DOI:
10.1039/D4SC00667D
(Edge Article)
Chem. Sci., 2024,
15, 6507-6514
Visible-light-enabled stereoselective synthesis of functionalized cyclohexylamine derivatives via [4 + 2] cycloadditions†
Received
28th January 2024
, Accepted 26th March 2024
First published on 26th March 2024
Abstract
An unprecedented intermolecular [4 + 2] cycloaddition of benzocyclobutylamines with α-substituted vinylketones, enabled by photoredox catalysis, has been developed. The current method enables facile access to highly functionalized cyclohexylamine derivatives that were otherwise inaccessible, in moderate to good yields with excellent diastereoselectivities. This protocol has some excellent features, such as full atom economy, good functional-group compatibility, mild reaction conditions, and an overall redox-neutral process. Additionally, an asymmetric version of this cycloaddition was preliminarily investigated via the incorporation of a chiral phosphoric acid (CPA), and moderate to good enantioselectivity could be effectively realized with excellent diastereoselectivity. Synthetic applications were demonstrated via a scale-up experiment and elaborations to access amino alcohol and cyclobutene derivatives. Based on the results of control experiments, a reasonable reaction mechanism was proposed to elucidate the reaction pathway.
Introduction
Cyclohexylamine and its derivatives are prevalent units in plenty of natural products, pharmaceuticals, agrochemicals, and biological molecules (Scheme 1a).1 For example, Rotigotine has been used for the treatment of Parkinson’s disease.1d Tametraline works as a norepinephrine–dopamine reuptake inhibitor.1a Terutroban is an important selective thromboxane prostanoid (TP) antagonist in medicinal chemistry.1e Owing to the great importance of these valuable structural motifs, it is necessary to establish efficient and elegant methods to access cyclohexylamine derivatives. The [4 + 2] cycloaddition is appreciated as a powerful synthetic tool to prepare valuable cycloalkane derivatives.2 The inherent ring-strain release of small-ring compounds serving as 1,n-synthons has been exploited as a powerful driving strategy through the cleavage of typically unreactive bonds, which has resulted in the wide use of these versatile molecules in organic synthesis.3 Among such small-ring compounds, the ring opening of cyclobutanes is generally less studied than that of cyclopropanes because of their smaller ring tensile force and slower ring-opening rate,4 but they could work as four-carbon synthons to prepare a diverse array of organic compounds to greatly broaden their use in organic synthesis.5
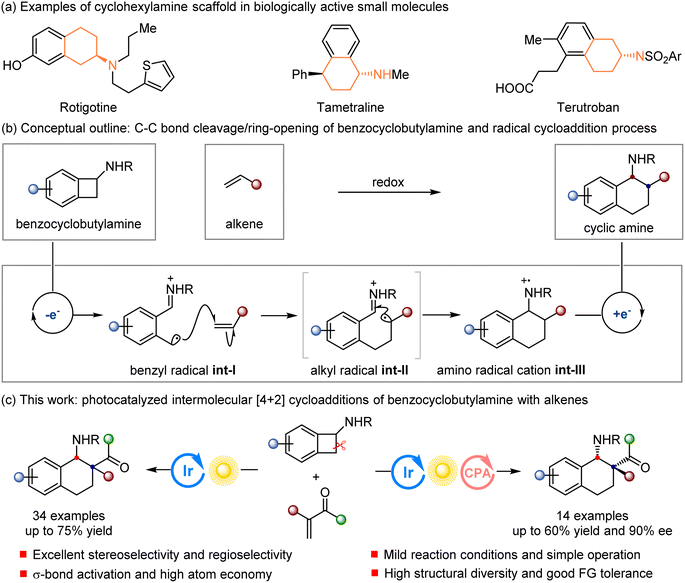 |
| Scheme 1 The proposal of photocatalyzed [4 + 2] cycloadditions for the synthesis of functionalized cyclohexylamine derivatives. | |
Transformations mediated by single-electron transfer (SET) have become a topic of intense study in the field of organic chemistry in recent decades, and have enabled the great application of easily available substrates with a wide tolerance of functional groups.6 We envisioned that such a powerful synthetic strategy could be utilized to prepare biologically important cyclohexylamine derivatives starting from readily accessible benzocyclobutylamines. As shown in Scheme 1b, a benzocyclobutylamine is expected to undergo one-electron oxidation for the formation of benzyl radical iminium ion int-I through an intramolecular ring-opening process. The subsequent radical addition of int-I to an alkene furnishes alkyl radical int-II, which undergoes a ring-closing step via the intramolecular addition of the alkyl radical to the iminium ion. Finally, the resulting amino radical cation int-III is reduced to achieve the final benzocyclohexylamine. The amine oxidation step can be mediated by enzymatic,7 chemical,8 electrochemical,9 and photochemical10 systems. Recently, photoredox catalysis has emerged as a powerful strategy to manipulate the redox chemistry of organic compounds, and features a strong ability to be merged with other catalysis types, high atom economy, mild reaction conditions, broad functional-group compatibility, and an overall redox-neutral process.11 Ring-opening-promoted cycloaddition reactions enabled by visible-light photoredox catalysis have been mainly focused on [3 + 2] cycloaddition reactions involving cyclopropylamines,3h,12 while cyclobutylamines, especially benzocyclobutylamines, as four-carbon synthons in [4 + 2] cycloaddition reactions have been rarely investigated.5 In 2017, Zheng and Wang developed an elegant visible-light-photocatalyzed intermolecular [4 + 2] annulation of benzocyclobutylanilines with alkynes, and the resulting intermediate 1,4-dihydronaphthalenes underwent a spontaneous elimination of anilines to generate naphthalenes.5d We envisioned that, using α-substituted vinyl ketones as the reaction partner, the cycloaddition reaction could be interrupted without the ensuing elimination.
With the design concept in Scheme 1b and the superiority of photoredox catalysis in mind, we envisaged that photoredox catalysis could be introduced in the [4 + 2] cycloaddition of benzocyclobutylamines with α-substituted vinyl ketones to construct functionalized synthetically valuable cyclohexylamine derivatives incorporating a unique all-carbon quaternary stereocenter.13 Two significant challenges need to be solved in the implementation of this protocol: (a) the ring opening of the benzocyclobutylamines would face three competitive C–C cleavage processes, resulting in different regioselectivities; (b) the highly stereoselective construction of cyclohexylamine derivatives containing adjacent tertiary and all-carbon quaternary stereocenters is always a formidable challenge and is disfavored in radical chemistry. Herein, we successfully realized a [4 + 2]-cycloaddition reaction of benzocyclobutylamines with unsaturated enones, enabled by photoredox catalysis, which would allow the forging of two contiguous stereogenic centers via C–C bond formation to access complex molecules with high regioselectivities and diastereoselectivities (Scheme 1c). In addition, a chiral phosphoric acid catalyst was introduced to provide a chiral environment for the generation of stereocenters by radical conjugate addition, and the asymmetric catalytic version of this cycloaddition could be realized via cooperative photoredox and chiral phosphoric acid catalysis.
Results and discussion
Optimization of reaction conditions
We began to examine the feasibility of our reaction design by using N-cyclobutyl aniline 1a and α-benzyl-substituted vinylketone 2a as the model substrates, promoting the reaction using a photosensitizer under irradiation with light. To our delight, the desired product 3a could be acquired in 75% yield with >20
:
1 dr when the transformation was performed with 2 mol% of Ir(dF(CF3)ppy)2(dtbbpy)]PF6 (PC-1) as a photosensitizer and NH4PF6 as an additive in a mixed solvent of THF/MeOH (10
:
1 volume ratio), under irradiation with a 7 W blue LED at 45 °C for 24 h (Table 1, entry 1, and see the ESI† for the comprehensive survey of reaction conditions). The preliminary condition survey of photosensitizers showed that they have a great effect on the reaction results and no further improvement was obtained (Table 1, entries 2–4). Then, we put emphasis on the base screening, and found that this [4 + 2] cycloaddition went smoothly in the presence of Na2HPO4 and K3PO4. Na2HPO4 provided a similar result to NH4PF6, affording product 3a in moderate yield with excellent diastereoselectivity (68% yield, >20
:
1 dr, Table 1, entry 5). Unsatisfactory diastereoselectivity was obtained when using K3PO4 as the base (63% yield, 3
:
1 dr, Table 1, entry 6). The subsequent solvent effect investigation revealed that pure MeOH and THF did not offer better results (Table 1, entries 7 and 8). Based on the reaction results of the bases and solvents, reaction temperature was also inspected to obtain better results (Table 1, entries 9 and 10). When the reaction temperature was increased from 45 °C to 65 °C, a decreased yield was obtained due to the decomposition of N-cyclobutyl aniline 1a. Poor reactivity was observed, with 46% yield, when this transformation was carried out at 25 °C (Table 1, entry 10). In addition, the photosensitizer, light and base are indispensable for this [4 + 2] cycloaddition process; no desired product 3a or poor reaction results were generated in the absence of any one of them (Table 1, entries 11–13; see ESI† for the details). In view of all reaction parameters, the optimal reaction conditions were established with good yield and excellent diastereoselectivity as displayed in entry 1.
Table 1 Optimization of reaction conditionsa
Substrate scope study
With the optimal reaction conditions in hand, the substrate scope of the photocatalyzed [4 + 2] cycloaddition between N-cyclobutyl anilines and α-substituted vinylketones was explored. These reaction results are summarized in Table 2. We found that N-cyclobutyl aniline substrates without a methoxy group (1b), or bearing benzyloxy (1c), dimethoxy (1d) groups on the benzo ring also worked as suitable reaction partners to deliver the desired annulation products 3b–3d in moderate yields (31–60%) with 13
:
1–>20
:
1 dr. A variety of N-cyclobutyl anilines were well tolerated; the substituent groups on the aryl ring have negligible effect on the reactivity and stereoselectivity. These substrates, bearing electron-withdrawing (CF3, CO2Et, Cl), electron-neutral (H) and electron-donating (MeO) groups on the phenyl ring, reacted smoothly to give the expected benzocyclohexylamine products 3a–3k in moderate yields with >20
:
1 dr. In addition, a wide range of α-benzyl substituted α,β-unsaturated ketones were applied to further investigate the substrate generality. It was found that the electronic properties and position of the substituted groups on the aromatic ring did not affect the reaction results; the corresponding benzocyclohexylamine products 3l–3q could be obtained in moderate to good yields (37–76%) with excellent diastereoselectivities (all >20
:
1 dr). Remarkably, a heteroaromatic 2-thienyl-substituted ketone was a viable substrate, affording the desired product 3r in good yield with moderate diastereoselectivity (65% yield, 4
:
1 dr). Moreover, alkyl ketones, namely cyclohexyl and phenylethyl substrates, worked efficiently, giving the expected products 3s and 3t in moderate yields with good diastereoselectivities (51% yield, 7
:
1 dr and 57% yield, 8
:
1 dr, respectively).
Table 2 Substrate scope study of photocatalyzed [4 + 2] cycloadditiona
Reaction conditions: PC-1 (2 mol%), 1 (0.2 mmol), 2 (1.5 equiv.), NH4PF6 (0.6 equiv.), THF/MeOH (10 : 1, 2 mL), 7 W blue LEDs, 24 h, isolated yield. Dr was determined via crude 1H NMR analysis.
|
|
Having examined the substrate scope of α-benzyl-substituted vinylketones, we turned our attention to investigating vinylketones with various substituents on the benzyl aromatic ring. A diverse range of vinylketones with different substituent groups on the benzyl aromatic ring performed efficiently in this cycloaddition reaction, delivering the corresponding benzocyclohexylamine products 3u–3z in moderate yields (50–64% yields) with excellent diastereoselectivity (all >20
:
1 dr). It is worth noting that an α-thienyl-substituted vinylketone also reacted well in this catalytic system to obtain the corresponding product 3aa in 66% yield with >20
:
1 dr. α-Naphthyl-substituted vinylketones with steric hindrance also underwent this transformation smoothly, generating the desired products 3bb and 3cc in moderate yields with excellent diastereoselectivities (55% yield, >20
:
1 dr and 63% yield, >20
:
1 dr, respectively). When the benzyl group in the vinylketone was replaced with other substituent groups, namely an α-neopentyl group, α-phenylpropyl group, chlorine atom, or phenyl group, this cycloaddition proceeded smoothly to furnish the corresponding products 3dd–3gg with moderate to excellent results (35–70% yields, 3
:
1–>20
:
1 dr). The above successes encouraged us to explore the viability of this catalytic platform for a challenging cyclic-ketone-derived terminal olefin. To our delight, an α,β-unsaturated-ketone-containing seven-membered ring also reacted with the N-cyclobutyl aniline substrate 1a smoothly, and the fascinating spirocyclic product 3hh could be obtained with moderate results (46% yield, 3
:
1 dr). In addition, N-cyclobutyl-4-(trifluoromethyl)aniline was investigated, and no reaction was observed. 1-Phenylprop-2-en-1-one was also examined; due to its instability and easy self-polymerization, the corresponding annulation product was obtained in less than 10% yield and without elimination of an amine byproduct. The relative configuration of product rac-3a was unambiguously determined via X-ray diffraction analysis (CCDC 2326949†),14 and those of the other cycloadducts were deduced on the basis of these results.
It is well-known that chiral amines and derivatives not only work as useful building blocks and chiral auxiliaries in asymmetric synthesis, but also frequently appear in a number of natural products and bioactive molecules.15 Among these versatile molecules, chiral cyclohexylamines and derivatives as important structural units are often found in diverse biologically active molecules.16 Therefore, developing reliable and facile synthetic approaches to access these useful scaffolds has remained a significant topic of interest. Motivated by the importance of chiral cyclohexylamines and derivatives, we intend to introduce chiral elements into the reaction system for the construction of chiral cyclohexylamine backbones. Owing to the extremely short lifetime of radicals and strong racemic background transformation, it is very challenging and remarkably difficult to realize sufficient enantiofacial differentiation with excellent control.17 We envisioned that good enantiocontrol may be achieved via hydrogen-bonding interactions between a chiral phosphoric acid catalyst and the substrates. Therefore, a series of chiral phosphoric acids (CPAs)18 were employed to promote the photocatalyzed [4 + 2] cycloaddition reaction between 1a and 2a (see the ESI† for the details). After systematic reaction-condition screening, chiral annulation product (1S,2S)-3a could be obtained in good yield with excellent diastereoselectivity and good enantioselectivity (60% yield, >20
:
1 dr, 85
:
15 er) promoted by the CPA (R)-C7 containing a 4-tBu-phenyl group on the 3-position of the naphthol skeleton.
With the optimal reaction conditions in hand, we turned our attention to the investigation of the substrate scope of this asymmetric [4 + 2] cycloaddition promoted by photo-redox/CPA synergistic catalysis. As summarized in Table 3, the compatibility of different N-cyclobutyl aniline substrates with a trifluoromethyl group, ethyl group or chlorine atom on the phenyl ring was firstly examined. The corresponding products (1S,2S)-3a, (1S,2S)-3f and (1S,2S)-3g could be obtained in moderate yields with high stereoselectivities and good enantioselectivities (47–60% yields, >20
:
1 dr, 75
:
25–85
:
15 er). Then, a number of α-benzyl-substituted vinylketones bearing electron-rich (meta-Me or ortho-Me), or electron-deficient (para-, meta- or ortho-Cl) groups on the aromatic ring performed well to afford the desired benzocyclohexylamine products ((1S,2S)-3m–(1S,2S)-3q) in moderate yields (36–56% yields), moderate to good enantioselectivities (80
:
20 to 95
:
5 er) and excellent diastereoselectivities (all >20
:
1 dr). In addition, a wide range of vinylketones bearing different substituent groups on the benzyl aromatic rings are suitable for this asymmetric cycloaddition protocol, delivering the benzocyclohexylamine ((1S,2S)-3u–(1S,2S)-3y) in 43–61% yields, 78
:
22 to 84
:
16 er, and >20
:
1 dr. Moreover, an α-thienyl-substituted vinylketone was well-tolerated, giving the corresponding cycloaddition product (1S,2S)-3aa with 60% yield, 84
:
16 er, and >20
:
1 dr. It is worth noting that the ee values of these products could be easily improved to excellent levels of 96
:
4 to 99.5
:
0.5 er after simple recrystallization. The absolute configuration of (1S,2S)-3a was unambiguously determined via X-ray diffraction analysis (CCDC 2321837†).14
Table 3 Preliminary study of asymmetric photocatalyzed [4 + 2] cycloadditiona

|
Entry |
R1 |
R2 |
R3 |
3
|
Yieldb (%) |
erc |
Reaction conditions: PC-1 (2 mol%), (R)-C7 (10 mol%), 1 (0.2 mmol), 2 (1.5 equiv.), NH4PF6 (0.6 equiv.), THF (2 mL), 7 W blue LEDs, 24 h.
Isolated yield.
The er values were determined via HPLC analysis. The er values within parentheses were determined after recrystallization.
|
1 |
p-CF3-C6H4 |
Bn |
C6H5 |
(1S,2S)-3a |
60 |
85 : 15 (99.5 : 0.5) |
2 |
p-EtO2C-C6H4 |
Bn |
C6H5 |
(1S,2S)-3f |
47 |
77.5 : 22.5 (96 : 4) |
3 |
p-Cl-C6H4 |
Bn |
C6H5 |
(1S,2S)-3g |
50 |
75 : 25 (98 : 2) |
4 |
p-CF3-C6H4 |
Bn |
m-Me-C6H4 |
(1S,2S)-3m |
48 |
80 : 20 (98.5 : 1.5) |
5 |
p-CF3-C6H4 |
Bn |
o-Me-C6H4 |
(1S,2S)-3n |
54 |
95 : 5 (97 : 3) |
6 |
p-CF3-C6H4 |
Bn |
p-Cl-C6H4 |
(1S,2S)-3o |
56 |
84 : 16 (99 : 1) |
7 |
p-CF3-C6H4 |
Bn |
m-Cl-C6H4 |
(1S,2S)-3p |
53 |
80 : 20 (98 : 2) |
8 |
p-CF3-C6H4 |
Bn |
o-Cl-C6H4 |
(1S,2S)-3q |
36 |
94 : 6 (96 : 4) |
9 |
p-CF3-C6H4 |
p-MeO-C6H4CH2 |
C6H5 |
(1S,2S)-3u |
54 |
82.5 : 17.5 (99 : 1) |
10 |
p-CF3-C6H4 |
m-MeO-C6H4CH2 |
C6H5 |
(1S,2S)-3v |
43 |
83 : 17 (98.5 : 1.5) |
11 |
p-CF3-C6H4 |
o-MeO-C6H4CH2 |
C6H5 |
(1S,2S)-3w |
43 |
78 : 22 (98.5 : 1.5) |
12 |
p-CF3-C6H4 |
p-Cl-C6H4CH2 |
C6H5 |
(1S,2S)-3x |
61 |
84 : 16 (99 : 1) |
13 |
p-CF3-C6H4 |
m-Cl-C6H4CH2 |
C6H5 |
(1S,2S)-3y |
58 |
83 : 17 (99 : 1) |
14 |
p-CF3-C6H4 |
2-Thienyl |
C6H5 |
(1S,2S)-3aa |
60 |
84 : 16 (99.5 : 0.5) |
Scale-up experiments and synthetic application
To further reveal the synthetic utility of this protocol, the photocatalyzed [4 + 2] cycloaddition of model substrates N-cyclobutyl aniline 1a and α-substituted vinylketone 2a was carried out on a 1.0 mmol scale under standard conditions. The desired annulation product 3a could be readily obtained while maintaining the yield and diastereoselectivity (69% yield, >20
:
1 dr, Scheme 2a). In addition, the enantioselective version also performed well, giving the chiral product (1S,2S)-3a while maintaining the previous results (59% yield, >20
:
1 dr, 85
:
15 er), and a high ee value can be obtained after simple recrystallization. Moreover, synthetic transformations of this highly functionalized annulation product 3a could be carried out to access other molecules that are inaccessible with known methods (Scheme 2b). For example, the ketone moiety in 3a was easily reduced by treatment with LiAlH4, resulting in amino alcohol product 4 in 71% yield with >20
:
1 dr. In addition, the fascinating cyclobutene product 5 could be formed in 92% yield in the presence of a Wittig reagent. The structure of rac-5 was unambiguously determined via X-ray diffraction analysis (CCDC 2321838†).14
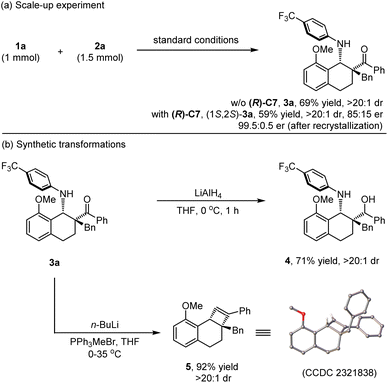 |
| Scheme 2 Scale-up experiments and synthetic elaborations. | |
Mechanistic studies
To gain mechanistic insights into this photocatalyzed [4 + 2] cycloaddition, several control experiments were performed. As shown in Scheme 3a, 2,2,6,6-tetramethylpiperidine-N-oxyl (TEMPO) was added to the reaction system under standard conditions as a radical scavenger. It was found that the transformation was completely inhibited and product 3a was not detected. This reaction result provides strong evidence to support the involvement of radical intermediates in the reaction process. Next, Stern–Volmer quenching experiments were performed using model substrates 1a and 2a. Strong quenching reaction results of photoexcited [IrIII*]
19 by substrate 1a (Eox1/2 = +1.13 V vs. SCE)5d favored a reductive quenching cycle (Scheme 3b). In contrast, substrate 2a did not quench the excited catalyst (Scheme 3c). Based on the control-experiment results, a possible mechanism for this cycloaddition is proposed in Scheme 3d. It begins with the one-electron oxidation of benzocyclobutylamine 1 by the excited-state photocatalyst [IrIII*] via a SET process to afford the reduced [IrII] and the amino radical cation int I, which goes through ring opening at the distal C–C bond and results in the formation of benzyl radical cation int II. Subsequently, the addition of int II to α-substituted vinylketone 2 furnished alkyl radical int III. The subsequent ring-closing process was achieved via intramolecular addition of the alkyl radical to the iminium ion to provide amino radical cation int IV. This was then reduced by the [IrII] species to give the desired product, benzocyclohexylamine 3. According to the absolute configuration of chiral annulation product 3a, a possible chiral phosphoric acid catalytic model is proposed in Scheme 3e. The non-covalent hydrogen bonding interactions between intermediate int III and the (R)-CPA catalyst were conducive to achieving good control of enantioselectivity in the intramolecular addition step of the alkyl radical to the imine.
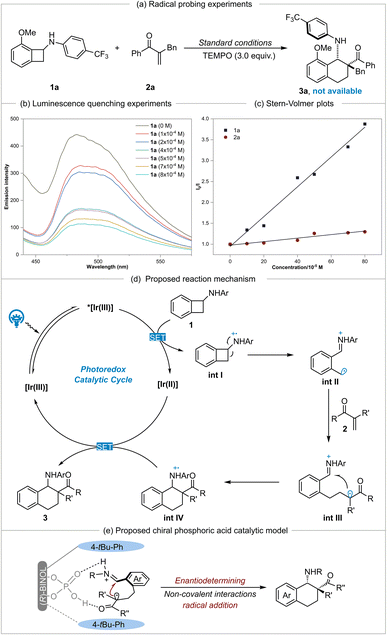 |
| Scheme 3 Control experiments and proposed reaction mechanism. | |
Conclusions
In summary, the first photocatalyzed intermolecular [4 + 2] cycloaddition of benzocyclobutylamines with α-substituted vinylketones was successfully developed, and a wide range of benzocyclohexylamines could be readily accessed in moderate to good yields with excellent diastereoselectivities. This protocol features broad substrate scope, good functional-group tolerance and mild reaction conditions. Additionally, the incorporation of a CPA in this photoredox catalysis ensures excellent diastereocontrol with moderate to good enantiocontrol over the newly formed C–C bond and the asymmetric version of this [4 + 2] cycloaddition was preliminarily realized. A reasonable reaction mechanism was proposed for this transformation, with the reaction pathway being elucidated on the basis of control-experiment results. A scale-up experiment and synthetic transformations to access amino alcohol and cyclobutene derivatives give this protocol potential applications in organic synthesis.
Data availability
All experimental procedures, characterisation data, mechanistic investigations, NMR spectra and HPLC spectra can be found in the ESI.†
Author contributions
C. J. W. conceptualized the project. C. J. W. and X. Q. D. supervised the investigation. Y. N. L., C. C., G. Z. and X. C. performed the research. C. J. W., X. Q. D. and Y. N. L. co-wrote the paper. All authors analyzed the data, discussed the results, and commented on the manuscript.
Conflicts of interest
There are no conflicts to declare.
Acknowledgements
This work was supported by the National Key R&D Program of China (2023YFA1506700), NSFC (22071186, 22071187, 22101216, 22271226, and 22371216), National Youth Talent Support Program, and Hubei Province NSF (2021CFA069). The authors thank Dr Ran Zhang from the Core Facility of Wuhan University for his generous support in the X-ray structure analysis.
Notes and references
-
(a) F. B. Thomas and N. G. Margaret, J. Pharmacol. Exp. Ther., 1974, 191, 518 Search PubMed;
(b) M. Periasamy, N. S. Kumar, S. Sivakumar, V. D. Rao, C. R. Ramanathan and L. Venkatraman, J. Org. Chem., 2001, 66, 3828–3833 CrossRef CAS PubMed;
(c) J. J. Chen, D. M. Swope, K. Dashtipour and K. E. Lyons, Pharmacotherapy, 2009, 29, 1452–1467 CrossRef CAS PubMed;
(d) M. H. S. A. Hamid, C. L. Allen, G. W. Lamb, A. C. Maxwell, H. C. Maytum, A. J. A. Watson and J. M. J. Williams, J. Am. Chem. Soc., 2009, 131, 1766–1774 CrossRef CAS PubMed;
(e) P.-F. Lesault, L. Boyer, G. Pelle, A. Covali-Noroc, D. Rideau, S. Akakpo, E. Teiger, J.-L. Dubois-Randé and S. Adnot, Br. J. Clin. Pharmacol., 2011, 71, 844–851 CrossRef CAS PubMed;
(f) X. Li, X. Ren, M. Guo, W. Li and Q. Yang, J. Catal., 2022, 413, 546–553 CrossRef CAS.
-
(a) T. G. Back, K. N. Clary and D. Gao, Chem. Rev., 2010, 110, 4498–4553 CrossRef CAS PubMed;
(b) Y. Liu, R. Song and J. Li, Sci. China: Chem., 2016, 59, 161–170 CrossRef CAS;
(c) B.-s. Jeon, S.-A. Wang, M. W. Ruszczycky and H.-w. Liu, Natural [4 + 2]-Cyclases, Chem. Rev., 2017, 117, 5367–5388 CrossRef CAS PubMed;
(d) X.-E. Cai, Z.-Y. Wang, W.-C. Tian, H. Liu, W.-T. Wei and K.-W. Lei, Org. Biomol. Chem., 2023, 21, 6068–6082 RSC.
-
(a) J. E. Baldwin, Chem. Rev., 2003, 103, 1197–1212 CrossRef CAS PubMed;
(b) P. R. Khoury, J. D. Goddard and W. Tam, Tetrahedron, 2004, 60, 8103–8112 CrossRef CAS;
(c) L. Jiao and Z.-X. Yu, J. Org. Chem., 2013, 78, 6842–6848 CrossRef CAS PubMed;
(d) T. F. Schneider, J. Kaschel and D. B. Werz, Angew. Chem., Int. Ed., 2014, 53, 5504–5523 CrossRef CAS PubMed;
(e) M. Murakami and N. Ishida, Chem. Rev., 2021, 121, 264–299 CrossRef CAS PubMed;
(f) J. Wang, S. A. Blaszczyk, X. Li and W. Tang, Chem. Rev., 2021, 121, 110–139 CrossRef CAS PubMed;
(g) P. Bellotti and F. Glorius, J. Am. Chem. Soc., 2023, 145, 20716–20732 CrossRef CAS PubMed;
(h) A. S. Harmata, B. J. Roldan and C. R. J. Stephenson, Angew. Chem., Int. Ed., 2023, 62, e202213003 CrossRef CAS PubMed.
-
(a) Y. Maeda and K. U. Ingold, J. Am. Chem. Soc., 1980, 102, 328–331 CrossRef CAS;
(b) J. H. Horner, F. N. Martinez, O. M. Musa, M. Newcomb and H. E. Shahin, J. Am. Chem. Soc., 1995, 117, 11124–11133 CrossRef CAS;
(c) O. M. Musa, J. H. Horner, H. Shahin and M. Newcomb, J. Am. Chem. Soc., 1996, 118, 3862–3868 CrossRef CAS;
(d) R. D. Bach and O. Dmitrenko, J. Am. Chem. Soc., 2004, 126, 4444–4452 CrossRef CAS PubMed;
(e)
K. B. Wiberg, in The Chemistry of Cyclobutanes, ed. Z. Z. Rappoport and J. F. Liebman, Wiley, Chichester, 2005, pp. 1–15 Search PubMed;
(f) J. Jin and M. Newcomb, J. Org. Chem., 2008, 73, 4740–4742 CrossRef CAS PubMed;
(g) W. Wu, B. Ma, J. I-Chia Wu, P. v. R. Schleyer and Y. Mo, Chem.–Eur. J., 2009, 15, 9730–9736 CrossRef CAS PubMed.
-
(a) J. Wang and N. Zheng, Angew. Chem., Int. Ed., 2015, 54, 11424–11427 CrossRef CAS PubMed;
(b) J. Wang, T. H. Nguyen and N. Zheng, Sci. China: Chem., 2016, 59, 180–183 CrossRef CAS;
(c) J. Wang, C. Mao, P. Feng and N. Zheng, Chem.–Eur. J., 2017, 23, 15396–15403 CrossRef CAS PubMed;
(d) Q. Wang and N. Zheng, ACS Catal., 2017, 7, 4197–4201 CrossRef CAS;
(e) Z. Luo, Z. Xing, R. Gao, Y. Han, J. Ren and Z. Wang, Org. Biomol. Chem., 2023, 21, 4637–4642 RSC;
(f) M. Chaumontet, P. Retailleau and O. Baudoin, J. Org. Chem., 2009, 74, 1774–1776 CrossRef CAS PubMed;
(g) M. Chaumontet, R. Piccardi and O. Baudoin, Angew. Chem., Int. Ed., 2009, 48, 179–182 CrossRef CAS PubMed.
-
(a) A. Studer and D. P. Curran, Nat. Chem., 2014, 6, 765–773 CrossRef CAS PubMed;
(b) N. Zhang, S. R. Samanta, B. M. Rosen and V. Percec, Chem. Rev., 2014, 114, 5848–5958 CrossRef CAS PubMed;
(c) M. Yan, J. C. Lo, J. T. Edwards and P. S. Baran, J. Am. Chem. Soc., 2016, 138, 12692–12714 CrossRef CAS PubMed;
(d) J. D. Bell and J. A. Murphy, Chem. Soc. Rev., 2021, 50, 9540–9685 RSC.
-
(a) G. Ottolina, S. Bianchi, B. Belloni, G. Carrea and B. Danieli, Tetrahedron Lett., 1999, 40, 8483–8486 CrossRef CAS;
(b) K.-Q. Ling, W.-S. Li and L. M. Sayre, J. Am. Chem. Soc., 2008, 130, 933–944 CrossRef CAS PubMed.
-
(a) R. K. Neff, Y.-L. Su, S. Liu, M. Rosado, X. Zhang and M. P. Doyle, J. Am. Chem. Soc., 2019, 141, 16643–16650 CrossRef CAS PubMed;
(b) B. Górski, A.-L. Barthelemy, J. J. Douglas, F. Juliá and D. Leonori, Nat. Catal., 2021, 4, 623–630 CrossRef.
-
(a) Y. Wang, X.-J. Zhao, X. Wu, L. Zhang, G. Li and Y. He, ChemElectroChem, 2022, 9, e202200378 CrossRef CAS;
(b) X. Sun and K. Zheng, Nat. Commun., 2023, 14, 6825 CrossRef CAS PubMed;
(c) Z. Tan, H. Zhang, K. Xu and C. Zeng, Sci. China: Chem., 2024, 67, 450–470 CrossRef CAS.
-
(a) T. Constantin, M. Zanini, A. Regni, N. S. Sheikh, F. Juliá and D. Leonori, Science, 2020, 367, 1021–1026 CrossRef CAS PubMed;
(b) L. Caiger, C. Sinton, T. Constantin, J. J. Douglas, N. S. Sheikh, F. Juliá and D. Leonori, Chem. Sci., 2021, 12, 10448–10454 RSC;
(c) Y. Kwon, J. Lee, Y. Noh, D. Kim, Y. Lee, C. Yu, J. C. Roldao, S. Feng, J. Gierschner, R. Wannemacher and M. S. Kwon, Nat. Commun., 2023, 14, 92 CrossRef CAS PubMed.
-
(a) A. R. Allen, E. A. Noten and C. R. J. Stephenson, Chem. Rev., 2022, 122, 2695–2751 CrossRef CAS PubMed;
(b) L. Capaldo, D. Ravelli and M. Fagnoni, Chem. Rev., 2022, 122, 1875–1924 CrossRef CAS PubMed;
(c) A. Y. Chan, I. B. Perry, N. B. Bissonnette, B. F. Buksh, G. A. Edwards, L. I. Frye, O. L. Garry, M. N. Lavagnino, B. X. Li, Y. Liang, E. Mao, A. Millet, J. V. Oakley, N. L. Reed, H. A. Sakai, C. P. Seath and D. W. C. MacMillan, Chem. Rev., 2022, 122, 1485–1542 CrossRef CAS PubMed;
(d) L. Chang, Q. An, L. Duan, K. Feng and Z. Zuo, Chem. Rev., 2022, 122, 2429–2486 CrossRef CAS PubMed;
(e) K. P. S. Cheung, S. Sarkar and V. Gevorgyan, Chem. Rev., 2022, 122, 1543–1625 CrossRef PubMed;
(f) M. J. Genzink, J. B. Kidd, W. B. Swords and T. P. Yoon, Chem. Rev., 2022, 122, 1654–1716 CrossRef CAS PubMed;
(g) N. Holmberg-Douglas and D. A. Nicewicz, Chem. Rev., 2022, 122, 1925–2016 CrossRef CAS PubMed;
(h) F. Juliá, T. Constantin and D. Leonori, Chem. Rev., 2022, 122, 2292–2352 CrossRef PubMed;
(i) P. R. D. Murray, J. H. Cox, N. D. Chiappini, C. B. Roos, E. A. McLoughlin, B. G. Hejna, S. T. Nguyen, H. H. Ripberger, J. M. Ganley, E. Tsui, N. Y. Shin, B. Koronkiewicz, G. Qiu and R. R. Knowles, Chem. Rev., 2022, 122, 2017–2291 CrossRef CAS PubMed;
(j) N. E. S. Tay, D. Lehnherr and T. Rovis, Chem. Rev., 2022, 122, 2487–2649 CrossRef CAS PubMed;
(k) X.-Y. Yu, J.-R. Chen and W.-J. Xiao, Chem. Rev., 2021, 121, 506–561 CrossRef CAS PubMed.
-
(a) Y. Yin, Y. Li, T. P. Gonçalves, Q. Zhan, G. Wang, X. Zhao, B. Qiao, K.-W. Huang and Z. Jiang, J. Am. Chem. Soc., 2020, 142, 19451–19456 CrossRef CAS PubMed;
(b) M.-M. Wang, T. V. T. Nguyen and J. Waser, Chem. Soc. Rev., 2022, 51, 7344–7357 RSC;
(c) Y. Dai, H. Huang, S. Liang, Y. Yin, X. Ban, X. Zhao and Z. Jiang, Org. Lett., 2023, 25, 4551–4555 CrossRef CAS PubMed.
- Z.-Y. Cao, F. Zhou and J. Zhou, Acc. Chem. Res., 2018, 51, 1443–1454 CrossRef CAS PubMed.
- Deposition numbers 2326949 (for rac-3a), 2321837 (for (1S,2S)-3a), and 2321838 (for rac-5) contain the supplementary crystallographic data for this paper.†.
-
(a) T. C. Nugent and M. El-Shazly, Adv. Synth. Catal., 2010, 352, 753–819 CrossRef CAS;
(b) A. Cabré, X. Verdaguer and A. Riera, Chem. Rev., 2022, 122, 269–339 CrossRef PubMed.
-
(a) T. F. Spande, H. M. Garraffo, M. W. Edwards, H. J. C. Yeh, L. Pannell and J. W. Daly, J. Am. Chem. Soc., 1992, 114, 3475–3478 CrossRef CAS;
(b) D. H. Appella, L. A. Christianson, I. L. Karle, D. R. Powell and S. H. Gellman, J. Am. Chem. Soc., 1996, 118, 13071–13072 CrossRef CAS;
(c)
G. A. Cordell, in The Alkaloids, Chemistry and Physiology, ed. R. H. F. Manske and R. G. A. Rodrigo, Academic Press, New York, 1979, ch. 3, vol. 17, pp. 199–384 Search PubMed;
(d)
J. E. Saxton, in The Alkaloids, Chemistry and Biology, ed. G. A. Cordell, Academic Press, San Diego, 1998, ch. 1, vol. 51, pp. 1–197 Search PubMed;
(e) F. Fülöp, Chem. Rev., 2001, 101, 2181–2204 CrossRef PubMed;
(f) M. M. Uy, S. Ohta, M. Yanai, E. Ohta, T. Hirata and S. Ikegami, Bioorg. Med. Chem. Lett., 2002, 12, 3037–3039 CrossRef CAS PubMed;
(g) Z. Jin, Nat. Prod. Rep., 2013, 30, 849–868 RSC;
(h) Z. Zhang, N. A. Butt and W. Zhang, Chem. Rev., 2016, 116, 14769–14827 CrossRef CAS PubMed;
(i) C. Liu, X. Yan, M. Wang, P. Qin, Z. Qi, M. Ji, X. Liu, P. V. Babu, X. Li and Z.-N. Cui, Bioorg. Med. Chem. Lett., 2017, 27, 271–276 CrossRef CAS PubMed;
(j) S. Tan, M. G. Banwell, W.-C. Ye, P. Lan and L. V. White, Chem.–Asian J., 2022, 17, e202101215 CrossRef CAS PubMed.
-
(a) F. Wang, P. Chen and G. Liu, Acc. Chem. Res., 2018, 51, 2036–2046 CrossRef CAS PubMed;
(b) X. Huang and E. Meggers, Acc. Chem. Res., 2019, 52, 833–847 CrossRef CAS PubMed;
(c) Q.-S. Gu, Z.-L. Li and X.-Y. Liu, Acc. Chem. Res., 2020, 53, 170–181 CrossRef CAS PubMed;
(d) Z.-L. Li, G.-C. Fang, Q.-S. Gu and X.-Y. Liu, Chem. Soc. Rev., 2020, 49, 32–48 RSC.
-
(a) T. Akiyama, J. Itoh, K. Yokota and K. Fuchibe, Angew. Chem. Int. Ed., 2004, 43, 1566–1568 CrossRef CAS PubMed;
(b) D. Uraguchi and M. Terada, J. Am. Chem. Soc., 2004, 126, 5356–5357 CrossRef CAS PubMed.
- C. K. Prier, D. A. Rankic and D. W. C. MacMillan, Chem. Rev., 2013, 113, 5322–5363 CrossRef CAS PubMed.
|
This journal is © The Royal Society of Chemistry 2024 |
Click here to see how this site uses Cookies. View our privacy policy here.