DOI:
10.1039/D3QO01575K
(Research Article)
Org. Chem. Front., 2024,
11, 135-141
Photoredox-catalyzed difunctionalization of alkenes with oxime esters and NH-sulfoximines†
Received
26th September 2023
, Accepted 7th November 2023
First published on 21st November 2023
Abstract
A visible-light-induced one-pot three-component reaction of cycloketone oxime esters, NH-sulfoximines, and alkenes was developed for the difunctionalization of alkenes. As a result, a series of versatile sulfoximido-cyanoalkylative products were synthesized under mild and sustainable reaction conditions (up to 98% yield). The significance of this protocol was highlighted by excellent regioselectivity, broad substrate scope, and excellent synthetic applications.
Sulfoximines have attracted tremendous attention due to their special structural unit that has widely existed in medicinal chemistry, agricultural science, and organic synthesis in the past few decades.1 As illustrated in Fig. 1, various bioactive properties were reported in representative compounds with sulfoximine moieties, including an anti-cancer agent, HIV-1 protease inhibitor, anti-inflammatory compound, insecticide, etc.2 Additionally, sulfoximines have been extensively used as chiral ligands, organic catalysts, and key intermediates for the construction of heterocyclic compounds.3 Considering their great significance, the exploration of mild and sustainable methodologies for sulfoximidation is important.
 |
| Fig. 1 Representative examples of compounds containing sulfoximines. | |
The one-pot multiple-component reaction has emerged as a powerful strategy for the construction of highly functionalized target molecules due to its convenience and high step economy.4 Among the one-pot multiple-component reactions, the difunctionalization of alkenes, installing two functional groups across a double bond, has been regarded as an effective and powerful synthetic method that constructs molecular diversity and complexity.5 In the difunctionalization of alkenes, β-selective sulfoximidation of alkenes has been well developed via the sulfoximinyl radical process under photocatalysis or electrocatalysis (Scheme 1a).6 However, examples of α-sulfoximidation in the difunctionalization of alkenes via a photocatalytic multicomponent radical cascade reaction are still scarce. In 2021, Zeng and colleagues reported a visible-light-promoted sulfoximido-chalcogenization process of alkenes using K2S2O8 as a radical initiator and tetrabutylammonium iodide (TBAI) as a catalyst, in which the phenylselenyl radical is a key intermediate initiating the radical addition process (Scheme 1b).7 However, such a difunctionalization procedure initiated by a carbon-centered radical is currently unknown.
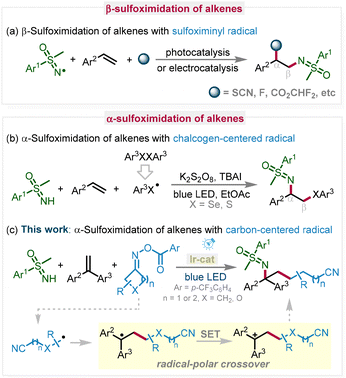 |
| Scheme 1 Difunctionalization of alkenes involving sulfoximines. | |
On the other hand, cyanoalkyl moieties play a significant and pivotal role in organic synthesis, pharmaceutical chemistry, and bioactive molecules.8 Meanwhile, cyanoalkyl compounds, as versatile and general synthons in organic synthetic chemistry, could be transformed into various important compounds, such as amides, carboxylic acids, esters, etc.9 Recently, cycloketone oxime esters have been considered effective cyanoalkylating reagents, which undergo N–O and β-C–C cleavage to furnish the corresponding cyanoalkylating compounds under visible-light irradiation,10 with significant contribution reported by the groups of Chen and Xiao,11 Wu,12 Studer,13 Leonori,14 and others.15 Given our ongoing research interest in photochemistry,16 we have herein developed a photoredox-catalyzed method for cyanoalkylsulfoximidation of alkenes using cycloketone oxime esters as cyanoalkylating reagents and NH-sulfoximines as sulfoximidating reagents via radical–polar crossover (Scheme 1c). Notably, this green photocatalytic strategy without any additives features excellent regioselectivity, broad substrate scope, target products with versatile functional transformation, and good scale-up potential.
Results and discussion
Initially, the model reaction of 1,1-diphenylethylene 1a and cyclobutanone oxime ester 2a with NH-sulfoximine 3a was carried out under blue LED irradiation (Table 1). Fortunately, the desired product 4a was obtained in 67% yield using Ir(dFppy)2(bpy)PF6 as a photocatalyst and dichloromethane (DCM) as a solvent (entry 1). Then, various photocatalysts, including Ir(dFppy)3, Ir(p-Fppy)2(bpy)PF6, fac-Ir(ppy)3, eosin Y, eosin B, and Rose Bengal, were evaluated. The results suggested that the best photocatalyst was Ir(p-Fppy)2(bpy)PF6, leading to the formation of 4a in 75% yield (entries 2–7). However, a series of solvents including 1,2-dichloroethane (DCE), tetrahydrofuran (THF), acetonitrile (CH3CN), dimethyl carbonate (DMC), N,N-dimethylformamide (DMF), and dimethyl sulfoxide (DMSO) did not improve the yield of the target product (entries 8–13). Next, when the reaction time was reduced to 12 h, product 4a was obtained in 77% yield (entries 14 and 15). Furthermore, the yield of 4a was 90% when the ratio of 1a
:
2a
:
3a was 4
:
1
:
4 (entry 16), along with the direct cyanoalkylation of 1a as a byproduct (9% yield). It is assumed that excess alkenes and sulfoximines might inhibit the generation of the byproduct, promoting the yield of the major product 4a (for details, see the ESI†). Control experiments did not generate the desired product 4a in the absence of light or a photocatalyst, indicating that light and a photocatalyst are vital factors for the photoredox transformation (entries 17 and 18). Moreover, the reaction failed to perform under air, suggesting that this reaction was sensitive to air. Finally, the optimal reaction conditions were established as follows: 1a (0.4 mmol), 2a (0.1 mmol), 3a (0.4 mmol), Ir(p-Fppy)2(bpy)PF6 (1 mol%), and DCM (1 mL) were stirred under N2 with the irradiation of a 40 W blue LED (456 nm) for 12 h.
Table 1 Optimization of reaction conditionsa
With the optimal reaction conditions in hand, the substrate scope of sulfoximines was explored (Scheme 2). The reactions of NH-sulfoximines bearing electron-donating or electron-withdrawing groups including –Me, –OMe, –F, –Cl, –Br, –COCH3, and –CN with 1a and 2a all proceeded well under the optimal reaction conditions, affording the desired products 4b–4h in good to excellent yields (74%–98%). The results suggested that the reaction was not obviously influenced by the electronic effect of the substituents. Then, meta- and ortho-substituted NH-sulfoximines were also considered as competent substrates to afford the products 4i–4l in 50%–71%. Additionally, when dichloro-substituted NH-sulfoximine was employed to react with 1a and 2a, the target product 4m was obtained in 65% yield. Finally, other aromatic ring substituted NH-sulfoximines, such as naphthalene and pyridine, were compatible with the reaction system, leading to the formation of the corresponding products 4n and4o in 62% and 80% yields, respectively.
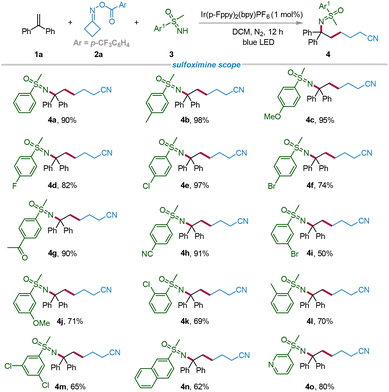 |
| Scheme 2 Substrate scope of sulfoximines. Reaction conditions: 1a (0.4 mmol), 2a (0.1 mmol), 3 (0.4 mmol), and Ir(p-Fppy)2(bpy)PF6 (1 mol%) in DCM (1 mL) with the irradiation of a blue LED under a N2 atmosphere for 12 h. Isolated yields are given. | |
To further investigate the universality of the photoreaction, we next examined the scope of cycloketone oxime esters. As shown in Scheme 3, various cyclobutanone oxime esters bearing different groups (–CO2Me, –CO2Et, –CO2tBu, and –Ph) could be smoothly converted into the corresponding primary cyanoalkyl target products 4p–4s in 52%–80% yields. To our delight, cyclopentanone oxime esters were also suitable for this transformation, delivering the corresponding products 4t and 4u, of which the secondary oxycyanoalkyl product 4t was synthesized in 54% yield and the tertiary cyanoalkyl three-component product 4u was obtained in 46% yield, showing the excellent compatibility of the substrates. Moreover, the scope of alkenes was also surveyed. The target products 4v–4ac were all synthesized via the green and sustainable photochemical strategy in moderate to good yields (52%–85%) from the corresponding symmetric and nonsymmetric 1,1-diarylalkenes. Unfortunately, when styrene and an aliphatic terminal olefin were employed as the substrates, no desired products were obtained (for details, see the ESI†).
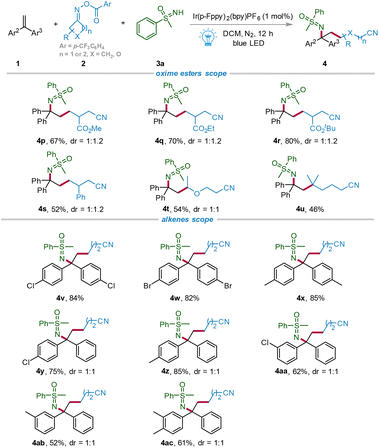 |
| Scheme 3 Substrate scope of oxime esters and alkenes. Reaction conditions: 1 (0.4 mmol), 2 (0.1 mmol), 3a (0.4 mmol), and Ir(p-Fppy)2(bpy)PF6 (1 mol%) in DCM (1 mL) with the irradiation of a blue LED under a N2 atmosphere for 12 h. Isolated yields are given. | |
Subsequently, a gram-scale reaction was performed on a 4 mmol scale under the standard conditions (Scheme 4a). To our delight, the target compound 4a was obtained in 84% isolated yield (1.35 g), indicating that this meaningful protocol has good practical potential. Considering the synthetic versatility of the cyanoalkylsulfoximidation of alkenes, a variety of transformations were further performed (Scheme 4b). For example, the hydrolysis–esterification of 4a using concentrated H2SO4 as a catalyst and ethanol as a solvent under reflux conditions delivered the desired product 5a in 78% yield. The hydrolysis–acidification of 4a with concentrated H2SO4 in acetic acid and water furnished 1,5-enoic acid 5b in 65% yield. To prove the generality of the photocatalyzed protocol, N-methylindole 3ad and indole 3ae could be smoothly applied to the reaction system, delivering the corresponding products 4ad and 4ae in 57% and 48% yields, respectively.
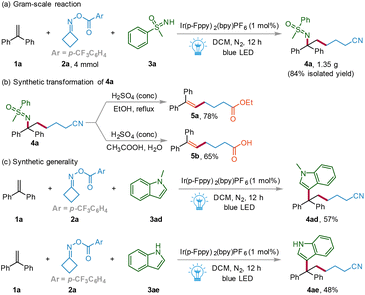 |
| Scheme 4 Synthetic applications. | |
In order to investigate the reproducibility of this sustainable protocol, sensitivity experiments were designed (for details, see the ESI†). It could be intuitively observed from the radar figure that the photoreaction was sensitive to air and tolerated other factors including concentration, water level, photocatalyst level, light intensity, and scale (Fig. 2).
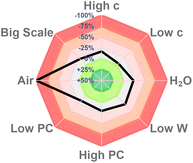 |
| Fig. 2 Sensitivity assessment. | |
Afterward, some fluorescence quenching experiments were conducted. As shown in Fig. 3, a linear relationship between the fluorescence intensity I0/I and concentration of 2a was observed, indicating that a single electron transfer (SET) process between the excited photocatalyst and 2a might take place.
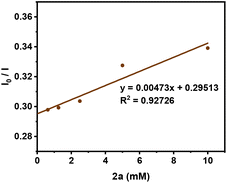 |
| Fig. 3 Fluorescence quenching experiments. | |
To gain deeper insight into the photoreaction mechanism, a series of radical trapping experiments were carried out (Scheme 5). The radical scavenger 2,2,6,6-tetramethylpiperidin-1-yl-oxidanyl (TEMPO) or 2,6-di-tert-butyl-4-methylphenol (BHT) was added into the model reaction which resulted in a dramatic decrease in the yield of the target product, suggesting that the photocatalytic transformation may involve a radical pathway. Furthermore, the radical adducts A and B were detected by high-resolution mass spectroscopy (HRMS), which proved the generation of cyanoalkyl radicals. Surprisingly, the radical adduct C was also detected by HRMS, reminding us that a reaction pathway involving the sulfoximinyl radical was also possible.
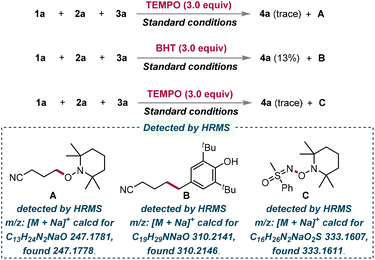 |
| Scheme 5 Radical trapping experiments. | |
The plausible mechanism was proposed based on the above detailed experimental results and previous reports (Scheme 6).7,17 Initially, the ground-state photocatalyst PC was excited to the excited-state PC* under the irradiation of a blue LED. Subsequently, cyclobutanone oxime ester 2a and PC* underwent a SET process to form the radical cation PC˙+ and the unstable N-centered radical 5, which rapidly underwent β-C–C cleavage to afford cyanoalkyl radical 6. The radical 6 was added to 1a to produce intermediate 7. Then, 7 was oxidized by PC˙+ to afford the cationic intermediate 8, which was attacked by nucleophile 3 to furnish the target product 4 (pathway A). Since the sulfoximinyl radical was detected by HRMS, pathway B involving the direct coupling of radical intermediate 7 and the in situ generated sulfoximinyl radical 10 could not be ruled out.
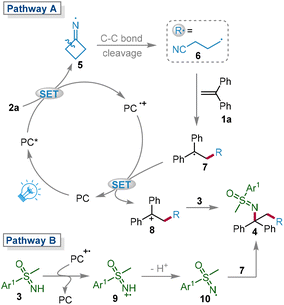 |
| Scheme 6 Proposed mechanism. | |
Conclusions
In conclusion, we have developed a green and sustainable one-pot three-component strategy for the cyanoalkylsulfoximidation of alkenes with cycloketone oxime esters and NH-sulfoximines via radical-polar crossover under irradiation of visible light. Various target products can be synthesized with excellent regioselectivity in moderate to excellent yields under mild conditions. Additionally, the superior synthetic applications of this photocatalytic protocol are reflected in the scalability and the formation of the cyanoalkylsulfoximidative product with versatility, which can be converted into a variety of important organic molecules.
Conflicts of interest
There are no conflicts to declare.
Acknowledgements
We acknowledge the financial support from the National Key R&D Program of China (2021YFB4001100 and 2021YFB4001101), the National Natural Science Foundation of China (21971224, 22071222, and 22171249), and the Science & Technology Innovation Talents in Universities of Henan Province (23HASTIT003).
References
-
(a) V. Bizet, R. Kowalczyk and C. Bolm, Fluorinated sulfoximines: syntheses, properties and applications, Chem. Soc. Rev., 2014, 43, 2426–2438 RSC;
(b) S. Otocka, M. Kwiatkowska, L. Madalińska and P. Kiełbasiński, Chiral Organosulfur Ligands/Catalysts with a Stereogenic Sulfur Atom: Applications in Asymmetric Synthesis, Chem. Rev., 2017, 117, 4147–4181 CrossRef CAS;
(c) G. C. Nandi and P. I. Arvidsson, Sulfonimidamides: Synthesis and Applications in Preparative Organic Chemistry, Adv. Synth. Catal., 2018, 360, 2976–3001 CrossRef CAS;
(d) T. Zhou, P.-F. Qian, J.-Y. Li, Y.-B. Zhou, H.-C. Li, H.-Y. Chen and B.-F. Shi, Efficient Synthesis of Sulfur-Stereogenic Sulfoximines via Ru(II)-Catalyzed Enantioselective C–H Functionalization Enabled by Chiral Carboxylic Acid, J. Am. Chem. Soc., 2021, 143, 6810–6816 CrossRef CAS;
(e) D. Zeng, Y. Ma, W.-P. Deng, M. Wang and X. Jiang, Divergent sulfur(VI) fluoride exchange linkage of sulfonimidoyl fluorides and alkynes, Nat. Synth., 2022, 1, 455–463 CrossRef.
-
(a) U. Lücking, Neglected sulfur(VI) pharmacophores in drug discovery: exploration of novel chemical space by the interplay of drug design and method development, Org. Chem. Front., 2019, 6, 1319–1324 RSC;
(b) F. Liu, H. Wang, S. Li, G. A. L. Bare, X. Chen, C. Wang, J. E. Moses, P. Wu and K. B. Sharpless, Biocompatible SuFEx Click Chemistry: Thionyl Tetrafluoride (SOF4)-Derived Connective Hubs for Bioconjugation to DNA and Proteins, Angew. Chem., Int. Ed., 2019, 58, 8029–8033 CrossRef CAS;
(c) P. Mäder and L. Kattner, Sulfoximines as Rising Stars in Modern Drug Discovery? Current Status and Perspective on an Emerging Functional Group in Medicinal Chemistry, J. Med. Chem., 2020, 63, 14243–14275 CrossRef;
(d) S. Li, G. Li, B. Gao, S. P. Pujari, X. Chen, H. Kim, F. Zhou, L. M. Klivansky, Y. Liu, H. Driss, D.-D. Liang, J. Lu, P. Wu, H. Zuilhof, J. Moses and K. B. Sharpless, SuFExable polymers with helical structures derived from thionyl tetrafluoride, Nat. Chem., 2021, 13, 858–867 CrossRef CAS;
(e) D. Zeng, Y. Ma, W.-P. Deng, M. Wang and X. Jiang, The Linkage of Sulfonimidoyl Fluorides and Unactivated Alkenes via Hydrosulfonimidoylation, Angew. Chem., Int. Ed., 2022, 61, e202207100 CrossRef CAS.
- W. Zheng, X. Chen, F. Chen, Z. He and Q. Zeng, Syntheses and Transformations of Sulfoximines, Chem. Rec., 2021, 21, 396–416 CrossRef CAS.
-
(a) Z. Wang, N. Meng, Y. Lv, W. Wei, H. Yue and G. Zhong, Photocatalyst-free visible-light-mediated three-component reaction of α-diazoesters, cyclic ethers and NaSCN to access organic thiocyanates, Chin. Chem. Lett., 2023, 34, 107599–107602 CrossRef CAS;
(b) Y.-H. Lu, C. Wu, J.-C. Hou, Z.-L. Wu, M.-H. Zhou, X.-J. Huang and W.-M. He, Ferrocene-Mediated Photocatalytic Annulation of N-Sulfonyl Ketimines on a Polycrystalline WSe2 Semiconductor Photocatalyst, ACS Catal., 2023, 13, 13071–13076 CrossRef CAS;
(c) Q. Wang, J. Xu, Z. Xu, Z. Wang, X. Tao, S. Ni, Y. Pan and Y. Wang, Catalyst-free electroreductive carboxylic acid–nitroarene coupling, Green Chem., 2023, 25, 7084–7091 RSC;
(d) Y.-H. Lu, Z.-T. Zhang, H.-Y. Wu, M.-H. Zhou, H.-Y. Song, H.-T. Ji, J. Jiang, J.-Y. Chen and W.-M. He, TBAI/H2O-cooperative electrocatalytic decarboxylation coupling-annulation of quinoxalin-2(1H)-ones with N-arylglycines, Chin. Chem. Lett., 2023, 34, 108036–108040 CrossRef CAS.
-
(a) G. Yin, X. Mu and G. Liu, Palladium(II)-Catalyzed Oxidative Difunctionalization of Alkenes: Bond Forming at a High-Valent Palladium Center, Acc. Chem. Res., 2016, 49, 2413–2423 CrossRef CAS;
(b) H. Jiang and A. Studer, Intermolecular radical carboamination of alkenes, Chem. Soc. Rev., 2020, 49, 1790–1811 RSC;
(c) Z.-L. Li, G.-C. Fang, Q.-S. Gu and X.-Y. Liu, Recent advances in copper-catalysed radical-involved asymmetric 1,2-difunctionalization of alkenes, Chem. Soc. Rev., 2020, 49, 32–48 RSC;
(d) D.-K. Wang, L. Li, Q. Xu, J. Zhang, H. Zheng and W.-T. Wei, 1,3-Difunctionalization of alkenes: state-of-the-art and future challenges, Org. Chem. Front., 2021, 8, 7037–7049 RSC.
-
(a) C. Wang, Y. Tu, D. Ma and C. Bolm, Photocatalytic Fluoro Sulfoximidations of Styrenes, Angew. Chem., Int. Ed., 2020, 59, 14134–14137 CrossRef CAS;
(b) H. Wang, M. Frings and C. Bolm, Halocyclizations of Unsaturated Sulfoximines, Org. Lett., 2016, 18, 2431–2434 CrossRef CAS;
(c) H. Wang, D. Zhang and C. Bolm, Photocatalytic Additions of 1-Sulfoximidoyl-1,2-Benziodoxoles to Styrenes, Chem. – Eur. J., 2018, 24, 14942–14945 CrossRef CAS;
(d) D. Zhang, H. Wang and C. Bolm, Photocatalytic difunctionalisations of alkenes with N-SCN sulfoximines, Chem. Commun., 2018, 54, 5772–5775 RSC;
(e) C. Wang, Y. Tu, D. Ma, C. Ait Tarint and C. Bolm, Photocatalytic Synthesis of Difluoroacetoxy-containing Sulfoximines, Org. Lett., 2021, 23, 6891–6894 CrossRef CAS;
(f) J.-L. Wan and J.-M. Huang, Electrochemically Enabled Sulfoximido-Oxygenation of Alkenes with NH-Sulfoximines and Alcohols, Org. Lett., 2022, 24, 8914–8919 CrossRef CAS.
- H. Chen, L. Chen, Z. He and Q. Zeng, Blue light-promoted radical sulfoximido-chalcogenization of aliphatic and aromatic alkenes, Green Chem., 2021, 23, 2624–2627 RSC.
-
(a) F. F. Fleming, Nitrile-containing natural products, Nat. Prod. Rep., 1999, 16, 597–606 RSC;
(b) F. F. Fleming, L. Yao, P. C. Ravikumar, L. Funk and B. C. Shook, Nitrile-Containing Pharmaceuticals: Efficacious Roles of the Nitrile Pharmacophore, J. Med. Chem., 2010, 53, 7902–7917 CrossRef CAS;
(c) R. López and C. Palomo, Cyanoalkylation: Alkylnitriles in Catalytic C-C Bond-Forming Reactions, Angew. Chem., Int. Ed., 2015, 54, 13170–13184 CrossRef.
- F. F. Fleming and Q. Wang, Unsaturated Nitriles: Conjugate Additions of Carbon Nucleophiles to a Recalcitrant Class of Acceptors, Chem. Rev., 2003, 103, 2035–2078 CrossRef CAS.
-
(a) X.-Y. Yu, J.-R. Chen and W.-J. Xiao, Visible Light-Driven Radical-Mediated C–C Bond Cleavage/Functionalization in Organic Synthesis, Chem. Rev., 2021, 121, 506–561 CrossRef CAS;
(b) W. Xiao and J. Wu, Recent advances for the photoinduced CC bond cleavage of cycloketone oximes, Chin. Chem. Lett., 2020, 31, 3083–3094 CrossRef CAS;
(c) F. Xiao, Y. Guo and Y.-F. Zeng, Recent Developments in Radical Cross-Coupling of Redox-Active Cycloketone Oximes, Adv. Synth. Catal., 2021, 363, 120–143 CrossRef CAS;
(d) X.-Y. Yu, Q.-Q. Zhao, J. Chen, W.-J. Xiao and J.-R. Chen, When Light Meets Nitrogen-Centered Radicals: From Reagents to Catalysts, Acc. Chem. Res., 2020, 53, 1066–1083 CrossRef CAS;
(e) X. Zhu and H. Fu, Photocatalytic cross-couplings via the cleavage of N–O bonds, Chem. Commun., 2021, 57, 9656–9671 RSC;
(f) P. Chen, H. Huang, Q. Tan, X. Ji and F. Zhao, Recent Advances in Molecule Synthesis Involving
C-C Bond Cleavage of Ketoxime Esters, Molecules, 2023, 28, 2667–2699 CrossRef CAS;
(g) Z. Qu, T. Tian, G.-J. Deng and H. Huang, Diverse catalytic systems for nitrogen-heterocycle formation from O-acyl ketoximes, Chin. Chem. Lett., 2023, 34, 107565–107574 CrossRef CAS.
-
(a) X.-Y. Yu, J.-R. Chen, P.-Z. Wang, M.-N. Yang, D. Liang and W.-J. Xiao, A Visible-Light-Driven Iminyl Radical-Mediated C–C Single Bond Cleavage/Radical Addition Cascade of Oxime Esters, Angew. Chem., Int. Ed., 2018, 57, 738–743 CrossRef CAS;
(b) B. Lu, Y. Cheng, L.-Y. Chen, J.-R. Chen and W.-J. Xiao, Photoinduced Copper-Catalyzed Radical Aminocarbonylation of Cycloketone Oxime Esters, ACS Catal., 2019, 9, 8159–8164 CrossRef CAS;
(c) P.-Z. Wang, B.-Q. He, Y. Cheng, J.-R. Chen and W.-J. Xiao, Radical C–C Bond Cleavage/Addition Cascade of Benzyl Cycloketone Oxime Ethers Enabled by Photogenerated Cyclic Iminyl Radicals, Org. Lett., 2019, 21, 6924–6929 CrossRef CAS;
(d) J. Chen, Y.-J. Liang, P.-Z. Wang, G.-Q. Li, B. Zhang, H. Qian, X.-D. Huan, W. Guan, W.-J. Xiao and J.-R. Chen, Photoinduced Copper-Catalyzed Asymmetric C–O Cross-Coupling, J. Am. Chem. Soc., 2021, 143, 13382–13392 CrossRef CAS;
(e) P.-Z. Wang, Y.-J. Liang, X. Wu, W. Guan, W.-J. Xiao and J.-R. Chen, Copper-Catalyzed Three-Component Photo-ATRA-Type Reaction for Asymmetric Intermolecular C–O Coupling, ACS Catal., 2022, 12, 10925–10937 CrossRef CAS;
(f) G.-Q. Li, F.-R. Meng, W.-J. Xiao and J.-R. Chen, Photoinduced copper-catalyzed asymmetric radical three-component cross-coupling of 1,3-enynes with oxime esters and carboxylic acids, Org. Chem. Front., 2023, 10, 2773–2781 RSC.
-
(a) J. Zhang, X. Li, W. Xie, S. Ye and J. Wu, Photoredox-Catalyzed Sulfonylation of O-Acyl Oximes via Iminyl Radicals with the Insertion of Sulfur Dioxide, Org. Lett., 2019, 21, 4950–4954 CrossRef CAS;
(b) Y. Li, R. Mao and J. Wu, N-Radical Initiated Aminosulfonylation of Unactivated C(sp3)–H Bond through Insertion of Sulfur Dioxide, Org. Lett., 2017, 19, 4472–4475 CrossRef CAS;
(c) J.-Q. Chen, N. Liu, Q. Hu, J. Liu, J. Wu, Q. Cai and J. Wu, Photocatalytic three-component radical cascade: a general route to heterocyclic-substituted alkyl sulfones, Org. Chem. Front., 2021, 8, 5316–5321 RSC.
- H. Jiang and A. Studer, Iminyl-Radicals by Oxidation of α-Imino-oxy Acids: Photoredox-Neutral Alkene Carboimination for the Synthesis of Pyrrolines, Angew. Chem., Int. Ed., 2017, 56, 12273–12276 CrossRef CAS.
-
(a) J. Davies, S. G. Booth, S. Essafi, R. A. W. Dryfe and D. Leonori, Visible-Light-Mediated Generation of Nitrogen-Centered Radicals: Metal-Free Hydroimination and Iminohydroxylation Cyclization Reactions, Angew. Chem., Int. Ed., 2015, 54, 14017–14021 CrossRef CAS;
(b) E. M. Dauncey, S. P. Morcillo, J. J. Douglas, N. S. Sheikh and D. Leonori, Photoinduced Remote Functionalisations by Iminyl Radical Promoted C–C and C–H Bond Cleavage Cascades, Angew. Chem., Int. Ed., 2018, 57, 744–748 CrossRef CAS.
-
(a) X. Shen, J.-J. Zhao and S. Yu, Photoredox-Catalyzed Intermolecular Remote C–H and C–C Vinylation via Iminyl Radicals, Org. Lett., 2018, 20, 5523–5527 CrossRef CAS;
(b) B. Zhao, C. Chen, J. Lv, Z. Li, Y. Yuan and Z. Shi, Photoinduced fragmentation-rearrangement sequence of cycloketoxime esters, Org. Chem. Front., 2018, 5, 2719–2722 RSC;
(c) B. Zhao, H. Tan, C. Chen, N. Jiao and Z. Shi, Photoinduced C—C Bond Cleavage and Oxidation of Cycloketoxime Esters, Chin. J. Chem., 2018, 36, 995–999 CrossRef CAS;
(d) P.-J. Xia, Z.-P. Ye, Y.-Z. Hu, D. Song, H.-Y. Xiang, X.-Q. Chen and H. Yang, Photocatalytic, Phosphoranyl Radical-Mediated N–O Cleavage of Strained Cycloketone Oximes, Org. Lett., 2019, 21, 2658–2662 CrossRef CAS;
(e) W. Zhang, Y.-L. Pan, C. Yang, L. Chen, X. Li and J.-P. Cheng, Metal-Free Direct C–H Cyanoalkylation of Quinoxalin-2(1H)-Ones by Organic Photoredox Catalysis, J. Org. Chem., 2019, 84, 7786–7795 CrossRef CAS;
(f) W. Zhang, Y.-L. Pan, C. Yang, X. Li and B. Wang, Ring-opening C(sp3)–C coupling of cyclobutanone oxime esters for the preparation of cyanoalkyl containing heterocycles enabled by photocatalysis, Org. Chem. Front., 2019, 6, 2765–2770 RSC;
(g) M.-M. Zhang, S.-H. Li, J.-L. Tu, Q.-Q. Min and F. Liu, Metal-free iminyl radical-mediated C–C single bond cleavage/functionalization of redox-active oxime esters, Org. Chem. Front., 2020, 7, 622–627 RSC;
(h) Y. Liu, Q.-L. Wang, Z. Chen, H. Li, B.-Q. Xiong, P.-L. Zhang and K.-W. Tang, Visible-light photoredox-catalyzed dual C–C bond cleavage: synthesis of 2-cyanoalkylsulfonylated 3,4-dihydronaphthalenes through the insertion of sulfur dioxide, Chem. Commun., 2020, 56, 3011–3014 RSC;
(i) T. Guan, J.-Y. Guo, Q.-H. Zhang, X.-W. Xu, X.-Y. Yu, Y. Zhang and K. Zhao, Photoredox-catalyzed regio- & stereoselective C(sp2)–H cyanoalkylation of enamides with cycloketone oximes via selective C–C bond cleavage/radical addition cascade, Green Chem., 2022, 24, 6524–6530 RSC.
-
(a) Y. Ma, F. Gao, W. Xiao, N. Li, S. Li, B. Yu and X. Chen, Two transition-metal-modified Nb/W mixed-addendum polyoxometalates for visible-light-mediated aerobic benzylic C–H oxidations, Chin. Chem. Lett., 2022, 33, 4395–4399 CrossRef CAS;
(b) P. Xiang, K. Sun, S. Wang, X. Chen, L. Qu and B. Yu, Direct benzylation reactions from benzyl halides enabled by transition-metal-free photocatalysis, Chin. Chem. Lett., 2022, 33, 5074–5079 CrossRef CAS;
(c) C.-H. Ma, L. Zhao, X. He, Y.-Q. Jiang and B. Yu, Visible-light-induced direct 3-ethoxycarbonylmethylation of 2-aryl-2H-indazoles in water, Org. Chem. Front., 2022, 9, 1445–1450 RSC;
(d) F. Gao, S. Zhang, Q. Lv and B. Yu, Recent advances in graphene oxide catalyzed organic transformations, Chin. Chem. Lett., 2022, 33, 2354–2362 CrossRef CAS.
- A. Wimmer and B. König, Visible-Light-Mediated Photoredox-Catalyzed N-Arylation of NH-Sulfoximines with Electron-Rich Arenes, Adv. Synth. Catal., 2018, 360, 3277–3285 CrossRef CAS.
|
This journal is © the Partner Organisations 2024 |
Click here to see how this site uses Cookies. View our privacy policy here.