DOI:
10.1039/D4RA03725A
(Paper)
RSC Adv., 2024,
14, 29184-29188
Suzuki–Miyaura cross-coupling of unprotected ortho-bromoanilines with benzyl, alkyl, aryl, alkenyl and heteroaromatic boronic esters†
Received
20th May 2024
, Accepted 14th August 2024
First published on 16th September 2024
Abstract
A Suzuki–Miyaura cross-coupling reaction was developed on unprotected ortho-bromoanilines. This operationally simple reaction was developed for the diversification of glucocorticoid receptor modulators (GRMs), showed compatibility to various boronic esters featuring unique functionalities, and was demonstrated on a gram scale.
The widely used Suzuki–Miyaura cross-coupling reaction employs the use of a palladium catalyst to generate carbon–carbon bonds between an organohalide or triflate and an organoboron nucleophile.1 Its wide use can be attributed to its mild reaction conditions, low toxicity, and commercial availability of starting materials.2 Of note, is the application towards natural product synthesis, pharmaceuticals, and fine chemical industries.3 Ortho-substituted anilines are a key structural element in several pharmacologically active compounds. Examples include inhibitors of fatty acid amide hydrolase (FAAH), phosphodiesterase-4 (PDE4), compounds for the prevention of nonalcoholic fatty liver disease (NAFLD), and angiotensin II receptor antagonists (Fig. 1a).4 Although the Suzuki–Miyaura reaction has been widely utilized, substrates with unprotected ortho-anilines are less common. Particularly challenging are sp2–sp3 couplings. And thus, the development of a cross-coupling method focusing on unprotected ortho-bromoanilines is of wide interest to the broader chemistry community. Reported methods for this type of coupling typically require protection of the free amine.5 Alternatively, methods exist with direct reactivity on various 2-haloanilines but with limitations on the substrates investigated and functionalities that can be tolerated.6 Our work focuses on expanding the scope of the reactivity of ortho-bromoanilines, with a focus on developing an operationally simple method (Fig. 1b).
 |
| Fig. 1 (a) Examples of pharmacologically active compounds containing ortho-substituted anilines. (b) Our work on coupling unprotected ortho-bromoanilines with benzyl, alkyl, aryl, alkenyl and heteroaromatic boronic esters. | |
As a part of a program to identify glucocorticoid receptor modulators (GRMs),7 the Suzuki cross-coupling of 1a was attempted as the diversification step for the preparation of a compound library.8 Using Pd(dppf)Cl2 as the catalyst with K2CO3 as base with boronate 2a in 10
:
1 dioxane/H2O at 90 °C, desired product 3a was isolated in 11% yield (Fig. 2). The low isolated yield was consistent with conversion by HPLC.
 |
| Fig. 2 Initial attempt with GRM substrate. | |
Since this result suggested a lack of catalyst turnover, a high throughput screen was conducted using ChemBeads9 to identify an optimal catalyst/ligand/base system with the goal of improving the yield. The best conditions identified from the screen used the preformed CataCXium A palladacycle10 with Cs2CO3 as the base in dioxane/H2O at 80 °C. These results were repeated on a 0.1 mmol scale at a 0.1 M concentration of 1a with 2 equiv. of boronate to give 51% yield of the desired product by NMR (Table 1, entry 1). Switching the solvent from dioxane to EtOAc or PhMe did not give any appreciable increases in yield (entries 2 and 3). The biggest increase in yield came from switching to 2-MeTHF as the solvent. The product was isolated in 95% yield (entry 4). To confirm the unique reactivity of the CataCXium A palladacycle, these optimized conditions were tested using several additional catalysts, all yielding little to no product (entries 5–10). Decreasing the catalyst loading to 5 mol% also had a detrimental effect on the yield (entry 11). Decreasing the boronate loading to 1.5 equiv. and increasing the reaction concentration to 0.3 M gave 91% yield. We chose to move forward with these conditions since they would be most amenable to scaling up the reaction.
Table 1 Reaction optimizationa

|
Entry |
Catalyst |
Solvent (conc.) |
2a equiv. |
NMR yieldb |
|
All reactions were carried out under an atmosphere of N2 using 0.1 mmol of starting material. NMR yields were calculated on the crude reaction mixtures, using 0.05 mmol of mesitylene as an internal standard. Isolated yield. 5 mol% catalyst used. |
1 |
CataCXium A Pd G3 |
Dioxanes (0.1) |
2 |
51% |
 |
2 |
CataCXium A Pd G3 |
EtOAc (0.1) |
2 |
56% |
3 |
CataCXium A Pd G3 |
PhMe (0.1) |
2 |
56% |
4 |
CataCXium A Pd G3 |
2-MeTHF (0.1) |
2 |
95%c |
5 |
Pd(Amphos)Cl2 |
2-MeTHF (0.1) |
2 |
22% |
6 |
SPhos Pd G4 |
2-MeTHF (0.1) |
2 |
0% |
7 |
Pd(OAc)2 |
2-MeTHF (0.1) |
2 |
0% |
8 |
Pd(dbe)3 |
2-MeTHF (0.1) |
2 |
0% |
9 |
XPhos Pd G3 |
2-MeTHF (0.1) |
2 |
0% |
10 |
(PPh3)2PdCl2 |
2-MeTHF (0.1) |
2 |
46% |
11d |
CataCXium A Pd G3 |
2-MeTHF (0.1) |
2 |
42% |
12 |
CataCXium A Pd G3 |
2-MeTHF (0.1) |
1.5 |
91%c |
The final optimized conditions were tested on a gram scale on substrate 1a with the desired product obtained in 80% yield. We also applied these conditions to two analogs, one bearing an electron rich aromatic ring and the other bearing an electron deficient aromatic ring. Our optimized conditions produced both products in excellent isolated yields (Fig. 3).
 |
| Fig. 3 Scale-up and limited scope of GRM substrate1. | |
To further explore our reaction, we chose to continue with a more generic substrate (1). To accommodate a wide variety of substrates, minor modifications were made to the optimized conditions including decreasing the catalyst loading to 5 mol%, decreasing the temperature to 70 °C and decreasing the concentration to 0.1 M (Fig. 4). With these modified conditions, substrate 3d, was isolated in 91% yield on a 0.5 mmol scale. Boronates containing electronically neutral and electron-donating substituents were compatible with these conditions and isolated in synthetically useful yields. An alkyl boronate with unprotected alcohol was also amenable in the reaction giving 3g in 82% yield, however sterically hindered alkyl boronates isopropylboronic acid pinacol ester and cyclohexylboronic acid pinacol ester did not give the desired product. Arylated substrates (3h–3n) were compatible with these conditions with the products isolated in up to 97% yield. Ketone containing compound 3h reacted smoothly in 80% yield. Aryl chloride and free alcohol substrates reacted in moderate yields (3i–3j). Both electron-rich and electron-poor aryl boronates afforded the coupled products in high yield (3k–3l). The nitrated substrate 3l precipitated out of solution after completion of the reaction and simple filtration led to the isolation of pure product without the need for chromatographic purification. Substrates 3m and 3n provide differentially protected anilines to aid in downstream synthesis. Alkenyl substrates also reacted smoothly giving good yields for the coupled products 3o–3u. The reaction was tolerant of benzoxazole, furan, isoquinoline, pyrazole, carbazole, indole and pyridine motifs (3v–3ab). Ortho-bromoanilines with different substituents were also tested. Methoxy and fluorinated analogs 3ac–3af were produced in good yields. Alternative electron withdrawing groups such as nitrile, nitro and sulfone were also tolerated in the reaction (3ag–3ai). Finally, the reaction was also successful in coupling an ortho/ortho’ substituted analog giving product 3aj in 40% yield.
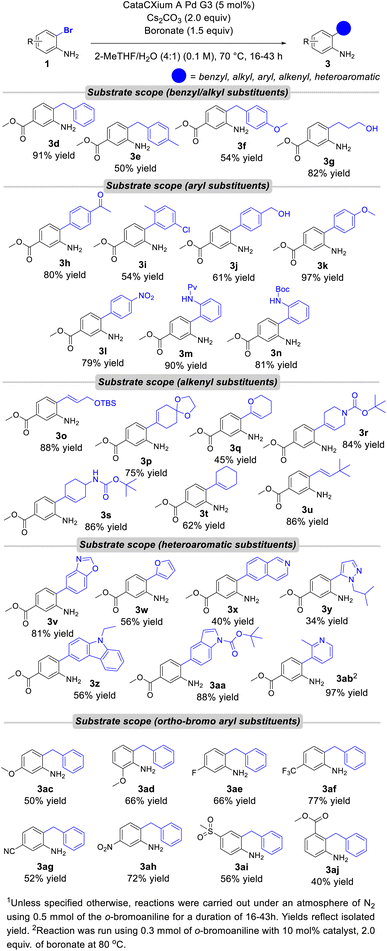 |
| Fig. 4 Substrate scope1. | |
In conclusion, we identified CataXCium A Pd G3 as a uniquely effective catalyst system for Suzuki–Miyaura cross-couplings on GRM substrate 1a. This method facilitates coupling of benzyl, alkyl, aryl, alkenyl, and heteroaromatic substituents on model substrate 1. Diverse functional group tolerability has been exemplified with support of electron-rich and electron-poor groups, halogenated aryls, protected and unprotected amines, and various heterocycles.
Data availability
The data supporting this article have been included as part of the ESI.†
Conflicts of interest
There are no conflicts to declare.
Acknowledgements
Authors CCM, AWD and ZQ are employees of AbbVie. AEL is a student in the Department of Chemistry and Biochemistry at Baylor University. The design, study conduct, and financial support for this research were provided by AbbVie. AbbVie participated in the interpretation of data, review and approval of the publication. The authors thank the following AbbVie scientists for their technical support of this project: Leena Bhatt, Rick Yarbrough and Ian Marsden. The authors also thank Chau-Wen Chou from the Proteomics and Mass Spectrometry Core Facility at the University of Georgia for high resolution mass spectrometry analysis. AbbVie paid for HRMS analysis on a per sample basis.
References
-
(a) K. T. Hylland, S. Øien-Ødegaard and M. Tilset, The Suzuki–Miyaura Cross-Coupling as the Key Step in the Syn-thesis of 2-Aminobiphenyls and 2,2’-Diaminobiphenyls: Application in the Synthesis of Schiff Base Complexes of Zn, Eur. J. Org Chem., 2020, 27, 4208–4226 CrossRef
;
(b) J. C. H. Lee and D. G. Hall In Metal-Catalyzed Cross-Coupling Reactions and More, ed. A. de Meijere, S. Bräse and M. Oestreich, Wiley, New York, 2013, pp. 65–132 Search PubMed
;
(c) N. Miyaura, T. Yanagi and A. Suzuki, The Palladium-Catalyzed Cross-Coupling of Phenylboronic Acid with Haloarenes in the Presence of Bases, Synth. Commun., 1981, 11, 513–519 CrossRef
. -
(a) A. T. K. Koshvandi, M. M. Heravi and T. Momeni, Current Applications of Suzuki–Miyaura Coupling Re-action in The Total Synthesis of Natural Products: An Update, Appl. Organomet. Chem., 2018, 32(3), e4210 CrossRef
;
(b) B. C. Barnard, Palladium-Catalysed C-C Coupling: Then and Now, Platin. Met. Rev., 2008, 52(1), 38–45 CrossRef
;
(c) A. Suzuki, Cross-Coupling Reactions via Organoboranes, J. Organomet. Chem., 2002, 653(1–2), 83–90 CrossRef
. -
(a) I. Maluenda and O. Navarro, Recent Developments in the Suzuki-Miyaura Reaction: 2010–2014, Molecules, 2015, 20, 7528–7557 CrossRef CAS PubMed
;
(b) F. Bellina, A. Carpita and R. Rossi, Palladium Catalysts for the Suzuki Cross-Coupling Reaction: An Overview of Recent Advances, Synthesis, 2004, 2419–2440 CAS
. -
(a) F. Vacondio, C. Silva, A. Lodola, A. Fioni, S. Rivara, A. Duranti, A. Tontini, S. Sanchini, J. R. Clapper, D. Piomelli, M. Mor and G. Tarzia, Structure-Property Relationships of a Class of Carba-mate-Based Fatty Acid Amide Hydrolase (FAAH) In-hibitors: Chemical and Biological Stability, ChemMedChem, 2009, 4(9), 1495–1504 CrossRef CAS PubMed
;
(b) J. Singh, M. E. Gurney, A. Burgin, V. Sandanayaka, A. Kiselyov, A. Motta, G. Schultz, G. Hategan and T. Hagen, Biaryl PDE4 Inhibitors for Treating Inflammation, US pat., US2009067600, 2009 Search PubMed
;
(c) K. Li, B. X. Wang, Y. Li and Q. Li, Amino-Aryl-Benzamide Compounds And Methods Of Use Thereof, US pat., US20210338610, 2021 Search PubMed
;
(d) D. J. Carini, J. J. V. Duncia and P. C. B. Wong, Pharmaceutical Compositions Comprising Angiotensin II Receptor Blocking Imidazoles and Diuretics, EP0733366, 1996
. -
(a) R. Emadi, A. B. Nekoo, F. Molaverdi, Z. Khorsandi, R. Sheibani and H. Sadeghi-Aliabadi, Applications of Palladium-Catalyzed C-N Cross-Coupling Reactions in Pharmaceutical Compounds, RSC Adv., 2023, 13(27), 18715–18733 RSC
;
(b) M. O. Tischler, M. B. Tóth and Z. Novák, Mild Palladium Catalyzed Ortho C–H Bond Functionalizations of Aniline Derivatives, Chem. Rec., 2017, 17, 184–199 CrossRef PubMed
;
(c) S. Scherer, A. Meudt, B. Lehnemann, A. Kalinin and V. Snieckus, Preparation of Anilineboronic Acids and Derivatives Thereof, US pat., US7196219, 2007 Search PubMed
;
(d) H. Fang, J. Yan and B. Wang, Biaryl Product Formation from Cross-coupling in Palladium catalyzed Borylation of a Boc Protected Aminobromoquinoline Compound, Molecules, 2004, 9, 178–184 CrossRef PubMed
. - For examples of cross-coupling strategies and inspiration, see:
(a) C. Pinilla, V. Salamanca, A. Lledós and A. C. Albéniz, Palladium-Catalyzed Ortho C–H Arylation of Unprotected Anilines: Chemo- and Regioselectivity Enabled by the Cooperating Ligand [2,2′-Bipyridin]-6(1H)-one, ACS Catal., 2022, 12, 14527–14532 CrossRef PubMed
;
(b) D. Yousif, S. Tombolato, E. Ould Maina, R. Po, P. Biagini, A. Papagni and L. Vaghi, Micellar Suzuki Cross-Coupling between Thiophene and Aniline in Water and under Air, Organics, 2021, 2, 415–423 CrossRef
;
(c) K. T. Hylland, S. Øien-Ødegaard and M. Tilset, The Suzuki–Miyaura Cross-Coupling as the Key Step in the Syn-thesis of 2-Aminobiphenyls and 2,2’-Diaminobiphenyls: Application in the Synthesis of Schiff Base Complexes of Zn, Eur. J. Org Chem., 2020, 27, 4208–4226 CrossRef
;
(d) Y. Chen, W. Chen, Y. Qiao and G. Zhou, B2N2-Embedded Polycylic Aromatic Hydrocarbons with Furan and Thiophene Derivatives Functionalized in Crossed Directions, Chem.–Eur. J., 2019, 25, 9326–9338 CrossRef PubMed
;
(e) O. M. Demchuk, K. Kapłon, L. Mazur, D. Strzelecka and K. M. Pietrusiewicz, Readily Available Catalysts for Demanding Suzuki-Miyaura Couplings under Mild Conditions, Tetrahedron, 2016, 72, 6668–6677 CrossRef
;
(f) P. Ruiz-Castillo and S. L. Buchwald, Applications of Palladium-Catalyzed C–N Cross-Coupling Reactions, Chem. Rev., 2016, 116, 12564–12649 CrossRef
;
(g) J. Rong, L. Deng, P. Tan, C. Ni, T. Gu and J. Hu, Radical Fluoroalkylation of Isocyanides with Fluorinated Sulfones by Visible-Light Photoredox Catalysis, Angew. Chem., Int. Ed., 2016, 55, 2743–2747 CrossRef
;
(h) Y. Chen, Y. Chen, Y. Hu, J. Qu and Y. Kang, Transalkylation via C-N Bond Cleavage of Amines Catalyzed by Super Organophotoreductant CBZ6, Org. Lett., 2023, 25(41), 7518–7522 CrossRef
;
(i) V. Pirovano, E. Brambilla, S. Rizzato, G. Abbiati, M. Bozzi and E. Rossi, Gold-Catalyzed Cascade Reactions of 4H-Furo[3,2-b]indoles with Allenamides: Synthesis of Indolin-3-one Derivatives, J. Org. Chem., 2019, 84(9), 5150–5166 CrossRef PubMed
;
(j) S. Ortgies and A. Breder, Selenium-Catalyzed Oxidative C(sp2)–H Amination of Alkenes Exemplified in the Expedient Synthesis of (Aza-)Indoles, Org. Lett., 2015, 17, 2748–2751 CrossRef
;
(k) C. L. Vandeusen, A. E. Walts and Y. S. Or, Preparation of tri-substituted thiazole compounds as eIF4 inhibitors and uses thereof, WO2021178488, 2021
. -
(a) C. C. Marvin, A. D. Hobson, M. J. McPherson, M. Hayes, J. Randolph, M. V. Patel, Z. Wang, D. Schmidt, T. Li, M. M. Fettis, A. Bischoff, J. Fitzgibbons, L. Wang, L. Wang, A. Hernandez, Jr, Y. Jia, C. Goess, S. Bryant, S. Mathieu and J. Xu, Anti-TNF Thioester Glucocorticoid Antibody-Drug Conjugate Fully Inhibits Inflammation with Minimal Effect on Systemic Corticosterone Levels In Mouse Arthritis Model, J. Med. Chem., 2024, 67, 9495–9515 CrossRef PubMed
;
(b) A. D. Hobson, J. Xu, D. S. Welch, C. C. Marvin, M. J. McPherson, B. Gates, X. Liao, M. Hollmann, M. J. Gattner, K. Dzeyk, H. Sarvaiya, V. M. Shenoy, M. M. Fettis, A. K. Bischoff, L. Wang, L. C. Santora, L. Wang, J. Fitzgibbbons, P. Salomon, A. Hernandez Jr, Y. Jia, C. A. Goess, S. L. Mathieu, S. H. Bryant, M. E. Larsen, B. Cui and Y. Tian, Discovery of ABBV-154, an anti-TNF Glucocorticoid Receptor Modulator Immunology Antibody-Drug Conjugate (iADC), J. Med. Chem., 2023, 66, 12544–12558 CrossRef PubMed
;
(c) A. D. Hobson, J. Xu, C. C. Marvin, M. J. McPherson, M. Hollmann, M. Gattner, K. Dzeyk, M. M. Fettis, A. K. Bischoff, L. Wang, J. Fitzgibbons, L. Wang, P. Salomon, Jr. , A. Hernandez, Y. Jia, H. Sarvaiya, C. A. Goess, S. L. Mathieu and L. C. Santora, Optimization of Drug-Linker to Enable Long-term Storage of Antibody-Drug Conjugate for Subcutaneous Dosing, J. Med. Chem., 2023, 66, 9161–9173 CrossRef
;
(d) A. D. Hobson, M. J. McPherson, M. E. Hayes, C. Goess, X. Li, J. Zhou, Z. Wang, Y. Yu, J. Yang, L. Sun, Q. Zhang, P. Qu, S. Yang, A. Hernandez Jr, S. H. Bryant, S. L. Mathieu, A. K. Bichoff, J. Fitzgibbons, L. C. Santora, L. Wang, L. Wang, M. M. Fettis, X. Li, C. C. Marvin, Z. Wang, M. V. Patel, D. L. Schmidt, T. Li, J. T. Randolph, R. F. Henry, C. Graff, Y. Tian, A. L. Aguirre and A. Shrestha, Discovery of ABBV-3363, an Anti-TNF Glucocorticoid Receptor Modulator Immunology Antibody Drug Conjugate, J. Med. Chem., 2022, 65, 15893–15934 CrossRef PubMed
;
(e) A. D. Hobson, M. J. McPherson, W. Waegell, C. A. Goess, R. J. Stoffel, X. Li, J. Zhou, Z. Wang, Y. Yu, A. Hernandez Jr, S. H. Bryant, S. L. Mathieu, A. K. Bischoff, J. Fitzgibbons, M. Pawlikowsha, S. Puthenveetil, L. C. Santora, L. Wang, L. Wang, C. C. Marvin, M. E. Hayes, A. Shrestha, K. A. Sarris and B. Li, Design and Development of Glucocorticoid Receptor Modulators as Immunology Antibody-Drug Conjugate Payloads, J. Med. Chem., 2022, 65, 4500–4533 CrossRef
. - Prepared according to literature procedure: A. D. Hobson, M. E. Hayes, A. Hernandez Jr, C. L. Ihle, C. C. Marvin, M. J. McPherson and W. Waegell, Glucocorticoid Receptor Agonist and Immunoconjugates Thereof, US pat., US2021161263, 2021 Search PubMed
. - N. P. Tu, A. W. Dombrowski, G. M. Goshu, A. Vasudevan, S. W. Djuric and Y. Wang, High-Throughput Reaction Screening with Nano-moles of Solid Reagents Coated on Glass Beads, Angew. Chem., Int. Ed., 2019, 58, 7987 CrossRef PubMed
. - F. Rataboul, A. Zapf, R. Jackstell, S. Harkal, T. Riermeier, A. Monsees, U. Dingerdissen and M. Beller, New Ligands for a General Palladium-Catalyzed Amination of Aryl and Heteroaryl Chlorides, Chem. Eur J., 2004, 10, 2983–2990 CrossRef PubMed
.
|
This journal is © The Royal Society of Chemistry 2024 |
Click here to see how this site uses Cookies. View our privacy policy here.