DOI:
10.1039/D4RA00367E
(Paper)
RSC Adv., 2024,
14, 9678-9690
Colour and surface functional properties of wool fabrics coated with gallnut, feijoa skin, and mango seed kernel tannin-stabilised Ag nanoparticles†
Received
14th January 2024
, Accepted 16th March 2024
First published on 22nd March 2024
Abstract
In the textile industry, textile materials are dyed and multi-functionalised by multi-step treatments that considerably increase the environmental impacts by increasing water and energy usage along with increasing the generation of volume of effluent. In this work, Ag nanoparticles (Ag NPs) were in situ formed and stabilised with gallnut, feijoa fruit skin, and mango seed kernel-derived tannins, and wool fabrics were coated simultaneously with these Ag NPs in the same bath. The Ag NP treatment produced dark to light olive-brown shades on wool fabrics. The treatment conditions for the treatment with Ag NPs were optimised to achieve the best results. The colour intensity, UV radiation absorption, antibacterial activity, surface electrical resistance, and durability of the treatment to washing were assessed by various methods. The gallnut-derived tannin (GNT)-stabilised Ag NP-coated wool fabrics showed overall the best results including excellent antibacterial activity against various types of bacteria. The treatment was durable to at least 20 cycles of IWS 7A washes (equivalent to 80 domestic washes). For the 0.5% Ag NPs on the weight of fibre (owf) dosage, the UV light transmission through the trisodium citrate-stabilised Ag NP-coated fabric at 365 and 311 nm was 6.37 and 0.95% respectively, which reduced to 1.63 and 0.20% for the fabric coated with GNT-stabilised Ag NPs providing excellent protection against UV radiation. The surface resistivity of wool fabric reduced from 1.1 × 1012 ohm cm−1 for the untreated fabric to 1.1 × 109 ohm cm−1 for the fabric coated with 2.0% owf GNT-stabilised Ag NPs. The stabilisation of Ag NPs with GNT prolonged the wash-durability by reducing the leaching of Ag NPs from the treated fabric. The developed method could be a sustainable alternative to traditional multi-stage treatments conducted in the textile industry with toxic synthetic dyes and finishing agents for the colouration and multifunctionalisation of wool fabrics.
Introduction
Antibacterial textiles are used in the healthcare sector to protect the wearer against a range of pathogens but many of them are made of non-biodegradable synthetic fibres treated with toxic antibacterial agents. Wool fabrics are popular for apparel production because of their various beneficial attributes, such as warmth, moisture absorbency, comfort, and fire retardancy.1 As wool fibre is a natural protein fibre, bacteria can grow on it which not only compromises the health and well-being of the wearer but also affects the strength of the fabric due to bacterial damage and produces a foul smell.2 Textiles can work as a vector for spreading diseases as close contact between bacterial-contaminated apparel and clean apparel provides an ideal basis for the transfer of microorganisms from the contaminated garment to the clean garment and then to human skin.3 The opposite also can happen, i.e., microorganisms from infected skin can transfer to apparel, and in close contact that microorganism can transfer to a non-infected person. To make textiles antibacterial, they are either treated with a leaching-type antibacterial agent (antimicrobial agents are released from fabric killing the bacteria), or a non-leaching antibacterial agent (bugs are killed when they encounter the antimicrobial-treated fabrics).
Until recently, quaternary ammonium compounds (QAC) were the popular antibacterial agents for textiles.4 The QACs are absorbed into the bacterial cell wall membrane causing the leakage of low-molecular weight components out of the cell resulting in the death of bacteria.5 However, they are now under scrutiny because of their bioaccumulation causing potential biological risks as they show toxicity towards soil organisms, negatively affecting the ecosystem.6,7 Moreover, QACs are difficult to bind to wool fibre surfaces as untreated wool fibre surfaces have no functional groups to bind them causing their release from treated fibres during laundering and a gradual loss of antibacterial activity. Other than quaternary ammonium compounds, polyhexamethylene biguanide hydrochloride,8 dimethylol dimethyl hydantoin,9 5-chloro-2-(2,4-dichlorophen-oxy)phenol or triclosan,10 para-amino benzene sulphonic acid,11 and N-halamines,12 have been studied to make textiles antibacterial. Some of them are not eco-friendly and some are even known to develop drug-resistant bacteria, posing a threat to human life.13 To replace toxic antibacterial agents, essential oils, and tannins extracted from various biomasses,14,15 and unmodified/modified chitosan.16–18 have been studied with limited success. Various bioderived antibacterial agents, such as eugenol,19 thymol,20 lysozyme,21 and capsaicin,22 have been studied to make textiles antibacterial with some level of success. Metallic nanoparticles of silver (Ag),23,24 gold,25 copper,26 and zinc oxide,27 have also been studied to make wool textiles multifunctional including antibacterial. Instead of a toxic reducing agent, different types of tannins derived from pomegranate leaves,28 elderberry fruits,29 neem leaves,30 black rice,31 and Mentha spicata L.,32 were studied as a reducing agent. The advantage of silver nanoparticles (Ag NPs) is that they also can produce a variety of shades on treated fabrics depending on their shape and size.
To make wool fabrics coloured, antibacterial, antistatic, and ultraviolet radiation (UV) protective, they are dyed and treated with various toxic dyes, antibacterial agents, UV absorbers, and quaternary ammonium compounds by multi-step treatments considerably increasing water and energy usage along with producing effluent containing these leftover dyes and chemicals.33–35 The traditional colouration of wool fabrics with acid and reactive dyes produces effluent that needs treatment as most of these dyes are recalcitrant and toxic.36 The colouration of textiles with gold and Ag NPs has been widely studied and they provide some levels of colourfastness to washing and light.37,38 Functionalisation of various textile fibres by in situ formed Ag NPs using trisodium citrate (TSC),39,40 peanut red skin extract,41 and Punica granatum peel extract,42 has already been reported. The published articles mainly reported the antibacterial and UV protection properties of textiles treated with Ag NPs but ignored the colouration aspects and other functional properties. Most of the treatments reported in these articles were carried out in a glass beaker without proper control of processing parameters.
In this article, simultaneous formation, and stabilisation of Ag NPs with tannins extracted from gallnut, mango seed kernel, and feijoa fruit skin, and colouration of wool fabrics with them were carried out in a single bath at a controlled and moderate temperature to reduce energy and water usage and to further improve the functional properties of wool fabric. To the best of our knowledge, no published article reported the colouration and multifunctionalisation of wool fabrics with Ag NPs reduced and stabilised with tannins extracted from gallnut, mango seed kernel, and feijoa fruit skins.
Experimental
Materials
The chlorine/Hercosett shrink-resist treated wool fabric of 140 g m−2 made from merino wool with an average diameter of 19 µm having 64 ends per cm and 62 picks per cm was procured locally. The feijoa fruit skin tannin (FFST) and mango seed kernel tannin (MSKT) were extracted from feijoa fruit skins and mango seed kernel waste according to the method described in our previously published article.14 The gallnut tannin (GNT), silver nitrate (AgNO3), TSC, acetic acid, and various antibiotics (ceftazidime, piperacillin, colistin, gentamicin, and amikacin) used for the control antibacterial treatment were purchased from Sigma-Aldrich Chemicals (USA). Sandozin MRN (a wetting agent) and Sandoclean PC (a non-ionic detergent) were supplied by Arkema Chemicals (Switzerland). The commercial wool fabric washing detergent (Softly®) used for the durability to washing assessment was supplied by Pental Products Limited (Australia). The bacterial cultures of Gram(−) Pseudomonas aeruginosa (NZRM 4034), and Gram(+) Staphylococcus aureus (NZRM 917) were procured from a New Zealand-based research institute (Environmental Science Research). The bacterial culture of Gram(−) Escherichia coli O157:H7, a wild-type strain was isolated from calf faeces collected from one of the local beef farms.
Synthesis of Ag NPs and colouration of wool fabrics
The one-pot synthesis of Ag NPs and treatment of wool fabric with them were carried out at a materials-to-liquor ratio of 1
:
30 using an Ahiba laboratory dyeing machine (Model: Ahiba Turbomat 1000, Datacolor International, Switzerland) using deionised water. The wool fabric used in this work was scoured with 1 g per L Sandoclean PC and 0.25 g per L Sandozin MRN at 50 °C for 30 min to remove dirt and spinning oil. The treatment bath was prepared with the required quantity of pre-dissolved AgNO3 solution and 0.25 g per L Sandozin MRN was added to it. A 20.0 g wool fabric sample was introduced into the bath and the pH was set at 3.0, 4.0, and 5.0 with acetic acid/sodium acetate buffer, and 7.0 and 9.0 with sodium carbonate. The temperature of the bath was then raised to 50 °C at 2.0 °C min−1, and the required quantity of pre-dissolved TSC or tannins was added to it as a reducing agent as well as a stabilizer for the formed Ag NPs. After 2 h, the bath was cooled, the liquor drained, and the treated fabric samples were collected. They were then washed with Sandoclean PC at 45 °C for 10 min followed by washing again with cold water and then dried. The concentration of Ag, tannins, and Ag to tannin ratio was varied to optimise the processing conditions to achieve the best results. The wool fabric coated with Ag NPs using an Ag to TSC ratio of 1
:
2 at 90 °C for 2 h was used as the control treatment.
Characterisation of Ag NP-coated fabrics
The measurements of colour strength of wool fabrics treated with Ag NPs were carried out by a published method.43 The reflectance values at the wavelength of maximum absorption of the treated samples were measured using a Datacolor spectrophotometer (Model: DC 550, Datacolor International, Switzerland), and the measurements were carried out under illuminant D65 using a 10° standard observer with UV component excluded and specular included. The colour fastness to washing of the Ag NP-coated fabrics was measured according to ISO Test Method 105-C03 1987: textiles – tests for colourfastness – Part C03: colourfastness to washing: test 3 by washing in a Gyro wash (Model 415/8) using a phosphate-free standard detergent. The colourfastness to washing grades was assessed comparing with 3 M greyscale.
The percent transmission of UV light through the untreated and Ag NP-coated wool fabrics was measured in the diffused reflectance mode by a UV-vis Spectrophotometer (Model: Evolution 220, ThermoFisher Scientific Inc., Waltham, USA) fitted with an integrating sphere accessory (ISA-220). The UV transmittance (%) was measured at a wavelength interval of 1 nm in the 290–380 nm spectral span. The antistatic properties of the treated fabrics were measured by measuring their surface resistivity. Before measuring, the fabric samples were preconditioned at 20 ± 2 °C and 45 ± 2% relative humidity for 48 h and then surface resistivity was measured by a surface/volume resistance meter (Model 152-1, Trek, Inc., Lockport, USA) equipped with a concentric ring probe at 5 positions with an applied voltage of 100 V.
The surface morphology of the wool fabrics coated with Ag NPs was investigated by using a scanning electron microscope (SEM) in the backscattered electron imaging mode. The fabric surfaces were scanned using a Hitachi SEM (Model: TM 3030 Plus, Hitachi Corporation, Tokyo, Japan) at an accelerated voltage of 15 kV without any conductive coating. The elemental analysis of S and Ag was carried out by an energy-dispersive X-ray (EDX) using the same Hitachi SEM equipped with Bruker's Quantax75 EDX attachment. For measuring the size of produced Ag NPs by transmission electron microscopy (TEM), Ag NPs were synthesised by the method used for colouration but without the addition of wool fabric samples. The Ag NP dispersion was concentrated by evaporating water and then a small amount of Ag NP dispersion was sprayed on a Formvar 200 mesh TEM copper grid, which was dried in a dust-free environment for 2 h. The Ag NP-coated copper grid was then scanned by using a Philips high-resolution TEM (Model: CM-200 FEG, Royal Philips Electronics, Holland) and micrographs were captured by using a Gatan digital camera. The acquired TEM images were used to measure the average particle size and distribution of particle size of Ag NPs synthesised using GNT and MSKT using ImageJ software (https://imagej.nih.gov/ij/download.html).
Assessment of antibacterial activity
The antibacterial activity of wool fabric samples, untreated and coated with Ag NPs stabilised with TSC and various tannins was determined against Escherichia coli, Pseudomonas aeruginosa, and Staphylococcus aureus using the agar disk diffusion method, with few modifications. The turbidity of the bacterial suspension was adjusted using sterile saline (0.83% NaCl) to match 0.5 McFarland standard, equivalent to an optical density between 0.08 and 0.1. Mueller Hinton agar (MHA) plates were inoculated with the suspension of each of the tested bacteria and the plates were left on a bench at room temperature for 5 min. Wool samples were cut into circular discs and UV-irradiated for 2 min to sterilise the samples. Each wool sample was placed in the centre of the inoculated MHA plates and wet with 30 µL sterile water so that the material sticks to the media and does not curl up. Appropriate controls such as wool sample without any antimicrobial agents, taken as a negative control and discs of five antibiotics viz.: ceftazidime (30 µg), piperacillin (100 µg), colistin (10 µg), gentamicin (10 µg) and amikacin (30 µg) were used in the experiment. The plates were incubated at 37 °C for 24 h and the zone of inhibition (the area around the samples or discs where the bacteria were unable to grow) was recorded. The zone of inhibition was calculated by subtracting the fabric sample diameter from the diameter of the bacterial inhibition zone circle. All the experiments were repeated thrice and the averages of the zones of inhibition were calculated to interpret the results.
Durability of the treatment to washing
The durability of the treatment of the wool fabric coated with in situ formed Ag NPs by various tannins was measured according to the International Wool Secretariate (IWS) 7A washing protocol by washing in a Wascator washing machine at 40 °C using a commercial wool washing detergent (Softly®). Each cycle of such washing is equivalent to four domestic washes. The fabric samples were washed for 5, 10, and 20 cycles wet-on-wet basis without in-between drying. The durability of the wool fabric coated with Ag NPs stabilised with various tannins to washing was assessed by comparing the colour strength and antibacterial activity of fabric samples after 5, 10, and 20 cycles with the colour strength and antibacterial performance of the unwashed fabric.
Statistical analysis
The statistical analyses were completed using Minitab software. The averages of the parameters of each batch were calculated and compared using the Student's t-test for independent samples with a percentage confidence interval equal to 95%.
Results and discussion
Colour intensity and shades produced
The fabric coated with Ag NPs using TSC produced a brown colour, but Ag NPs stabilised with tannins produced dark to light olive colour shades with tonal changes, that are quite different than the colours produced by Ag NPs or polyphenols extracted from mango seed kernel and feijoa fruit skin.14,23 Fig. 1 shows the colour strength (K/S) of wool fabric treated with 0.5 and 1.5% owf Ag using various tannins and TSC at pH 4. The wool fabrics treated with 0.5% Ag NPs using various tannins and TSC hardly produced any colour, and the produced colour strength was poor. Of the tannins and TSC studied, the GNT produced the highest colour strength and MSKT provided the lowest colour strength. All tannins studied showed the highest colour strength at 420 nm. The second-highest colour strength was provided by FFST. TSC showed slightly lower colour strength compared to the colour strength provided by FFST but considerably better compared to the colour strength provided by MKST (only 3.44). However, the increase in applied dosage of Ag to 1.5% owf highly increased the colour strength of the treated fabrics. For 1.5% Ag NP dosage, GNT produced the highest colour strength and TSC produced the lowest colour strength. The colour strength achieved with GNT and FFST was quite high, 11.19 and 8.34 respectively, which is considerably higher compared to the colour strength produced by TSC-stabilised Ag NPs or GNT and FFST alone without Ag NPs at the same concentrations and conditions reported in our previously published articles,14,23 The results achieved suggest that MSKT has a very poor reducing potential and GNT has a strong reducing power. Therefore, the wool fabric coated with GNT-stabilised Ag NPs produced the deepest colour. Therefore, further optimisation of treatment conditions was carried out using GNT only.
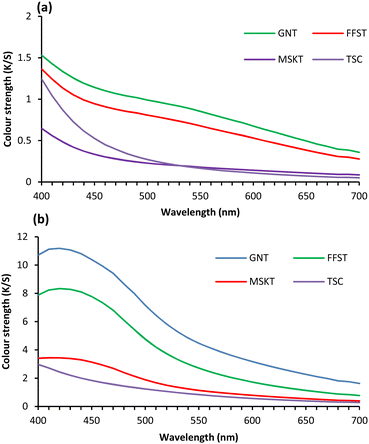 |
| Fig. 1 Wool fabric treated with 0.5 (a) and 1.5% (b) owf Ag using three types of tannins at 50 °C for 2 h at pH 4. | |
Fig. 2(a) shows the effect of treatment pH on the colour strength of the wool fabrics coated with GNT-stabilised Ag NPs and the wavelength of the maximum absorption for the fabrics treated at various pHs was 410 nm. The fabric treated at pH 3 showed the lowest colour strength (2.05) but the fabric treated at pH 4 showed the highest colour strength (12.14) which is consistent with the acrylic fabric coated with TSC-stabilised Ag NPs reported in our previously published article.40 The colour strength produced by tannin-stabilised Ag NPs was quite good and comparable to the shades produced by synthetic dyes at that application level. The colour strength gradually decreased with a further increase in the treatment pH. Therefore, pH 4 is the optimum pH for the colouration of wool fabric with Ag NPs stabilised with tannins.
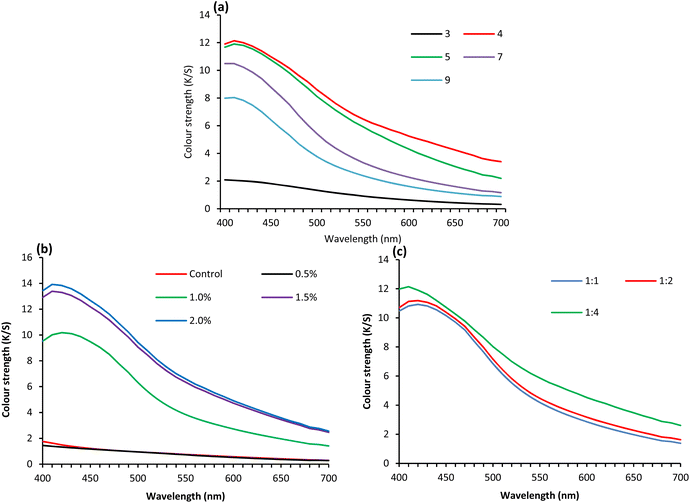 |
| Fig. 2 Colour strength wool fabric dyed with 1 (a), 3 (b), and 5% (c) lignin sulphonate using various metals at 25% owl. | |
The isoelectric point of the wool fibre surface in an aqueous solution is at pH 4.5, i.e., below, and above pH 4.5, the wool fibre surface is cationic and anionic respectively.43 The cationic charge intensity of the wool fibre surface increases with a decrease in pH. The optimum pH for the conversion of AgNO3 to Ag NP is also pH 4.40 The stabilisation of Ag NPs with GNT made them anionic. Therefore, an increase in pH above 4.5 decreases the absorption of Ag NPs into wool and consequently, a decrease in pH from 4.5 increases the absorption of Ag NPs into wool. However, a decrease in pH from 4 decreases the conversion to Ag NPs, and wool fibre pores are blocked by Ag NPs due to increased affinity towards wool fibre surface at that pH as many GNT stabilised Ag NPs tried to enter wool fibre at the same time. Therefore, at pH below 4, the colour intensity of the treated fabric considerably decreased.
Fig. 2(b) shows the effect of Ag dosage on the colour strength of the fabric treated with Ag NPs stabilised with GNT using an Ag to TSC ratio of 1
:
2. The control fabric coated with 0.5% owf Ag and stabilised with TSC showed no absorption peak like the wool fabric coated with 0.5% owf Ag using Ag to GNT ratio of 1
:
3 at 50 °C for 2 h and the colour strength produced was very poor (1.38). However, the fabric coated with TSC-stabilised Ag NPs showed slightly better colour strength (1.45) than the fabric coated with Ag NPs using GNT as a stabilizer, suggesting that the colour introduced in the fabric was mainly produced by Ag NPs, not by the tannins. The fabric coated with 1.0% owf Ag showed the highest colour strength (10.18) at 420 nm but with an increase in Ag concentration, the colour strength also increased but the wavelength of maximum absorption moved towards a lower wavelength due to the decreased particle size. The increase in Ag dosage to 1.5% owf increased the colour strength to 13.4 at 410 nm. However further increase in ag dosage to 2% owf only marginally increased the colour strength of the fabric to 13.9, and therefore 1.5% owf is the optimum Ag dosage.
Fig. 2(c) shows the effect of the Ag to GNT ratio on the colour strength of wool fabric coated with Ag NPs. The colour strength increased with an increase in the ratio of Ag to GNT and the maximum colour strength was observed for the Ag to GNT ratio of 1
:
4 which increased the colour strength to 12.1 from 10.9 exhibited by the fabric coated with Ag NPs using Ag to GNT ratio of 1
:
1. For the 1
:
1 and 1
:
2 ratios, the maximum colour strength was observed at 420 nm but for 1
:
4 Ag to GNT ratio, the maximum colour strength was shifted to 410 nm, probably due to decrease in Ag NP size. Overall, the effect of the Ag to tannin ratio had only a marginal effect as an increase in colour strength achieved by increasing the Ag to tannin ratio was relatively small.
Protection from UV radiation
Solar UV radiation is very harmful to human skin and is one of the main causes of skin cancer. Apparel that does not allow UV radiation transmission through the fabric is therefore highly beneficial to keep humans protected from the harmful effect of solar UV radiation and apparel made from such a kind of treated fabric is very good for outdoor activities. The untreated wool fabric has very poor UV protection capability as it has high UV light transmission through the fabric. Sobolewski et al. measured the UV protection of different fabrics using a standard photo-therapy wall with TL-01 UV phototherapeutic tube lamps as an irradiation source together with the dressed mannequin to be irradiated and the UV intensity was measured by a spectrophotometer.44 They found that the UV intensities were the highest at 311 (UV-B) and 365 (UV-A) nm. Therefore, in this study, we measured UV transmission through various fabric samples at 311 and 365 nm wavelengths to measure their UV protection capability.
The UV transmittance less than 2.5%, 2.6–4.1 and 4.2 to 6.7% are considered ‘excellent’, ‘very good’ and ‘good’ respectively.45 For wool fabric samples coated with 0.5 and 1.5% owf Ag NPs using TSC and various tannins, for all of them the UV transmission through the fabrics considerably decreased as shown in Fig. 3. The UV protection exhibited by the fabrics showed similar trends and TSC-stabilised Ag NP-coated fabric showed the poorest UV protection as the UV transmission was the highest. The UV transmission through the fabric at 290 and 360 nm was 1.50 and 5.6% as shown in Fig. 3(a) but still showed good UV protection capability. Wool fabrics coated with Ag NPs stabilised with various tannins exhibited excellent UV protection capability. Of the tannins studied, GNT and FFST showed the best protection against UV radiation at a lower dosage of Ag NPs as the fabric coated with GNT and FFST showed the lowest UV light transmission at all wavelengths. The increase in Ag dosage to 1.5% considerably increased the UV protection capability of wool fabrics as shown in Fig. 3(b). Overall, GNT provided the best performance as the treated fabric had UV transmission through the fabric at 360 and 290 nm only 0.21 and 0.13% respectively. Tannins have many polyphenolic groups capable of absorbing UV radiation,46 and therefore their application as a stabilizing agent for the stabilisation of Ag NPs considerably improved the coated fabrics' UV protection capability.
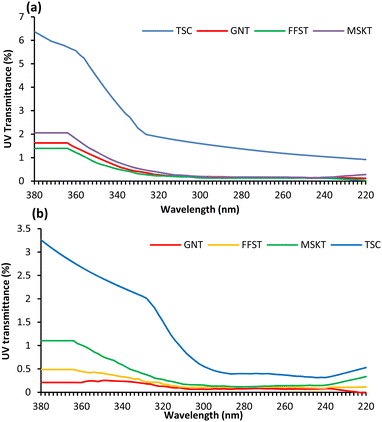 |
| Fig. 3 UV transmittance of wool fabric coated with 0.5 (a) and 1.5% (b) owf Ag using three types of tannins at 50 °C for 2 h at pH 4. | |
Fig. 4(a) shows the effect of treatment pH on UV transmission through the fabric treated with GNT-stabilised Ag NPs. Wool fabrics coated with Ag NPs at all pHs showed excellent UV protection but of them, the fabric treated at pH 3.0 showed the highest UV transmission as the UV transmission at 311 and 365 nm are 0.12 and 1.04% respectively, which showed the worst protection but still provided excellent protection against the UV radiation. The fabric treated at pH 4.0 showed the best UV protection as UV transmission at 311 and 365 nm was only 0.04 and 0.09% respectively. Therefore, the optimum pH to achieve the best UV protection was pH 4.0.
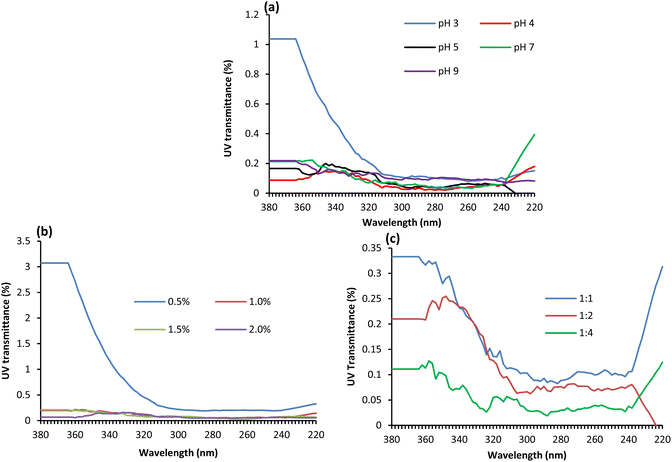 |
| Fig. 4 Effect of pH (a), Ag concentration (b), and Ag to GNT ratio (c) on the UV transmittance of the treated wool fabric. | |
Fig. 4(b) shows the effect of applied dosages of Ag NPs on UV transmission through wool fabric samples. The wool fabric treated with 0.5% Ag NPs showed poor UV absorption capability as the UV light transmission through the fabric at 311 and 365 nm was 0.28 and 3.07% respectively, which is still quite good. The UV transmission through the fabric decreased with an increase in the Ag NP dosage. For the fabric treated with 2.0% owf Ag NPs, the UV transmission through the fabric at 311 and 365 nm reduced to 0.05 and 0.07% respectively. On the other hand, Fig. 4(c) shows the effect of the Ag to GNT ratio on the UV transmission of the fabric treated with 0.5% owf Ag at pH 4. The UV transmission through the fabric decreased with an increase in the Ag to GNT ratio.
Antibacterial properties
Table 1 shows the antibacterial performance of wool fabrics coated with Ag NPs stabilised with GNT, FFST, and MSKT after 0, 10, and 20 washes. The wool fabric samples coated with Ag NPs stabilised by TSC, GNT, FFST, and MSKT all showed excellent antibacterial performance against all the types of bacteria studied in this work, but against Pseudomonas aeruginosa, the antibacterial performance was much better compared to the other two types of bacteria replicated by the larger zone of inhibition (9.45 mm). The antibacterial performance against Escherichia coli was inferior to the antibacterial performance against the other two types of bacteria and still, no bacterial growth was observed above or underneath the tested samples for any type of bacteria. After 10 washes, the wool fabric coated with Ag NPs stabilised with all types of stabilizers studied in this work slightly lost their antibacterial performance due to the loss of the stabilizing agent (tannins) and or Ag NPs which is replicated by the small loss in the size of the zone of inhibition. However, after 20 washes the wool fabric coated with TSC-stabilised Ag NPs mostly lost its antibacterial performance, especially against Escherichia coli and some levels against Staphylococcus aureus. Some bacterial growth was observed underneath the fabric samples. However, wool fabrics coated with Ag NPs stabilised with GNT, FFST, and MSKT still retained their high antibacterial activity against all types of bacteria studied in this work. The wool fabric sample coated with MSKT-stabilised Ag NPs showed slightly better antibacterial performance compared to the Ag NPs stabilised by the other two tannins. The results achieved suggest that the application of tannin either enhanced or prevented the loss of Ag NPs during washing resulting in strong antibacterial performance retained even after 20 washes.
Table 1 Antimicrobial performance of wool fabric coated with 0.5% Ag NPs at 50 °C for 2 h using Ag to tannin/TSC ratio of 1
:
2 after 0, 10, and 20 washes
Treatment ID |
Zone of inhibition (mm) |
Escherichia coli |
Pseudomonas aeruginosa |
Staphylococcus aureus |
Control |
0 ± 0 |
0 ± 0 |
0 ± 0 |
![[thin space (1/6-em)]](https://www.rsc.org/images/entities/char_2009.gif) |
Before washing |
TSC |
2.9 ± 0.4 |
9.5 ± 0.5 |
6.7 ± 0.3 |
GNT |
4.2 ± 0.5 |
8.9 ± 0.7 |
7.2 ± 0.3 |
FFST |
1.6 ± 0.4 |
10.2 ± 0.5 |
4.9 ± 1.1 |
MSKT |
1.8 ± 0.4 |
9.5 ± 0.7 |
5.8 ± 1.1 |
![[thin space (1/6-em)]](https://www.rsc.org/images/entities/char_2009.gif) |
After 10 washes |
TSC |
2.5 ± 0.2 |
7.3 ± 0.3 |
4.5 ± 0.2 |
GNT |
3.2 ± 0.7 |
7.8 ± 0.5 |
5.2 ± 0.1 |
FFST |
1.0 ± 0.3 |
8.3 ± 0.4 |
3.8 ± 0.7 |
MSKT |
1.4 ± 0.3 |
7.7 ± 0.8 |
4.8 ± 1.0 |
![[thin space (1/6-em)]](https://www.rsc.org/images/entities/char_2009.gif) |
After 20 washes |
TSC |
0 |
0 |
0 |
GNT |
1.1 ± 0.1 |
6.3 ± 0.7 |
4.4 ± 0.2 |
FFST |
0.9 ± 0.3 |
6.1 ± 0.2 |
4.1 ± 0.6 |
MSKT |
1.0 ± 0.0 |
6.2 ± 0.5 |
3.2 ± 0.2 |
Ag NPs and natural tannins are known to have good antibacterial properties against a wide range of bacteria.14,23 Therefore, it can be expected that the inclusion of tannins for the stabilisation of Ag NPs should enhance their antibacterial performance. The antibacterial mechanisms of Ag NPs and tannin-stabilised Ag NPs are quite different. Ag NPs adhere to the surface of the cell wall and penetrate bacterial cells disrupting intracellular structures (mitochondria, ribosomes) and biomolecules (protein, lipids, and DNA) causing the death of bacteria.47 Ag NPs also release Ag ions, which also can be responsible for bacterial death. Ag ions work in various modes, such as they can control the production of adenosine triphosphate (ATP) by attaching to the ATP synthesis enzyme in the cell wall can penetrate the cell wall and attach to DNA causing the DNA alteration or they can resist the respiratory chain of enzymes.48,49 Natural polyphenols act as an inhibitor of the NorA efflux pump, which is considered the main mechanism responsible for their antibacterial activity.50 Here, tannin-functionalised Ag NPs showed a synergistic effect, combining the antimicrobial potential of Ag NPs, Ag ions and tannins. Therefore, wool fabrics coated with various tannin-stabilised Ag NPs showed better antibacterial activity and durability of antibacterial activity to washing compared to the wool fabrics coated with TSC-stabilised NPs or polyphenols extracted from mango seed kernel and feijoa fruit skins alone.
Antistatic properties
The antistatic property of textiles is related to the moisture content of the fibres and at the relative humidity conditions the antistatic property is measured. The higher the moisture content or relative humidity, the lower the static charges build up in the textiles. In this work, we measured the static electricity of the treated fabric at 45% relative humidity. Wool fabric has some level of antistatic properties because of its high moisture content but that is not enough for various applications, such as use in service stations, as static charge built up on wool-made apparel can cause fire, and therefore it needs antistatic treatment. The antistatic properties of a fabric can be measured by measuring its surface resistivity. The lower the surface resistivity the higher the surface electrical conductivity and the better the antistatic property. Fig. 5 shows the surface resistivity of untreated wool fabric and wool fabrics coated with various dosages of GNT-stabilised Ag NPs. The untreated wool fabric showed very high surface resistivity, 1.1 × 1012 ohm cm−1, which suggests its poor antistatic properties but is still lower compared to fabrics made of non-shrink-resist-treated wool fibre (1.3 × 1012).23 The removal of hydrophobic 18-methyl eicosanoic acid bonded to wool through thioester linkage and coating with Hercosett resin (synthetic polyamide resin) made wool fibre surface hydrophilic and increased its moisture content increasing the antistatic property. However, for the fabric coated with GNT-stabilised Ag NPs, the surface resistivity of wool fabrics considerably decreased increasing its antistatic properties. The surface resistivity of wool fabric decreased to 1.1 × 109 ohm cm−1 for the wool fabric coated with 2% owf Ag NPs, which was better compared to the wool fabric coated with TSC-stabilised Ag NPs and wool fabric coated with these tannins alone.14,23 The wool fabric used in this work was shrink-resist treated wool fabric, which is hydrophilic compared to the hydrophobic untreated wool fabric used in previous works.14,23 The results suggest that the modification of Ag NPs with GNT enhanced the surface electrical conductivity of the treated fabrics enhancing the antistatic property of the treated wool fabric.
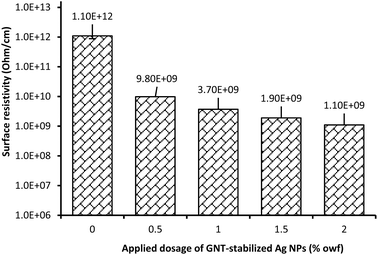 |
| Fig. 5 Antistatic properties of wool fabric treated with various concentrations of Ag NPs stabilised with GNT. | |
Durability of the treatment to washing
Fig. 6(a) shows the effect of an increase in the number of cycles of IWS 7A washing on the colour strength of the fabric treated with 0.5% owf Ag NPs stabilised with various tannins, and colour difference (ΔE) compared to the untreated control fabric. Washing had more effect on the colour strength of wool fabrics treated with FFST and MSKT-stabilised Ag NPs compared to the wool fabrics coated with GNT-stabilised Ag NPs. All samples had the most decrease in colour strength in the first 5 washes and less reduction in colour strength was observed for the next 5 washes. For the wool fabric coated with GNT-stabilised Ag NPs, the colour strength dropped from 1.53 to 1.42 after 5 washing compared to the decrease in colour strength of 1.37 to 1.18 and 0.65 to 0.47 for wool fabric samples coated with FFST and MSKT-stabilised Ag NPs. After 20 washing cycles, the colour strength was decreased to 1.36, 1.05, and 0.35 for the wool fabric coated with GNT, FFST, and MSKT-stabilised Ag NPs respectively.
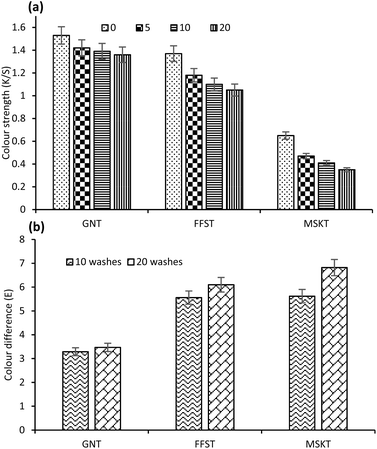 |
| Fig. 6 Effect of cycles of washes on the colour strength (a) and colour difference (b) of wool fabric treated with 0.5% owf Ag NPs stabilised with various tannins. | |
Fig. 6(b) shows the effect of washing on the colour difference of wool fabrics coated with Ag NPs stabilised with various tannins. The optical images of 0, 10, and 20 cycles of washed fabrics are presented in Fig. S1 (ESI).† The lowest and the highest colour difference was observed for the wool fabric sample coated with GNT and MSKT-stabilised Ag NPs respectively. The highest increase in colour difference was observed for the 10-cycle washed fabric samples, which is consistent with the decrease in colour strength of the fabric observed after washing. The colour difference only slightly increased in the subsequent washing cycles. The results of a decrease in colour strength and an increase in colour difference after washing suggest that MSKT had the highest molecular weight. Therefore, less was absorbed into the wool fibre (mostly remained on the fibre surface) and was lost during various cycles of washing.
Elemental analysis
Fig. 7 shows the EDX spectra and elemental analysis results of wool fabrics treated with Ag NPs stabilised by TSC, GNT, FFST, and MSKT. The measurement of Ag content is important as its content in the treated fabrics determines its antibacterial efficacy, UV protection capability, antistatic properties, and durability of the treatment to washing. The S and Ag contents of wool fabric coated with TSC-stabilised Ag NPs were 6.02 and 1.41% respectively. The wool fabric coated with GNT-stabilised Ag NPs showed the highest Ag content (2.17%) but of the tannin-stabilized Ag NPs, MSKT-stabilised Ag NPs showed the lowest Ag content. The fabric coated with the TSC-stabilised Ag NPs also had low Ag content. The Ag content of wool fabric coated with FFST-stabilised Ag NPs was in between the Ag contents of the fabric coated with Ag NPs stabilised with the other two tannins. Therefore, the wool fabric coated with GNT-stabilised Ag NPs showed the highest Ag content and therefore exhibited the best results in terms of colour, UV protection, antistatic and antibacterial properties, and the durability of the treatment to washing.
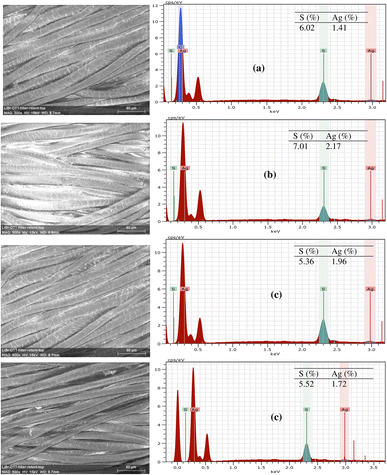 |
| Fig. 7 EDX spectra of wool fabrics coated with 1.5% owf Ag using TSC (a), GNT (b), FFST (c), and MSKT (d) as a reducing and stabilizing agent. | |
Fig. S2 (ESI)† shows the elemental distribution of S and Ag on wool fabrics coated with Ag nanoparticles stabilised with various tannins. For all tannins, the S and Ag elements are uniformly distributed on the surface of wool fibres suggesting very uniform treatment of wool fabrics coated with Ag NPs stabilised with various tannins.
Surface morphologies
Fig. 8 shows the SEM micrographs of wool fibres covered with 1.5% Ag NPs stabilised with TSC, GNT, FFST, and MSKT. In the case of wool fabric coated with TSC-stabilised Ag NPs, deposition of nanoparticles on some fibres is visible but few nanoparticles are visible on other wool fibres suggesting Ag NPs formed an ununiform coating on the wool fibre surface. In the case of FFST, a smaller number of Ag NPs are visible on the wool fibre surface compared to GNT and MSKT, but they are quite uniformly distributed on the wool fibre surface. The highest number of Ag NPs are visible for the GNT and MSKT, which shows a more uniformly distributed Ag NPs, as well as a greater number of Ag NPs on the wool fibre surface compared to FFST and TSC, studied in this work.
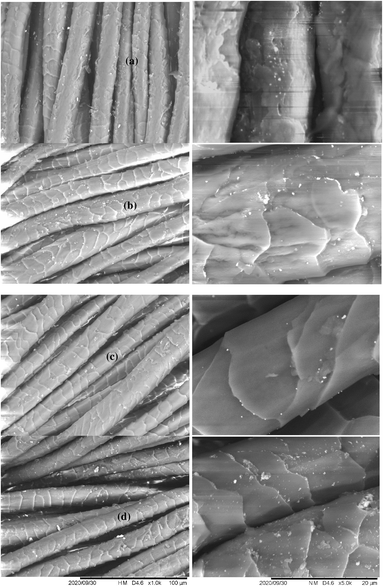 |
| Fig. 8 Wool fabric treated with 1.5% Ag NPs stabilized by TSC (a), GNT (b), FFST (c), and MSKT (d) using an Ag to tannin ratio of 1 : 2. | |
Fig. S3 (ESI)† shows SEM micrographs of wool fabric coated with various concentrations of Ag NPs stabilised with GNT at an Ag to GNT ratio of 1
:
2. The micrographs show typical wool fibre surfaces with scales but show no evidence of fibre damage or cracks suggesting the treatment with Ag NPs did not cause any damage to wool fibres. The presence of Ag NPs on wool fibre surfaces is visible for all the concentrations of Ag NPs studied in this work. The coverage of the wool fibre surface with Ag NPs increased with an increase in the concentration of Ag NPs as few NPs are visible on the wool fibre surface in the case of wool fabric coated with 0.5% owf Ag. For 1.5% owf Ag, the surface of wool fibres is quite uniformly covered but for 2% owf Ag NPs, the scale edges are more densely covered by Ag NPs than the other parts of the surface of fibres.
Fig. 9 shows the TEM image of Ag NPs formed by GNT and MSKT and their particle size distribution. GNT produced much smaller Ag NPs compared to the MSKT and also showed hardly any agglomeration, but MSKT produced Ag NPs that formed agglomeration. In the case of GNT, the size of Ag NPs ranged from 6.4 nm to 22.3 nm with an average particle size of 11.5 nm. On the other hand, MSKT produced Ag NPs ranging from 13 nm to 44 nm with an average particle size of 26.7 nm. The reason GNT produced smaller size Ag NPs compared to MSKT is unknown but probably the molecular weight of MSKT was considerably higher compared to GNT. Díaz-Cruz et al. observed that for the Ag NPs formed by poly(ethylene glycol) as a reducing and stabilizing agent, the size of Ag NPs increased with an increase in the molecular weight of the polymer.51
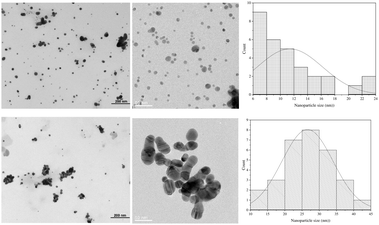 |
| Fig. 9 TEM images of Ag NPs stabilized with GNT (top) and MSKT (bottom), and their particle size distribution. | |
ATR-FTIR spectral analysis
The ATR-FTIR spectral analysis was carried out to determine the interactions between wool fibre and tannin-stabilised Ag NPs. Fig. 10 shows the ATR-FTIR spectra of the control untreated wool fabric and also wool fabric coated with 1.5% owf Ag NPs stabilised with GNT, FFST and MSKT. The spectrum of control wool fabric shows typical wool fibre keratin-related IR bands at 1036, 1261, 1388, 1509, 1625, 2866, 2963, and 3269 cm−1 associated with sulphonic acid moiety (formed due to the breakage of disulphide linkage by chlorine oxidation), amide (III), amide (II), amide (I), symmetric CH stretching vibrations, asymmetric CH stretching vibrations. And hydroxyl groups, respectively.52,53 Conversely, the spectra of wool fabrics coated with Ag NPs stabilised with all three types of tannins also show the same IR bands, but their position moved to 1021, 1392, 1508, 1640, and 3260 cm−1. The tannins have distinctive IR bands at 700–800, 1600–1700, and 3200–3600 cm−1,54 but they are overlapped with the amide (I) and hydroxyl groups of wool keratin protein. However, the intensity of the IR band between 700 to 800 regions increased for the wool fabric treated with Ag NPs with various tannins suggesting the presence of tannins in the fabric. The decrease in the intensity and change in the position of the various amide and hydroxyl bands compared to the untreated control fabric suggest the formation of ionic bonds between anionic hydroxyl groups of tannins and cationic amino groups of wool and also anionic groups of tannin with the cationic Ag NPs.54,55
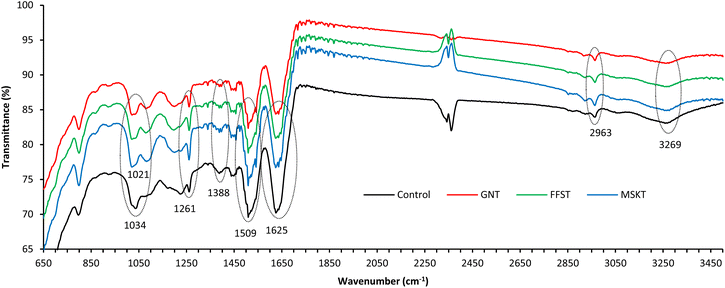 |
| Fig. 10 ATR-FTIR spectra of wool fabrics treated with 1.5% owf Ag NPs stabilized with GNT, FFST, and MSKT. | |
Mechanism of binding of tannin-stabilised Ag NPs to wool fibre
Fig. 11 shows the formation of tannin-stabilised Ag NPs and their possible binding mechanism with wool fibre. Normally, Ag NPs are positively charged,56 and they cannot bind to positively charged wool fibres in acidic conditions. The positively charged Ag NPs are stabilised by tannins by forming ionic bonds with the weak negatively charged phenolic groups of tannins forming negatively charged tannin-stabilised Ag NPs. In acidic conditions below pH 4.5, the amino groups (–NH2) of wool fibres are ionised forming cationic –NH3+ groups, which form ionic bonding with the weakly anionic phenolic groups of tannin-stabilised Ag NPs. The amino, carboxyl and hydroxyl groups of wool fibre can also form hydrogen bonding with phenolic hydroxyl groups of tannin. The described mechanisms are consistent with the FTIR spectra shown in Fig. 10. The stabilisation of nanoparticles with tannins provides them high stabilisation and forming ionic bonds with wool provides the treatment high durability to washing and reduces the leaching of Ag NPs during washing.
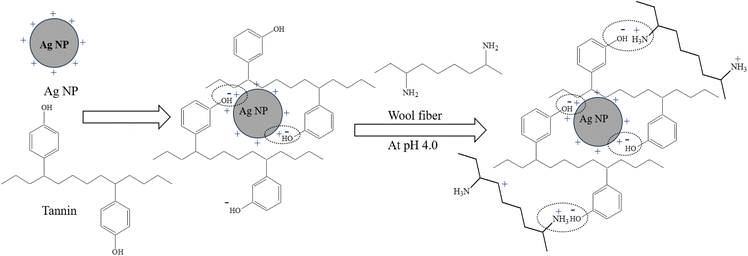 |
| Fig. 11 Possible mechanism of binding of tannin-stabilised Ag NPs to wool fibre. | |
Conclusions
This work demonstrates that tannins extracted from biomass can be utilised for the in situ formation and stabilisation of Ag NPs in wool fibres for the colouration and multi-functionalisation of wool fabrics. The optimum pH for the in situ formation of Ag NPs reduced by tannins extracted from gallnuts is 4 as the maximum colour strength was achieved at this pH. The colour strength increased with an increase in Ag concentration and also the Ag to tannin ratio. The maximum colour strength was achieved at 2% owf Ag concentration and Ag to tannin ratio of 1
:
4. Of the tannins studied, GNT extracted from gallnut produced the highest colour strength, and MSKT extracted from mango seed kernel produced the poorest colour strength for both the lowest and the highest Ag concentration studied in this work. The wool fabrics coated with 1% owf and above Ag NPs stabilised with GNT showed excellent protection against solar UV-A and UV-B radiation as the UV transmission was less than 0.2% and the UV protection performance was considerably better compared to the wool fabric coated with the same concentrations of Ag but stabilised with TSC. Of the tannins studied, GNT from gallnut provided the best protection against the harmful effects of UV and antibacterial properties, and MSKT provided the lowest but still was better than TSC. In terms of the durability to washing, still GNT provided the best performance. The SEM micrographs reveal that the surface coverage of wool fibre increased with an increase in the concentrations of Ag and GNT-stabilised Ag NPs provided the best coverage of the surface of wool fibres. Natural biomass-derived tannins can be used for the in situ formation and stabilisation of Ag NPs in wool fibres instead of sodium borohydride and other harmful reducing agents. The developed method can be used in the textile industry for the sustainable colouration and functionalisation of various types of textile fibres.
Author contributions
Mohammad Mahbubul Hassan: conceptualization, resources, project administration, funding acquisition, supervision, methodology, data analysis, data curation, formal analysis, and writing – writing original draft, review & editing. Tanushree Gupta: methodology, formal analysis, writing – original draft.
Conflicts of interest
There are no conflicts to declare.
Acknowledgements
The author acknowledges the financial support received from the Ministry of Business Innovation and Employment (MBIE) of the New Zealand Government through Grant # C10X0824. The author wishes to acknowledge the help received from Barbara Müller of RJ Hill Laboratories Limited, Christchurch (New Zealand) for helping in carrying out the antibacterial assessment of the various treated wool fabrics.
Notes and references
- M. M. Hassan and C. M. Carr, A review of the sustainable methods in imparting shrink resistance to wool fabrics, J. Adv. Res., 2019, 18, 39–60 CrossRef CAS PubMed.
- J. Szostak-Kotowa, Biodeterioration of textiles, Int. Biodeterior. Biodegrad., 2004, 53, 165–170 CAS.
- L. Teufel, A. Pipal, K. C. Schuster, T. Staudinger and B. Redl, Material-dependent growth of human skin bacteria on textiles investigated using challenge tests and DNA genotyping, J. Appl. Microbiol., 2010, 108, 450–461 CAS.
- M. M. Hassan and M. Sunderland, Antimicrobial and insect-resist wool fabrics by coating with microencapsulated antimicrobial and insect-resist agents, Prog. Org. Coat., 2015, 85, 221–229 CrossRef CAS.
- M. Tischer, G. Pradel, K. Ohlsen and U. Holzgrabe, Quaternary ammonium salts and their antimicrobial potential: targets or nonspecific interactions?, ChemMedChem, 2012, 7, 22–31 CrossRef CAS PubMed.
- B. Sarkar, M. Megharaj, D. Shanmuganathan and R. Naidu, Toxicity of organoclays to microbial processes and earthworm survival in soils, J. Hazard. Mater., 2013, 261, 793–800 CAS.
- E. Grabinska-Sota, Genotoxicity and biodegradation of quaternary ammonium salts in aquatic environments, J. Hazard. Mater., 2011, 195, 182–187 CAS.
- Y. Gao and R. Cranston, An effective antimicrobial treatment for wool using polyhexamethylene biguanide as the biocide, Part 1: Biocide uptake and antimicrobial activity, J. Appl. Polym. Sci., 2010, 117, 3075–3082 CAS.
- G. Sun, X.-G. Xu, J. R. Bickett and J. F. Williams, Durable and regenerable antibacterial finishing of fabrics with a new hydantoin derivative, Ind. Eng. Chem. Res., 2001, 40, 1016–1021 CrossRef CAS.
- Z. U. Iyigundogdu, O. Demir, A. B. Asutay and F. Sahin, Developing novel antimicrobial and antiviral textile products, Appl. Biochem. Biotechnol., 2017, 181, 1155–1166 CrossRef CAS PubMed.
- M. M. Hassan, Enhanced thermal stability, hydrophobicity, UV radiation resistance, and antibacterial properties of wool fabric treated with P-aminobenzenesulphonic acid, RSC Adv., 2020, 10, 17515–17523 CAS.
- M. B. Dickerson, A. A. Sierra, N. M. Bedford, W. J. Lyon, W. E. Gruner, P. A. Mirau and R. R. Naik, Keratin-based antimicrobial textiles, films, and nanofibres, J. Mater. Chem. B, 2013, 1, 5505–5514 RSC.
- S. B. Levy and M. Bonnie, Antibacterial resistance worldwide: Causes, challenges and responses, Nat. Med., 2004, 10, S122–S129 CAS.
- M. M. Hassan, Enhanced insect-resistance, UV protection, and antibacterial and antistatic properties exhibited by wool fabric treated with polyphenols extracted from mango seed kernel and feijoa peel, RSC Adv., 2021, 11, 1482–1492 RSC.
- M. M. Hassan, Antimicrobial coatings for textiles, in Handbook of Antimicrobial Coatings, ed. M. M. Misra, Elsevier, The Netherlands, 2017, pp. 321–355 Search PubMed.
- Y. Wang, N. Zhang, Q. Wang, Y. Yu and P. Wang, Chitosan grafting via one-enzyme double catalysis: An effective approach for improving performance of wool, Carbohydr. Polym., 2021, 252, 117157 CrossRef CAS PubMed.
- P. Zhang, N. Zhang, Q. Wang, P. Wang, J. Yuan, J. Shen and X. Fan, Disulphide bond reconstruction: A novel approach for grafting of thiolated chitosan onto wool, Carbohydr. Polym., 2019, 203, 369–377 CrossRef CAS PubMed.
- M. M. Hassan, Binding of a quaternary ammonium polymer-grafted-chitosan onto a chemically modified wool fabric surface: Assessment of mechanical, antibacterial and antifungal properties, RSC Adv., 2015, 5, 35497–35505 RSC.
- A. M. Montagut, A. Granados, C. Lazurko, A. Al-Khoury, E. J. Suuronen, E. I. Alarcon, R. M. Sebastián and A. Vallribera, Triazine mediated covalent antibiotic grafting on cotton fabrics as a modular approach for developing antimicrobial barriers, Cellulose, 2019, 26, 7495–7505 CrossRef CAS.
- M. M. Hassan, Wash-durability, surface, and antibacterial properties of wool fabric treated with nature-derived thymol and totarol under subcritical CO2, aqueous, and ethanol media, Process Saf. Environ. Prot., 2023, 177, 355–365 CrossRef CAS.
- N. Zhang, Z. Deng, Q. Wang, M. Zhou, P. Wang and Y. Yu, Phase-transited lysozyme with secondary reactivity for moisture-permeable antibacterial wool fabric, Chem. Eng. J., 2022, 432, 134198 CrossRef CAS.
- X. Liu, T. Lin, B. Peng and X. Wang, Antibacterial activity of capsaicin-coated wool fabric, Text. Res. J., 2012, 82, 584–590 CrossRef CAS.
- M. M. Hassan, Enhanced colour, hydrophobicity, UV radiation absorption and antistatic properties of wool fabric multi-functionalised with silver nanoparticles, Colloids Surf., A, 2019, 581, 123819 CrossRef CAS.
- M. M. Hassan, Antibacterial and antifungal thioglycolic acid-capped silver nanoparticles and their application on wool fabric as a durable antimicrobial treatment, ChemistrySelect, 2017, 2, 504–512 CrossRef CAS.
- J. Hong, C. Park and Y. Kim, Photothermal properties of wool fabrics coloured with SiO2@AuNPs, Colloids Surf., A, 2019, 574, 115–121 CrossRef CAS.
- A. B. Rezaie, M. Montazer and M. M. Rad, A cleaner route for nanocolouration of wool fabric via green assembling of cupric oxide nanoparticles along with antibacterial and UV protection properties, J. Cleaner Prod., 2017, 166, 221–231 CAS.
- A. Nazari, M. Montazer and M. Dehghani-Zahedani, Mothproofing of wool fabric utilizing ZnO nanoparticles optimised by statistical models, J. Ind. Eng. Chem., 2014, 20, 4207–4214 CrossRef CAS.
- N. Swilam and K. A. Nematallah, Polyphenols profile of pomegranate leaves and their role in green synthesis of silver nanoparticles, Sci. Rep., 2020, 10, 14851 CAS.
- R. Mariychuk, J. Porubská, M. Ostafin, M. Čaplovičová and A. Eliašová, Green synthesis of stable nanocolloids of monodisperse silver and gold nanoparticles using natural polyphenols from fruits of Sambucus nigra L., Appl. Nanosci., 2020, 10, 4545–4558 CrossRef CAS.
- K. Varghese Alex, P. Tamil Pavai, R. Rugmini, M. Shiva Prasad, K. Kamakshi and K. C. Sekhar, Green synthesised Ag nanoparticles for bio-sensing and photocatalytic applications, ACS Omega, 2020, 5, 13123–13129 CrossRef CAS PubMed.
- Z. Yu, J. Liu, H. He, Y. Wang, Y. Zhao, Q. Lu, Y. Qin, Y. Ke and Y. Peng, Green synthesis of silver nanoparticles with black rice (Oryza sativa L.) extract endowing carboxymethyl chitosan modified cotton with high anti-microbial and durable properties, Cellulose, 2021, 28, 1827–1842 CrossRef CAS.
- M.-H. Moosavy, M. de la Guardia, A. Mokhtarzadeh, S. A. Khatibi, N. Hosseinzadeh and N. Hajipour, Green synthesis, characterisation, and biological evaluation of gold and silver nanoparticles using Mentha spicata essential oil, Sci. Rep., 2023, 13, 7230 CrossRef CAS PubMed.
- W. Tian, Y. Hu, W. Wang and D. Yu, Synthesis of a Gemini quaternary ammonium salt and its reaction with wool fabric using click chemistry, RSC Adv., 2015, 5, 91932–91936 RSC.
- L. Hu, A. Hou, K. Xie and A. Gao, Light-induced production of reactive oxygen species by a novel water-soluble benzophenone derivative containing quaternary ammonium groups and its assembly on the protein fibre surface, ACS Appl. Mater. Interfaces, 2019, 11, 26500–26506 CrossRef CAS PubMed.
- M. M. Hassan, Enhanced antistatic and mechanical properties of corona plasma treated wool fabrics treated with 2,3-epoxypropyltrimethylammonium chloride, Ind. Eng. Chem. Res., 2014, 53, 10954–10964 CrossRef CAS.
- M. M. Hassan and C. M. Carr, A critical review on recent advancements of the removal of reactive dyes from dyehouse effluent by ion-exchange adsorbents, Chemosphere, 2018, 209, 201–219 CrossRef CAS PubMed.
- T. Borrmann, T. H. Lim, H. Cope, K. Lucas and M. Lorden, Gold nanoparticles on wool in a comparative study with molecular gold catalysts, Gold Bull., 2013, 46, 13–18 CrossRef CAS.
- S. Mowafi, H. Kafafy, A. Arafa, K. Haggag and M. Rehan, Facile and environmentally benign in situ synthesis of silver nanoparticles for multifunctionalisation of wool fibres, Environ. Sci. Pollut. Res., 2018, 25, 29054–29069 CrossRef CAS PubMed.
- F. M. Kelly and J. H. Johnston, Coloured and functional silver nanoparticle-wool fibre composites, ACS Appl. Mater. Interfaces, 2011, 3, 1083–1092 CrossRef CAS PubMed.
- M. M. Hassan and K. Koyama, Multifunctional acrylic fibres prepared via in-situ formed silver nanoparticles: Physicochemical, UV radiation protection, and antistatic properties, Dyes Pigm., 2018, 159, 517–526 CrossRef CAS.
- M. Rehan, N. S. Elshemy, K. Haggag, A. S. Montaser and G. E. Ibrahim, Phytochemicals and volatile compounds of peanut red skin extract: simultaneous colouration and in situ synthesis of silver nanoparticles for multifunctional viscose fibres, Cellulose, 2020, 27, 9893–9912 CrossRef CAS.
- A. Krkobabić, M. Radetić, H.-H. Tseng, T. S. Nunney, V. Tadić, T. Ilic-Tomic and D. Marković, Green in situ synthesis of Ag- and Cu-based nanoparticles on viscose fabric using a Punica granatum peel extract, Appl. Surf. Sci., 2023, 611, 155612 Search PubMed.
- M. M. Hassan, Energy-efficient dyeing of wool fabrics with sulphonic acid derivatives of aniline by oxidation polymerisation, Sustainable Mater. Technol., 2021, 29, e00290 CrossRef CAS.
- P. S. Sobolewski, J. W. Krzyścin, J. Jarosławski, J. Wink, A. Lesiak and J. Narbutt, Controlling adverse and beneficial effects of solar UV radiation by wearing suitable clothes – Spectral transmission of different kinds of fabrics, J. Photochem. Photobiol., B, 2014, 140, 105–110 CAS.
- E. Louris, E. Sfiroera, G. Priniotakis, R. Makris, H. Siemos, C. Efthymiou and M. N. Assimakopoulos, IOP Conf. Ser.: Mater. Sci. Eng., 2018, 459, 012051 Search PubMed.
- M. Ferri, K. Papchenko, M. Degli Esposti, G. Tondi, M. G. De Angelis, D. Morselli and P. Fabbri, Fully biobased polyhydroxyalkanoate/tannin films as multifunctional materials for smart food packaging applications, ACS Appl. Mater. Interfaces, 2023, 15, 28594–28605 CrossRef CAS PubMed.
- M. K. Rai, S. D. Deshmukh, A. P. Ingle and A. K. Gade, Silver nanoparticles: the powerful nanoweapon against multidrug-resistant bacteria, J. Appl. Microbiol., 2012, 112, 841–852 CrossRef CAS PubMed.
- U. Klueh, V. Wagner, S. Kelly, A. Johnson and J. D. Bryers, Efficacy of silver-coated fabric to prevent bacterial colonization and subsequent device-based biofilm formation, J. Biomed. Mater. Res., 2000, 53, 621–631 CrossRef CAS PubMed.
- S. Kumar, S. Howdle and H. Münstedt, Polyamide/silver antimicrobials: effect of filler types on the silver ion release, J. Biomed. Mater. Res., Part B, 2005, 75, 311–319 Search PubMed.
- S. Belhaoues, S. Amri and M. Bensouilah, Major phenolic compounds, antioxidant and antibacterial activities of Anthemis praecox Link aerial parts, S. Afr. J. Bot., 2020, 131, 200–205 CrossRef CAS.
- C. Díaz-Cruz, G. Alonso Nuñez, H. Espinoza-Gómez and L. Z. Flores-López, Effect of molecular weight of PEG or PVA as reducing-stabilizing agent in the green synthesis of silver-nanoparticles, Eur. Polym. J., 2016, 83, 265–277 CrossRef.
- M. M. Hassan, Valorisation of sulphonated lignin as a dye for the sustainable colouration of wool fabric using sustainable mordanting agents: enhanced colour yield, colourfastness, and functional properties, RSC Sustainability, 2024, 2, 676–685 RSC.
- M. M. Hassan, Wool fabrics coated with an anionic Bunte salt-terminated polyether: Physicomechanical properties, stain resistance, and dyeability, ACS Omega, 2018, 3, 17656–17667 CrossRef CAS PubMed.
- G. Montes-Hernandez, M. Di Girolamo, G. Sarret, S. Bureau, A. Fernandez-Martinez, C. Lelong and E. Y. Vernain, In situ formation of silver nanoparticles (Ag-NPs) onto textile fibres, ACS Omega, 2021, 6, 1316–1327 CrossRef CAS PubMed.
- M. Milošević, M. Radoičić, Z. Šaponjić, T. Nunney, C. Deeks, V. Lazić, T. Radetić and M. Radetić, In situ photoreduction of Ag+-ions by TiO2 nanoparticles deposited
on cotton and cotton/PET fabric, Cellulose, 2014, 21, 3781–3795 CrossRef.
- X. Chen, S. Hu, X. Qin, M. Chen, J. Zhang, X. Bao and C. Zhang, Single-atom cationic Ag and metallic Ag nanoparticles supported on Al2O3 as catalysts for water-resistant and CO2-selective HCHO oxidation, ACS Appl. Nano Mater., 2023, 6, 8763–8771 CAS.
|
This journal is © The Royal Society of Chemistry 2024 |
Click here to see how this site uses Cookies. View our privacy policy here.