DOI:
10.1039/D3RA07325D
(Review Article)
RSC Adv., 2024,
14, 7981-7991
Synthesis and pharmacological activity of vinpocetine derivatives
Received
27th October 2023
, Accepted 22nd February 2024
First published on 7th March 2024
Abstract
Vinpocetine and its derivatives were extensively employed in the treatment of ischemic stroke, serving as effective cerebrovascular vasodilators. They could also be utilized for neuroprotection, anti-inflammatory purposes, anti-aging interventions, insomnia treatment, and antidepressant effects. However, due to issues such as hepatic first-pass effect, low bioavailability, and poor patient compliance with multiple dosing, the secondary development of Vinpocetine to address these limitations became a prominent area of research. Five primary methodologies were employed for the synthesis of Vinpocetine derivatives. These included substitution on the A ring to modify the 14-ester group, alteration of the 16-ethyl group, simplification of the D and E rings, and modification of the conformation of Vinpocetine. This paper summarized the current synthesis and activity studies of Vinpocetine and its derivatives, with the aim of providing a reference for the discovery of more potent derivatives of Vinpocetine.
1 Introduction
Vinpocetine is a semi-synthetic indole alkaloid of Vincamine derived from the small vine periwinkle flower of the family Oleaceae, also known as ethyl apo-periwinkle-22-acetate, which is an indole alkaloid with a high degree of lipid solubility and easily crosses the blood–brain barrier into brain tissue1–3 and is therefore widely used in cerebrovascular diseases such as stroke and cognitive impairment (Fig. 1). It was developed by Gedeon Richter Pharmaceuticals in Hungary and marketed in 1978. It is marketed in Japan under the trade name Calan and abroad under the trade name Covington. Northeast Pharmaceutical General Factory's production of Vinpocetine API and tablets, which not only increases cerebral blood flow in ischemic brain areas, but also relaxes vascular smooth muscle, enhances the deformation ability of red blood cells, promotes the release of oxygen from hemoglobin and removes atherosclerotic plaque, etc. It is known as one of the world's top ten beneficial anti-ageing drugs. Therefore, it has also become an important direction of research in recent years. This paper summarizes the research on Vinpocetine derivatives in recent years and lays the foundation for future research on Vinpocetine derivatives.
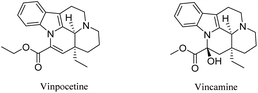 |
| Fig. 1 Vinpocetine and Vincamine. | |
2. Studies on the pharmacological effects of Vinpocetine
In recent years, the pharmacological aspects of Vinpocetine have been actively studied at home and abroad. A review of the relevant domestic and international literature shows that Vinpocetine has a variety of pharmacological activities, it is a good cerebral vasodilator, neuroprotective agent, anti-inflammatory agent, etc. It also has antioxidant, anti-aging and antidepressant effects. The literature on the pharmacological effects of Vinpocetine was collected and summarized.
2.1 Neuroprotective effects
There are many targets of action of Vinpocetine, such as phosphodiesterase type 1 (PDE1) proved to be the first target of action of Vinpocetine in the 1990s. It can enhance neuronal plasticity by regulating PDE1 to increase the level of the second messenger cAMP (cyclic adenosine monophosphate)/cGMP (cyclic guanosine monophosphate), and improves the ability of neural reorganization in the brain (Fig. 2).4 Vinpocetine is a good neuroprotective agent that dilates cerebral blood vessels, increases the oxygen content of arterial and venous blood in the brain, improves the utilization of blood oxygen in the brain and improves the metabolism of hypoxic brain tissue.5 Vinpocetine is also widely used in the treatment of Alzheimer's disease and studies have shown that it can improve the degeneration of neurons caused by changes in Aβ (amyloid-beta), leading to symptoms such as memory.6 Vinpocetine is known to be an effective treatment for ischaemic strokes and has been shown to improve blood circulation in the eye and also to regulate normal function during retinal ischaemia, reducing LDH (lactate dehydrogenase) to normal levels in the retina during ischaemia and increasing glucose utilisation.7 Zhao found that Vinpocetine has neuroprotective effects on oxidative stress, inflammation and apoptosis induced by brain I/R injury, and its mechanism may be related to the PI3K/AKT pathway of astrocytes. As shown in Fig. 3, Vinpocetine significantly reduced inflammation induced by brain I/R injury by reducing astrocyte activation.8 Shekarian9 found that Vinpocetine improves Aβ-induced neuronal plasticity damage. It can prevent the synaptic plasticity of hippocampal PP-DG pathway induced by Aβ (amyloid-beta). Depression is a typical condition of depressive disorders and is commonly seen in high pressure populations. A study by Ni showed that when Vinpocetine was combined with fluoxetine hydrochloride (Prozac), the improvement in sleep disturbance factors was more pronounced compared to fluoxetine hydrochloride alone.10
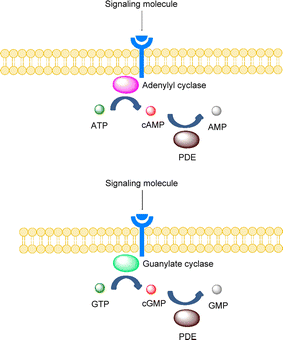 |
| Fig. 2 Vinpocetine alters cAMP/cGMP levels via PDE1.4 | |
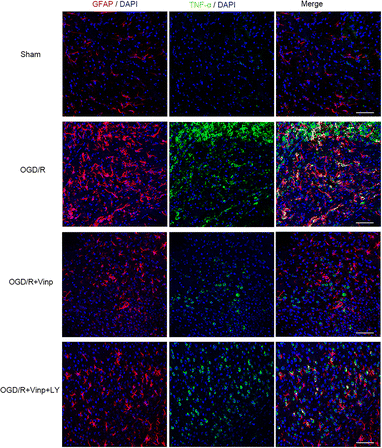 |
| Fig. 3 Effects of Vinpocetine on reactive astrocytes and TNF-α in the rat cerebral cortex after I/R injury. Brain slices were analyzed with double immunostaining using GFAP (red, a reactive astrocytic marker) and TNF-α (green, a pro-inflammatory cytokine).8 | |
2.2 Treatment of ischaemic strokes
Ischemic stroke is an acute cerebrovascular disease caused by blockage of cerebral blood vessels triggering local ischemia and hypoxia in brain tissue, which leads to neurological dysfunction or loss of function in the corresponding area. It is commonly seen in middle-aged and elderly people, with a high mortality and disability rate, and the incidence is on the rise as China gradually enters an ageing population. Dong found that Vinpocetine could promote the uptake and utilization of oxygen and glucose by ischemic and hypoxic nerve cells, improve energy metabolism, promote aerobic oxidation and ATP (adenosine triphosphate) production, thus greatly increasing the production of energy in diseased cells, resulting in reduced cerebral edema and reduced oxygen radical production to achieve the protective effect of cerebral ischemia.11 Several studies have found that Vinpocetine improves neurological impairments and significantly improves daily mobility.12,13 Studies have shown that there is structural and functional plasticity and reconstruction of the central nervous system after stroke. After the patient's vital signs are stable, early use of Vinpocetine injection combined with appropriate exercise therapy can promote physical recovery, improve quality of life and reduce the occurrence of sequelae. Svab14 found that Vinpocetine can reduce Ca2+ induced mitochondrial Ca2+ release and Ca2+ induced mitochondrial swelling. The rate of mitochondrial ATP (adenosine triphosphate) synthesis was decreased and the activity of ATP (adenosine triphosphate) ase was increased. The correlation of mitochondrial mechanism in the anti-ischemia effect of Vinpocetine was also discussed. Bagri15 found that Vinpocetine treatment had a significant restorative effect on rats with traumatic brain injury, with improved cognitive and motor function changes, and also restored the biochemical changes caused by traumatic brain injury. Han16 found that Vinpocetine alleviates ischemic stroke by regulating levels of the NLRP3 inflammasome, NF-kB, and pro-inflammatory cytokines in vivo.
2.3 Antioxidant and anti-inflammatory effects
Oxygen free radicals are associated with a variety of diseases (cerebrovascular disease, cardiovascular disease, etc.) and are highly reactive chemicals produced by biological systems during many physiological and pathophysiological processes and play an important role in cellular metabolism and cellular defense systems, but the accumulation of large amounts of oxygen free radicals can have toxic effects on tissues and cells. Horvath found that Vinpocetine protected erythrocytes from oxygen free radical damage etc.17 Several studies have found that the antioxidant effects of perphenazine may be due to its ability to reduce dopamine (DA) secretion in the striatum, reducing the production of oxidative stress. Santos18 found that Vinpocetine inhibited ROS formation and lipid peroxidation in rat brain synaptosomes. As shown in Fig. 4 Vinpocetine also prevented the formation of reactive oxygen species. Vinpocetine has been shown to be a good anti-inflammatory agent. Vinpocetine inhibits the expression of NF-κB-dependent inflammatory molecules by directly inhibiting IKK (inhibitor kinase) activity, attenuating IKK-mediated phosphorylation of IκB and increasing the stability of IκB, which leads to IκB binding to NF-κB.19–21 Colombo22 found that Vinpocetine can improve acetic acid-induced colitis by inhibiting neutrophil recruitment, abdominal hyperalgesia, oxidative stress, pro-inflammatory cytokine production, and NF-κB activation. In addition, Vinpocetine can also have a strong ameliorative effect on AP by modulating Sirt1 and thus acting on nuclear factor (Nrf2), activating it to induce its nuclear translocation and thus acting on TNF to reduce oxidative stress, inflammatory processes and apoptosis in AP.23 In smooth muscle there are several forms of cyclic nucleotide phosphodiesterase's (PDE) and Vinpocetine inhibits one of them (Ca2+ PDE) to relax smooth muscle demonstrated that Vinpocetine alleviates spasticity and reduces the presence of several inflammatory markers in amyotrophic lateral sclerosis (ALS), but the exact mechanism remains undiscovered.24 In addition, the combination of Vinpocetine and nimodipine has been shown to be effective in myocardial infarction, and Vinpocetine has been found to inhibit osteogenic differentiation of VSMC by inducing phosphorylation of ERK and Akt to prevent vascular calcification.25 Vinpocetine inhibits cellular senescence by significantly increasing insulin secretion from aged pancreatic islets and reducing oxidative stress and inflammation to break the vicious cycle of oxidative stress and senescence.26
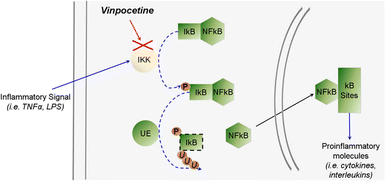 |
| Fig. 4 Schematic representation of IKK inhibition by Vinpocetine. | |
2.4 Other roles
Vinpocetine also showed significant improvement in novel coronary pneumonia. A review of the data found that the anti-inflammatory effects of Vinpocetine are mainly mediated through the regulation of inducer-κB kinase (IKK) and the degradation of IκB which regulates NF-κB activity. The interaction between SARS-CoV-2 and angiotensin-converting enzyme 2 (ACE2) activates the NF-κB signaling pathway to trigger inflammatory responses in the lung. Therefore, Vinpocetine may regulate the development of COVID-19-induced inflammation by modulating the NF-κB pathway and reducing PDE activity27 (as shown in Fig. 5). Vinpocetine has been shown to enhance memory in animal models.28
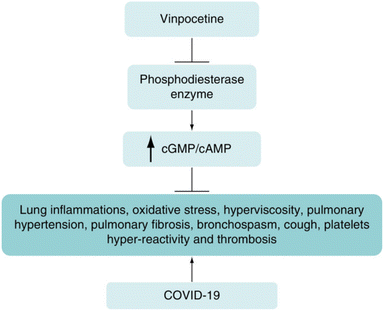 |
| Fig. 5 Vinpocetine ameliorates COVID-19 inflammation by modulating NF-κB pathways and reducing PDE activity. | |
2.5 Mode of drug metabolism of Vinpocetine
The current low oral utilization of Vinpocetine is due to its strong metabolism by human liver cells, which can be hydrolyze into apo-Vinpocetine by enzymes in the liver after entering the body. Therefore, how to reduce the hepatic first-pass effect of Vinpocetine in humans is also a hot topic of research at present. For example, Aldawsari29 administered the drug intranasally by means of surface-tailored intranasal emulsions to promote blood–brain barrier penetration. In contrast, Wang prepared nanoparticles loaded with Vinpocetine (VIN mPEG-PLA) by emulsion solvent evaporation, and the addition of mPEG-PLA NPs significantly enhanced the systemic absorption of Vinpocetine compared to conventional Vinpocetine injections.30 Vinpocetine also alleviates nicotine and ethanol-induced hyperactivity and memory/learning deficits through cAMP (cyclic adenosine monophosphate) and cGMP (cyclic guanosine monophosphate) signaling.31
2.6 Combined use of Vinpocetine
A study conducted by Zhang revealed that the combination of Vinpocetine and sorafenib inhibits Vinpocetine-induced cytoprotective autophagy. This combination demonstrated synergistic anti-hepatocellular carcinoma (HCC) activity by activating GSK-3β. Additionally, the Vinpocetine and sorafenib combination reversed sorafenib resistance through the PI3K/protein kinase B/GSK-3β signaling axis.32 In a study by Ansari, it was found that both nimodipine and Vinpocetine individually exhibited cardioprotective effects. However, the combination of nimodipine and Vinpocetine resulted in superior recovery in terms of oxidative stress, myocardial membrane injury, hemodynamics, histopathology, and ultrastructural changes.33 Wang discovered that the combined administration of Vinpocetine and ozagrel may offer beneficial effects for patients with cerebral ischemia compared to the singular administration of Vinpocetine.34 Ishihara observed that the combination of vincristine and idebenone had a prolonged growth effect on CA pyramidal layer population spikes in the guinea pig hippocampal slice region.35 In a study by Zhang, the combination of vincristine and dexamethasone was found to reduce the expression levels of serum inflammatory cytokines, including TLR2, TLR4, interleukin (IL)-20, IL-8, tumor necrosis factor-alpha, interferon-gamma, monocyte chemotactic protein 2, and interferon-inducible protein 20. This reduction in cytokine expression alleviated cognitive deficits in nasopharyngeal carcinoma patients after radiotherapy.36 Hu demonstrated that the combination of Vinpocetine and coenzyme CoQ10 ameliorated neuronal damage, promoted mitochondrial phagocytosis, and alleviated cognitive deficits in ischemia-reperfusion (IR) mice.37 Park38 found that both vincristine and ferulic acid improved memory function by reducing amyloid β-protein tangles in the hippocampus by enhancing insulin action induced in Alzheimer's disease in type 2 diabetic rats. It is further suggested that the combination of the two may improve the efficacy against memory impairment, but related studies have not yet been conducted.
3. Synthesis of Vinpocetine
Vinpocetine is a semi-synthetic derivative of perphenazine, whose basic backbone consists of four six-membered rings and one five-membered ring, which can be labelled as A, B, C, D, E according to the order. Vinpocetine can be synthesized either by chemical means by total synthesis or by semi-synthesis by extracting perphenazine from plants as raw material. As designed by Qin perphenazine is obtained by dehydration of sulfoxide chloride, hydrolysis by sodium hydroxide, and then esterification of sulfoxide chloride and ethanol to obtain perphenazine (Scheme 1).39 Industry often uses perphenazine as a raw material for the solid acid-base catalyzed synthesis of perphenazine. This method reduces the production cost of perphenazine and the production process does not produce acid-base wastewater, which is green. We have found that when using perphenazine as a raw material and FeCl3 and SnCl4 as catalysts, no intermediates are formed and perphenazine is directly converted to perphenazine with simple operation and yields of up to 80%, which can be used for synthesis above the kilogram level.
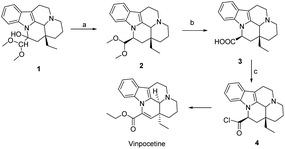 |
| Scheme 1 Preparation of Vinpocetine by thionyl chloride method (a) SOCl2, DMF, 25 °C; (b) NaOH, H2O, EtOH, 80 °C; (c) SOCl2, EtOH, 78 °C. | |
The total synthesis strategy of perphenazine is generally divided into two types, (1) the direct construction of the ABCD ring from the AB ring and (2) the construction of the CD ring from the AB ring followed by the construction of the E ring by combining with the three-carbon ring. The chemical synthesis of perphenazine alkaloids was mainly concentrated in the 1960s and 1980s. In 1997, Alves40,41 designed a multi-step conversion synthesis method using 2-ethyl cyclopentanone as the raw material, and then obtained the key chiral lactone by asymmetric Michael alkylation induced by chiral imine, and then by transesterification, double Koehler-Napier cyclization and hydrogenation reduction. The key intermediates for the synthesis of Vinpocetine was obtained by transesterification, double Koehler-Napier cyclization and hydrogenation reduction, and finally Vinpocetine was obtained, as shown in Scheme 2.
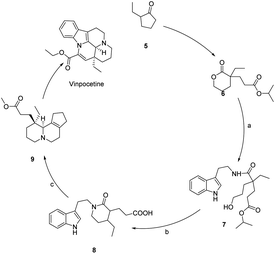 |
| Scheme 2 Synthesis of Vinpocetine from 2-ethylcyclopentanone (a) tryptamine, DMF, 100 °C, (b) CBr4, CH2Cl2, KH, 18-crown-6, THF, (c) POCl3, H2, Pd/C. | |
A key intermediate for the synthesis of perphenazine can also be made from tryptamine Wenkert's enamine, which constructs ABCD teracycle and has good industrial value but is complex to operate. Nemes42 devised a splitting-based method for the preparation of perphenazine, using Wenkert's enamine as a raw material, which was cyclized by Michael addition with different reagents, and by tartaric acid splitting was followed by several reactions to produce perphenazine, perphenazine, etc. This method is simple and the intermediates obtained from the reaction can also be reductively opened under different conditions to give the trans structure of Vinpocetine, as shown in Scheme 3.
 |
| Scheme 3 Preparation of Vinpocetine (a) bromopyruvate oxime, benzyltriethylammonium chloride, CH2Cl2, NaOH/H2O; (b) tartaric acid, CH2Cl2, ethanol, p-toluenesulfonic acid, toluene, HClO4; (c) NaBH4, ethanol. | |
4. Preparation of Vinpocetine derivatives and study of their biological activity
Vinpocetine is a poorly water-soluble active agent, which has an extremely short half-life. These deficiencies may lead to reduced bioavailability and lower brain concentrations, limiting its clinical application. A review of the literature reveals that most of the reports on derivatives of Vinpocetine are limited to chemical synthesis, with few reports on studies of their biological activity. Therefore, the literature on the derivatives of Vinpocetine was collected and summarized. There are five main ways to prepare the derivatives of Vinpocetine: substitution of different groups on the A ring, alteration of the ester group structure at the 14 position, alteration of the ethyl structure at the 16 position, simplification of the D and E rings, and alteration of the conformation of Vinpocetine. I will describe each of these five aspects next.
4.1 Substitution of different structural groups on the A ring
The introduction of different substituents on the A-ring is one of the common methods for structural modification of Vinpocetine, and systematic structural modification of the A-ring of Vinpocetine has been reported in the 1970s, but mostly at the chemical synthesis level. Pathway for obtaining derivatives of cetin. The introduction of nitro, amide, hydroxyl and methyl groups on the A-ring can alter the original biological activity. Fitos43 found that the introduction of nitro (compound 13) at the 11 position of Vinpocetine enhanced its esterophilicity, and when NHCOCF3 (compound 14) and NHCOPh were introduced the esterophilicity became less as the group was increased. Moldvai44 used Vinpocetine as a raw material and added an excess of chloro-sulphonic acid to chloroform and reacted at 0–5 °C to obtain sulfonyl chloride introduced at the 10 or 11 position in Vinpocetine, both in similar yields. Since sulfonyl chloride is more sensitive, sulfonyl chloride was also converted to sulfonamide group to obtain the derivatives of Vinpocetine (compound 15) with sulfonamide group introduced at 11 and 10 positions. A series of A-ring substituted derivatives were prepared by varying the reactants, as shown in Fig. 6, and it was found that the peripheral vasodilatory effect was enhanced by the introduction of the sulfonamide group, and by the introduction of the 3-methoxypropylamine group. It has been found that when Vinpocetine is substituted with bromine at the 10 position (compound 16) it is also an effective drug for the treatment of ischaemic diseases of the brain and heart, which are increasing in our society today. It can be used as a vasodilator for vascular tissue.45
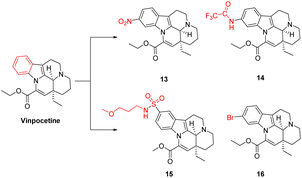 |
| Fig. 6 Modification of Vinpocetine's A ring. | |
4.2 Change in the structure of the E and D rings
The basic structure of Vinpocetine is thickened by five rings, A, B, C, D and E. There are three chiral centers, and the route of total synthesis is relatively complex and not easy for industrial production. In recent years, domestic and foreign research has focused on the conformational relationship of Vinpocetine and structural simplification, as shown in Fig. 7, and found that only A, B, C and D may be more beneficial for industrial synthesis of cerebrovascular therapeutic drugs.
 |
| Fig. 7 Modification of Vinpocetine's D ring and E ring. | |
Xu46 used tryptamine as a raw material and underwent a series of reactions to obtain compound 24, which had better vasodilatory activity compared with the usual Vinpocetine compounds. In addition, Wincott's enamine can also be used as a raw material for various acrylic vinegars, which are first subjected to a Michel addition reaction and then reduced to obtain compound 22, which doubles the limb blood flow and quadruples the cerebral blood flow as compared to the usual Vinpocetine compounds, and which is currently used clinically for the treatment of cerebrovascular diseases. In addition, enamine can also react with propylene eye in an addition reaction to obtain the perchlorate product, and the hydrogenation catalyzed by 5% palladium–cerium at atmospheric pressure gave compound 26, and the results of animal pharmacological experiments showed that the vasodilatory activity of compound 26 was even stronger than that of the control (ethanol dehydrated Vinpocetine) (Fig. 8).
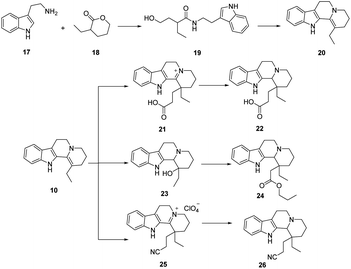 |
| Fig. 8 Modification of Vinpocetine's E ring. | |
As shown in Fig. 9, Nemes47 designed and synthesized derivatives of perphenazine by destroying the E ring of perphenazine and found that the compound exhibited significant peripheral vasodilating effects and that replacing the carboxyl ester on the compound with a dialkylaminomethyl chain (compound 27) enhanced neuroprotection and LPO inhibitory activity but completely lost its vascular and anti-hypoxic properties. A structural analog of perphenazine has been discovered (compound 28), which forms a ring through an oxygen atom at positions 17 and 14.48 The most representative derivative of the simplified D-ring of Vinpocetine is vinconate (compound 29), which has been marketed in Japan for the treatment of cerebral ischemic, cognitive enhancement and brain stimulation. Studies have shown that vinacate also stimulates muscarinic acetylcholine receptor-mediated phosphatidylinositol flipping in rat cortical slices, accelerating phosphatidylserine translocation thereby contributing to this accelerates the coupling of muscarinic receptors to G proteins, resulting in vasodilation. And vinconate ameliorated to some extent the reduction in [3H]QNB, [3H]hemicholine-3 and [3H]myo-inositol binding in the brain of aging rats, which may have beneficial effects on age-related changes in neurotransmission and cognitive function.49
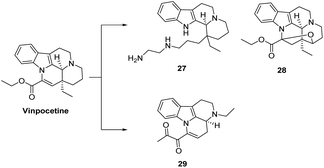 |
| Fig. 9 Disruption of the E-loop of Vinpocetine or formation of a connecting bridge between the D-loop and the E-loop. | |
The alkaloid perphenazine and its semisynthetic product ethyl perphenazine have been widely used abroad as therapeutic agents for cerebrovascular diseases, but considering that perphenazine and perphenazine are both pentacyclic thickeners with complex structures, which are difficult to synthesize, combined their work on simplifying perphenazine abroad to design 1,2,3,4,5,6,7,12,12b-octahydroindolo[2,3-a]thiazine derivatives. The aim of this study was to find structurally simple and highly effective drugs for cerebrovascular diseases. A series of 1,2,3,4,5,6,7,12,12b-octahydroindolo[2,3-a] thiazine derivatives (compounds 30–38) were synthesized from allylamine as shown in Method 4, but their specific activities are still under further investigation (Scheme 4).
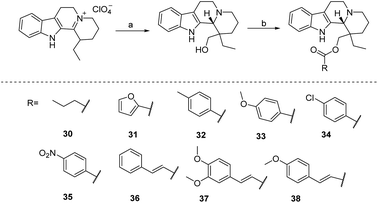 |
| Scheme 4 Preparation of Vinpocetine derivatives from enamine Wenkert (a) HCHO, htinig base, N2; (b) RCOCl. | |
4.3 Change of ester group at position 14
Changing the ester group at the 14 position of perphenazine is a common method for the preparation of perphenazine derivatives, e.g. the substitution of the ester group at the 14 position with an amide results in enhanced peripheral vasodilating activity and longer duration of action compared to perphenazine. Sheng50 hydrolysis of Vinpocetine was carried out to obtain compound 39, which was then reacted with DMF, 1,2-dibromoethane or 1,3-dibromopropane, and K2CO3 at room temperature to obtain compound 40. Compound 40 was then processed with various amines and K2CO3 in DMF at 70 °C and nitrogen to obtain the target compounds (compounds 41–46), which were evaluated for their antioxidant activities, laying the foundation for the development of novel cerebrovascular drugs (Scheme 5).
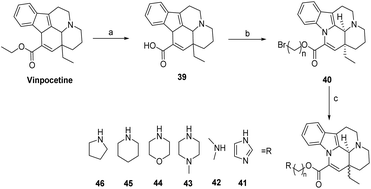 |
| Scheme 5 Change Vinpocetine 14 ester group (a) NaOH, EtOH, reflux, N2, AcOH; (b) Br(CH2)nBr, K2CO3, DMF, rt; (c) R/K2CO3, DMF, 70 °C, N2. | |
Nemes47 introduced Na atoms in the form of ionic bonds in the 14th position of the ester group as a raw material for Vinpocetine to obtain compound 47, which was then reacted with different substrates to obtain a series of Vinpocetine derivatives with different R groups (compounds 48–51) as shown in Scheme 6. The antioxidant effects of several compounds were also investigated in vitro using both enzymatic lipid peroxidation (NADPH-induced LPO) and non-enzymatic lipid peroxidation (Fe2+-stimulated LPO) methods. Compounds 48–51 were found to be active inhibitors of LPO in both NADPH- or Fe2+-induced rat brain microsomes and rat brain homogenate, proliferation and termination processes of Fe2+-induced LPO in rat brain homogenates.
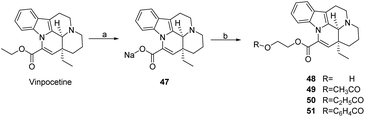 |
| Scheme 6 Sodium atoms were introduced to the 14-position ester group (a) NaOH, EtOH, reflux; (b) DMF, 100 °C, ClCH2CH2OR. | |
In Scheme 7, Kawashima51 converted the ester group in the 14th position to a (nitrooxy)alkyl alcohol via bromoalkyl alcohols and AgNO3–CH3CN to give compound 52. And the activity of compound 52 on vertebral artery blood flow (VBF) and femoral artery blood flow (FBF) in anesthetized dogs decreases as n on the ester group in the 14th position increases with a longer chain.
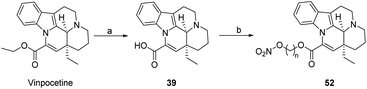 |
| Scheme 7 Nitrooxy group is introduced into the ester group at the 14 positions (a) ester hydrolysis; (b) O2NO(CH2)nOH, SOCl2, DMF. | |
This is shown in Fig. 10, several studies have shown that the introduction of a nitro group at position 14 of Vinpocetine enhances (compound 53) the vasodilatory activity of Vinpocetine derivatives by releasing NO. These derivatives were able to dose-dependently (0.03–1.0 mg kg−1, iv) enhance cerebral blood flow in Beagle dogs, and the enhancement of vertebral and femoral blood flow was 3.8 and 4.7 times higher than that of Vinpocetine, respectively. The results showed that these derivatives showed enhanced neuronal protection and anti-lipid peroxidation, but completely lost the original anti-hypoxic and vasodilatory activity of Vinpocetine. If the ester group is hydrolyzed to give apo-Vinpocetine, the peripheral vasodilatory activity is preserved, but the cerebrovascular activity is greatly reduced. This shows that the presence of the ester group at position 14 plays an important role in the cerebrovascular activity of Vinpocetine. Some data suggest that eburnamonine (compound 55), obtained by replacing the ester group at position 14 by a carbonyl group, is used to treat cerebrovascular disease, but it has little activity against HL-60 (human leukemia cells) and MDA-MB231 (breast cancer cells), but in canine studies increased oxygen and glucose consumption in the brain and improved brain by increasing blood flow supply.52
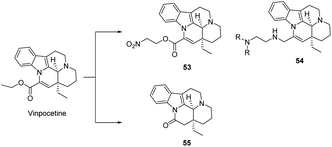 |
| Fig. 10 Introduction of nitro or amino groups to the 14-position ester group of Vinpocetine, etc. | |
Nag53 designed the synthesis of compounds containing [18F] at the 14 position and obtained the title compound 25 in 39% yield by reacting commercially available apovincamine acid with 1-bromo-2-fluoroethane for 21 h. It was also found that fluoroperoxetine (compound 56) was extremely similar to peroxetine in terms of its binding to human TPOS proteins, and was produced from ethyl bromide toluenesulfonate via kryptofix-mediated 18F-fluorination nucleophilic reaction in o-DCB to produce the alkylating agent [18F] fluoroethyl bromide in an alkaline solution in DMF of apo-veneic acid and heated at 140 °C for 5 min. After HPLC purification, irradiation at 35 μA for 15 to 20 min gave 1.9–2.7 GBq [18F] fluoroperoxetine (compound 57); and a PET study of plasma metabolism in crab-eating monkeys showed that [18F] fluoroperoxetine has high blood–brain barrier permeability, a regional uptake pattern and rapid clearance from the NHP brain (Scheme 8).
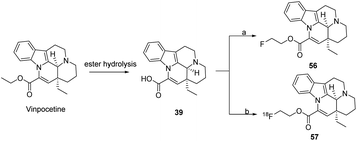 |
| Scheme 8 Introduction of the F group into the ester group at position 14 (a) FCH2CH2Br, NaHCO3, DMF, rt; (b) 18FCH2CH2Br, NaHCO3, DMF, rt. | |
As shown in Scheme 9, Pan54 synthesized a series of Vinpocetine derivatives, including thiocarbamate derivatives, methyl thiocarbamate derivatives, thiocarbamoyl derivatives and thiocarbamate derivatives. The inhibition activity of the synthesized compounds against PDE1A was measured and found to be enhanced by the introduction of fluorine atoms on the phenyl group in the thiocarbamates, with the inhibition activity of the fluorophenyl substituted compound 63 being 5-fold higher than that of Vinpocetine with an IC50 value of 3.68 ± 0.21 μM. The morpholinecarbonyl substituted compound 70 in the acylthiocarbamate derivatives showed better inhibition activity than the Vinpocetine showed better inhibitory activity with an IC50 value of 8.42 ± 0.67 μM. The interaction of the highly effective compounds with PDE1A was also determined by surface plasmon resonance (SPR) binding and these compounds showed higher binding affinity for PDE1A compared to Vinpocetine, which was consistent with the inhibitory activity. The vasorelaxant effect of Vinpocetine and its derivatives on endothelium-intact rat thoracic aortic rings was investigated and it was found that the relaxing effect of compound 63 was significantly enhanced compared to Vinpocetine, whereas the relaxing effect of compound 70 was significantly diminished.
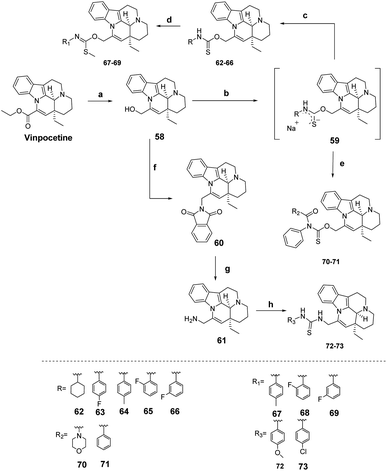 |
| Scheme 9 Introduction of a thioamino group into the 14-position ester group (a) LiAlH4, dry THF; (b) isothiocyanates, NaH (60%), dry THF; (c) NH4Cl (aq.); (e) dry pyridine, ArCOCl; (d) MeI, K2CO3, DMF; (f) phthalimide, PPh3, DEAD, dry THF; (g) N2H4·H2O, EtOH, reflux; (h) isothiocyanates, acetone. | |
This is shown in Fig. 11, Fitos43 found that enhancement of the hydrophobic group at the 14-ester group significantly enhanced the binding affinity of the compound. Mazák55 determined the lipophilicity of different Vinpocetine derivatives by reverse thin-layer chromatography and found that the hydroxyl derivative of Vinpocetine (compound 74) was more lipophilic than the Vinpocetine derivative, probably because the chain length and substituents of most molecules are highly esterophilic in nature. The introduction of hydroxyl group at the 14 position affects the lipophilicity of the Vinpocetine backbone, while the addition of methylene and ethylene reduces the polarity of the side chain and enhances the lipophilicity of the compound. A series of derivatives of Vinpocetine designed by Pudleiner56 were able to promote tubule formation in vascular endothelial cells, and compounds 75–77 were more effective than Vinpocetine apovinaminic acid (AVA) (compound 39), the main metabolite of Vinpocetine produced by ester hydrolysis, is a hydrophilic acid. It has been shown that although AVA is less lipophilic, it is also able to cross the blood–brain barrier and, as apovinaminic acid (AVA) is an acidic compound, it is more readily absorbed into the stomach through gastric tissues, but the main site of absorption of Vinpocetine is in the small intestine.57 A number of studies have shown that when the 14-ester group of Vinpocetine was replaced by an alcoholic hydroxyl group (compound 78), which also reduces [3H]barbitoxin binding in rat cortical synaptosomes, protects cortical neurons, is a potent blocker of voltage-gated Na+ channels, and is effective in suppressing seizures.
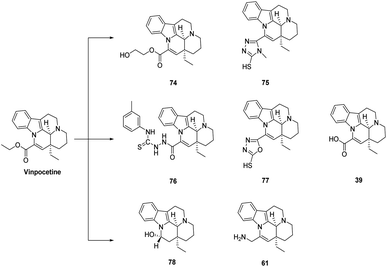 |
| Fig. 11 Change of the 14-ester group. | |
Therefore, Le devised a synthetic route for the introduction of an amine group at the 14-position of perphenazine, using apo-perphenazine as a raw material, reducing it to an intermediate, and then reacting it with phthalimide in the Mitsunobu reaction and then with hydrazine hydrate in ethanol solution to hydrazinolate it to give compound 61.58
Wang59 designed and synthesized a series of Vinpocetine derivatives from Vinpocetine and evaluated them as type 2 diabetic pancreatic β-cell protectors. Firstly, commercially available Vinpocetine was subjected to a dehydration reaction in the presence of toluenesulfonic acid (TsOH) to give apovinamine, which was further hydrolysed to give compound 39, which showed a stronger inhibition of apoptosis in INS-1 cells compared to the Vinpocetine analogues. Esterification with different alcohols under concentrated sulfuric acid conditions afforded a series of compounds 80–81, which exhibited similar potency to compound 39. The resulting series of compounds were then activated with p-toluenesulfonyl chloride (TsCl) and coupled with different amines in the presence of K2CO3 to give the target compounds 82–85 in higher yields. Compound 82, when the introduced group was smaller, had higher compared to the Vinpocetines the activity of compound 83 was similar to that of the Vinpocetines when larger groups were introduced, but compound 85 did not show any improvement in activity when hydroxyl groups were introduced at the end. Alternatively, treatment of Vinpocetine with lithium aluminium hydride (LiAlH4) gave the corresponding product compound 58, with strong apoptosis-inhibiting activity, which was then reacted with different acyl chlorides to give compounds 86–88, which showed a significant improvement in activity compared to Vinpocetine. Activation of the compounds with 4-nitrophenyl carbonate followed by coupling with different amines gave compounds 89. Amine groups can also be introduced directly at the 14-ester site, for example by reacting compound 58 with benzoyls under the conditions of diisopropyl azodicarboxylate (DIAD) and triphenylphosphine (PPh3) to give compound 61 as shown in Fig. 16. Acetylation of compound 61 gave compounds 90–93. The amine derivatives 91 and 92 containing methyl or ethyl groups were both more active than their parent compounds 61, propyl and benzyl substituted compounds 92 and 93, and lost their pancreatic β-cell protective activity. The introduction of the amine group allowed for a variety of reactions, for example with different acyl chlorides to give the corresponding products compounds 94–96, with all the amine derivatives showing improved activity compared to the Vinpocetine except for the aryl-substituted product compound 97, which was reacted with 4-nitrobenzyl carbamate followed by substitution with different amines to give compound 97 (Fig. 12).
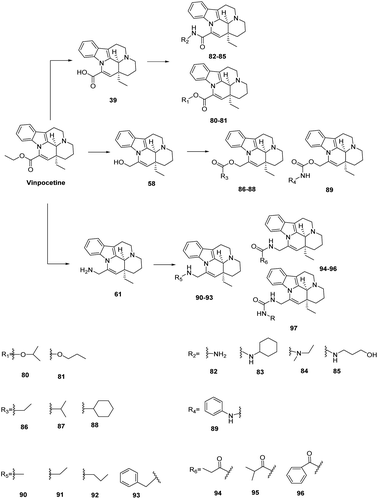 |
| Fig. 12 Introduction of different groups on the 14-position ester group of Vinpocetine. | |
4.4 16-Bit ethyl change
The presence of the ethyl group at the 16 position also plays a crucial role in the activity of Vinpocetine. Incze60 synthesize a Vinpocetine derivative (compound 98) substituted with 2,2,2-trifluoroethyl at the 16 position and found that the trifluoroethyl substitution deprived Vinpocetine of all its original biological activity, thus showing that the presence of the ethyl group at the 16 position is crucial for its activity (Fig. 13).
 |
| Fig. 13 Introduction of trifluoromethyl on the 16-position ethyl group of Vinpocetine. | |
4.5 Effect of different configurations of Vinpocetine on its activity
The basic backbone of Vinpocetine has two chiral center (3 and 16 positions) and it was found that the trans structures (3S, 16R) (compound 99) and (3R, 16S) (compound 100) of Vinpocetine had stronger LPO inhibitory activity than the cis (3S, 16S) Vinpocetine and had a stronger vasodilatory effect (Fig. 14). And 2′-hydroxyethyl trans-Vinpocetine (compound 101) was considered to be the most potent compound in terms of LPO inhibitory activity. Sheng50 designed and synthesized a series of novel alkylamino-substituted cis- and trans-Vinpocetine derivatives using Vinpocetine as a raw material, but the specific activity still needs further investigation. Nemes47 tested a series of Vinpocetine trans-structured derivatives for their antioxidant and antiamnesic effects, using enzyme-catalyzed lipid peroxidation (NADPH-induced LPO) and non-enzymatic the antioxidant effects of the trans-structural derivatives of Vinpocetine were measured in the whole rat brain using enzyme-catalyzed lipid peroxidation (NADPH-induced LPO) and non-enzymatic lipid peroxidation (Fe2+-stimulated LPO). The in vivo effectiveness of the trans-structural derivatives of Vinpocetine on learning function was measured by passive avoidance experiments and a water maze learning task. It was found that those with the trans structure (trans-3R, 16S) showed the most significant antiamnesic activity. It also showed strong inhibitory activity against NADH induced lipid peroxidation in the brain with an IC50 of 7 μM and against Fe2+ induced lipid peroxidation with an IC50 of 13.7 μM, which was 10 times more active than its cis-isomer, suggesting that the trans backbone of the compound facilitates its interaction with liposomes on the cell membrane.
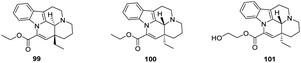 |
| Fig. 14 Vinpocetine derivatives in different configurations. | |
4.6 Changing the C-ring of Vinpocetine
Norwood61 designed a series of derivatives from structurally simple and inexpensive analogues of perphenazine by cleavage transformation of the C ring and by ring distortion through both ring distortion and ring fusion to find new ideas for the design of perphenazine derivatives. As shown in Fig. 15, a series of chemical selective ring cracking, ring rearrangement and ring fusion reactions were used to obtain a series of compounds using Vinpocetine analogue Vinblastine as raw material. Using this ring aberration approach, a complex and diverse library of 80 indole-based small molecules was synthesized and a subpopulation of the library was evaluated against 168 G protein-coupled receptor (GPCR) drug targets. The 168 G protein-coupled receptor (GPCR) drug target was screened by cell-based assays and compound 104 was found to have good selectivity for HCRTR2 in the GPCR panel with good acidic stability. The efficacy of compound 104 was also explored through a morphine-seeking mouse model, which further showed that the in vivo efficacy effects of compound 104 were due to HCRTR2 antagonism, and these data suggest that ring distortions can be used to redesign the bioactivity of perphenazine. Molecular modelling was used to determine the binding mode of compound 104 at the HCRTR2 antagonist binding site, which can be used to develop improved HCRTR2 antagonists using compound 104 as a template for the rational design of new drugs for the treatment of opioid abuse.
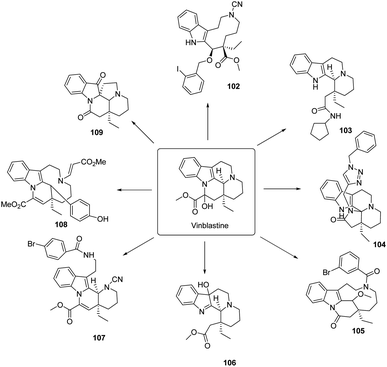 |
| Fig. 15 Change of the 16-bit ethyl group. | |
5. Conclusions
Since its introduction in 1978, Vinpocetine has been used for the treatment of ischaemic cerebrovascular disease. However, due to the hepatic first-pass effect of Vinpocetine, which leads to its reduced bioavailability, and the short half-life of Vinpocetine after oral administration, there are problems such as poor patient compliance after multiple doses, etc. The clinical limitations of Vinpocetine have been highlighted in recent years, so there is an urgent need for in-depth and extensive secondary development. In the past decades, the chemical synthesis of Vinpocetine and its derivatives has been improved, but most of them are only chemically synthesized. In recent years, new activities of Vinpocetine have been discovered, such as the ability to improve mental activity and memory in healthy individuals, and its effectiveness in confusion, inattention, irritability, dizziness, visual and auditory abnormalities, and mood swings. Furthermore, research into the activity of Vinpocetine derivatives is increasingly becoming a focus of interest. For example, Vinpocetine derivatives have been shown to be promising for the treatment of epilepsy. It is also known as one of the “top 10 anti-ageing drugs” and it is believed that the medicinal potential of perphenazine will be explored as more research is carried out. From the synthesis of perphenazine derivatives reported nowadays, most of them are centered around the A ring, D ring, E ring, ethyl group at 16 position, 14-position ester group, and the modification of the C ring, and the results are both good and bad, e.g., the trifluoroethyl substitution almost loses all the original biological activities of perphenazine. This indicates that the presence of the ethyl group at position 16 is essential for its activity and should be retained. It can give us new insights in the further development and utilization of Vinpocetine (Fig. 16).
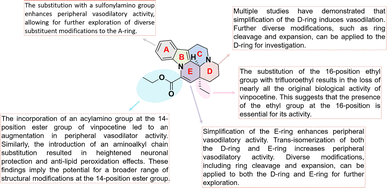 |
| Fig. 16 Constitutive relationship of Vinpocetine. | |
Conflicts of interest
There are no conflicts to declare.
Acknowledgements
This research was funded by the Guizhou Provincial Basic Research Program (Natural Science) (ZK[2021] general 560 and ZK[2023] general 404), the Youth Science and Technology Talent Growth Project of Guizhou Province (Qian Jiao He KY Zi[2022]253), the Project of Guizhou Provincial Health Commission (gzwkj2022-232 and gzwkj2022-467), 2023 Natural Science Research Project of Guizhou Provincial Department of Education (Qian Jiao Ji[2023]69) and Key Disciplines of Traditional Chinese Medicine and Ethnic Medicine in Guizhou Province during the 14th Five-Year Plan (QZYYZDXK(JS)-2021-03).
Notes and references
- T. E. Fandy, I. Abdallah and M. Khayat, Cancer Chemother. Pharmacol., 2016, 77, 259–267 CrossRef CAS.
- L. Zhang and L. Yang, Molecules, 2014, 20, 335–347 CrossRef PubMed.
- A. Dubey, N. Kumar and A. Mishra, Int. J. Pharm. Sci., 2020, 11, 6590–6597 CAS.
- Y. S. Zhang, J. D. Li and C. Yan, Eur. J. Pharmacol., 2018, 819, 30–34 CrossRef CAS PubMed.
- M. K. Jha, M. H. Rahman and H. Sheikh, Int. J. Pharm. Sci. Res., 2012, 3, 346–352 CAS.
- M. Shekarian, A. Komaki and S. Shahidi, Behav. Brain Res., 2020, 383, 112512 CrossRef CAS.
- L. Nivison-Smith, B. J. Brien and M. Truong, Am. J. Physiol.: Cell Physiol., 2015, 308, 737–749 CrossRef.
- M. Zhao, S. Hou and L. Feng, Front. Neurosci., 2020, 14, 223 CrossRef PubMed.
- M. Shekarian, I. Salehi and S. Raoufi, Front. Neurosci., 2023, 24, 20 CAS.
- J. Z. Ni, J. Health Psychol., 2006, 5, 548–549 Search PubMed.
- L. L. Dong, L. J. Jiang and Y. Liu, Pharmaceutical, 2016, 35, 1059–1063 Search PubMed.
- M. H. Wu, Y. G. Jin and Y. Y. Zhang, J. Integr. Med. Cardiovasc. Dis., 2020, 8, 37–63 Search PubMed.
- E. Bagoly, G. Fehér and L. Szapáry, Orv. Hetil., 2007, 148, 1353–1358 Search PubMed.
- G. Svab, J. Doczi and A. A. Gerencser, Neurochem. Res., 2019, 44, 2435–2447 CrossRef CAS PubMed.
- K. Bagri and R. Deshmukh, Inflammopharmacology, 2022, 30, 2243–2259 CrossRef CAS PubMed.
- D. Han, J. Wang and L. Wen, J. Cardiovasc. Pharmacol., 2021, 77, 208 CrossRef CAS PubMed.
- B. Horvath, Z. Marton and R. Halmosi, Clin. Neuropharmacol., 2002, 25, 37–42 CrossRef CAS PubMed.
- M. S. Santos, A. I. Duarte and P. I. Moreira, Free Radical Res., 2000, 32, 57–66 CrossRef CAS PubMed.
- A. E. Medina, Proc. Natl. Acad. Sci. U. S. A., 2010, 107, 9921–9922 CrossRef CAS.
- Z. Ping, W. Xiaomu and X. Xufang, Neurol. Sci., 2019, 40, 113–120 CrossRef PubMed.
- F. Zhang, C. Yan and C. Wei, Transl. Stroke Res., 2018, 9, 174–184 CrossRef CAS PubMed.
- B. B. Colombo, V. Fattori and T. H. Zaninelli, Inflammation, 2018, 41, 1276–1289 CrossRef CAS.
- W. Y. Abdelzaher, S. M. Ahmed and N. N. Welson, Biomed. Pharmacother., 2021, 133, 110976 CrossRef CAS.
- Alsuntangled Group, Amyotrophic Lateral Scler. Frontotemporal Degener., 2021, 22, 316–319 CrossRef.
- Y. Y. Ma, L. Sun and X. J. Chen, PLoS One, 2016, 11, e0162295 CrossRef.
- S. Moini, M. Rahimifard and M. Baeeri, Drug Res., 2021, 71, 438–447 CrossRef.
- H. M. Al-Kuraishy, A. I. Al-Gareeb and M. S. Fageyinbo, Future Sci. OA, 2022, 797 CrossRef PubMed.
- K. J. Meador, B. Leeman-Markowski and A. E. Medina, Epilepsy Behav., 2021, 119, 107988 CrossRef.
- H. M. Aldawsari, S. M. Badr-Eldin and N. Y. Assiri, Drug Delivery, 2022, 29, 2671–2684 CrossRef CAS PubMed.
- R. Wang and Y. Xu, Artif. Cells Nanomed. Biotechnol., 2017, 45, 157–162 CrossRef CAS PubMed.
- Y. Abreu, A. C. Carvalho and G. Skinner, Neurotoxicology, 2018, 66, 150–159 CrossRef.
- Z. Y. Zhang, S. M. Dong and Y. H. Liu, Anticancer Drugs, 2021, 32, 727–733 CrossRef CAS PubMed.
- M. A. Ansari, A. Iqubal and R. Ekbba, Biomed. Pharmacother., 2019, 109, 1372–1380 CrossRef CAS.
- J. C. Wang, J. Clin. Neurosci., 2014, 21, 661–663 CrossRef CAS PubMed.
- K. Ishihara, H. Katsuki and M. Sugimura, Neuropharmacology, 1989, 28, 569–573 CrossRef CAS PubMed.
- P. Zhang, Y. Cao and S. Chen, Pharmacology, 2021, 106, 37–44 CrossRef CAS PubMed.
- F. Hu, H. Nie and R. Xu, Brain Res., 2022, 1792, 148032 CrossRef CAS PubMed.
- S. Park, N. R. Moon and S. Kang, J. Funct. Foods, 2022, 95, 105180 CrossRef CAS.
- C. Qin, D. Y. Ma and S. Y. Liu, Chemical Technology and Development, 2013, vol. 42, pp. 9–11 Search PubMed.
- J. C. F. Alves, A. B. C. Simas and P. R. R. Costa, Asymmetry, 1997, 8, 1963–1966 CrossRef CAS.
- J. C. Alves, A. B. Simas and P. R. Costa, Tetrahedron: Asymmetry, 1999, 10, 297–306 CrossRef CAS.
- A. Nemes, L. Czibula and G. Visky, Heterocycles, 1991, 2329 CrossRef CAS.
- I. Fitos, J. Visy and M. Simony, Biochem. Pharmacol., 1991, 41, 377–383 CrossRef CAS PubMed.
- I. Moldvai, E. Temesvari-Major and C. Szantay, Arch. Pharm., 1997, 330, 190–198 CrossRef CAS PubMed.
- T. Katsuragi, M. Ohba and R. Mori, Gen. Pharmacol., 1984, 15, 43–45 CrossRef CAS PubMed.
- J. Y. Xu, Biochemicals Formulations, 1985, vol. 3, pp. 147–151 Search PubMed.
- A. Nemes, L. Czibula and C. J. Szántay, J. Med. Chem., 2008, 51, 479–486 CrossRef CAS.
- I. Moldvai, T. Gati and C. J. Szantay, J. Org. Chem., 2006, 71, 3768–3772 CrossRef CAS.
- T. Araki, H. Kato and K. Shuto, Eur. J. Pharmacol., 1996, 2, 343–349 CAS.
- H. M. Sheng, Z. J. Xiang and Y. E. Jun-Ting, Chin. J. Nat. Med., 2011, 1, 51–57 Search PubMed.
- Y. Kawashima, T. Ikemoto and A. Horiguchi, J. Med. Chem., 1993, 36, 815–819 CrossRef CAS PubMed.
- A. Vas and B. Gulyás, Med. Res. Rev., 2005, 25, 737–775 CrossRef CAS.
- S. Nag, R. Krasikova and A. J. Airaksinen, Bioorg. Med. Chem. Lett., 2019, 29, 2270–2274 CrossRef CAS.
- B. W. Pan, Y. Shi and C. W. Li, Bioorg. Med. Chem. Lett., 2020, 30, 126472 CrossRef CAS PubMed.
- K. Mazák, J. Vámos and A. Nemes, J. Chromatogr., 2003, 996, 195–203 CrossRef.
- P. Pudleiner and L. Vereczkey, Eur. J. Drug Metab. Pharmacokinet., 1993, 18, 317–321 CrossRef CAS PubMed.
- S. L. Erdõ, P. Molnár and V. Lakics, Eur. J. Pharmacol., 1996, 314, 69–73 CrossRef PubMed.
- G. Lewin and C. Schaeffer, Tetrahedron Lett., 1998, 39, 9689–9692 CrossRef CAS.
- J. Wang, X. Lv and J. Xu, Eur. J. Med. Chem., 2020, 188, 111976 CrossRef CAS.
- M. Incze, F. Soti and Z. Kardos-Balogh, Arch. Pharm., 1990, 323, 331–335 CrossRef CAS.
- V. M. Norwood and A. C. Brice-Tutt, J. Med. Chem., 2020, 63, 5119–5138 CrossRef PubMed.
Footnote |
† These authors contributed equally to this work. |
|
This journal is © The Royal Society of Chemistry 2024 |