DOI:
10.1039/D4TC00109E
(Paper)
J. Mater. Chem. C, 2024,
12, 4261-4266
Poly(3,4-ethylenedioxythiophene)/poly(bis(4-phenoxysulfonic acid)phosphazene) conductive composites: an alternative interfacial layer to PEDOT
:
PSS†
Received
9th January 2024
, Accepted 25th February 2024
First published on 26th February 2024
Introduction
Among the family of conjugated conducting polymers, poly(3,4-ethylenedioxythiophene) (PEDOT) is one of the most studied due to its unique properties such as high transparency in the visible region, high environmental stability, and tunable conductivity.1 Although it was initially designed as a solution-processable material, it was found to be insoluble in water or common organic solvents. PEDOT's solution processability problem could be overcome by the incorporation of the water-soluble polyanionic charge balancer poly(styrene sulfonic acid) (PSS) which allows the dispersion of the whole macromolecular salt system (PEDOT
:
PSS) in water.2 Since solution processability was achieved, PEDOT
:
PSS has become a prominent material for a number of applications including organic electrodes,3 batteries4 and supercapacitors,5 and artificial muscle applications.6 However, the acidic and hygroscopic nature of PSS itself brought some unwanted properties to the final conducting material.7 For example, if PEDOT
:
PSS is used between a transparent conducting electrode, usually indium-doped tin oxide (ITO), and the active layer in an OLED, PSS etches the ITO which results in migration of indium and tin to the inner layers.8,9 This results in the degradation of the device and shortened device lifetime, which is an issue that must be overcome to compete with inorganic semiconductor-based technologies. In addition to its hygroscopicity and acidity, PSS is prone to degradation in the presence of radicals,10–12 or oxidants,13 while PEDOT is usually synthesised inside an aqueous solution of PSS in the presence of very strong oxidants such as ammonium persulfate14 or sodium persulfate.15,16 Therefore, a polymer backbone with a high oxidative stability is highly sought to cope with the instability problems. In contrast to styrene-based polymers, poly(aryloxyphosphazenes) are viable alternatives with some unique properties.17 Poly(phosphazenes) have alternating P and N atoms on the main chain which are in their highest oxidation state and thus the poly(phosphazene) backbone is highly resistant to thermal and chemical oxidative conditions.17 Whilst poly(dichlorophosphazene) is unstable in a moist environment, it is an effective precursor for lots of different and highly stable poly(phosphazenes) by the macromolecular nucleophilic substitution of the chlorine atoms by alcohols, phenols, or nucleophilic amines in the presence of a base. Although it is typically challenging to synthesise sulfonic acid functionalised poly(aryloxyphosphazenes), some of us recently introduced a simple synthetic procedure for the preparation of fully or partially sulfonated poly(aryloxyphosphazenes) by covalent protection of the sulfonic acid moiety.18 This progress then led us to develop single-component water-soluble PEDOT-grafted sulfonic acid functionalised poly(aryloxyphosphazene) copolymers.19 However, growing PEDOT graft arms on another polymer requires a specially designed precursor which has thiophene anchors on its backbone, therefore it is not as simple to polymerise as EDOT in the presence of poly(bis(4-oxybenzene sulfonic acid)phosphazene), i.e., PSAP, which is the polyphosphazene analogue of the PSS.
In this study, we prepared a series of PEDOT
:
xPSAP composites by varying the EDOT feed ratio into aqueous solutions of PSAP which were then chemically oxidised to PEDOT by using a fixed oxidant-to-monomer ratio ((NH4)2S2O8, APS / EDOT of 6
:
5). Towards the application of PSAP in optoelectronic devices, we synthesised the poly(dichlorophosphazene) by living cationic polymerisation of the monomer Cl3P
NSiMe3, which leads to low dispersity (Đ) and controlled molecular weight (Mw) poly(phosphazenes), followed by functionalisation with 4-oxybenzene sulfonic acid.20 The resulting composites were then tested as an alternative hole transport layer (HTL) to PEDOT
:
PSS (Al 4083) on Super Yellow (SY)-based OLED devices with a configuration of indium-doped tin oxide (ITO)/HTL/SY/Ca/Al.
Results and discussion
The preparation of PEDOT
:
xPSAP macromolecular salt systems were very similar to the preparation of PEDOT
:
PSS, as depicted in Scheme 1. In this study the precursor, poly(dichlorophosphazene) (PDCP), was synthesised from the living cationic polymerisation of Cl3P
NSiMe3 as it gives quantitative conversion and an almost monodisperse polymer with a controlled molecular weight.21 The PDCP synthesised by this route had a Mw of 2.05 × 105 Da and Đ = 1.25 with a monomer-to-initiator (PCl5) ratio of 400/1, which was very similar to the literature value as determined by gel permeation chromatography or size exclusion chromatography measurements. Then, PDCP was successfully derivatised to poly(bis(4-oxybenzene sulfonic acid) phosphazene) (PSAP) in a two-step process: first, a nucleophilic substitution reaction of PDCP with ethyl 4-hydroxybenzene sulfonate in the presence of NaH and then conversion to sulfonic acid by treatment with dilute H2SO4.18 After purification of the PSAP by a few ethanol precipitations, a 2% (w/w) stock water solution was used for the chemical oxidative polymerisation of EDOT to PEDOT in the presence of ammonium peroxydisulfate (APS, (NH4)2S2O8). The EDOT feed ratio (w/w) was varied between 1
:
1 to 1
:
11 in odd-number intervals, whilst keeping the EDOT:APS stoichiometric ratio fixed as 1
:
1.2, to optimise the conductivity, optical, and morphological properties of the resulting PEDOT
:
xPSAP composites.
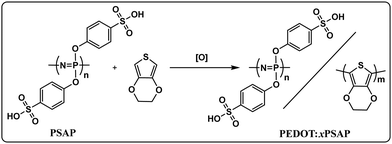 |
| Scheme 1 Synthetic pathway to PEDOT : xPSAP. | |
The PEDOT
:
xPSAP blends prepared using different EDOT feed ratios were studied using Fourier-transform infrared (FTIR) spectroscopy, as shown in Fig. 1. PSAP itself has characteristic vibration peaks of –OH, –CH and C
C stretching at 3460, 3100, and 1595 and 1493 cm−1, respectively. The strong vibration peaks at 1360 and 1042 cm−1, and 1122 cm−1 can be attributed respectively to S
O (symmetrical, and asymmetrical) and S–O stretching, whilst antisymmetric P–O stretching is observed at 934 cm−1. The spectrum of commercial PEDOT
:
PSS (Al 4083) has peaks at very similar frequencies with additional PEDOT aliphatic C–H stretching peaks at 2860 and 2915 cm−1, and C–S stretching peaks at 937, 808 and 675 cm−1 (Fig. 1). A prominent change in the spectra of the PEDOT
:
xPSAP composites can be seen between 2750 to 3365 cm−1. The –OH stretching moved to 3220 cm−1, which is an indication of intermolecular bonding between PSAP and PEDOT. Furthermore, the remaining free –OH stretching peak at 3460 cm−1 increases with the decreasing PEDOT ratio (Fig. 1). Since PSAP has no aliphatic groups and thus no aliphatic –CH stretching, the peaks between 2760 to 2890 cm−1 can be assigned to aliphatic –CH vibrations of PEDOT. The relative intensity of this peak increases with increasing amount of PEDOT in the PEDOT
:
xPSAP blends. Another apparent difference in the FTIR spectra of PEDOT
:
PSS and PEDOT
:
xPSAP compared to PSAP is found in the range between 1660 and 1306 cm−1. The two distinct bands at ∼1650 and 1404 cm−1, which have increased intensity with increasing PEDOT ratio in the PEDOT
:
xPSAP composites, can be attributed to the C
C and C–C stretching of PEDOT, also observed in the spectrum of commercial PEDOT
:
PSS (Al 4083).
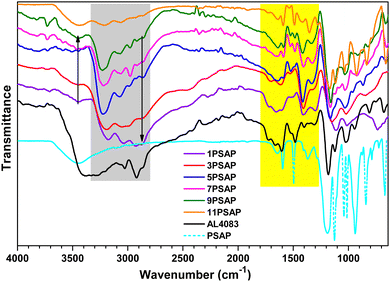 |
| Fig. 1 Solid state(film) FTIR spectra of PSAP, PEDOT : PSS and PEDOT : xPSAP samples. | |
The interaction of PEDOT with PSAP in PEDOT
:
xPSAPs was further investigated by UV-Vis absorption measurements (Fig. 2). The poly(phosphazene) backbone does not have absorption in the UV-Vis and NIR range and therefore its fully sulfonated benzene sulfonic acid derivative PSAP will not affect the optical properties of PEDOT. Fig. 2 shows a strong absorption band at 800 nm arising from the polaron transitions of PEDOT for all the materials. The absorption intensity increases by the increasing PEDOT ratio in PEDOT
:
xPSAPs. PEDOT
:
PSS, AL4083 grade, has a PEDOT-to-PSS ratio of 1
:
6 (w/w) and shows very similar absorption behaviour to PEDOT:5PSAP, which has the PEDOT-to-PSAP ratio of 1
:
5 (w/w). This difference is not surprising since PSS has one sulfonic acid per repeating unit of the polymer, whereas PSAP has two sulfonic acid moieties. The PEDOT polaron absorption in the NIR region of the spectra remains almost the same for all materials measured except PEDOT:1PSAP which has an absorption maximum at 925 nm, and then its absorption remains constant up to 1400 nm. Hence, it can be said that all the samples have high polaron concentration ensuring electrical conductivity whilst there is no sign of neutral PEDOT absorption around ∼600 nm.
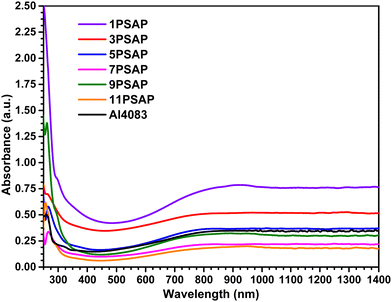 |
| Fig. 2 Comparative UV-Vis spectra of PEDOT : xPSAP and PEDOT : PSS (Al4083) samples in 0.2% water. | |
The similarity between PEDOT
:
PSS and PEDOT
:
xPSAPs is also evident in the surface morphology as shown from the tapping mode AFM images presented in Fig. 3. The PEDOT:1PSAP film shows some aggregates on the surface which may be caused by an insufficient amount of PSAP to stabilise the PEDOT dispersion in solution. On the other hand, the PEDOT:11PSAP film begins to show potential phase segregation, albeit the height difference between the domains and the bulk remains reasonably small. It can be expected that if the PSAP content were increased there would be further phase segregation. However, in general, the films show good uniformity and as such it can be expected that the PEDOT rich layers are homogenously distributed in the PEDOT
:
xPSAPs, emphasising the good film formation which may arise from the use of poly(aryloxyphosphazenes). The PEDOT
:
xPSAP water dispersions were stable at room temperature without any precipitation over 3 months and only the PEDOT:1PSAP had minor precipitated particles which could be redispersed simply by shaking. Hence, the PEDOT
:
xPSAP water dispersions have similar morphological features to PEDOT
:
PSS and may be an alternative to PEDOT
:
PSS for organic electronic device applications.
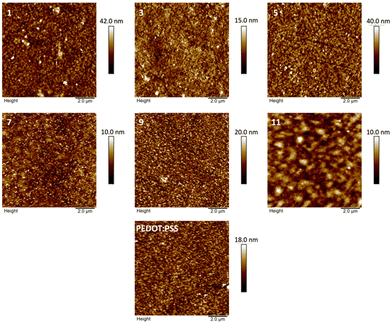 |
| Fig. 3 AFM topography images of PEDOT : xPSAP thin films. The root mean square roughness (Rq) for the films were as follows: PEDOT:1PSAP – 5.82 nm; PEDOT:3PSAP – 2.27 nm; PEDOT:5PSAP – 5.90 nm; PEDOT:7PSAP – 1.35 nm; PEDOT:9PSAP – 3.10 nm; PEDOT:11PSAP – 1.50 nm and PEDOT : PSS (Al4083) – 2.64 nm. | |
Thermal stability is another important property of interfacial layers for application in organic electronics which affects the device's lifetime. The TGA plots of the PEDOT
:
xPSAPs are given in Fig. 4 and the results have been summarised in Table 1. As can be seen from Fig. 4 and Table 1, most of the PEDOT
:
xPSAPs decompose over 250 °C except PEDOT:1PSAP which has the highest PEDOT content. Although it was proven that PSAP and its derivatives have high oxidative and thermal stability,17 the PEDOT
:
xPSAPs show lower thermal stability compared to PEDOT
:
PSS (Fig. 4). The decrease of thermal stability with the increase in PEDOT composition, or comparatively lower thermal stability of PEDOT
:
xPSAP at a similar PEDOT content to PEDOT
:
PSS, is modest and perhaps not particularly significant for most device applications. However, a possible explanation for the lower thermal stability of the PEDOT
:
xPSAPs may be the lack of radical traps in the chemically inert polyphosphazenes which cannot stabilise these species that evolved during the decomposition of PEDOT. Unlike PSAP, ortho- positions of the benzene ring of PSS are prone to abstract radicals which subsequently delocalise on to the α-position of the main polymer chain by stabilisation, and then chain scission takes place.12,13 It is possible that the presence of PEDOT, which already carries the radical polarons, may repair the PSS chain by donating electrons in a process similar to a previously observed cerium-mediated repair of poly(α-methyl styrene sulfonate) analogue.11 On the other hand, the radical species on the PEDOT may react with the radical carrying α-position of the main PSS chain to form a covalent bond and thus result in a more stable PEDOT adduct. Hence, this may result in a more thermally stable PEDOT
:
PSS material, and thus explains why the thermal decomposition rate of PEDOT
:
PSS is lower than PEDOT
:
xPSAPs at a higher (PEDOT:1PSAP and PEDOT:3PSAP) or similar PEDOT content, i.e., PEDOT:5PSAP&PEDOT:7PSAP.
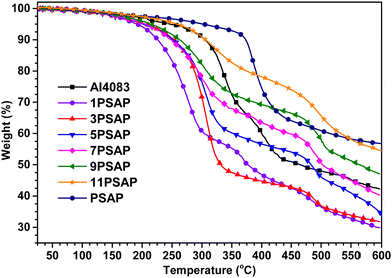 |
| Fig. 4 Comparative TGA results of PSAP, PEDOT : xPSAP and PEDOT : PSS. | |
Table 1 Selected thermal and conductivity data for PEDOT
:
PSS, PSAP and PEDOT
:
xPSAP
Material |
T
d (°C) |
% Residue |
R
s (Ω □−1) |
Conductivity (S cm−1) |
Room temperature ionic conductivity value from ref. 18.
|
PSAP |
373.9 |
56.8 |
— |
0.04a |
PEDOT:1PSAP |
225.6 |
29.8 |
4.9 × 102 |
2.4 × 10−1 |
PEDOT:3PSAP |
250.8 |
31.7 |
1.1 × 104 |
1.1 × 10−2 |
PEDOT:5PSAP |
251.2 |
34.8 |
1.0 × 105 |
1.1 × 10−3 |
PEDOT:7PSAP |
248.0 |
40.3 |
1.1 × 106 |
1.2 × 10−4 |
PEDOT:9PSAP |
254.9 |
46.9 |
1.2 × 106 |
1.1 × 10−4 |
PEDOT:11PSAP |
305.6 |
54.4 |
1.2 × 106 |
9.3 × 10−5 |
AL4083 |
304.6 |
42.3 |
1.1 × 106 |
9.6 × 10−5 |
Although PEDOT:1PSAP has lower thermal stability compared to PEDOT
:
PSS and other analogues in the series, it has an initial conductivity of 0.24 S cm−1 similar to high conductivity grade pristine PEDOT
:
PSS22 (Table 1). On the other hand, the conductivity decreases to 11 mS cm−1 in the PEDOT:3PSAP sample and continues to decrease with a decrease of PEDOT content in PEDOT:5PSAP, PEDOT:7PSAP PEDOT:9PSAP and PEDOT:11PSAP, which have a very similar conductivity to commercial Al4083.
Organic light-emitting diodes were fabricated using the PEDOT
:
xPSAP materials as hole transport layers (HTLs) in place of PEDOT
:
PSS. The device structure was as follows: ITO/HTL/Super Yellow/Ca/Al. A summary of the device performance achieved using different HTLs is shown in Table 2, while current density–voltage-luminance and external quantum efficiency (EQE) vs luminance plots are shown in Fig. 5 and Fig. S1–S7 (ESI†), respectively.
Table 2 Summary of OLED performance with PEDOT
:
xPSAP hole transport layers
HTL |
V
on (V) |
L
max (cd m−2) |
EQEmax (%) |
PEDOT:1PSAP |
2.6 |
28 100 |
1.40 |
PEDOT:3PSAP |
2.6 |
34 700 |
1.80 |
PEDOT:5PSAP |
2.5 |
55 300 |
2.14 |
PEDOT:7PSAP |
2.9 |
17 100 |
1.82 |
PEDOT:9PSAP |
2.5 |
61 300 |
2.20 |
PEDOT:11PSAP |
2.5 |
34 500 |
2.15 |
AL4083 |
2.7 |
65 300 |
1.81 |
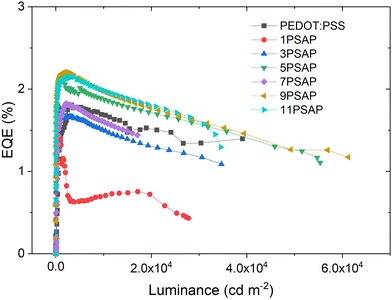 |
| Fig. 5 External quantum efficiency (EQE) vs. luminance plots for OLEDs containing PEDOT : PSS or PEDOT : xPSAP hole transport layers. | |
When the hole transport layer contains PEDOT:1PSAP or PEDOT:3PSAP, the EQE and maximum luminance are lower than observed for the equivalent PEDOT
:
PSS containing device. However, OLEDs PEDOT:5PSAP, PEDOT:7PSAP, PEDOT:9PSAP and PEDOT:11PSAP all show superior EQEs with respect to the PEDOT
:
PSS based analogue, although the maximum luminance is greatest in the latter. Optimum performance for OLEDs studying the PEDOT
:
xPSAP series as hole transport layers is achieved in terms of both maximum luminance and EQE for PEDOT:9PSAP. This can be attributed to a combination of factors, with a suitable conductivity ensuring better charge carrier balance in the emissive layer, better thermal stability and smooth surface topography. When the PSAP concentration is increased further, the apparent phase segregation (Fig. 3) begins to limit performance. Overall, we have highlighted how PSAP can be used effectively as a polyelectrolyte for PEDOT blends used as hole transport layers in organic light-emitting diodes.
Conclusion
In this manuscript we have reported the synthesis and application of PEDOT blends with poly(bis(4-phenoxysulfonic acid)phosphazene) (PSAP) polyelectrolyte. The PEDOT
:
PSAP films show a high polaron concentration and electrical conductivities comparable to the commercially available PEDOT
:
PSS blend (Al4083). The composite materials were applied in organic light-emitting diodes as hole transport layers, with devices containing PEDOT:5PSAP, PEDOT:9PSAP and PEDOT:11PSAP giving improved efficiency compared to a PEDOT
:
PSS-containing analogue. As a result, we have demonstrated that PSAP is a promising polyelectrolyte for use in conductive materials for organic electronics applications.
Experimental
Materials and methods
Ammonium peroxydisulfate (APS) was obtained from Alfa Aesar and 4-hydroxybenzenesulfonic acid hydrate (>85%) was obtained from Tokyo Chemical Industry Co. (TCI) and used without further purification. The deuterated solvents, CDCl3 and D2O for NMR spectroscopy and the following chemicals were obtained from Merck: NaH (60% suspension in mineral oil), ethanol, H2SO4 (98%), tetrahydrofuran (THF), 3,4-ethylenedioxythiophene (EDOT), PCl5 (98%) and triethyl orthoformate (98%). Super Yellow, poly(1,4-phenylenevinylene) (SY), was obtained from Sigma–Aldrich. PEDOT
:
PSS (AL4083) was obtained from Ossila Ltd. Deionised (DI) water (18 MΩ) was obtained from a Millipore water purification system. All other reagents and solvents were reagent grade quality and obtained from commercial suppliers.
Equipment
FT-IR spectra of the thin film samples of PEDOT
:
PSS and PEDOT
:
xPSAPs were recorded on a Jasco FT-IR 4100 spectrometer in attenuated total reflectance (ATR) mode in the range of 4000–650 cm−1. 1H, 13C, 31P NMR measurements were recorded in CDCl3 and D2O solutions on a Bruker 500 MHz spectrometer. UV-Vis spectra were recorded on a Shimadzu UV-2600 in the range of 250 to 1400 nm in 0.2% water solutions. Thermal properties of the samples were measured by using Netzsch TG209 F3 Tarsus TGA instrument between 25–600 °C at a 20 °C min−1 heating rate under constant flow of 30 mL N2. Atomic force microscopy (AFM) was carried out using a Bruker Innova AFM. DC conductivities of the PEDOT
:
PSAP thin films were measured by using a Signatone four-point probe with Keithley 2450 source meter. The conductivity of spin-cast PEDOT
:
xPSAP samples were calculated from the following equation,
where σ is the conductivity (S cm−1), t is the thickness (cm) of the sample (measured by AFM analysis) (cm), Rs is the sheet resistance (Ω □−1).
Synthesis
Poly(dichlorophosphazene) (PDCP) was synthesised from the room temperature living cationic condensation polymerisation of Cl3P
NSiMe3 with a monomer/initiator (PCl5) ratio of 400
:
1, according to literature procedure.2131P NMR (CDCl3) δ = −17.5 [br s, –Cl2![[P with combining low line]](https://www.rsc.org/images/entities/b_char_0050_0332.gif)
N–]. GPC/SEC (5% Bu4NBr, THF mobile phase) Mw = 2.05 × 105, PDI = 1.25.
Poly(bis(4-phenoxysulfonic acid)phosphazene) was synthesised by reacting PDCP with ethyl 4-hydroxybenzene sulfonate and subsequent transformation to free acid form according to the literature procedure.1831P NMR (D2O) δ = −20.5 [br s, 1P, –N =
(OPhSO3H)2]. 1H NMR (D2O) δ = 6.5 and 7.1 ppm [two br s, aromatic phenyl protons].
Preparation of poly(3,4-ethylenedioxythiophene)/poly(bis(4-phenoxysulfonic acid) phosphazene) (PEDOT
:
xPSAP) water dispersions.
Poly(bis(4-phenoxysulfonic acid) phosphazene) (PSAP) (2.0 g, 5.12 mmol) was dissolved in deionised water (100 mL) to obtain a 2% (w/w) stock solution and used for preparation of composites by varying the EDOT feed ratio (n/n). Specific reagent amounts are given in Table 3 and the general experimental procedure was as follows. A 10 mL aliquot of 2% PSAP solution in water was taken from the above stock solution in a round-bottomed 25 mL flask and the corresponding stoichiometric amount of EDOT (Table 3) was added under vigorous stirring. The mixture was stirred for half an hour and the calculated amount of oxidant (APS) in water was added slowly. The reaction turned light blue in 1 hour and was allowed to stir for 2 days. The resulting dark blue mixture was then filtered through a 0.45 μm PTFE syringe filter and labelled as PEDOT
:
xPSAP where x denotes the PSAP weight ratio. {1H}31P NMR (D2O) δ = −20.5 [br s, 1P,
(OPhR)2, R = –SO3H]. 1H NMR (D2O) δ = 6.5 and 7.1 ppm [two br s, aromatic phenyl protons], δ = 3.66 ppm [br s, –OC
2C
2O–, PEDOT]. 13C NMR (D2O) δ = 120.1 and 131.9 ppm (disubstituted benzene), 127.3 (br d) and 152.9 ppm (di substituted benzene ipso carbons), δ = 115.2 and 141.5 ppm (br s, quaternary C atoms of PEDOT), δ = 62.5, 63.9, 65.4 ppm [br m, –O
H2
H2O–, PEDOT].
Table 3 Used reagent amounts for the preparation of PEDOT
:
xPSAP composites
Material |
EDOT |
APS |
PSAP/EDOT Ratio (w/w) |
mg |
mmol |
mg |
mmol |
PEDOT:1PSAP |
50.0 |
0.35 |
96.3 |
0.42 |
1
|
PEDOT:3PSAP |
16.7 |
0.12 |
32.9 |
0.14 |
3
|
PEDOT:5PSAP |
10.0 |
0.07 |
19.3 |
0.08 |
5
|
PEDOT:7PSAP |
7.14 |
0.05 |
13.8 |
0.06 |
7
|
PEDOT:9PSAP |
5.56 |
0.04 |
10.7 |
0.05 |
9
|
PEDOT:11PSAP |
4.55 |
0.03 |
8.2 |
0.04 |
11
|
Device fabrication
OLED devices were fabricated on ITO substrates purchased from Kintec with a sheet resistance of 7 Ω □−1 and ITO thickness of 150 nm. The ITO substrates were cleaned under ultra-sonication using deionised water, acetone, and isopropanol consecutively for 10 minutes. The substrates were dried by blowing compressed air and then treated with oxygen plasma for 10 minutes. The PEDOT
:
xPSAPs and PEDOT
:
PSS (Heraeus) samples were filtered into 5 mL vials using a 0.45 μm PVDF filter and were spin coated onto the ITO substrates at 3000 rpm for 60 seconds using a Specialty Coating Systems G3P-8 spin coater and the films were annealed at 120 °C for 20 minutes. The films were then transferred to a glove box (MBraun) with controlled oxygen and moisture (O2 < 0.1 ppm, H2O < 0.1 ppm). Super Yellow (SY) solution (5 mg mL−1) in anhydrous toluene was prepared a day earlier and kept stirring at 40 °C to ensure complete dissolution of the polymer. Then the SY active layer was spin coated onto the PEDOT
:
xPSAP or PEDOT
:
PSS films using a spin coater at 1400 rpm for 60 seconds to obtain a thickness of ∼90 nm. 40 nm of Ca (Sigma Aldrich) and 60 nm of Al (Kurt J. Lesker) were respectively deposited by physical vapor deposition at a rate of 0.5 to 1 Å s−1, using an MBRAUN thermal evaporator at pressures of ∼10−6 mbar. The final OLED device structures were glass/ITO/HTL (PEDOT
:
xPSAP or PEDOT
:
PSS)/SY/Ca/Al and each OLED pixel had an area of 1.5 × 3.5 mm2. Current–voltage-luminance (IVL) of the devices were measured using a SCS4200 (Keithley) semiconductor characterisation system interfaced with a L203 luminance meter (Irradian).
Data availability
The raw data that supports this manuscript can be found at http://dx.doi.org/10.5525/gla.researchdata.1540.
Author contributions
E. B. Ç.: conceptualization, data curation, formal analysis, investigation, writing – original draft. J. C.: data curation, investigation, methodology, writing – original draft. P. J. S.: funding acquisition, project administration, supervision, writing – review & editing. F. H.: Conceptualisation, funding acquisition, project administration, supervision, writing – original draft.
Conflicts of interest
There are no conflicts to declare.
Acknowledgements
We greatly acknowledge the Royal Society for funding our research under the grant number NAF_R2_180625. We also acknowledge TUBITAK for their support for FH (grant number 113Z314) and EBC (2219-International Postdoctoral Research Fellowship). JC thanks the EPSRC for funding (grant number EP/T022477/1).
References
- Y. Jiang, T. Liu and Y. Zhou, Adv. Funct. Mater., 2020, 30, 2006213 CrossRef CAS.
- L. Groenendaal, F. Jonas, D. Freitag, H. Pielartzik and J. R. Reynolds, Adv. Mater., 2000, 12, 481–494 CrossRef CAS.
- G. Huseynova, Y. Hyun Kim, J.-H. Lee and J. Lee, J. Inf. Disp., 2020, 21, 71–91 CrossRef CAS.
- D. H. Yoon, S. H. Yoon, K.-S. Ryu and Y. J. Park, Sci. Rep., 2016, 6, 19962 CrossRef CAS PubMed.
- L. Manjakkal, A. Pullanchiyodan, N. Yogeswaran, E. S. Hosseini and R. Dahiya, Adv. Mater., 2020, 32, 1907254 CrossRef CAS PubMed.
- K. Rohtlaid, G. T. M. Nguyen, S. Ebrahimi-Takalloo, T. N. Nguyen, J. D. W. Madden, F. Vidal and C. Plesse, Adv. Mater. Technol., 2021, 6, 2001063 CrossRef CAS.
- J. Cameron and P. J. Skabara, Mater. Horiz., 2020, 7, 1759–1772 RSC.
- A. Sharma, G. Andersson and D. A. Lewis, Phys. Chem. Chem. Phys., 2011, 13, 4381–4387 RSC.
- J. Kettle, H. Waters, Z. Ding, M. Horie and G. C. Smith, Sol. Energy Mater., 2015, 141, 139–147 CrossRef CAS.
- L. Gubler, S. M. Dockheer and W. H. Koppenol, J. Electrochem. Soc., 2011, 158, B755 CrossRef CAS.
- T. M. Nolte, T. Nauser and L. Gubler, Phys. Chem. Chem. Phys., 2020, 22, 4516–4525 RSC.
- S. M. Dockheer, L. Gubler, P. L. Bounds, A. S. Domazou, G. G. Scherer, A. Wokaun and W. H. Koppenol, Phys. Chem. Chem. Phys., 2010, 12, 11609–11616 RSC.
- G. Madras and B. J. McCoy, Chem. Eng. Sci., 1997, 52, 2707–2713 CrossRef.
- D. A. Ahmad Ruzaidi, M. M. Mahat, Z. Mohamed Sofian, N. A. Nor Hashim, H. Osman, M. A. Nawawi, R. Ramli, K. A. Jantan, M. F. Aizamddin, H. H. Azman, Y. H. Robin Chang and H. H. Hamzah, Polymers, 2021, 13, 2901 CrossRef CAS PubMed.
- S. Ebrahimi, M. Nasiri, S. Agbolaghi, F. Abbasi and R. Sarvari, J. Polym. Res., 2018, 25, 236 CrossRef.
- F. Louwet, L. Groenendaal, J. Dhaen, J. Manca, J. Van Luppen, E. Verdonck and L. Leenders, Synth. Met., 2003, 135–136, 115–117 CrossRef CAS.
- E. B. Çelebi and F. Hacıvelioğlu, Polymer, 2022, 255, 125175 CrossRef.
- E. B. Çelebi and F. Hacıvelioğlu, Polym. Chem., 2017, 8, 3022–3033 RSC.
- E. Büşra Çelebi, N. Demirhan and F. Hacıvelioğlu, J. Mater. Chem. C, 2018, 6, 2672–2677 RSC.
- E. B. Çelebi and F. Hacıvelioğlu, New J. Chem., 2021, 45, 19364–19372 RSC.
- H. R. Allcock, S. D. Reeves, C. R. de Denus and C. A. Crane, Macromolecules, 2001, 34, 748–754 CrossRef CAS.
- T. Horii, Y. Li, Y. Mori and H. Okuzaki, Polym. J., 2015, 47, 695–699 CrossRef CAS.
|
This journal is © The Royal Society of Chemistry 2024 |
Click here to see how this site uses Cookies. View our privacy policy here.