl-based CBSnOCB12,13 and CBSnCB31,57 series. As would be expected, the values of TNI are considerably higher for the dimers containing a cyanoterphenyl fragment. This may be attributed to the enhanced interaction strength parameter associated with the cyanoterphenyl fragment, i.e., the strength of the intermolecular interactions such as London dispersion forces which exist between the mesogenic units is increased with the additional aromatic ring compared to a cyanobiphenyl fragment due to the more anisotropic π-conjugated moiety present, and the increase in molecular shape anisotropy. The differences in TNI between these series are similar in magnitude to those reported previously on replacing a biphenyl with a terphenyl fragment.26,95 The values of TNTBN are also higher for the dimers containing cyanoterphenyl although the differences are not so large. Presumably this reflects that the shapes of these molecules are rather similar, see Fig. 8, and this is predominantly a shape-driven transition, but, as noted earlier, the interaction strength parameter associated with the cyanoterphenyl is larger than that of the cyanobiphenyl moiety and this will increase TNTBN. This smaller difference may be somewhat surprising considering that the enhancement in the intermolecular interactions should be consistent for both transitions suggesting that it is the driving forces of these phases causing the difference in the transition temperatures. Namely, that the transition temperature for the conventional nematic phase is increased when the molecule has a greater degree of linearity whereas for the NTB phase molecular bend is the driving force and so a more bent molecular structure is preferred and this effect was similarly described by Shimoura et al. with regards to the CBnCT series.94 The increase in TNTBN, as seen for the members of the CBSnCT series, does allow CBS6CT, CBS8CT and CBS10CT to all exhibit enantiotropic NTB–N phase transitions. This is despite the presence of a sulfur atom which normally leads to more monotropic phase behaviour, further highlighting the effect of the cyanoterphenyl moiety on the transition temperatures.
An apparent exception to this general behaviour is the values of TNTBN shown by CBS2CT and CBS4CT that appear higher than would be expected. This is particularly true for CBS2CT and suggests that its molecular shape may differ from those having longer spacers. We investigated this possibility by calculating the probabilities of differing bend angles for these dimers using a conformational GUI tool developed by Mandle et al.35 The histogram plots for CBS2CT and CB6SCT are shown in Fig. 10. CBS6CT shows a monomodal bend angle distribution with a most common value of around 110°, as would be expected for an odd-parity dimer. By comparison, CBS2CT shows a bimodal distribution with a significant fraction of highly bent, hairpin-like conformations. These extremely bent conformations have been discussed previously107,108 and presumably contribute to the higher values of TNTBN than would otherwise be expected. Their presence is also consistent with the optical birefringence of the CBS2CT being higher than that of the longer homologues but as was discussed earlier this may also be attributed to the progressive dilution of the highly anisotropic biphenyl and terphenyl cores with less anisotropic aliphatic chains of increasing length. The large increase in TNI on passing from CBS2CT to CBS4CT presumably also reflects these bent conformations exhibited by the former that would serve to reduce TNI. On increasing the spacer length these conformations are no longer observed, see Fig. 10.
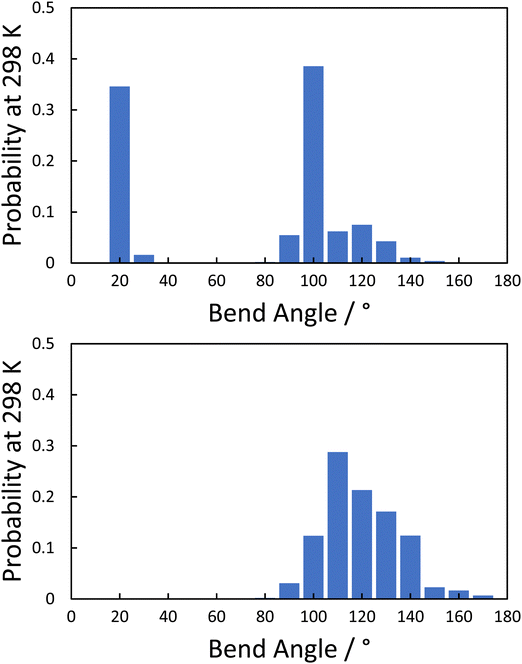 |
| Fig. 10 Histogram plots of the probability of a given bend angle for (top) CBS2CT and (bottom) CBS6CT. | |
Conclusions
The introduction of a cyanoterphenyl fragment in place of a cyanobiphenyl unit in the CBSnCB and CBSnOCB series, to give the CBSnCT and CBSnOCT series, respectively, increased the values of both TNI and TNTBN. The values of TNI for the cyanoterphenyl-based odd-membered dimers increased by over 100 K compared to those of the corresponding cyanobiphenyl-based dimers, whereas the values of TNTBN increase much more modestly. However, this does allow CBS6CT, CBS8CT and CBS10CT to all exhibit enantiotropic NTB–N phase transitions. This reflects that the shapes of the dimers are rather similar and the increase in TNI may be attributed to the higher strength interaction parameter associated with cyanoterphenyl. An exception to this general behaviour is CBS2CT for which the value of TNI appears lower whereas that of TNTBN appears higher than expected. This is attributed to presence of highly bent conformations that promote the formation of the NTB phase. While the transition temperatures of these dimers are still too high to be used for applications on their own, the very high birefringence values observed does give them a greater degree of potential if used in mixtures, for example, in the electrically controlled selective reflection of light, electrically tunable lasers and in beam steering applications.109–111
Data availability
The data supporting this article have been included as part of the ESI.†
Conflicts of interest
There are no conflicts of interest to declare.
Acknowledgements
Many thanks to Dr Richard Mandle for many helpful discussions in particular on the use of the conformer GUI tool. We would like to dedicate this work to Professor Corrie Imrie who tragically passed away in January 2025 during the submission process of this work and whose contribution was instrumental both to the conceptualisation of this research but also to the article which has been produced.
References
- M. Cestari, S. Diez-Berart, D. A. Dunmur, A. Ferrarini, M. R. De La Fuente, D. J. B. Jackson, D. O. Lopez, G. R. Luckhurst, M. A. Perez-Jubindo, R. M. Richardson, J. Salud, B. A. Timimi and H. Zimmermann, Phys. Rev. E, 2011, 84, 031704 CrossRef CAS PubMed.
- R. J. Mandle, Molecules, 2022, 27, 2689 CrossRef CAS PubMed.
- C. Tschierske, Liq. Cryst., 2018, 45, 2221–2252 CrossRef CAS.
- C. T. Imrie, R. Walker, J. M. D. Storey, E. Gorecka and D. Pociecha, Crystals, 2022, 12, 1245 CrossRef CAS.
-
R. B. Meyer, in Molecular Fluids: Les Houches Summer School in Theoretical Physics 1973, ed. R. Balian and G. Weil, Gordon and Breach, New York, 1976, pp. 273–373 Search PubMed.
- I. Dozov, Europhys. Lett., 2001, 56, 247–253 CrossRef CAS.
- V. Borshch, Y. K. Kim, J. Xiang, M. Gao, A. Jákli, V. P. Panov, J. K. Vij, C. T. Imrie, M. G. Tamba, G. H. Mehl and O. D. Lavrentovich, Nat. Commun., 2013, 4, 2635 CrossRef CAS PubMed.
- R. Walker, D. Pociecha, G. J. Strachan, J. M. D. Storey, E. Gorecka and C. T. Imrie, Soft Matter, 2019, 15, 3188–3197 RSC.
- R. J. Mandle, C. T. Archbold, J. P. Sarju, J. L. Andrews and J. W. Goodby, Sci. Rep., 2016, 6, 36682 CrossRef PubMed.
- K. Adlem, M. Čopič, G. R. Luckhurst, A. Mertelj, O. Parri, R. M. Richardson, B. D. Snow, B. A. Timimi, R. P. Tuffin and D. Wilkes, Phys. Rev. E, 2013, 88, 022503 CrossRef CAS PubMed.
- C. Greco, G. R. Luckhurst and A. Ferrarini, Soft Matter, 2014, 10, 9318–9323 RSC.
- E. Cruickshank, M. Salamończyk, D. Pociecha, G. J. Strachan, J. M. D. Storey, C. Wang, J. Feng, C. Zhu, E. Gorecka and C. T. Imrie, Liq. Cryst., 2019, 46, 1595–1609 CrossRef CAS.
- Y. Arakawa, K. Komatsu and H. Tsuji, New J. Chem., 2019, 43, 6786–6793 RSC.
- D. A. Paterson, J. P. Abberley, W. T. A. Harrison, J. M. D. Storey and C. T. Imrie, Liq. Cryst., 2017, 44, 127–146 CAS.
- Y. Arakawa, K. Komatsu, S. Inui and H. Tsuji, J. Mol. Struct., 2020, 1199, 126913 CrossRef CAS.
- R. J. Mandle, E. J. Davis, C. C. A. Voll, C. T. Archbold, J. W. Goodby and S. J. Cowling, Liq. Cryst., 2015, 42, 688–703 CAS.
- E. Forsyth, D. A. Paterson, E. Cruickshank, G. J. Strachan, E. Gorecka, R. Walker, J. M. D. Storey and C. T. Imrie, J. Mol. Liq., 2020, 320, 114391 CrossRef CAS.
- A. Lesac, U. Baumeister, I. Dokli, Z. Hameršak, T. Ivšić, D. Kontrec, M. Viskić, A. Knežević and R. J. Mandle, Liq. Cryst., 2018, 45, 1101–1110 CrossRef CAS.
- W. D. Stevenson, H. X. Zou, X. B. Zeng, C. Welch, G. Ungar and G. H. Mehl, Phys. Chem. Chem. Phys., 2018, 20, 25268–25274 RSC.
- Y. Arakawa, Y. Ishida, K. Komatsu, Y. Arai and H. Tsuji, Tetrahedron, 2021, 95, 132351 CrossRef CAS.
- E. Cruickshank, G. J. Strachan, K. Thapa, D. Pociecha, M. Salamończyk, J. M. D. Storey, E. Gorecka, O. D. Lavrentovich and C. T. Imrie, Liq. Cryst., 2024, 51, 1446–1470 CrossRef CAS.
- R. Walker, D. Pociecha, J. M. D. Storey, E. Gorecka and C. T. Imrie, Chem. – Eur. J., 2019, 25, 13329–13335 CrossRef CAS PubMed.
- D. A. Paterson, C. A. Crawford, D. Pociecha, R. Walker, J. M. D. Storey, E. Gorecka and C. T. Imrie, Liq. Cryst., 2018, 45, 2341–2351 CrossRef CAS.
- J. P. Abberley, R. Walker, J. M. D. Storey and C. T. Imrie, Liq. Cryst., 2020, 47, 1232–1245 CrossRef CAS.
- E. Cruickshank, R. Walker, G. J. Strachan, C. H. F. Goode, M. M. Majewska, D. Pociecha, E. Gorecka, J. M. D. Storey and C. T. Imrie, J. Mol. Liq., 2023, 391, 123226 CrossRef CAS.
- R. Walker, D. Pociecha, J. M. D. Storey, E. Gorecka and C. T. Imrie, J. Mater. Chem. C, 2021, 9, 5167–5173 RSC.
- E. Cruickshank, K. Anderson, J. M. D. Storey, C. T. Imrie, E. Gorecka, D. Pociecha, A. Makal and M. M. Majewska, J. Mol. Liq., 2022, 346, 118180 CrossRef CAS.
- A. F. Alshammari, A. Zattarin, A. Pearson, E. Cruickshank, M. Majewska, D. Pociecha, J. M. D. Storey, E. Gorecka, C. T. Imrie and R. Walker, Liq. Cryst., 2024, 51, 2300–2312 CrossRef CAS.
- D. A. Paterson, M. Gao, Y.-K. Kim, A. Jamali, K. L. Finley, B. Robles-Hernández, S. Diez-Berart, J. Salud, M. R. de la Fuente, B. A. Timimi, H. Zimmermann, C. Greco, A. Ferrarini, J. M. D. Storey, D. O. López, O. D. Lavrentovich, G. R. Luckhurst and C. T. Imrie, Soft Matter, 2016, 12, 6827–6840 RSC.
- Y. Arakawa, T. Shiba and K. Igawa, Liq. Cryst., 2024, 51, 1506–1522 CrossRef CAS.
- Y. Arakawa, K. Komatsu, T. Shiba and H. Tsuji, Mater. Adv., 2021, 2, 1760–1773 RSC.
- G. J. Strachan, W. T. A. Harrison, J. M. D. Storey and C. T. Imrie, Phys. Chem. Chem. Phys., 2021, 23, 12600–12611 RSC.
- J. P. Abberley, S. M. Jansze, R. Walker, D. A. Paterson, P. A. Henderson, A. T. M. Marcelis, J. M. D. Storey and C. T. Imrie, Liq. Cryst., 2017, 44, 68–83 CAS.
- A. F. Alshammari, D. Pociecha, R. Walker, J. M. D. Storey, E. Gorecka and C. T. Imrie, Soft Matter, 2022, 18, 4679–4688 RSC.
- J. L. Hobbs, C. J. Gibb, E. Cruickshank, R. Walker and R. J. Mandle, Liq.
Cryst., 2024, 51, 1022–1034 CrossRef CAS.
- Y. Arakawa, K. Komatsu, Y. Ishida, T. Shiba and H. Tsuji, Materials, 2022, 15, 1709 CrossRef CAS PubMed.
- M. R. Tuchband, D. A. Paterson, M. Salamończyk, V. A. Norman, A. N. Scarbrough, E. Forsyth, E. Garcia, C. Wang, J. M. D. Storey, D. M. Walba, S. Sprunt, A. Jákli, C. Zhu, C. T. Imrie and N. A. Clark, Proc. Natl. Acad. Sci. U. S. A., 2019, 116, 10698–10704 CrossRef CAS PubMed.
- R. J. Mandle and J. W. Goodby, ChemPhysChem, 2016, 17, 967–970 CrossRef CAS PubMed.
- W. D. Stevenson, J. An, X. B. Zeng, M. Xue, H. X. Zou, Y. S. Liu and G. Ungar, Soft Matter, 2018, 14, 3003–3011 RSC.
- F. P. Simpson, R. J. Mandle, J. N. Moore and J. W. Goodby, J. Mater. Chem. C, 2017, 5, 5102–5110 RSC.
- R. J. Mandle and J. W. Goodby, Angew. Chem., Int. Ed., 2018, 57, 7096–7100 CrossRef CAS PubMed.
- R. J. Mandle, Chem. Rec., 2018, 18, 1341–1349 CrossRef CAS PubMed.
- M. G. Tamba, U. Baumeister, G. Pelzl and W. Weissflog, Ferroelectrics, 2014, 468, 52–76 CrossRef CAS.
- S. P. Sreenilayam, Y. P. Panarin, J. K. Vij, V. P. Panov, A. Lehmann, M. Poppe, M. Prehm and C. Tschierske, Nat. Commun., 2016, 7, 11369 CrossRef CAS PubMed.
- S. P. Sreenilayam, V. P. Panov, J. K. Vij and G. Shanker, Liq. Cryst., 2017, 44, 244–253 CAS.
- S. M. Jansze, A. Martínez-Felipe, J. M. D. Storey, A. T. M. Marcelis and C. T. Imrie, Angew. Chem., Int. Ed., 2015, 127, 653–656 CrossRef.
- R. Walker, D. Pociecha, A. Martinez-Felipe, J. M. D. Storey, E. Gorecka and C. T. Imrie, Crystals, 2020, 10, 175 CrossRef CAS.
- R. Walker, D. Pociecha, M. Salamończyk, J. M. D. Storey, E. Gorecka and C. T. Imrie, Mater. Adv., 2020, 1, 1622–1630 RSC.
- R. Walker, D. Pociecha, J. P. Abberley, A. Martínez-Felipe, D. A. Paterson, E. Forsyth, G. Lawrence, P. A. Henderson, J. M. D. Storey, E. Gorecka and C. T. Imrie, Chem. Commun., 2018, 54, 3383–3386 Search PubMed.
- R. Walker, D. Pociecha, C. A. Crawford, J. M. D. Storey, E. Gorecka and C. T. Imrie, J. Mol. Liq., 2020, 303, 112630 CrossRef.
- E. Gorecka, N. Vaupotič, A. Zep, D. Pociecha, J. Yoshioka, J. Yamamoto and H. Takezoe, Angew. Chem., Int. Ed., 2015, 54, 10155–10159 CrossRef CAS PubMed.
- R. Walker, D. Pociecha, M. Salamonczyk, J. M. D. Storey, E. Gorecka and C. T. Imrie, ChemPhysChem, 2023, 24, e202200807 CrossRef CAS PubMed.
- A. Ožegović, A. Knežević, J. Novak, S. Šegota, P. Davidson and A. Lesac, ChemPhysChem, 2024, 25, e202400065 CrossRef PubMed.
- N. Tufaha, C. J. Gibb, J. M. D. Storey and C. T. Imrie, Liq. Cryst., 2023, 50, 1362–1374 CrossRef CAS.
- A. A. Dawood, M. C. Grossel, G. R. Luckhurst, R. M. Richardson, B. A. Timimi, N. J. Wells and Y. Z. Yousif, Liq. Cryst., 2016, 43, 2–12 CrossRef CAS.
- A. A. Dawood, M. C. Grossel, G. R. Luckhurst, R. M. Richardson, B. A. Timimi, N. J. Wells and Y. Z. Yousif, Liq. Cryst., 2017, 44, 106–126 CAS.
- Y. Arakawa, Y. Arai, K. Horita, K. Komatsu and H. Tsuji, Crystals, 2022, 12, 1734 CrossRef CAS.
- Y. Arakawa, K. Horita and K. Igawa, Liq. Cryst., 2023, 50, 2216–2228 Search PubMed.
- D. Wang, J. Liu, W. Zhao, Y. Zeng, J. Huang, J. Fang and D. Chen, Chem. – Eur. J., 2022, 28, e202202146 CrossRef CAS PubMed.
- M. Hird, A. J. Seed, K. J. Toyne, J. W. Goodby, G. W. Gray and D. G. McDonnell, J. Mater. Chem., 1993, 3, 851–859 RSC.
- Y. Arakawa, Y. Sasaki, N. Haraguchi, S. Itsuno and H. Tsuji, Liq. Cryst., 2018, 45, 821–830 CrossRef CAS.
- Y. Arakawa and H. Tsuji, Mol. Cryst. Liq. Cryst., 2017, 647, 422–429 CrossRef CAS.
- Y. Arakawa and H. Tsuji, J. Mol. Liq., 2019, 289, 111097 CrossRef.
- E. Cruickshank, G. J. Strachan, J. M. D. Storey and C. T. Imrie, J. Mol. Liq., 2021, 346, 117094 CrossRef.
- Y. Arakawa, Y. Ishida, Y. Sasaki, S. Sasaki, M. Tokita and H. Tsuji, Mater. Adv., 2022, 3, 3218–3228 RSC.
- Y. Arakawa, Y. Ishida, T. Shiba, K. Igawa, S. Sasaki and H. Tsuji, CrystEngComm, 2022, 24, 1877–1890 RSC.
- Z. Raszewski, W. Piecek, L. Jaroszewicz, L. Soms, J. Marczak, E. Nowinowski-Kruszelnicki, P. Perkowski, J. Kedzierski, E. Miszczyk, M. Olifierczuk, P. Morawiak and R. Mazur, J. Appl. Phys., 2013, 114, 53104 CrossRef.
- Z. Raszewski, W. Piecek, L. Jaroszewicz, E. Nowinowski-Kruszelnicki, P. Perkowski, L. Soms, R. Dabrowski, J. Kedzierski, M. Olifierczuk, M. Mrukiewicz, E. Miszczyk, P. Morawiak, R. Mazur and K. Kowiorski, Adv. Mater. Res., 2014, 909, 12–18 Search PubMed.
- H. R. H. R. Stapert, S. Del Valle, E. J. K. E. J. K. Verstegen, B. M. I. B. M. I. Van der Zande, J. Lub and S. Stallinga, Adv. Funct. Mater., 2003, 13, 732–738 CrossRef CAS.
- I. M. Syed, S. Kaur, H. E. Milton, D. Mistry, J. Bailey, P. B. Morgan, J. Cliff Jones, H. F. Gleeson, H. E. Milton, P. B. Morgan, J. H. Clamp, H. F. Gleeson, J. C. Jones and H. F. Gleeson, Opt. Express, 2015, 23, 9911–9916 CrossRef CAS PubMed.
- N. Bennis, T. Jankowski, O. Strzezysz, A. Pakua, D. C. Zografopoulos, P. Perkowski, J. M. Sánchez-Pena, J. M. López-Higuera and J. F. Algorri, Sci. Rep., 2022, 12, 012107 CrossRef PubMed.
- M. Reuter, N. Vieweg, B. M. Fischer, M. Mikulicz, M. Koch, K. Garbat and R. D
browski, APL Mater., 2013, 1, 012107 CrossRef.
- H. Coles and S. Morris, Nat. Photonics, 2010, 4, 676–685 CrossRef CAS.
- G. Si, Y. Zhao, E. S. P. Leong and Y. J. Liu, Materials, 2014, 7, 1296 CrossRef CAS PubMed.
- N. Kawatsuki, A. Yamashita, M. Kondo, T. Matsumoto, T. Shioda, A. Emoto and H. Ono, Polymer, 2010, 51, 2849–2856 CrossRef CAS.
- Y. Arakawa, S. Inui and H. Tsuji, Tetrahedron, 2022, 122, 132958 CrossRef CAS.
- Y. Arakawa, S. Inui, K. Igawa and H. Tsuji, Liq. Cryst., 2019, 46, 1621–1630 CrossRef CAS.
- G. Stepanafas, E. Cruickshank, S. Brown, M. M. Majewska, D. Pociecha, E. Gorecka, J. M. D. Storey and C. T. Imrie, Mater. Adv., 2023, 5, 525–538 RSC.
- M. Alaasar, C. Anders, R. Pashameah and A. F. Darweesh, Liq. Cryst., 2023, 50, 2397–2412 CrossRef CAS.
- R. Saha, C. Feng, C. Welch, G. H. Mehl, J. Feng, C. Zhu, J. Gleeson, S. Sprunt and A. Jákli, Phys. Chem. Chem. Phys., 2021, 23, 4055–4063 RSC.
- A. J. Seed, K. J. Toyne and J. W. Goodby, J. Mater. Chem., 1995, 5, 653–661 RSC.
- A. Seed, Chem. Soc. Rev., 2007, 36, 2046–2069 RSC.
- H. A. Ahmed and A. Aboelnaga, Liq. Cryst., 2022, 49, 804–811 CrossRef CAS.
- M. Wang, H. Song, C. Wu, B. Liu, Z. Wang and H. Yang, Crystals, 2023, 13, 916 CrossRef CAS.
- G. J. Cross, A. J. Seed, K. J. Toyne, J. W. Goodby, M. Hird and M. C. Artal, J. Mater. Chem., 2000, 10, 1555–1563 RSC.
- R. J. Mandle, Liq. Cryst., 2023, 50, 534–542 CrossRef CAS.
- A. J. Seed, K. J. Toyne, J. W. Goodby and D. G. McDonnell, J. Mater. Chem., 1995, 5, 1–11 RSC.
- Z. Fang and C. Wu, Liq. Cryst., 2020, 47, 1086–1099 CrossRef CAS.
- A. U. Petersen, M. Jevric, R. J. Mandle, M. D. Kilde, F. P. Jørgensen, J. W. Goodby and M. B. Nielsen, Aust. J. Chem., 2018, 71, 422–434 CrossRef CAS.
- Y. Arakawa and Y. Arai, Materials, 2024, 17, 3278 CrossRef CAS PubMed.
- E. E. Pocock, R. J. Mandle and J. W. Goodby, Soft Matter, 2018, 14, 2508–2514 RSC.
- R. J. Mandle, M. P. Stevens and J. W. Goodby, Liq. Cryst., 2017, 44, 2046–2059 CrossRef CAS.
- G. J. Strachan, M. M. Majewska, D. Pociecha, J. M. D. Storey and C. T. Imrie, ChemPhysChem, 2023, 24, e202200758 CrossRef CAS PubMed.
- Y. Shimoura and Y. Arakawa, Crystals, 2025, 15, 120 CrossRef.
- R. Walker, M. Majewska, D. Pociecha, A. Makal, J. M. Storey, E. Gorecka and C. T. Imrie, ChemPhysChem, 2020, 22, 461–470 CrossRef PubMed.
- H. Cao-Cen, J. Zhao, L. Qiu, D. Xu, Q. Li, X. Chen and F. Yan, J. Mater. Chem., 2012, 22, 12842–12850 RSC.
-
M. J. Frisch, G. W. Trucks, H. B. Schlegel, G. E. Scuseria, M. A. Robb, J. R. Cheeseman, G. Scalmani, V. Barone, B. Mennucci, G. A. Petersson, H. Nakatsuji, M. Caricato, X. Li, H. P. Hratchian, A. F. Izmaylov, J. Bloino, G. Zheng, J. L. Sonnenberg, M. Hada, M. Ehara, K. Toyota, R. Fukuda, J. Hasegawa, M. Ishida, T. Nakajima, Y. Honda, O. Kitao, H. Nakai, T. Vreven, J. A. Montgomery, J. E. Peralta, F. Ogliaro, M. Bearpark, J. J. Heyd, E. Brothers, K. N. Kudin, V. N. Staroverov, R. Kobayashi, J. Normand, K. Raghavachari, A. Rendell, J. C. Burant, S. S. Iyengar, J. Tomasi, M. Cossi, N. Rega, J. M. Millam, M. Klene, J. E. Knox, J. B. Cross, V. Bakken, C. Adamo, J. Jaramillo, R. Gomperts, R. E. Stratmann, O. Yazyev, A. J. Austin, R. Cammi, C. Pomelli, J. W. Ochterski, R. L. Martin, K. Morokuma, V. G. Zakrzewski, G. A. Voth, P. Salvador, J. J. Dannenberg, S. Dapprich, A. D. Daniels, O. Farkas, J. B. Foresman, J. V. Ortiz, J. Cioslowski and D. J. Fox, Gaussian 09, Revis. B.01, Gaussian, Inc., Wallingford CT, 2010 Search PubMed.
- M. Tarini, P. Cignoni and C. Montani, IEEE Trans. Vis. Comput. Graph., 2006, 12, 1237–1244 Search PubMed.
-
G. A. Landrum, 2016.
- S. Wang, J. Witek, G. A. Landrum and S. Riniker, J. Chem. Inf. Model., 2020, 60, 2044–2058 CrossRef CAS PubMed.
- S. Riniker and G. A. Landrum, J. Chem. Inf. Model., 2015, 55, 2562–2574 CrossRef CAS PubMed.
- M. J. Vainio and M. S. Johnson, J. Chem. Inf. Model., 2007, 47, 2462–2474 CrossRef CAS PubMed.
- P. Sathyanarayana, M. Mathew, Q. Li, V. S. S. Sastry, B. Kundu, K. V. Le, H. Takezoe and S. Dhara, Phys. Rev. E: Stat., Nonlinear, Soft Matter Phys., 2010, 81, 010702 CrossRef CAS PubMed.
- S. Gauza, J. Li, S. T. Wu, A. Spadło, R. D
browski, Y. N. Tzeng and K. L. Cheng, Liq. Cryst., 2005, 32, 1077–1085 CrossRef CAS.
- D. Pociecha, C. A. Crawford, D. A. Paterson, J. M. D. Storey, C. T. Imrie, N. Vaupotič and E. Gorecka, Phys. Rev. E, 2018, 98, 052706 CrossRef CAS.
- A. Ferrarini, G. R. Luckhurst, P. L. Nordio and S. J. Roskilly, J. Chem. Phys., 1994, 100, 1460–1469 CrossRef CAS.
- C. T. Archbold, R. J. Mandle, J. L. Andrews, S. J. Cowling and J. W. Goodby, Liq. Cryst., 2017, 44, 2079–2088 CAS.
- G. Yu and M. R. Wilson, Soft Matter, 2022, 18, 3087–3096 RSC.
- J. Xiang, Y. Li, Q. Li, D. A. Paterson, J. M. D. Storey, C. T. Imrie and O. D. Lavrentovich, Adv. Mater., 2015, 27, 3014–3018 CrossRef CAS PubMed.
- J. Xiang, A. Varanytsia, F. Minkowski, D. A. Paterson, J. M. D. Storey, C. T. Imrie, O. D. Lavrentovich and P. Palffy-Muhoray, Proc. Natl. Acad. Sci. U. S. A., 2016, 113, 12925–12928 CrossRef CAS PubMed.
- N. Vaupotič, M. Ali, P. W. Majewski, E. Gorecka and D. Pociecha, ChemPhysChem, 2018, 19, 2566–2571 CrossRef PubMed.
Footnotes |
† Electronic supplementary information (ESI) available. See DOI: https://doi.org/10.1039/d4cp04189e |
‡ Present address: School of Pharmacy, Applied Sciences and Public Health, Robert Gordon University, Aberdeen, AB10 7GJ, UK. |
§ Deceased 14th January 2025. |
|
This journal is © the Owner Societies 2025 |
Click here to see how this site uses Cookies. View our privacy policy here.