DOI:
10.1039/D4TA05086J
(Paper)
J. Mater. Chem. A, 2024, Advance Article
Aromatic pore surface with multiple adsorption sites for one-step C2H4 acquisition from C2H6/C2H4 mixture†
Received
22nd July 2024
, Accepted 26th August 2024
First published on 28th August 2024
Abstract
Developing a C2H6-selective adsorbent for the efficient one-step purification of C2H4 from C2H6/C2H4 mixture is of great significance but is still challenging because of the extremely close physicochemical properties of C2H6 and C2H4. We herein report a metal–organic framework Ni-3-F, which possesses an aromatic pore surface and shows high-density distribution of N/O/F atoms on its pore wall. Such a special pore environment provides the C2H6 molecule with multitudinous binding sites. Adsorption experiments show that Ni-3-F exhibits a higher C2H6 adsorption capacity than C2H4 in the range of 273–313 K and delivers a high C2H6/C2H4 (1/99) selectivity of 1.80 at 298 K. Practical breakthrough experiments show that Ni-3-F can achieve efficient C2H6/C2H4 separation and realize a relatively high C2H4 yield (27.14 L kg−1) at 298 K and 1 bar. Furthermore, according to its comparatively stable structure, Ni-3-F can maintain good separation performance at different temperatures (298–318 K), flow rates (1.05–2.95 mL min−1) and relative humidities (33–100%). Computational simulations reveal that the aromatic pore surface and multiple N/O/F sites comprehensively provide C2H6 with more C–H⋯π and C–H⋯N/O/F supramolecular interactions, which allows Ni-3-F to preferentially adsorb C2H6 over C2H4.
Introduction
As the most important raw material in petrochemical industry, ethylene (C2H4) has been widely used for producing polyethylene, vinyl chloride, polyvinyl chloride and other chemicals.1–3 Until 2023, more than 200 million tons C2H4 had been produced by cracking petroleum hydrocarbons or pyrolysing ethane at high temperature.4 However, during the C2H4 production process, C2H6 is always inevitably produced as an impurity.5–7 In order to obtain polymer-grade C2H4 for its downstream applications, additional separation processes are required. Presently, in the industry, C2H4 is generally purified via cryogenic distillation at high pressure and low temperature, which not only requires exceedingly high equipment investment and complex operation processes, but is also extremely energy-intensive.8–10 The above purification technology cannot meet the requirements of sustainable and green development. Thus, a low-cost and high-efficiency separation method is urgently desired.
Adsorptive separation technology based on porous adsorbents has been deemed as a promising alternative. In recent years, with the boom in porous adsorbents, adsorptive separation technology has achieved rapid development and some significant separation processes, such as C3H8/C3H6,11–14 C2H2/CO2,15–19 H2/D2,20–22 C3F6/C3F8 and so on,23,24 have been widely investigated. However, owing to the physical properties such as the molecular size, quadrupole moment and polarizability of C2H6 (4.4 Å, 0.65 × 10−26 esu cm2 and 44.7 × 10−25 cm3, respectively), which are extremely close to those of C2H4 (4.2 Å, 1.5 × 10−26 esu cm2 and 42.52 × 10−25 cm3, respectively), separating C2H6/C2H4 mixture is thus extraordinarily challenging.25,26 Furthermore, in order to directly acquire high-purity C2H4 from C2H6/C2H4 mixture, constructing a C2H6-selective adsorbent is of great significance.5,27–29 According to the literature and our group's previous reports, designing porous materials with aromatic pores can efficiently enhance C2H6 adsorption through multiple C–H⋯π interactions.27,30,31 Moreover, in view of the more C–H bonds in the C2H6 molecule, inserting more electronegative groups such as nitrogen, oxygen and fluorine atoms in the framework can provide more C–H⋯N/O/F supramolecular interactions, which are helpful in increasing the uptake of C2H6.32 Therefore, if we prepare a porous adsorbent with an aromatic pore surface and a high-density distribution of N/O/F binding sites in the pore wall, it may effectively realize preferential ethane adsorption and achieve high-efficiency C2H4 purification from C2H6/C2H4 mixture.
Based on the above considerations, we herein employed a fluorinated organic ligand (3-fluoro-4-(pyridine-4-yl)benzoic acid) and constructed a novel quadruple interpenetrated MOF material (Ni-3-F). Benefiting from the high-density distribution of N/O/F atoms on its pore wall, Ni-3-F can provide multiple supramolecular bonding sites for C2H6 molecule, which makes it preferentially adsorb C2H6 rather than C2H4. At 298 K and 1 bar, Ni-3-F can adsorb 77.44 cm3 g−1 of C2H6 but can only absorb 65.13 cm3 g−1 of C2H4. Due to its different adsorption for C2H6 and C2H4, Ni-3-F can efficiently capture C2H6 and provide high-purity C2H4 from the C2H6/C2H4 mixture (1/99, v/v). At 298 K and 1 bar, Ni-3-F can provide 27.14 L kg−1 of polymer-grade C2H4 from the C2H6/C2H4 mixture with a volume ratio of 1/99. More importantly, due to its high structural stability, Ni-3-F can even realize excellent C2H6/C2H4 separation at high temperature (313 K), high flow rate (2.95 mL min−1) as well as high-humidity condition (100% relative humidity). Theoretical calculations show that Ni-3-F provides more supramolecular interactions with C2H6 than C2H4, which is the key to realize efficient C2H6/C2H4 separation.
Experimental section
Materials and physical measurements
All chemicals were purchased from suppliers and used directly without further purification. 1H NMR spectra were recorded using a Bruker AVANCE III 400 (400 MHz) spectrometer. Single-crystal X-ray diffraction experiments were carried out on an XtaLAB Synergy R diffractometer. The powder X-ray diffraction (PXRD) data was recorded using a Rigaku MiniFlex600 X-ray diffractometer with Cu Kα radiation (λ = 1.5406 Å), scanning at 5° min−1 in the range of 4–50°. The sample was heated in air to the expected temperature and stored for about 10 min to collect PXRD patterns at different temperatures. The thermogravimetric analysis curves was recorded on an NETZSCH-STA-449C unit at a heating rate of 10 °C min−1 in a nitrogen atmosphere.
Synthesis of ligand (3-fluoro-4-(pyridin-4-yl)benzoic acid)
The mixture of pyridin-4-ylboronic acid (3.7 g, 30 mmol), 4-bromo-3-fluorobenzoic acid (6.6 g, 30 mmol), Na2CO3 (8.5 g, 80 mmol) and Pd(PPh3)4 (2.4 g, 2.4 mmol) were dissolved in 130 mL H2O and 150 mL 1,4-dioxane and heated to 105 °C for 48 h under N2 atmosphere. After the reaction was completed, the resulting mixture was cooled to room temperature and filtered. The solids were washed with ethanol (3 × 20 mL) to collect the filtrate. Then, the filtrate was dried under vacuum condition. Finally, the filtrate was acidified using 3 M HCl to pH = 3 and then filtered. The solid was dried to obtain a white powder. The ligand purity was verified by 1H NMR. 1H NMR (400 MHz, DMSO-d6): δ, 13.44 (s, 1H), 8.68–8.70 (m, 2H), 7.74–7.88 (m, 3H), 7.61–7.63 (m, 2H).
Synthesis of Ni-3-F
Ni(NO3)2·6H2O (29.08 mg, 0.1 mmol) and 3-fluoro-4-(pyridin-4-yl)benzoic acid (21.72 mg, 0.1 mmol) were dissolved in 7.5 mL DMF and 0.15 mL triethylamine. Then, they were transferred to a 25 mL Teflon-lined stainless-steel container and heated at 150 °C for 3 days. After cooling, green block crystals could be obtained by filtering and washing multiple times with DMF.
Gas sorption experiment
All gas adsorption experiments were performed on an ASAP 3020 surface analyzer. Fresh samples were soaked in acetone for 7 days; during this time, fresh acetone was replaced 3 times each day. Before the adsorption experiments, the exchanged sample was treated in vacuum at room temperature for at least 6 hours. Then, in the adsorption tube, the sample was heated at 80 °C for 10 hours with a high vacuum (less than 100 μm Hg) to obtain activated Ni-3-F for the next adsorption tests. All adsorption experiments were carried out at liquid nitrogen temperature of 77 K or water bath temperature of 273–313 K.
Breakthrough measurements
The breakthrough experiments were carried out by an HP-MC41 gas separation test system and a GC9860-5CNJ gas chromatograph. The breakthrough separation experiments for C2H6/C2H4 (1/99, v/v) were conducted in a fixed bed under ambient conditions. The sample column is a transparent quartz column with a length of 200 mm and an inner diameter of 3 mm. All gas flow rates are controlled by a mass flow controller, and gas flow rates outside the column are measured by a gas chromatography (GC) detector. The initially activated Ni-3-F crystal (sample mass: 1.1395 g) was tightly packed into the column and then activated for 10 hours at 80 °C with Ar gas flow rate of 15 mL min−1. In continuous cycle experiments, the sample was purified and regenerated by Ar purging at 80 °C for 3 h.
Results and discussion
Crystal structure
Single crystal X-ray diffraction analysis shows that Ni-3-F crystallizes in the orthorhombic system and Pnna space group. In the structure of Ni-3-F, the Ni(II) center adopts a tetra-coordination model, in which two pairs of pyridine and carboxyl groups from four neighboring 3-fluoro-4-(pyridin-4-yl)benzoic acid ligands are coordinated with the Ni(II) cation to form a 4-connected tetrahedral node (Fig. 1a). If simplifying the 3-fluoro-4-(pyridin-4-yl)benzoic acid ligand as a 2-connected linker, Ni-3-F possesses a 3D framework with specific dia topology (Fig. 1b). Additionally, in order to stabilize its structure, four independent frameworks interpenetrate with each other to form a 3D structure with 1D channel (Fig. 1c). The pore size is approximately 6.7 Å (calculated by zeo++ package) along the crystallographic b-axis (Fig. 1d and e). Furthermore, the porosity and the solvent-accessible pore volume void of Ni-3-F calculated by PLATON33,34 are 38.1% and 1214.76 Å3, respectively. More importantly, despite the fourfold interpenetration, all the N/O/F atoms are distributed on the surface pore of Ni-3-F, which may provide more accessible binding sites for the C2H6 molecules.
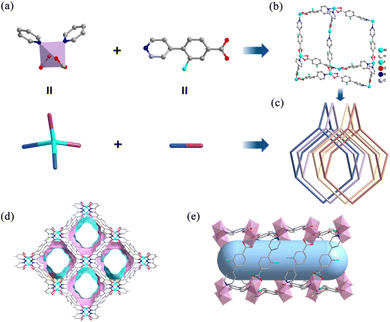 |
| Fig. 1 (a) The coordination environment of Ni(II) ions in Ni-3-F. (b) The perspective view of a single set of a diamond network in Ni-3-F. (c) Four-fold interpenetrated dia nets in Ni-3-F. (d) The 1D channel viewed along the b-axis in Ni-3-F. (e) The rectangular 1D channel viewed along the a-axis. | |
Stability of Ni-3-F
The phase purity of Ni-3-F can be verified by powder X-ray diffraction (PXRD) experiments. As can be seen in Fig. S1,† the PXRD pattern of the as-synthesized sample matches well with the simulated ones. After Ni-3-F is soaked in common organic solvents, such as methanol, ethanol, acetone, acetonitrile and dichloromethane for 5 days, the PXRD patterns show that it can maintain its original structure (Fig. S2†). Furthermore, in order to determine its thermostability, thermogravimetric analysis (TGA) and variable temperature X-ray diffraction (VT-PXRD) experiments were conducted. As shown in Fig. S3,† there is no obvious weight loss until 350 °C. From the variable temperature X-ray diffraction (VT-PXRD) experiments (Fig. S4†), we find that the framework can be maintained at least up to 200 °C. The above results indicate that Ni-3-F has excellent structural stability, which can serve as a potential adsorbent in the actual industrial environment.
Gas sorption
On the basis of the structural stability and specially endowed channel, we conducted the gas adsorption tests for Ni-3-F. Firstly, the permanent porosity of Ni-3-F was established by N2 adsorption experiments at 77 K. As seen in Fig. S5,† the 77 K N2 isotherm exhibits reversible type-I adsorption behavior and the saturation adsorption capacity reaches 150.8 cm3 g−1. The corresponding Brunauer–Emmett–Teller (BET) surface area is 583.4 m2 g−1. According to the abundance of F atoms and densely distributed N/O atoms on the pore wall, we speculate that Ni-3-F may generate stronger interaction for C2H6 molecule.35–39 In order to verify our hypothesis, we carried out single-component adsorption experiments for C2H6 and C2H4. As shown in Fig. 2a and b, the adsorption curves for C2H6 increase more sharply than those for C2H4 from 273 K to 313 K. At the same temperature, the maximum adsorption capacity of C2H6 for Ni-3-F is always higher than that of C2H4. For example, at 298 K and 100 kPa, the uptake for C2H6 is 77.44 cm3 g−1, which is higher than that of some reported C2H6-selective adsorbents, such as UiO-67 (67.42 cm3 g−1),27 Cu(Qc)2 (41.5 cm3 g−1),40 ZIF-8 (56 cm3 g−1)41 and FJI-H11-Me (58.0 cm3 g−1)30 but lower than that of some top-ranking materials, like Ni-MOF 2 (133 cm3 g−1),42 MFM-300(In) (114.24 cm3 g−1)13 and Co-9-ina (84.1 cm3 g−1)37 under the same conditions. In comparison, the uptake for C2H4 is only 65.13 cm3 g−1. More importantly, in the low-pressure region, the adsorption isotherm for C2H6 also rises more sharply than that for C2H4, which indicates that Ni-3-F shows the stronger affinity for C2H6 (Fig. 2c). In order to investigate the cycle performance of Ni-3-F on C2H6 and C2H4, five continuous cycles of C2H6 and C2H4 adsorption experiments at 298 K were recorded. As seen in Fig. 2d and S6,† after five cycles, the C2H6 and C2H4 adsorption capacity show no obvious decrease.
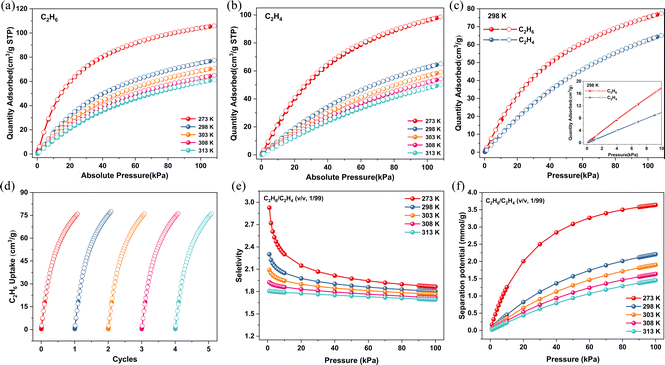 |
| Fig. 2 Single-component adsorption isotherms of C2H6 (a) and C2H4 (b) in the range of 273–313 K. (c) The comparison of C2H6 and C2H4 isotherms for Ni-3-F at 298 K. (d) Five cycles of C2H6 adsorption isotherms at 298 K. (e) IAST selectivity and (f) separation potential for C2H6/C2H4 (1/99) at 273 K, 298 K, 303 K, 308 K and 313 K. | |
In order to further assess the different interactions of C2H6 and C2H4, the heat of adsorption (Qst) was calculated with adsorption isotherms at 273 K and 298 K. As shown in Fig. S7–S9,† the Qst for C2H6 (25.47 kJ mol−1) is much higher than that for C2H4 (21.09 kJ mol−1) at zero coverage, implying that Ni-3-F indeed shows stronger interactions towards C2H6, which is beneficial for the preferential capture of C2H6 in the separation experiments. It is worth noting that compared with some known C2H6-selective adsorbents, such as JNU-2 (29.4 kJ mol−1),43 MUF-15 (29.2 kJ mol−1),44 Co-9-ina (30.6 kJ mol−1),37 Fe2(O2)(dobdc) (66.8 kJ mol−1),4 PCN-250 (33.47 kJ mol−1)29 and Cu(Qc)2 (28.8 kJ mol−1),40 the Qst for C2H6 adsorption (25.47 kJ mol−1) on Ni-3-F is lower. Such low Qst highlights Ni-3-F as a promising C2H6-selective adsorbent with a low regenerative energy requirement.
In order to evaluate the adsorption selectivity for C2H6 and C2H4, an ideal adsorbed solution theory (IAST) calculation was employed on the basis of the single-component C2H6 and C2H4 adsorption isotherms at different temperatures (Fig. S10–S19†). As we can see from Fig. 2e, the adsorption selectivity for C2H6/C2H4 (1/99, v/v) at 298 K can reach 1.80, which is higher than that of some reported MOFs, such as In-soc-MOF-1 (1.4),45 MIL-142A (1.5)46 and Cu(ina)2 (1.3).40 More importantly, as the temperature increases, the IAST selectivities show no obvious decrease. Even at 313 K, the C2H6/C2H4 (1/99) selectivity can also climb to 1.69 at 1 bar, which indicates that Ni-3-F may achieve the separation of C2H6 and C2H4 at high temperature conditions. In order to further assess the separation ability of Ni-3-F for C2H6/C2H4, we calculated the separation potential (Δq) at different temperatures. As demonstrated in Fig. 2f, at 298 K and 1 bar, the Δq for C2H6/C2H4 (1/99) can reach 2.21 mmol g−1. When the temperature rises to 313 K, the Δq can also be 1.45 mmol g−1. All the above results collectively illustrate that Ni-3-F can not only realize the separation of C2H6 and C2H4 but give a high productivity of C2H4 in the separation process.
Theoretical mechanism calculations
In order to further explore the mechanism of Ni-3-F preferentially adsorbing C2H6 rather than C2H4, theoretical calculations were carried out. As shown in Fig. 3, both C2H6 and C2H4 could form C–H⋯F and C–H⋯π interactions with the framework. However, it is found that the C2H4 molecule interacts with the framework only through C–H⋯π interactions (the separations between C and C atoms, 3.50–4.07 Å) and C–H⋯F interactions (the separations between H and F atoms, 2.66 Å) (Fig. 3a). By contrast, C2H6 has more interactions with the framework, including C–H⋯π interactions with benzene rings (C⋯C separations, 3.66–4.16 Å), C–H⋯F interactions with F atoms (H⋯F separations, 3.43 Å and 2.82 Å), C–H⋯O interactions between the carboxyl group (H⋯O separation, 3.39 Å), as well as C–H⋯N interactions with pyridine (H⋯N, 2.91 Å and 3.15 Å) (Fig. 3b). The static binding energies of C2H6 and C2H4 are 48.48 and 42.40 kJ mol−1, respectively, which is consistent with the experimentally observed abnormal affinity between the framework and the gas molecules. The above results show that the presence of multiple interaction sites can produce more interaction between Ni-3-F and C2H6, which is conducive to the one-step separation of C2H6/C2H4 to obtain high-purity C2H4.
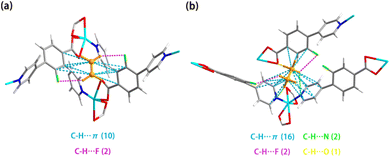 |
| Fig. 3 Calculated preferential adsorption locations of C2H4 (a) and C2H6 (b) in Ni-3-F (the purple dotted line represents C–H⋯F interactions; the blue dotted line represents C–H⋯π interactions; the yellow dotted line represents C–H⋯O interactions; the green dotted line represents C–H⋯N interactions). | |
Column breakthrough tests
In order to verify the actual separation ability of Ni-3-F for C2H6/C2H4, we carried out breakthrough experiments in a packed bed with activated Ni-3-F sample. We used C2H6/C2H4 with a volume ratio of 1/99 to perform column breakthrough experiments. As shown in Fig. 4a, under the condition of 298 K and 1 bar, C2H4 always flows out of the fixed bed first, and after a certain time, C2H6 could be detected at the end of the fixed bed. The separation time of pure C2H4 is 28.8 min g−1. According to the gas flow rate of 1.05 mL min−1, after one separation cycle, about 27.14 L kg−1 polymer-grade C2H4 could be directly collected.
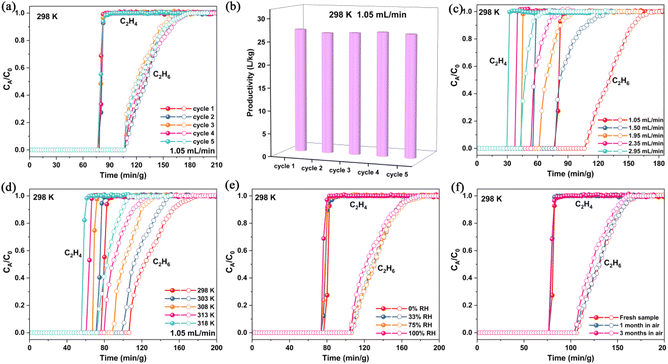 |
| Fig. 4 Ni-3-F breakthrough experiments for C2H6/C2H4 (1/99, v/v). (a) Cyclic breakthrough tests of Ni-3-F for C2H6/C2H4 (1/99, v/v) with the flow rate of 1.05 mL min−1. (b) Five-cycle C2H4 yields for C2H6/C2H4 (1/99, v/v) at 1.05 mL min−1 and 298 K. (c) Breakthrough experiments of Ni-3-F at 298 K with different flow rates. (d) Breakthrough experiments of Ni-3-F at different temperatures with a gas flow rate of 1.05 mL min−1. (e) Breakthrough experiments of Ni-3-F under different relative humidity conditions and 298 K with a flow rate of 1.05 mL min−1. (f) Breakthrough experiments of Ni-3-F after exposing to air for different time duration with a gas flow rate of 1.05 mL min−1. | |
To the best of our knowledge, the adsorbent that may be applied in practical industry should have good durability. As depicted in Fig. 4b, after 5 cycles of breakthrough tests, the separation performance shows no significant decline, which proves that Ni-3-F has desirable repeatability and can served as an ideal physical adsorbent in the industrial environment. As we know, the gas flow rates usually have an influence on gas separation. Therefore, the breakthrough experiments with different gas flow rates have also been investigated. As shown in Fig. 4c, when the gas flow rate gradually increases, Ni-3-F could still maintain excellent C2H6/C2H4 separation performance. Furthermore, in consideration of the high-temperature separation atmosphere in the industry and the inevitability of water vapor in the air, the adsorbent should also hold good separation ability in high temperature and high-humidity conditions. As shown in Fig. 4d, when the temperature is progressively enhanced, Ni-3-F could always realize one-step acquisition of high-purity C2H4 from the C2H6/C2H4 mixture. Even when the temperature increases to 318 K, 14.74 L kg−1 of C2H4 can be obtained. More importantly, when we introduce the moist C2H6/C2H4 mixture into the fixed bed, polymer-grade C2H4 can also be eluted out. As shown in Fig. 4e, the breakthrough curves in different humidity conditions could be coincident with that of the fresh sample, implying that the moisture has no effect on the separation of C2H6/C2H4. Moreover, Ni-3-F also exhibits excellent air stability, which can be demonstrated by the almost overlapping separation curves of the samples placed in air for 1 or 3 months with fresh samples (Fig. 4f). On the whole, the above results all show that Ni-3-F can realize the excellent separation of C2H6/C2H4 and can work well under various harsh conditions.
Conclusions
In summary, we have successfully prepared a four-fold interpenetrating MOF material (Ni-3-F), which has high-density of N/O/F atoms and realizes the preferential adsorption of C2H6 from C2H6/C2H4. At 298 K, the adsorption capacity of C2H6 (77.44 cm3 g−1) is significantly higher than that of C2H4 (65.13 cm3 g−1), and the selectivity of C2H6/C2H4 (1/99, v/v) can reach 1.80. The breakthrough experiments proved that high-purity C2H4 (>99.95%) can be directly obtained from the mixture of C2H6/C2H4 (1/99, v/v) in one step, and the yield reaches 27.14 L kg−1. More importantly, Ni-3-F can maintain good separation performance at different temperatures, flow rates and relative humidity conditions. The theoretical calculation results show that C2H6 can have a variety of interactions with the framework, and the interactions between Ni-3-F and C2H6 are obviously stronger than those of C2H4. All the above results indicate that the aromatic pore surface with multiple adsorption sites can successfully obtain high-purity C2H4 from the C2H6/C2H4 mixture in one step.
Data availability
All data included in this study are available upon request by contacting the corresponding author.
Author contributions
M. Y. W. determined the project. Y. Q. Z. and M. Y. W. designed the MOF synthesis methods. Y. Q. Z. and Z. Y. J. synthesized Ni-3-F. Y. Q. Z. and Y. Z. Z. carried out the power X-ray diffraction. Y. Q. Z. and Y. Z. Z. performed the single-crystal X-ray diffraction experiments. Y. Q. Z. and Z. Y. J. collected data of single-component gas sorption measurements and dynamic breakthrough experiments. Y. Q. Z. wrote the manuscript with support from M. Y. W. All authors contributed to revise the manuscript.
Conflicts of interest
There are no conflicts to declare.
Acknowledgements
This work is supported by NSFC (22271282) and the Self-deployment Project Research Program of Haixi Institutes, Chinese Academy of Sciences with the grant number of CXZX-2022-JQ04. Additionally, this work is also supported by Fujian Science & Technology Innovation Laboratory for Optoelectronic Information of China (No. 2021ZR120) and NSF of Fujian Province (No. 2021J01517 and 2020J06034).
Notes and references
- K. H. Cho, J. W. Yoon, J. H. Lee, J. C. Kim, D. Jo, J. Park, S.-K. Lee, S. K. Kwak and U. H. Lee, Design of Pore Properties of an Al-Based Metal-Organic Framework for the Separation of an Ethane/Ethylene Gas Mixture via Ethane-Selective Adsorption, ACS Appl. Mater. Interfaces, 2023, 15, 30975–30984 CrossRef CAS PubMed.
- X. Lin, Y. Yang, X. Wang, S. Lin, Z. Bao, Z. Zhang and S. Xiang, Functionalized metal-organic and hydrogen-bonded organic frameworks for C2H4/C2H6 separation, Sep. Purif. Technol., 2024, 330, 125252 CrossRef CAS.
- S.-M. Wang, M. Shivanna, S.-T. Zheng, T. Pham, K. A. Forrest, Q.-Y. Yang, Q. Guan, B. Space, S. Kitagawa and M. J. Zaworotko, Ethane/Ethylene Separations in Flexible Diamondoid Coordination Networks via an Ethane-Induced Gate-Opening Mechanism, J. Am. Chem. Soc., 2024, 146, 4153–4161 CrossRef CAS PubMed.
- L. Li, R.-B. Lin, R. Krishna, H. Li, S. Xiang, H. Wu, J. Li, W. Zhou and B. Chen, Ethane/ethylene separation in a metal-organic framework with iron-peroxo sites, Science, 2018, 362, 443–446 CrossRef CAS.
- K. Zhang, J.-J. Pang, X. Lian, Z.-H. Song, Y.-C. Yuan, H. Huang, Z.-Q. Yao and J. Xu, A pacs-type metal-organic framework with high adsorption capacity for inverse C2H6/C2H4 separation, New J. Chem., 2024, 48, 10577–10583 RSC.
- L. Zhang, L. Li, E. Hu, L. Yang, K. Shao, L. Yao, K. Jiang, Y. Cui, Y. Yang, B. Li, B. Chen and G. Qian, Boosting Ethylene/Ethane Separation within Copper(I)-Chelated Metal-Organic Frameworks through Tailor-Made Aperture and Specific π-Complexation, Adv. Sci., 2020, 7, 1901918 CrossRef CAS.
- D. Lv, P. Zhou, J. Xu, S. Tu, F. Xu, J. Yan, H. Xi, W. Yuan, Q. Fu, X. Chen and Q. Xia, Recent advances in adsorptive separation of ethane and ethylene by C2H6-selective MOFs and other adsorbents, Chem. Eng. J., 2022, 431, 133208 CrossRef CAS.
- A. Noonikara-Poyil, H. Cui, A. A. Yakovenko, P. W. Stephens, R. B. Lin, B. Wang, B. Chen and H. V. R. Dias, A Molecular Compound for Highly Selective Purification of Ethylene, Angew. Chem., Int. Ed., 2021, 60, 27184–27188 CrossRef CAS PubMed.
- Z. Di, X. Zheng, Y. Qi, H. Yuan and C.-P. Li, Recent Advances in C2 Gases Separation and Purification by Metal-Organic Frameworks, Chin. J. Struct. Chem., 2022, 41, 2211031–2211044 CAS.
- S. Mukherjee, D. Sensharma, K.-J. Chen and M. J. Zaworotko, Crystal engineering of porous coordination networks to enable separation of C2 hydrocarbons, Chem. Commun., 2020, 56, 10419–10441 RSC.
- Y. Wang, N. Y. Huang, X. W. Zhang, H. He, R. K. Huang, Z. M. Ye, Y. Li, D. D. Zhou, P. Q. Liao, X. M. Chen and J. P. Zhang, Selective Aerobic Oxidation of a Metal-Organic Framework Boosts Thermodynamic and Kinetic Propylene/Propane Selectivity, Angew. Chem., Int. Ed., 2019, 58, 7692–7696 CrossRef CAS.
- Z. Zhang, Q. Ding, X. Cui, X.-M. Jiang and H. Xing, Fine-Tuning and Selective-Binding within an Anion-Functionalized Ultramicroporous Metal-Organic Framework for Efficient Olefin/Paraffin Separation, ACS Appl. Mater. Interfaces, 2020, 12, 40229–40235 CrossRef CAS.
- L. Guo, M. Savage, J. H. Carter, X. Han, I. da Silva, P. Manuel, S. Rudić, C. C. Tang, S. Yang and M. Schröder, Direct Visualization of Supramolecular Binding and Separation of Light Hydrocarbons in MFM-300(In), Chem. Mater., 2022, 34, 5698–5705 CrossRef CAS PubMed.
- Y. Wang, T. Li, L. Li, R. B. Lin, X. Jia, Z. Chang, H. M. Wen, X. M. Chen and J. Li, Construction of Fluorinated Propane-Trap in Metal-Organic Frameworks for Record Polymer-Grade Propylene Production under High Humidity Conditions, Adv. Mater., 2023, 35, 2207955 CrossRef CAS.
- X. Mu, Y. Xue, M. Hu, P. Zhang, Y. Wang, H. Li, S. Li and Q. Zhai, Fine-tuning of pore-space-partitioned metal-organic frameworks for efficient C2H2/C2H4 and C2H2/CO2 separation, Chin. Chem. Lett., 2023, 34, 107296 CrossRef CAS.
- H. Li, C. Chen, Z. Di, Y. Liu, Z. Ji, S. Zou, M. Wu and M. Hong, Rational Pore Design of a Cage-like Metal-Organic Framework for Efficient C2H2/CO2 Separation, ACS Appl. Mater. Interfaces, 2022, 14, 52216–52222 CrossRef CAS.
- H. Li, C. Liu, C. Chen, Z. Di, D. Yuan, J. Pang, W. Wei, M. Wu and M. Hong, An Unprecedented Pillar-Cage Fluorinated Hybrid Porous Framework with Highly Efficient Acetylene Storage and Separation, Angew. Chem., Int. Ed., 2021, 60, 7547–7552 CrossRef CAS.
- M. Guo, Z. Jiang, S. Wu, C. Zhang, S. Zhu, L. Li, H. Zhang, L. Liu, S. Xiang and Z. Yao, Auxiliary ligand influence on interpenetration and C2H2/CO2 separation in NDI based isoreticular MOFs, Inorg. Chem. Commun., 2024, 165, 112578 CrossRef CAS.
- K. Jiang, Y. Gao, P. Zhang, S. Lin and L. Zhang, A new perchlorate-based hybrid ultramicroporous material with rich bare oxygen atoms for high C2H2/CO2 separation, Chin. Chem. Lett., 2023, 34, 108039 CrossRef CAS.
- F. Banijamali, A. Maghari, G. Schutz and M. Hirscher, Hydrogen and deuterium separation on metal organic frameworks based on Cu- and Zn-BTC: an experimental and theoretical study, Adsorption, 2021, 27, 925–935 CrossRef CAS.
- F. Wu, L. Li, Y. Tan, E.-S. M. El-Sayed and D. Yuan, The competitive and synergistic effect between adsorption enthalpy and capacity in D2/H2 separation of M2(m-dobdc) frameworks, Chin. Chem. Lett., 2021, 32, 3562–3565 CrossRef CAS.
- J. Teufel, H. Oh, M. Hirscher, M. Wahiduzzaman, L. Zhechkov, A. Kuc, T. Heine, D. Denysenko and D. Volkmer, MFU-4-A Metal-Organic Framework for Highly Effective H2/D2 Separation, Adv. Mater., 2012, 25, 635–639 CrossRef PubMed.
- W. Xia, Y. Yang, L. Sheng, Z. Zhou, L. Chen, Z. Zhang, Z. Zhang, Q. Yang, Q. Ren and Z. Bao, Temperature-dependent molecular sieving of fluorinated propane/propylene mixtures by a flexible-robust metal-organic framework, Sci. Adv., 2024, 10, eadj6473 CrossRef CAS.
- M. Zheng, W. Xue, T. Yan, Z. Jiang, Z. Fang, H. Huang and C. Zhong, Fluorinated MOF-Based Hexafluoropropylene Nanotrap for Highly Efficient Purification of Octafluoropropane Electronic Specialty Gas, Angew. Chem., Int. Ed., 2024, 136, e202401770 CrossRef.
- B. Zhu, J.-W. Cao, S. Mukherjee, T. Pham, T. Zhang, T. Wang, X. Jiang, K. A. Forrest, M. J. Zaworotko and K.-J. Chen, Pore Engineering
for One-Step Ethylene Purification from a Three-Component Hydrocarbon Mixture, J. Am. Chem. Soc., 2021, 143, 1485–1492 CrossRef CAS.
- H. Shuai, J. Liu, Y. Teng, X. Liu, L. Wang, H. Xiong, P. Wang, J. Chen, S. Chen, Z. Zhou, S. Deng and J. Wang, Pillar-layered metal-organic frameworks with benchmark C2H2/C2H4 and C2H6/C2H4 selectivity for one-step C2H4 production, Sep. Purif. Technol., 2023, 323, 124392 CrossRef CAS.
- Y. Han, L. Liu, Z. Han and M. Wu, Engineering ethane-trapping metal-organic framework for efficient ethylene separation under high humid conditions, Sep. Purif. Technol., 2024, 342, 127011 CrossRef CAS.
- L. Yang, Q. Gao, Y.-M. Zhang, R. Wang and L.-Z. Chen, Efficient C2H6/C2H4 adsorption separation by a microporous heterometal-organic framework, J. Colloid Interface Sci., 2023, 652, 1093–1098 CrossRef CAS PubMed.
- Y. Chen, Z. Qiao, H. Wu, D. Lv, R. Shi, Q. Xia, J. Zhou and Z. Li, An ethane-trapping MOF PCN-250 for highly selective adsorption of ethane over ethylene, Chem. Eng. Sci., 2018, 175, 110–117 CrossRef CAS.
- Z. Di, C. Liu, J. Pang, S. Zou, Z. Ji, F. Hu, C. Chen, D. Yuan, M. Hong and M. Wu, A Metal-Organic Framework with Nonpolar Pore Surfaces for the One-Step Acquisition of C2H4 from a C2H4 and C2H6 Mixture, Angew. Chem., Int. Ed., 2022, 61, e202210343 CrossRef CAS.
- Y. Zhou, C. Chen, R. Krishna, Z. Ji, D. Yuan and M. Wu, Tuning Pore Polarization to Boost Ethane/Ethylene Separation Performance in Hydrogen-Bonded Organic Frameworks, Angew. Chem., Int. Ed., 2023, 62, e202305041 CrossRef CAS PubMed.
- G.-D. Wang, H.-H. Wang, W.-J. Shi, L. Hou, Y.-Y. Wang and Z. Zhu, A highly stable MOF with F and N accessible sites for efficient capture and separation of acetylene from ternary mixtures, J. Mater. Chem. A, 2021, 9, 24495–24502 RSC.
- A. L. Spek, Structure validation in chemical crystallography, Acta Crystallogr., Sect. D: Biol. Crystallogr., 2009, 65, 148–155 CrossRef CAS.
- A. L. Spek, Single-crystal structure validation with the program PLATON, J. Appl. Crystallogr., 2003, 36, 7–13 CrossRef CAS.
- C. X. Chen, Z. W. Wei, T. Pham, P. C. Lan, L. Zhang, K. A. Forrest, S. Chen, A. M. Al-Enizi, A. Nafady, C. Y. Su and S. Ma, Nanospace Engineering of Metal-Organic Frameworks through Dynamic Spacer Installation of Multifunctionalities for Efficient Separation of Ethane from Ethane/Ethylene Mixtures, Angew. Chem., Int. Ed., 2021, 60, 9680–9685 CrossRef CAS PubMed.
- L. Yang, L. Yan, W. Niu, Y. Feng, Q. Fu, S. Zhang, Y. Zhang, L. Li, X. Gu, P. Dai, D. Liu, Q. Zheng and X. Zhao, Adsorption in Reversed Order of C2 Hydrocarbons on an Ultramicroporous Fluorinated Metal-Organic Framework, Angew. Chem., Int. Ed., 2022, 61, e202204046 CrossRef CAS PubMed.
- S.-M. Wang, H.-R. Liu, S.-T. Zheng, H.-L. Lan, Q.-Y. Yang and Y.-Z. Zheng, Control of pore structure by the solvent effect for efficient ethane/ethylene separation, Sep. Purif. Technol., 2023, 304, 122378 CrossRef CAS.
- E. Wu, X.-W. Gu, D. Liu, X. Zhang, H. Wu, W. Zhou, G. Qian and B. Li, Incorporation of multiple supramolecular binding sites into a robust MOF for benchmark one-step ethylene purification, Nat. Commun., 2023, 14, 6146 CrossRef CAS PubMed.
- M. H. Yu, H. Fang, H. L. Huang, M. Zhao, Z. Y. Su, H. X. Nie, Z. Chang and T. L. Hu, Tuning the Trade-Off between Ethane/Ethylene Selectivity and Adsorption Capacity within Isoreticular Microporous Metal-Organic Frameworks by Linker Fine-Fluorination, Small, 2023, 19, 2300821 CrossRef CAS PubMed.
- R.-B. Lin, H. Wu, L. Li, X.-L. Tang, Z. Li, J. Gao, H. Cui, W. Zhou and B. Chen, Boosting Ethane/Ethylene Separation within Isoreticular Ultramicroporous Metal-Organic Frameworks, J. Am. Chem. Soc., 2018, 140, 12940–12946 CrossRef CAS PubMed.
- U. Böhme, B. Barth, C. Paula, A. Kuhnt, W. Schwieger, A. Mundstock, J. Caro and M. Hartmann, Ethene/Ethane and Propene/Propane Separation via the Olefin and Paraffin Selective Metal-Organic Framework Adsorbents CPO-27 and ZIF-8, Langmuir, 2013, 29, 8592–8600 CrossRef.
- Y. Ye, Y. Xie, Y. Shi, L. Gong, J. Phipps, A. M. Al-Enizi, A. Nafady, B. Chen and S. Ma, A Microporous Metal-Organic Framework with Unique Aromatic Pore Surfaces for High Performance C2H6/C2H4 Separation, Angew. Chem., Int. Ed., 2023, 62, e202302564 CrossRef CAS.
- H. Zeng, X.-J. Xie, M. Xie, Y.-L. Huang, D. Luo, T. Wang, Y. Zhao, W. Lu and D. Li, Cage-Interconnected Metal-Organic Framework with Tailored Apertures for Efficient C2H6/C2H4 Separation under Humid Conditions, J. Am. Chem. Soc., 2019, 141, 20390–20396 CrossRef CAS.
- O. T. Qazvini, R. Babarao, Z.-L. Shi, Y.-B. Zhang and S. G. Telfer, A Robust Ethane-Trapping Metal-Organic Framework with a High Capacity for Ethylene Purification, J. Am. Chem. Soc., 2019, 141, 5014–5020 CrossRef CAS PubMed.
- H. Wu, Y. Chen, D. Lv, R. Shi, Y. Chen, Z. Li and Q. Xia, An indium-based ethane-trapping MOF for efficient selective separation of C2H6/C2H4 mixture, Sep. Purif. Technol., 2019, 212, 51–56 CrossRef CAS.
- Y. Chen, H. Wu, D. Lv, R. Shi, Y. Chen, Q. Xia and Z. Li, Highly Adsorptive Separation of Ethane/Ethylene by An Ethane-Selective MOF MIL-142A, Ind. Eng. Chem. Res., 2018, 57, 4063–4069 CrossRef CAS.
Footnote |
† Electronic supplementary information (ESI) available: Experimental procedures. CCDC 2369828. For ESI and crystallographic data in CIF or other electronic format see DOI: https://doi.org/10.1039/d4ta05086j |
|
This journal is © The Royal Society of Chemistry 2024 |