DOI:
10.1039/D4SC03624G
(Edge Article)
Chem. Sci., 2024,
15, 13800-13806
Double strain-release enables formal C–O/C–F and C–N/C–F ring-opening metathesis†
Received
3rd June 2024
, Accepted 20th July 2024
First published on 27th July 2024
Abstract
Metathesis reactions have been established as a powerful tool in organic synthesis. While great advances were achieved in double-bond metathesis, like olefin metathesis and carbonyl metathesis, single-bond metathesis has received less attention in the past decade. Herein, we describe the first C(sp3)–O/C(sp3)–F bond formal cross metathesis reaction between gem-difluorinated cyclopropanes (gem-DFCPs) and epoxides under rhodium catalysis. The reaction involves the formation of a highly electrophilic fluoroallyl rhodium intermediate, which is capable of reacting with the oxygen atom in epoxides as weak nucleophiles followed by C–F bond reconstruction. The use of two strained ring substrates is the key to the success of the formal cross metathesis, in which the double strain release accounts for the driving force of the transformation. Additionally, azetidine also proves to be a suitable substrate for this transformation. The reaction offers a novel approach for the metathesis of C(sp3)–O and C(sp3)–N bonds, presenting new opportunities for single-bond metathesis.
Introduction
The advent of metathesis reactions has promoted a revolution in synthetic chemistry and related areas,1 offering innovative methodologies that are conceptually novel and strategically advanced for molecular construction. Among them, double-bond metathesis, typified by various types of olefin metathesis, has become an indispensable toolbox in modern organic chemistry. Besides, carbonyl-olefin metathesis has gradually drawn the attention of chemists and become a powerful technique for molecular backbone editing of carbonyl compounds.2,3 In contrast, research on single-bond metathesis has not garnered sufficient attention and only limited examples were developed,4,5 likely due to the less reactive σ-bonds compared with the π-bond. As for the metathesis of the C–O single bond, Jutzi disclosed that the pentamethylcyclopentadienyl silicon(II) cation (Cp*Si(II)+) expressed reactivity for the ring-closing C–O metathesis of ethers, which was applied in the degradation of oligo(ethyleneglycol) diethers (Scheme 1a).6 The Morandi group reported that simple and cheap Fe(OTf)3 was an efficient catalyst, providing an elegant and practical approach for the ring-closing C–O metathesis of aliphatic ethers.7 Subsequently, other types of Lewis acids8 and Brønsted acids9 were also found to be capable catalysts in C–O/C–O metathesis reactions, which greatly enriched the reactions implemented for ether synthesis.
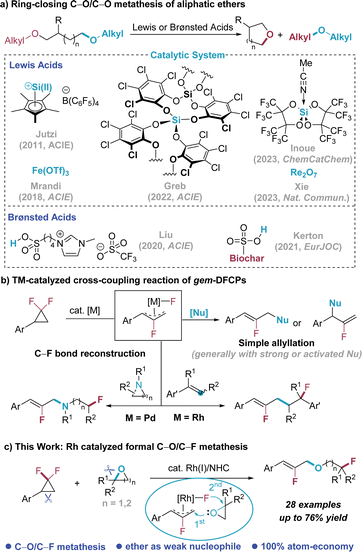 |
| Scheme 1 Metathesis reactions involving the C–O single bond and their background. | |
While there are noticeable advances in the area of single-bond metathesis regarding the C–O bond, these reactions are mainly restricted in C–O/C–O bond metathesis with the use of Lewis/Brønsted acid-activation mode.6–9 Given the significance of cross metathesis in structural editing of molecular backbones, as an analogue of carbonyl–olefin metathesis in the double-bond metathesis area,2,3 extending C–O/C–O single bond metathesis to C–O/C–X (X is an element other than oxygen) metathesis would substantially enrich the diversity of the reaction pattern. However, the development of C–O/C–X metathesis is a challenging task and there has been no reported method to the best our knowledge.
On the other hand, the development of new strategies to introduce fluorine into organic molecules has emerged as one of the primary research focuses, as the introduction of the fluorine can endow the organic molecules with distinct properties.10 Recently, the use of gem-difluorinated cyclopropanes (gem-DFCPs)11 as a new type of reagents to synthesize fluorine-containing compounds has caught considerable attention, most of which involve a fluoroallyl intermediate under transition-metal catalysis.12–14 Our group has been continuously interested in the development of new reaction modes of gem-DFCPs especially using rhodium catalysis.15 It was found that the fluoroallyl-Rh intermediate can be highly electrophilic that is able to react with weak or non-polar nucleophiles, like simple arenes15a and olefins,15b–d which is distinct from other transition-metal catalized reactions with gem-DFCPs that generally necessitate strong or activated nucleophiles (Scheme 1b, top).13 Meanwhile, Liu and co-workers disclosed that the use of cyclic amines as strong nucleophiles can allow C–F bond reconstruction16 with gem-DFCPs as bifunctional reagents under Pd catalysis;17a our group also revealed the unique usage of gem-DFCPs in the Rh-catalyzed olefin fluoroallylation reaction17b (Scheme 1b, bottom).
Along this line and considering the importance and challenges in C–O/C–X metathesis, we envisage that the highly electrophilic fluoroallyl-Rh intermediate can also be captured by using epoxide as an unusual nucleophile (1st bond formation), though epoxides are generally used as electrophiles in organic synthesis,18 and the following reconstruction of the C–F bond (2nd bond formation) would eventually lead to the formal C–O/C–F ring-opening metathesis (Scheme 1c). Herein, we discover that the formal C–O/C–F ring-opening metathesis can be realized between gem-DFCPs and epoxides by using Rh catalysis that consists of [Rh(CO)2Cl]2 as the pre-catalyst and N-heterocyclic carbene (NHC) as the ligand. The strain release from the two strained three-membered substrates19,20 provides the driving force for the whole transformation, with cleavage and formation of many inert chemical bonds including C–C, C–O and C–F bonds (Scheme 1c). In addition, this strategy can also be applied to the reaction with azetidine to achieve formal C(sp3)–N/C(sp3)–F ring opening metathesis.
Results and discussion
In our initial experiments, 4-(2,2-difluorocyclopropyl)-1,1′-biphenyl (1a) was selected as the model substrate to investigate its reactivity with 1,1-dimethyloxirane (2a) using Rh catalysis (Table 1). Under previously established reaction conditions where olefins were used as the nucleophiles,15b the desired product 3a was obtained, albeit in low yield (entry 1). The widely used ligand in the Rh-catalyzed reaction of gem-DFCPs, binap (2,2′-bis(diphenylphosphino)-1,1′-binaphthyl),12d only results in decomposition of the substrate 1a (entry 2). The bidentate phosphine ligand dcpf (1,1′-bis(dicyclohexylphosphino)ferrocene) also yielded an unsatisfactory outcome (entry 3). In contrast, the utilization of NHC ligands notably improved the reaction efficiency, producing the product 3a in 43% or 60% yield with IMes (1,3-bis(2,4,6-trimethylphenyl)imidazol-2-ylidene) or IPr (1,3-bis(2,6-di-isopropylphenyl)imidazol-2-ylidene) as the ligand, respectively (entries 4 and 5). Subsequent solvent screening revealed that a switch to fluorobenzene slightly decreased the reaction yield (entry 6). The use of DCM as the solvent totally shut down the C–O/C–F ring-opening metathesis, leading to the decomposition of the substrate 1a (entry 7). A remarkable improvement was observed when PhCF3 was chosen as the reaction solvent, delivering the desired product with a yield of 56% (entry 8). The influence of the silver salt was then investigated, and it was found that other silver salts were less efficient than AgBF4 in this transformation (entries 9–11). Similar to our previous work,15b a great promotion in yield was observed when the catalytic amount of MeCN was added as an additive (entry 12). After systematically screening the nitrile additives, the yield of 3a was further improved to 76% (72% isolated yield) in the presence of a bulky nitrile nPr2CHCN (entry 13).
Table 1 Optimization of the reaction conditionsa
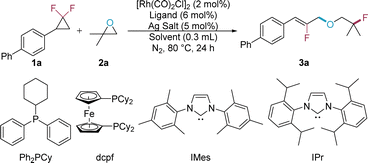
|
Entry |
Ligand |
Solvent |
Ag salt |
Yieldb |
Reactions were performed with 1a (0.1 mmol), 2a (0.3 mmol), [Rh(CO)2Cl]2 (2 mol%), ligand (6 mol%), Ag salt (5 mol%) in 0.3 mL of solvent at 80 °C for 24 h.
The yield was determined by 1H NMR using 1,1,2,2-tetrachloroethane (TCE) as an internal standard.
MeCN (25 mol%) was added.
n
Pr2CHCN (25 mol%) was added.
Isolated yield is shown in parentheses.
|
1 |
Ph2PCy |
PhCl |
AgBF4 |
17% |
2 |
Binap |
PhCl |
AgBF4 |
0% |
3 |
dcpf |
PhCl |
AgBF4 |
19% |
4 |
IMes |
PhCl |
AgBF4 |
37% |
5 |
IPr |
PhCl |
AgBF4 |
45% |
6 |
IPr |
PhF |
AgBF4 |
44% |
7 |
IPr |
DCM |
AgBF4 |
0% |
8 |
IPr |
PhCF3 |
AgBF4 |
56% |
9 |
IPr |
PhCF3 |
AgSbF6 |
24% |
10 |
IPr |
PhCF3 |
AgPF6 |
0% |
11 |
IPr |
PhCF3 |
AgOTf |
41% |
12c |
IPr |
PhCF3 |
AgBF4 |
68% |
13d |
IPr |
PhCF3 |
AgBF4 |
76%(72%)e |
Upon establishing the optimized reaction conditions, we advanced to investigate the substrate scope of the formal C–O/C–F ring-opening metathesis reaction (Scheme 2). For gem-DFCPs (Scheme 2a), simple phenyl gem-DFCP (3b) yielded a satisfactory result. Aryl gem-DFCPs with varying positional methyl group (3c, 3d) were well tolerated, in which the ortho-substituted one (3d) even contributes a slightly enhanced yield. Aside from the methyl group, the methoxy group at different positions of the aromatic ring is also well tolerated (3e, 3f). Other electron-donating groups such as tert-butyl (3g) were tested, in which the substrate furnishes the corresponding product in 64% yield. In the case of halogen-substituted substrates, both fluorine- (3h, 3i) and chlorine-containing (3j, 3k) gem-DFCPs present good reactivity; while the bromine-substituted one (3l) gives a diminished yield. The substrate with two substitutions provides the corresponding products (3m, 3n) in moderate to good yields. Furthermore, ester groups (3o, 3p) were capable of preservation under these reaction conditions. Naphthyl (3q) and benzofuryl (3r) gem-DFCPs are qualified substrates for this transformation, forming the desired products in modest yields. Finally, alkyl gem-DFCP is unreactive under the current reaction conditions.
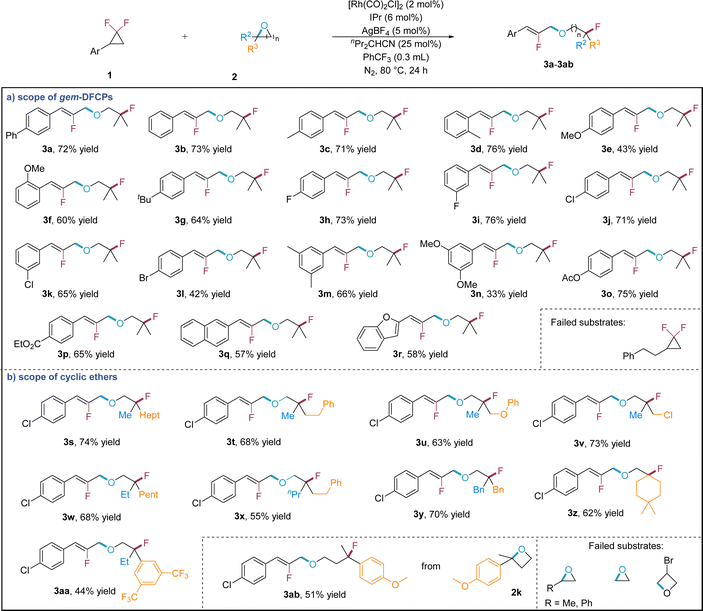 |
| Scheme 2 Scopes of the Rh-catalyzed ring-opening C–O/C–F cross metathesis reaction. Reactions were performed with 1 (0.1 mmol), 2 (0.3 mmol), [Rh(CO)2Cl]2 (2 mol%), IPr (6 mol%), AgBF4 (5 mol%), nPr2CHCN (25 mol%) in 0.3 mL of PhCF3 at 80 °C for 24 h, and isolated yields were provided. | |
We then tested the reaction scope of epoxide substrates (Scheme 2b). The reaction proceeds smoothly when one of the methyl groups in the epoxide substrate is replaced with different types of alkyl groups (3s–3v), of which electron-rich arene (3u) and primary alkyl chloride (3v) can be tolerated in this transformation. Similarly, a wide range of epoxides that are substituted with two alkyl groups perform very well, producing the corresponding products in 55–70% yields (3w–3z). Beyond alkyl substitution, the reaction protocol exhibits compatibility with aryl substitution directly on the epoxide substrate, exemplified by 3,5-bis(trifluoromethyl)phenyl oxirane (3aa). Apart from epoxides, it was found that the range of nucleophiles can be further extended to oxetane, providing the corresponding product (3ab) with modest yield by using 2-(4-methoxyphenyl)-2-methyloxetane (2k) as the substrate. However, mono-substituted and simple epoxides as well as mono-substituted oxetane show no reactivity in this formal C–O/C–F ring-opening metathesis reaction.
With the success of the formal C–O/C–F bond metathesis under Rh catalysis, we are wondering whether this Rh-catalyzed protocol can be extended to other C–heteroatom single bond metathesis reactions. It was found that 3-methyleneazetidine 4 can be employed as the nucleophile to couple with gem-DFCPs, which leads to the formal C(sp3)–N/C(sp3)–F bond metathesis (Scheme 3). The reaction is very efficient, in which only 0.5 mol% of [Rh(CO)2Cl]2 is required to achieve 93% yield of the product 5a for the model reaction (see ESI† for more details on the condition optimization). The reaction scope of gem-DFCPs is then thoroughly investigated. A wide range of aryl gem-DFCPs, including those with electron-donating (5b–5d), electron-neutral (5d, 5e) and electron-withdrawing (5g–5n) substituents regardless of the position, furnish the target products in good yields, except for the ortho-substituted case (5l) which is in diminished yield. Besides, disubstituted variants(5o–5q), naphthyl (5r), benzofuryl (5s) and ferrocenyl (5t) substrates all work very well under the optimized reaction conditions, in which the structure of product 5r is confirmed by X-ray crystallographic analysis.21 Note that the reaction of alkyl gem-DFCP (5u) proceeds smoothly under the same reaction conditions, affording the corresponding product in 72% yield. During our investigation on finding suitable N-protecting groups of 3-methyleneazetidine in this reaction, it is found that several candidates with N-protecting groups including phenyl, benzyl, triphenylmethyl and Cbz (benzyloxycarbonyl) show no reactivity, indicating a big influence of the N-protecting group on the efficiency of the reaction. It is speculated that nitrogen with a smaller protecting group would poison the rhodium catalyst by strong coordination, while substrates with a bulkier (CPh3) or carbonyl (Cbz) protecting group would decrease the nucleophilic ability of the nitrogen. Finally, 3-methyl and simple azetidines express some reactivity but afford the target products with very low yields.
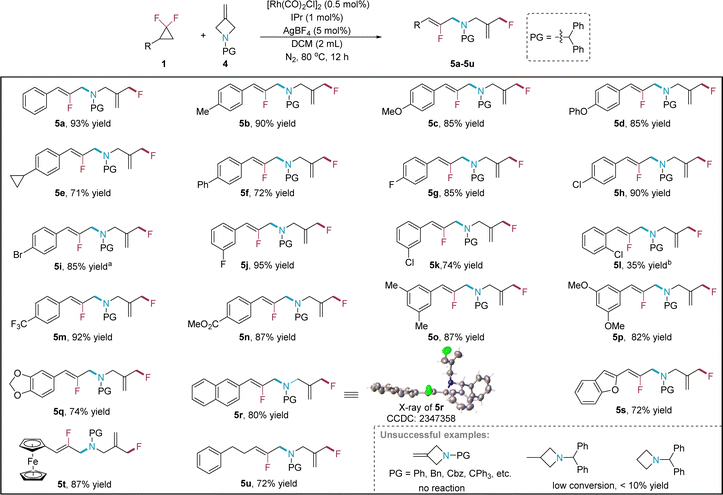 |
| Scheme 3 Substrate scope of the Rh-catalyzed ring-opening C–N/C–F metathesis reaction. Reaction conditions: 1 (0.3 mmol), 4 (0.2 mmol), [Rh(CO)2Cl]2 (0.5 mol%), IPr (1 mol%), AgBF4 (5 mol%) in 2 mL of solvent at the indicated temperature for 12 h, isolated yields were provided. aReaction was performed with [Rh(CO)2Cl]2 (2 mol%), IPr (4 mol%). bReaction was performed for 24 h. | |
After investigating the reaction scope, we then move to explore the synthetic utility of these fluorine-containing ether and amine products (Scheme 4). The reduction of 3a under a hydrogen atmosphere with Pd/C gives ether 6 in 96% yield, in which the two fluorine substituents can be retained in the transformation. Furthermore, the alkyl fluoride moiety in 3a can smoothly undergo alkylation (7)22 and allylation (8)23 in the presence of a Lewis acid, wherein the substitution shifts to the α-carbon of the oxygen, possibly via an arrangement of the carbon cation (Scheme 4a). As for the fluorine-containing amine products, while the N-protecting group is restricted as diphenylmethyl, it can facilely undergo deprotection with trifluoroacetic acid (TFA) to liberate the free amine 9 in 85% yield. The facile deprotection allows the installation of other functionalities on the nitrogen on demand. For example, the free amine 9 undergoes benzylation with benzyl bromide (BnBr), efficiently producing the desired molecule 10 in 96% yield (Scheme 4b). These reactions further enrich the diversity and complexity of the formal single bond metathesis products.
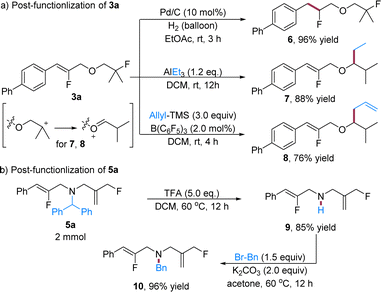 |
| Scheme 4 Synthetic applications. | |
We proposed a simplified catalytic cycle for the formal single bond metathesis reaction (Scheme 5). In the initial stage, a highly active cationic Rh(I) species is generated from the neutral Rh precatalyst in the presence of AgBF4. The gem-DFCP subsequently undergoes C–C bond oxidative addition to form rhodium complex A, which then experiences β-fluoride elimination to form the key intermediate fluoroallyl Rh(III) species B. Owing to the highly electrophilic properties of the species B, it can be attacked by a weak nucleophile of the oxygen in the epoxide, which generates an oxonium intermediate C. Following ring-opening, the resulting carbon cation of species D is trapped by the fluoride (from Rh–F or BF4),17b,24 which yields the product 3 and releases the rhodium catalyst to complete the catalytic cycle. For 3-methyleneazetidine as the coupling partner, the fluoroallyl Rh(III) species B undergoes nucleophilic attack by the nitrogen to give ammonium intermediate E, which is then trapped by the fluoride17b,24 at the methylene site in the ring (see ESI† for more details).
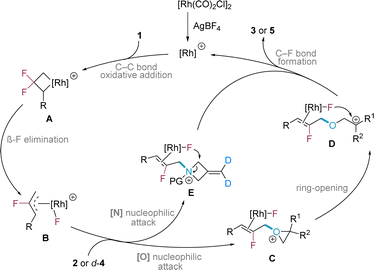 |
| Scheme 5 Proposed mechanism. | |
Conclusions
In conclusion, we have successfully developed a rhodium-catalyzed method for the formal C(sp3)–O/C(sp3)–F cross metathesis reaction between gem-DFCPs and epoxides. The reaction features the use of ether oxygen as a weak nucleophile and a remarkable 100% atom economy. The use of two strained three-membered substrates via double-strain release offers the driving force for the formal single-bond metathesis, which involves the cleavage and formation of many inert chemical bonds including C–C, C–O and C–F bonds. The developed method can also be applied to the formal C(sp3)–N/C(sp3)–F cross metathesis between gem-DFCPs and 3-methyleneazetidine. We believe such advancements will not only contribute to the research area of single-bond metathesis, but also provide efficient and versatile synthetic methods for the construction of complex fluorine-containing compounds.
Data availability
All the data have been presented in the manuscript and ESI.†
Author contributions
Y. Z. and J. J. contributed equally to this work. Y. X. conceived the project. Y. Z., J. J. and X. S. carried out the experiments. Y. Z., J. J., Y. X. and C. G. co-wrote the manuscript.
Conflicts of interest
The authors declare no conflict of interest.
Acknowledgements
This work is supported by the National Natural Science Foundation (grant no. 22371189 and 22001180) and the “Thousand Young Talents Program of China” (grant no. 15-YINGXIA). Dr Xuejiao Song, Dr Xunxun Deng and Dr Tianli Zheng in Public Health and Preventive Medicine Provincial Experiment Teaching Center at Sichuan University are acknowledged for the support for instrument management.
References
-
(a)
R. H. Grubbs, Handbook of Metathesis, Wiley-VCH, Weinheim, 2003 CrossRef;
(b) R. H. Grubbs, S. J. Miller and G. C. Fu, Acc. Chem. Res., 1995, 28, 446–452 CrossRef CAS;
(c) M. Schuster and S. Blechert, Angew. Chem., Int. Ed., 1997, 36, 2036–2056 CrossRef;
(d) A. Fürstner, Angew. Chem., Int. Ed., 2000, 39, 3012–3043 CrossRef;
(e) A. Hoveyda and A. Zhugralin, Nature, 2007, 450, 243–251 CrossRef CAS PubMed;
(f) O. M. Ogba, N. C. Warner, D. J. O'Leary and R. H. Grubbs, Chem. Soc. Rev., 2018, 47, 4510–4544 RSC;
(g) A. H. Hoveyda, C. Qin, X. Z. Sui, Q. Liu, X. Li and A. Nikbakht, Acc. Chem. Res., 2023, 56, 2426–2446 CrossRef CAS PubMed.
- For reviews, see:
(a) A. Deiters and S. F. Martin, Chem. Rev., 2004, 104, 2199–2238 CrossRef CAS;
(b) H. Albright, A. J. Davis, J. L. Gomez-Lopez, H. L. Vonesh, P. K. Quach, T. H. Lambert and C. S. Schindler, Chem. Rev., 2021, 121, 9359–9406 CrossRef CAS PubMed.
- For selected examples, see:
(a) J. Ludwig, P. Zimmerman and J. Gianino, Nature, 2016, 533, 374–379 CrossRef CAS PubMed;
(b) L. Ma, W. Li, H. Xi, X. Bai, E. Ma, X. Yan and Z. Li, Angew. Chem., Int. Ed., 2016, 55, 10410–10413 CrossRef CAS PubMed.
- For reviews, see:
(a) B. N. Bhawal and B. Morandi, Angew. Chem., Int. Ed., 2019, 58, 10074–10103 CrossRef CAS PubMed;
(b) B. Yu and H. Huang, Chin. J. Org. Chem., 2022, 42, 2376–2389 CrossRef CAS.
- For typical examples, see:
(a) D. Hollmann, S. Bahn, A. Tillack and M. Beller, Angew. Chem., Int. Ed., 2007, 46, 8291–8294 CrossRef CAS PubMed;
(b) N. A. Stephenson, J. Zhu, S. H. Gellman and S. S. Stahl, J. Am. Chem. Soc., 2009, 131, 10003–10008 CrossRef CAS PubMed;
(c) K. Baba, M. Tobisu and N. Chatani, Angew. Chem., Int. Ed., 2013, 52, 11892–11895 CrossRef CAS PubMed;
(d) Z. Lian, B. N. Bhawal, P. Yu and B. Morandi, Science, 2017, 356, 1059–1063 CrossRef CAS PubMed;
(e) S. Okumura, F. Sun, N. Ishida and M. Murakami, J. Am. Chem. Soc., 2017, 139, 12414–12417 CrossRef CAS PubMed;
(f) Y. Ma, L. Zhang, Y. Luo, M. Nishiura and Z. Hou, J. Am. Chem. Soc., 2017, 139, 12434–12437 CrossRef CAS PubMed;
(g) J. Zhu, R. Zhang and G. Dong, Nat. Chem., 2021, 13, 836–842 CrossRef CAS PubMed.
- K. Leszczyńska, A. Mix, R. J. F. Berger, B. Rummel, B. Neumann, H.-G. Stammler and P. Jutzi, Angew. Chem., Int. Ed., 2011, 50, 6843–6846 CrossRef PubMed.
- T. Biberger, S. Makai, Z. Lian and B. Morandi, Angew. Chem., Int. Ed., 2018, 57, 6940–6944 CrossRef CAS PubMed.
-
(a) N. Ansmann, T. Thorwart and L. Greb, Angew. Chem., Int. Ed., 2022, 61, e202210132 CrossRef CAS PubMed;
(b) F. S. Tschernuth, L. Bichlmaier and S. Inoue, ChemCatChem, 2023, 15, e202300281 CrossRef CAS;
(c) H. Liu, Q. Huang, R.-Z. Liao and Y. Xie, Nat. Commun., 2023, 14, 1883 CrossRef CAS PubMed.
-
(a) H. Wang, Y. Zhao, F. Zhang, Y. Wu, R. Li, J. Xiang, Z. Wang, B. Han and Z. Liu, Angew. Chem., Int. Ed., 2020, 59, 11850–11855 CrossRef CAS PubMed;
(b) J. L. Vidal, O. M. Wyper, S. L. MacQuarrie and F. M. Kerton, Eur. J. Org Chem., 2021, 2021, 6052–6056 CrossRef CAS.
-
(a)
V. P. Reddy, Organofluorine Chemistry, Elsvier, London, 2017, pp. 134–204 Search PubMed;
(b)
J. Eicher and N. Braun, Modern Synthesis Processes and Reactivity of Fluorinated Compounds, Elsvier, Oxford, 2020, pp. 8–23 Search PubMed;
(c) D. O'Hagan, Chem. Soc. Rev., 2008, 37, 308–319 RSC;
(d) S. Purser, P. R. Moore, S. Swallow and V. Gouverneur, Chem. Soc. Rev., 2008, 37, 320–330 RSC;
(e) C. Ni and J. Hu, Chem. Soc. Rev., 2016, 45, 5441–5454 RSC;
(f) D. L. Orsi and R. A. Altman, Chem. Commun., 2017, 53, 7168–7181 RSC;
(g) O. O. Grygorenko, K. P. Melnykov, S. Holovch and O. Demchuk, ChemCatChem, 2022, 17, e202200365 CAS.
-
(a) W. R. Dolbier and M. A. Battiste, Chem. Rev., 2003, 103, 1071–1098 CrossRef CAS PubMed;
(b) M. Fedoryński, Chem. Rev., 2003, 103, 1099–1132 CrossRef PubMed;
(c) K. S. Adekenova, P. B. Wyatt and S. M. Adekenova, Beilstein J. Org. Chem., 2021, 17, 245–272 CrossRef CAS PubMed;
(d) F. Wang, T. Luo, J. Hu, Y. Wang, H. S. Krishnan, P. V. Jog, S. K. Ganesh, G. K. S. Prakash and G. A. Olah, Angew. Chem., Int. Ed., 2011, 50, 7153–7157 CrossRef CAS PubMed;
(e) A. García-Domínguez, T. H. West, J. J. Primozic, K. M. Grant, C. P. Johnston, G. G. Cumming, A. G. Leach and G. C. Lloyd-Jones, J. Am. Chem. Soc., 2020, 142, 14649–14663 CrossRef PubMed.
- For reviews, see:
(a) X. Song, C. Xu and M. Wang, Tetrahedron Lett., 2017, 58, 1806–1816 CrossRef CAS;
(b) L. Lv, H. Qian and Z. Li, ChemCatChem, 2022, 14, e202200890 CrossRef CAS;
(c) Y. Zhu, Y. Zeng, Z.-T. Jiang and Y. Xia, Synlett, 2023, 34, 1–13 CrossRef CAS;
(d) Y. Zeng, Z.-T. Jiang and Y. Xia, Chem. Commun., 2024, 60, 3764–3773 RSC.
- For representative examples, see:
(a) E.-A. A. Xu, B. Xiao, Q.-Q. Lu, Y.-L. Wang, C.-G. Yu and Y. Fu, Angew. Chem., Int. Ed., 2015, 54, 8231–8235 CrossRef PubMed;
(b) J. Wenz, C. A. Rettenmeier, H. Wadepohl and L. H. Gade, Chem. Commun., 2016, 52, 202–205 RSC;
(c) J. Ni, B. Nishonov, A. Pardaev and A. Zhang, J. Org. Chem., 2019, 84, 13646–13654 CrossRef CAS PubMed;
(d) Z. Fu, J. Zhu, S. Guo and A. Lin, Chem. Commun., 2021, 57, 1262–1265 RSC;
(e) P.-Z. Zhou, X. Yang, J. Wang, C. Ge, W. Feng, Y.-M. Liang and Y. Zhang, Org. Lett., 2021, 23, 4920–4924 CrossRef CAS PubMed;
(f) B. Xiong, X. Chen, J. Liu, X. Zhang, Y. Xia and Z. Lian, ACS Catal., 2021, 11, 11960–11965 CrossRef CAS;
(g) L. Lv and C.-J. Li, Angew. Chem., Int. Ed., 2021, 60, 13098–13104 CrossRef CAS PubMed;
(h) L. Lv, H. Qian, Y. Ma, S. Huang, X. Yan and Z. Li, Chem. Sci., 2021, 12, 15511–15518 RSC;
(i) W. Yuan, X. Li, Z. Qi and X. Li, Org. Lett., 2022, 24, 2093–2098 CrossRef CAS PubMed;
(j) L. Lv, H. Qian, A. B. Crowell, S. Chen and Z. Li, ACS Catal., 2022, 12, 6495–6505 CrossRef CAS;
(k) L. Wu, M. Wang, Y. Liang and Z. Shi, Chin. J. Chem., 2022, 40, 2345–2355 CrossRef CAS;
(l) X. Wang and F. W. Patureau, Chem. Commun., 2023, 59, 486–489 RSC;
(m) J. Sun, H. Ye, F. Sun, Y.-Y. Pan, X.-W. Zhu and X.-X. Wu, Org. Lett., 2023, 25, 5220–5225 CrossRef CAS PubMed;
(n) H. Qian, H. D. Nguyen, L. Lv, S. Chen and Z. Li, Angew. Chem., Int. Ed., 2023, 62, e202303271 CrossRef CAS PubMed;
(o) H. Qian, Z. P. Cheng, Y. Luo, L. Lv, S. Chen and Z. Li, J. Am. Chem. Soc., 2024, 146, 24–32 CrossRef CAS PubMed;
(p) S. Qi, Y. Hua, L. Pan, J. Yang and J. Zhang, Chin. J. Chem., 2024, 42, 823–828 CrossRef CAS.
- For other representative reactions using gem-DFCPs without TM, see:
(a) S. M. Banik, K. M. Mennie and E. N. Jacobsen, J. Am. Chem. Soc., 2017, 139, 9152–9155 CrossRef CAS PubMed;
(b) H. Liu, Y. Li, D.-X. Wang, M.-M. Sun and C. Feng, Org. Lett., 2020, 22, 8681–8686 CrossRef CAS PubMed;
(c) H. Liu, L. Tian, H. Wang, Z.-Q. Li, C. Zhang, F. Xue and C. Feng, Chem. Sci., 2022, 13, 2686–2691 RSC;
(d) Y.-R. Zhao, Z.-Y. Ma, L. Liu, P. Hao, X.-H. Duan and M. Hu, J. Org. Chem., 2023, 88, 3787–3793 CrossRef CAS PubMed;
(e) B. Zhao, Z. Pan, J. Pan, H. Deng, X. Bu, M. Ma and F. Xue, Green Chem., 2023, 25, 3095–3102 RSC;
(f) X. Wu, Y. Zeng, Z.-T. Jiang, Y. Zhu, L. Xie and Y. Xia, Org. Lett., 2022, 24, 8429–8434 CrossRef CAS PubMed.
-
(a) Z.-T. Jiang, J. Huang, Y. Zeng, F. Hu and Y. Xia, Angew. Chem., Int. Ed., 2021, 60, 10626–10631 CrossRef CAS PubMed;
(b) Y. Zeng, H. Gao, Y. Zhu, Z.-T. Jiang, G. Lu and Y. Xia, ACS Catal., 2022, 12, 8857–8867 CrossRef CAS;
(c) Y. Zeng, H. Yang, J. Du, Q. Huang, G. Huang and Y. Xia, Chem. Sci., 2022, 13, 12419 RSC;
(d) Y. Zeng and Y. Xia, Angew. Chem., Int. Ed., 2023, 62, e202307129 CrossRef CAS PubMed;
(e) Z.-T. Jiang, Z. Chen and Y. Xia, Angew. Chem., Int. Ed., 2024, 63, e202319647 CrossRef CAS PubMed;
(f) X. Wu, X. Song and Y. Xia, Adv. Sci., 2024, 11, 2401243 CrossRef CAS PubMed;
(g) H. Yang, Y. Zeng, X. Song, L. Che, Z.-T. Jiang and Y. Xia, Angew. Chem., Int. Ed., 2024, 63, e202403602 CrossRef CAS PubMed.
- For representative examples on C–F bond reconstruction, see:
(a) H. Fujimoto, T. Kodama, M. Yamanaka and M. Tobisu, J. Am. Chem. Soc., 2020, 142, 17323–17328 CrossRef CAS PubMed;
(b) F. Wang, Y. Nishimoto and M. Yasuda, J. Am. Chem. Soc., 2021, 143, 20616–20621 CrossRef CAS PubMed;
(c) X. Yu, Q.-Y. Meng, C. G. Daniliuc and A. Studer, J. Am. Chem. Soc., 2022, 144, 7072–7079 CrossRef CAS PubMed;
(d) F. Wang, Y. Nishimoto and M. Yasuda, Angew. Chem., Int. Ed., 2022, 61, e202204462 CrossRef CAS PubMed;
(e) E. A. McKnight, R. Arora, E. Pradhan, Y. H. Fujisato, A. J. Ajayi, M. Lautens, T. Zeng and C. M. Le, J. Am. Chem. Soc., 2023, 145, 11012–11018 CrossRef CAS PubMed;
(f) T. Yoshida, M. Ohta, T. Emmei, T. Kodama and M. Tobisu, Angew. Chem., Int. Ed., 2023, 62, e202303657 CrossRef CAS PubMed;
(g) A. Garg, N. J. Gerwien, C. Fasting, A. Charlton and M. N. Hopkinson, Angew. Chem., Int. Ed., 2023, 62, e202302860 CrossRef CAS PubMed;
(h) X. Yu, A. Maity and A. Studer, Angew. Chem., Int. Ed., 2023, 62, e202310288 CrossRef CAS PubMed.
-
(a) D. Li, C. Shen, Z. Si and L. Liu, Angew. Chem., Int. Ed., 2023, 62, e202310283 CrossRef CAS PubMed;
(b) Y. Zeng, Z.-T. Jiang, Y. Zhu, J. Chen, H. Zhang and Y. Xia, Nat. Commun., 2024, 15, 4317 CrossRef CAS PubMed; the experimental section of this work was posted as:
(c)
Y. Zeng, Z.-T. Jiang, Y. Zhu, J. Chen, H. Zhang, Y. Xia, ChemRxiv, 2023, preprint, DOI:10.26434/chemrxiv-2023-8mj8n, a week later Liu's work (ref. 17a) online.
-
(a)
J. Alvarez-Builla, J. J. Vaquero and J. Barluenga, Modern Heterocyclic Chemistry, Wiley-VCH, Weinheim, 2011 CrossRef;
(b) C.-Y. Huang and A. G. Doyle, Chem. Rev., 2014, 114, 8153–8198 CrossRef CAS PubMed;
(c) C. Wang, Synthesis, 2017, 49, 5307–5319 CrossRef CAS;
(d) H.-H. Wang, X.-D. Wang, G.-F. Yin, Y.-F. Zeng, J. Chen and Z. Wang, ACS Catal., 2022, 12, 2330–2347 CrossRef CAS.
- For reviews, see:
(a) Y. Wang and Z.-X. Yu, Acc. Chem. Res., 2015, 48, 2288–2296 CrossRef CAS PubMed;
(b) G. Fumagalli, S. Stanton and J. F. Bower, Chem. Rev., 2017, 117, 9404–9432 CrossRef CAS PubMed;
(c) T. R. McDonald, R. Mills, M. S. West and S. A. L. Rousseaux, Chem. Rev., 2021, 121, 3–79 CrossRef CAS PubMed;
(d) J. Wang, S. A. Blaszczyk, X. Li and W. Tang, Chem. Rev., 2021, 121, 110–139 CrossRef CAS PubMed;
(e) Y. Cohen, A. Cohen and I. Marek, Chem. Rev., 2021, 121, 140–161 CrossRef CAS PubMed;
(f) V. Pirenne, B. Muriel and J. Waser, Chem. Rev., 2021, 121, 227–263 CrossRef CAS PubMed;
(g) R. Vicente, Chem. Rev., 2021, 121, 162–226 CrossRef CAS PubMed.
- For representative examples, see:
(a) Y. Zheng, W. Huang, R. K. Dhungana, A. Granados, S. Keess, M. Makvandi and G. A. Molander, J. Am. Chem. Soc., 2022, 144, 23685–23690 CrossRef CAS PubMed;
(b) T. Yu, J. Yang, Z. Wang, Z. Ding, M. Xu, J. Wen, L. Xu and P. Li, J. Am. Chem. Soc., 2023, 145, 4304–4310 CrossRef CAS PubMed;
(c) T. V. T. Nguyen, A. Bossonnet, M. D. Wodrich and J. Waser, J. Am. Chem. Soc., 2023, 145, 25411–25421 CrossRef CAS PubMed;
(d) S. Dutta, Y.-L. Lu, J. E. Erchinger, H. Shao, E. Studer, F. Schäfer, H. Wang, D. Rana, C. G. Daniliuc, K. N. Houk and F. Glorius, J. Am. Chem. Soc., 2024, 146, 5232–5241 CrossRef CAS PubMed;
(e) C.-L. Li, Y. Yang, Y. Zhou, Z.-C. Duan and Z.-X. Yu, J. Am. Chem. Soc., 2023, 145, 5496–5505 CrossRef CAS PubMed;
(f) N. Ishida, W. Ikemoto and M. Murakami, J. Am. Chem. Soc., 2014, 136, 5912–5915 CrossRef CAS PubMed;
(g) R. Li, B. Li, H. Zhang, C.-W. Ju, Y. Qin, X.-S. Xue and D. Zhao, Nat. Chem., 2021, 13, 1006–1016 CrossRef CAS PubMed;
(h) M. Liu, N. Yan, H. Tian, B. Li and D. Zhao, Angew. Chem., Int. Ed., 2024, 63, e202319187 CrossRef CAS PubMed.
- Deposition number 2347358 (for 5r) contains the supplementary crystallographic data for this paper. These data are provided free of charge by the joint Cambridge Crystallographic Data Centre and Fachinformationszentrum Karlsruhe Access Structures service.
- M. Aoyama and S. Hara, Tetrahedron, 2009, 65, 3682–3687 CrossRef CAS.
- K. Hirano, K. Fujita, H. Yorimitsu, H. Shinokubo and K. Oshima, Tetrahedron Lett., 2004, 45, 2555–2557 CrossRef CAS.
- Unlike our previous work (ref. 17b), where the C–F bond reconstruction was highly dependent on the BF4−/BF3 cycle, we found that the reactions proceed smoothly when AgBF4 is replaced with AgOTf or NaBArF (see Table 1, entry 11 for the ether substrate and Table S1,† entries 14 and 15 for the azetidine substrate). This suggests that C–F bond reconstruction here is more likely through the trap of cations by the Rh–F intermediate rather than via the BF4−/BF3− cycle.
Footnotes |
† Electronic supplementary information (ESI) available. Crystallographic data for 5r has been deposited at the CCDC under 2347358. For ESI and crystallographic data in CIF or other electronic format see DOI: https://doi.org/10.1039/d4sc03624g |
‡ These authors contributed equally to this work. |
|
This journal is © The Royal Society of Chemistry 2024 |
Click here to see how this site uses Cookies. View our privacy policy here.