DOI:
10.1039/D3QO01422C
(Research Article)
Org. Chem. Front., 2024,
11, 120-126
Difluorocarbene as a C–F source for the construction of fluorinated benzothiazoles†
Received
1st September 2023
, Accepted 12th November 2023
First published on 13th November 2023
Abstract
Difluorocarbene has served as a versatile intermediate for the incorporation of fluorinated groups into organic molecules. Extensive studies have shown that difluorocarbene can act as a CF2 source and a nonfluorinated C1 source. However, the use of difluorocarbene as a C–F source remains a challenging task. Herein we disclose that difluorocarbene can function as a C–F source for the cyclization of 2-aminobenzenethiols, allowing for the construction of fluorinated benzothiazoles, which may provide new possibilities for drug developments due to the magic effects of the fluorine element and the pharmacological properties of benzothiazoles.
Introduction
Owing to the unique properties of fluorine, including its strong electronegativity and a small atomic radius, the incorporation of a fluorinated group into organic molecules may lead to changes in their physicochemical properties, such as increasing the lipophilicity and membrane permeability of biologically active molecules.1 The high demand for the presence of fluorinated groups in organic molecules has stimulated significant efforts for the development of efficient methods for fluorine incorporation.2
Difluorocarbene, a singlet carbene which is destabilized by the fluorine inductive effect and stabilized by the back-donating effect of fluorine lone pairs, has served as a versatile intermediate for the incorporation of various fluorine-containing groups, especially a –CF2– unit.3 Typical transformations of difluorocarbene include [2 + 1] cycloaddition with alkenes or alkynes,4 X–H difluoromethylation (X = N, O, S, etc.),5 and coupling with other carbenes to give gem-difluoroalkenes6 (Scheme 1a, right side). In these typical transformations, difluorocarbene usually acts as an electrophilic species. Its electrophilicity also allows it to be a bipolar CF2 source, i.e., difluorocarbene can be first attached to a nucleophile to generate a NuCF2− species, which would then readily attack an electrophile to form NuCF2E (Scheme 1a, right side).3b,7 The coordination of difluorocarbene to transition metals may lead to a reversal of the reactivity of the difluorocarbene carbon from electrophilicity to nucleophilicity.3e,8 The coordination model offers new opportunities for difluorocarbene chemistry,9 and has found applications in the synthesis of unique R-CF2-R′ molecules (Scheme 1a, left side).8b,10 In all of the above reactions, difluorocarbene functions as a CF2 source without the loss of any fluorine atom. Recent studies have shown that difluorocarbene can also act as a nonfluorinated C1 precursor of various functional groups, such as carbonyl,8b,11 cyanide12 and isocyanide13 groups (Scheme 1b). The complete loss of the two fluorine atoms is mainly ascribed to the participation of neighboring groups. The outstanding accomplishments in difluorocarbene chemistry have revealed that difluorocarbene can act as both a CF2 source and a nonfluorinated C1 source. However, the use of difluorocarbene as a C–F source remains a challenging task, which was described only by very few reports (Scheme 1c, the first equation).14
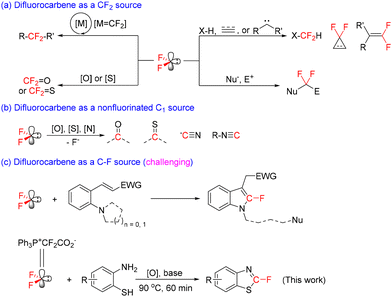 |
| Scheme 1 Transformations of difluorocarbene. | |
We recently developed an efficient difluorocarbene reagent, Ph3P+CF2CO2− (PDFA),15 the use of which led us to make new exciting discoveries in difluorocarbene chemistry3d,12b,16 and which has been widely used by other research groups.7d,17 We found that difluorocarbene can be captured by a suitable oxygen source, a sulfur source and a nitrogen source to in situ generate carbonyl fluoride (CF2
O),16d thiocarbonyl fluoride (CF2
S),16a–c and the cyanide anion (CN−),12 respectively (Scheme 1a, left side). These transformations have been developed as synthetic tools for 18O-trifluoromethoxylation, 18F-trifluoromethylthiolation and cyanodifluoromethylation reactions. In these reactions, difluorocarbene acts as a CF2 source (CF2
O or CF2
S) or a nonfluorinated C1 source (CN−). These interesting findings prompted us to further investigate new synthetic utilities of difluorocarbene, and allowed us to discover the use of difluorocarbene as a C–F source for cyclization of 2-aminobenzenethiols, allowing for the construction of a thiazole unit and the access to fluorinated benzothiazoles (Scheme 1c, the second equation).
Results and discussion
We have previously found that difluorocarbene generated from Ph3P+CF2CO2− can react with elemental sulfur (S8) to produce thiocarbonyl fluoride (CF2
S), an active intermediate which would readily be captured by 2-aminobenzenethiol (1a) to afford a HCF2S-substituted benzothiazole via sequential transformations.16b However, only a very low yield was obtained (31%). In order to find out the reason for the low yield, we decided to determine what the major byproduct was. To our surprise, a fluorinated benzothiazole (3a) was observed (Table 1, entry 1). Apparently, elemental sulfur did not convert difluorocarbene into CF2
S in this process. Instead, elemental sulfur may only act as an oxidant. This rationale encouraged us to examine other reaction solvents (entries 2–4) and other oxidants (entries 5–9). NMP (1-methylpyrrolidin-2-one) was found to be a suitable reaction solvent (entry 4), and besides elemental sulfur (entry 4), both NFSI (N-fluorobenzenesulfonimide) (entry 6) and SeO2 (entry 7) also seemed to be good oxidants. Elemental sulfur was not used for further screening because we were afraid that its side reaction with difluorocarbene to form CF2
S may suppress the desired process. The conversion was not quite sensitive towards the reaction temperature (entries 10–12), and a reaction temperature of 90 °C gave the desired product in 40% yield (entry 11). Increasing the loading of the NFSI oxidant increased the yield significantly (entries 13 and 14). The conversion of 1a into 3a involves deprotonation, and thus various bases were screened (entries 15–18). The use of NaH as a base increased the yield slightly from 52% (entry 13) to 56% (entry 18). The yield was increased further by increasing the loading of NaH (entries 19 and 20). The loading of Ph3P+CF2CO2− was also examined (entries 21 and 22), and a good yield was obtained by using 2.5 equiv. of Ph3P+CF2CO2− (entry 22). Although BrCF2CO2K can easily generate difluorocarbene, the use of BrCF2CO2K instead of Ph3P+CF2CO2− cannot give a good yield (entry 23) (please see the ESI† for the optimization of reaction conditions). The good yield obtained with the use of Ph3P+CF2CO2− as a difluorocarbene reagent is partially because the equilibrium between difluorocarbene and the phosphonium ylide (:CF2 + Ph3P ↔ Ph3P+-CF2−)3d may stabilize difluorocarbene.
Table 1 Optimization of reaction conditions
With the optimal reaction conditions in hand (Table 1, entry 22), we then investigated the substrate scope of the use of difluorocarbene as a C–F source for the cyclization of vicinal aminobenzenethiols. As shown in Scheme 2, the process could be extended to a wide range of 2-aminobenzenethiols. The substrates substituted by an electron-withdrawing group such as a halogen atom or a CF3O unit can be converted smoothly into the desired products. However, the position of the electron-withdrawing group dramatically affects the reaction. No expected product was observed if the 4-position is substituted by a Cl or Br atom (3f and 3g), probably because the electron-withdrawing effect results in lower nucleophilicity of the ortho amino group. For the electron-donating groups, their substitution sites have no significant side effects. The structure of 3y was confirmed by X-ray analysis. The replacement of the SH group with an OH or a NH2 group in the substrate failed to give the desired products.
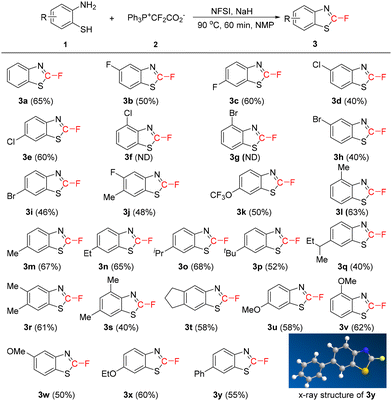 |
| Scheme 2 Substrate scope of the use of difluorocarbene as a C–F source for the cyclization of vicinal aminobenzenethiols. Isolated yields are shown. Reaction conditions: substrate 1 (0.5 mmol), Ph3P+CF2CO2− (2.5 equiv.), NFSI (2 equiv.), NaH (3 equiv.) in NMP (5 mL) under an N2 atmosphere at 90 °C for 60 min. ND = not detected. | |
Benzothiazoles, occurring naturally, are an important group of heterocyclic compounds that exhibit a broad spectrum of pharmacological properties such as antitumor, antimicrobial, antidiabetic, anticonvulsant and anti-inflammatory properties.18 Considering the positive biological effects of the fluorine element, the incorporation of a fluorine atom into benzothiazoles may provide new possibilities for drug development of benzothiazoles. However, the efficient synthesis of 2-fluoro-benzothiazoles remains a significant challenge and only a handful of methods have been reported.19 Furthermore, in most reports, only one example was investigated, and the substrate scope is unknown.19b–e Arisawa and co-workers described the use of ArSC6F5 as a fluorine source to allow for Rh-catalyzed transformations of benzothiazole ethers into 2-fluoro-benzothiazoles.19a The reactions are highly efficient, but the fluorine source may be too expensive since only one fluorine atom in ArSC6F5 is useful. In contrast to these previous reports, our protocol is quite attractive as thiazoles can be easily constructed with the simultaneous incorporation of a fluorine atom.
Since NFSI can act as both an oxidant and an electrophilic fluorination reagent, it is necessary to figure out what role NFSI plays in this reaction and whether the fluorine atom in the product comes from NFSI. As mentioned above, NFSI should be just an oxidant. Indeed, the use of SeO2 (Scheme 3, eqn (1)) or elemental sulfur (Scheme 3, eqn (2)), both of which are nonfluorinated oxidants, instead of NFSI can also give the desired product in moderate to good yields, reflecting that the fluorine atom in the product is not from NFSI. The path that benzothiazole is first formed and then undergoes fluorination with NFSI is excluded based on the evidence that no product 3a was detected for the fluorination of benzothiazole with NFSI irrespective of whether NaH is used or not (Scheme 3, eqn (3) and (4)), further suggesting that NFSI is not a fluorination reagent in this process.
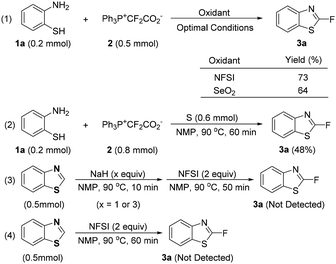 |
| Scheme 3 Exclusion of NFSI as a fluorine source and the exclusion of benzothiazole as a necessary intermediate. All yields are 19F NMR yields. | |
On the basis of the above results, we propose the reaction mechanism as shown in Scheme 4. Deprotonation of the thiol group (pKa of the PhS-H group is 7.2)20 produces the ArS− anion, which attacks difluorocarbene generated from Ph3P+CF2CO2− to give SCF2− intermediate A (path I). SCF2− is an alkyl anion and thus may act as a strong base to deprotonate the neighboring NH2 group to provide intermediate B.5d,21 In this intermediate, the NH− moiety is a strong electron-donating group and may favor the donation of the sulfur lone pair to the antibonding orbital of the C–F bond to deliver intermediate C. The subsequent attack of the nitrogen on the C
S unit furnishes intermediate D, which is oxidized to afford the final product. On the other hand, the amino group in the substrate may also attack difluorocarbene to generate intermediate E (path II). The donation of the nitrogen lone pair results in the formation of intermediate F,3f and the following intramolecular nucleophilic attack also provides intermediate D.
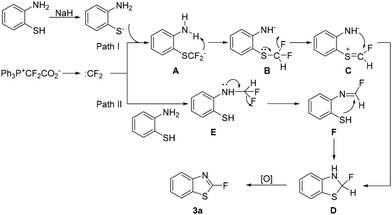 |
| Scheme 4 Plausible reaction mechanism. | |
More evidence was collected to support that D is a key intermediate. It is reasonable to speculate that D may be accumulated without the presence of any oxidant. However, this intermediate may be quite unstable since the donation of the N/S lone pair may easily lead to the elimination of a fluoride ion to produce benzothiazole (5a). Indeed, we couldn't isolate this intermediate at all, and benzothiazole (5a) was observed (Scheme 5, eqn (1)). Under these conditions, product 3a was not produced, further supporting the proposed mechanism. Besides, the S–H difluoromethylation product (4a) was also generated, supporting path I shown in Scheme 4. By the way, no N-CF2H product was isolated. However, path II shown in Scheme 4 is still plausible. The unsuccessful isolation should be because the N-CF2H group is highly unstable. Since it is hard to isolate intermediate D, we then performed a reaction under optimal conditions except that 20 min of reaction time instead of 60 min was used (Scheme 5, eqn (2)). Fortunately, intermediate D was detected by HRMS (EI).
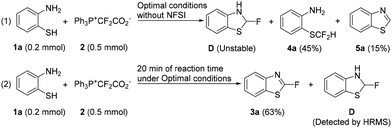 |
| Scheme 5 Evidence to support intermediate D. NMR yields are shown. | |
Conclusions
In summary, we have described the use of difluorocarbene as a C–F source for the cyclization of vicinal aminobenzenethiols to deliver fluorinated 2-fluoro-benzothiazoles. This work represents an exciting discovery of difluorocarbene chemistry. The protocol allows for the easy construction of a thiazole unit and the simultaneous incorporation of a fluorine atom. The facile access to 2-fluoro-benzothiazoles may provide more opportunities for drug development of benzothiazole derivatives.
Conflicts of interest
There are no conflicts to declare.
Acknowledgements
The authors thank the National Key Research and Development Program of China (2021YFF0701700), the National Natural Science Foundation of China (21971252, 21991122, 22271181), and the Science and Technology Commission of Shanghai Municipality (22ZR1423600) for financial support.
References
-
(a) S. Purser, P. R. Moore, S. Swallow and V. Gouverneur, Fluorine in medicinal chemistry, Chem. Soc. Rev., 2008, 37, 320–330 RSC;
(b)
P. Kirsch, Modern Fluoroorganic Chemistry: Synthesis, Reactivity, Applications, Wiley-VCH, Weinheim, Germany, 2nd edn, 2013 CrossRef;
(c) J. Wang, M. Sánchez-Roselló, J. L. Aceña, C. del Pozo, A. E. Sorochinsky, S. Fustero, V. A. Soloshonok and H. Liu, Fluorine in Pharmaceutical Industry: Fluorine-Containing Drugs Introduced to the Market in the Last Decade (2001–2011), Chem. Rev., 2014, 114, 2432–2506 CrossRef CAS;
(d) D. O'Hagan and H. Deng, Enzymatic fluorination and biotechnological developments of the fluorinase, Chem. Rev., 2015, 115, 634–649 CrossRef;
(e) E. P. Gillis, K. J. Eastman, M. D. Hill, D. J. Donnelly and N. A. Meanwell, Applications of Fluorine in Medicinal Chemistry, J. Med. Chem., 2015, 58, 8315–8359 CrossRef CAS;
(f) Y. Zhou, J. Wang, Z. Gu, S. Wang, W. Zhu, J. L. Aceña, V. A. Soloshonok, K. Izawa and H. Liu, Next Generation of Fluorine-Containing Pharmaceuticals, Compounds Currently in Phase II–III Clinical Trials of Major Pharmaceutical Companies: New Structural Trends and Therapeutic Areas, Chem. Rev., 2016, 116, 422–518 CrossRef CAS;
(g) N. A. Meanwell, Fluorine and Fluorinated Motifs in the Design and Application of Bioisosteres for Drug Design, J. Med. Chem., 2018, 61, 5822–5880 CrossRef CAS.
-
(a) J. Charpentier, N. Fruh and A. Togni, Electrophilic trifluoromethylation by use of hypervalent iodine reagents, Chem. Rev., 2015, 115, 650–682 CrossRef CAS;
(b) C. Alonso, E. Martinez de Marigorta, G. Rubiales and F. Palacios, Carbon trifluoromethylation reactions of hydrocarbon derivatives and heteroarenes, Chem. Rev., 2015, 115, 1847–1935 CrossRef CAS;
(c) H. Xiao, Z. Zhang, Y. Fang, L. Zhu and C. Li, Radical trifluoromethylation, Chem. Soc. Rev., 2021, 50, 6308–6319 RSC;
(d) X.-H. Xu, K. Matsuzaki and N. Shibata, Synthetic Methods for Compounds Having CF3-S Units on Carbon by Trifluoromethylation, Trifluoromethylthiolation, Triflylation, and Related Reactions, Chem. Rev., 2015, 115, 731–764 CrossRef CAS;
(e) X. Zhang and P. Tang, Recent advances in new trifluoromethoxylation reagents, Sci. China: Chem., 2019, 62, 525–532 CrossRef CAS;
(f) X. Jiang and P. Tang, Advances in Enantioselective Construction of Trifluoromethoxylated Stereogenic Carbon Centers, Chin. J. Chem., 2020, 38, 101–102 CrossRef CAS;
(g) J. B. I. Sap, C. F. Meyer, N. J. W. Straathof, N. Iwumene, C. W. am Ende, A. A. Trabanco and V. Gouverneur, Late-stage difluoromethylation: concepts, developments and perspective, Chem. Soc. Rev., 2021, 50, 8214–8247 RSC;
(h) S. Barata-Vallejo, S. M. Bonesi and A. Postigo, Trifluoromethoxylation Reactions of (Hetero) arenes, Olefinic Systems and Aliphatic Saturated Substrates, Chem. – Eur. J., 2022, 28, e202201776 CrossRef CAS.
-
(a) C. Ni and J. Hu, Recent Advances in the Synthetic Application of Difluorocarbene, Synthesis, 2014, 46, 842–863 CrossRef;
(b) A. D. Dilman and V. V. Levin, Difluorocarbene as a Building Block for Consecutive Bond-Forming Reactions, Acc. Chem. Res., 2018, 51, 1272–1280 CrossRef CAS;
(c) W. Zhang and Y. Wang, Recent advances in carbon-difluoroalkylation and -difluoroolefination with difluorocarbene, Tetrahedron Lett., 2018, 59, 1301–1308 CrossRef CAS;
(d) J.-H. Lin and J.-C. Xiao, Fluorinated Ylides/Carbenes and Related Intermediates from Phosphonium/Sulfonium Salts, Acc. Chem. Res., 2020, 53, 1498–1510 CrossRef CAS;
(e) W. Zhou, W.-J. Pan, J. Chen, M. Zhang, J.-H. Lin, W. Cao and J.-C. Xiao, Transition-metal difluorocarbene complexes, Chem. Commun., 2021, 57, 9316–9329 RSC;
(f) X. Ma, J. Su and Q. Song, Unconventional Transformations of Difluorocarbene with Amines and Ethers, Acc. Chem. Res., 2023, 56, 592–607 CrossRef CAS;
(g) K. Fuchibe and J. Ichikawa, Generation of difluorocarbenes and introduction of fluorinated one carbon units into carbonyl and related compounds, Chem. Commun., 2023, 59, 2532–2540 RSC.
-
(a) Y. Fujioka and H. Amii, Boron-Substituted Difluorocyclopropanes: New Building Blocks of gem-Difluorocyclopropanes, Org. Lett., 2008, 10, 769–772 CrossRef CAS;
(b) F. Wang, T. Luo, J. Hu, Y. Wang, H. Krishnan, P. Jog, S. Ganesh, G. Prakash and G. Olah, Synthesis of gem-difluorinated cyclopropanes and cyclopropenes: trifluoromethyltrimethylsilane as a difluorocarbene source, Angew. Chem., Int. Ed., 2011, 50, 7153–7157 CrossRef CAS;
(c) F. Wang, W. Zhang, J. Zhu, H. Li, K.-W. Huang and J. Hu, Chloride ion-catalyzed generation of difluorocarbene for efficient preparation of gem-difluorinated cyclopropenes and cyclopropanes, Chem. Commun., 2011, 47, 2411–2413 RSC;
(d) P. Rulliere, P. Cyr and A. B. Charette, Difluorocarbene Addition to Alkenes and Alkynes in Continuous Flow, Org. Lett., 2016, 18, 1988–1991 CrossRef CAS;
(e) A. Garcia-Dominguez, T. H. West, J. J. Primozic, K. M. Grant, C. P. Johnston, G. G. Cumming, A. G. Leach and G. C. Lloyd-Jones, Difluorocarbene Generation from TMSCF3: Kinetics and Mechanism of NaI-Mediated and Si-Induced Anionic Chain Reactions, J. Am. Chem. Soc., 2020, 142, 14649–14663 CrossRef CAS.
-
(a) L. Li, F. Wang, C. Ni and J. Hu, Synthesis of gem-Difluorocyclopropa(e)nes and O-, S-, N-, and P-Difluoromethylated Compounds with TMSCF2Br, Angew. Chem., Int. Ed., 2013, 52, 12390–12394 CrossRef CAS;
(b) X.-Y. Deng, J.-H. Lin, J. Zheng and J.-C. Xiao, Difluoromethylation and gem-difluorocyclopropenation with difluorocarbene generated by decarboxylation, Chem. Commun., 2015, 51, 8805–8808 RSC;
(c) Q. Xie, C. Ni, R. Zhang, L. Li, J. Rong and J. Hu, Efficient Difluoromethylation of Alcohols Using TMSCF2Br as a Unique and Practical Difluorocarbene Reagent under Mild Conditions, Angew. Chem., Int. Ed., 2017, 56, 3206–3210 CrossRef CAS;
(d) J. B. I. Sap, C. F. Meyer, J. Ford, N. J. W. Straathof, A. B. Durr, M. J. Lelos, S. J. Paisey, T. A. Mollner, S. M. Hell, A. A. Trabanco, C. Genicot, C. W. am Ende, R. S. Paton, M. Tredwell and V. Gouverneur, [18F]Difluorocarbene for positron emission tomography, Nature, 2022, 606, 102–108 CrossRef CAS.
-
(a) J. D. Park, A. F. Benning, F. B. Downing, J. F. Laucius and R. C. McHarness, Synthesis of Tetrafluoroethylene-Pyrolysis of Monochlorodifluoromethane, Ind. Eng. Chem., 1947, 39, 354–358 CrossRef CAS;
(b) M. Hu, C. Ni, L. Li, Y. Han and J. Hu, gem-Difluoroolefination of Diazo Compounds with TMSCF3 or TMSCF2Br: Transition-Metal-Free Cross-Coupling of Two Carbene Precursors, J. Am. Chem. Soc., 2015, 137, 14496–14501 CrossRef CAS;
(c) J. Zheng, J.-H. Lin, L.-Y. Yu, Y. Wei, X. Zheng and J.-C. Xiao, Cross-Coupling between Difluorocarbene and Carbene-Derived Intermediates Generated from Diazocompounds for the Synthesis of gem-Difluoroolefins, Org. Lett., 2015, 17, 6150–6153 CrossRef CAS;
(d) Z. Zhang, W. Yu, C. Wu, C. Wang, Y. Zhang and J. Wang, Reaction of Diazo Compounds with Difluorocarbene: An Efficient Approach towards 1,1-Difluoroolefins, Angew. Chem., Int. Ed., 2016, 55, 273–277 CrossRef CAS;
(e) L. Li, C. Ni, Q. Xie, M. Hu, F. Wang and J. Hu, TMSCF3 as a Convenient Source of CF2=CF2 for Pentafluoroethylation, (Aryloxy)tetrafluoroethylation, and Tetrafluoroethylation, Angew. Chem., Int. Ed., 2017, 56, 9971–9975 CrossRef CAS.
-
(a) M. Huiban, M. Tredwell, S. Mizuta, Z. Wan, X. Zhang, T. L. Collier, V. Gouverneur and J. Passchier, A broadly applicable [18F]trifluoromethylation of aryl and heteroaryl iodides for PET imaging, Nat. Chem., 2013, 5, 941–944 CrossRef CAS;
(b) J. Zheng, J.-H. Lin, X.-Y. Deng and J.-C. Xiao, 1,8-Diazabicyclo[5.4.0]undec-7-ene (DBU)-Promoted Decomposition of Difluorocarbene and the Subsequent Trifluoromethylation, Org. Lett., 2015, 17, 532–535 CrossRef CAS;
(c) V. O. Smirnov, A. D. Volodin, A. A. Korlyukov and A. D. Dilman, Trapping of Difluorocarbene by Frustrated Lewis Pairs, Angew. Chem., Int. Ed., 2020, 59, 12428–12431 CrossRef CAS;
(d) E. A. Ilin, V. O. Smirnov, A. D. Volodin, A. A. Korlyukov and A. D. Dilman, ortho-Dialkylamino arylboranes as efficient reagents for difluorocarbene trapping, Chem. Commun., 2020, 56, 7140–7142 RSC;
(e) H. Liang, R. Liu, M. Zhou, Y. Fu, C. Ni and J. Hu, Difluorocarbene-Triggered Cyclization: Synthesis of (Hetero)arene-Fused 2,2-Difluoro-2,3-dihydrothiophenes, Org. Lett., 2020, 22, 7047–7051 CrossRef CAS;
(f) V. O. Smirnov, A. D. Volodin, A. A. Korlyukov and A. D. Dilman, All-carbon phosphoranes via difluorocarbene trapping, Chem. Commun., 2021, 57, 4823–4826 RSC;
(g) Y. Cai, W. Zhu, S. Zhao, C. Dong, Z. Xu and Y. Zhao, Difluorocarbene-Mediated Cascade Cyclization: The Multifunctional Role of Ruppert-Prakash Reagent, Org. Lett., 2021, 23, 3546–3551 CrossRef CAS;
(h) L. Peng, H. Wang and C. Guo, Copper-Catalyzed Enantioselective Difluoromethylation of Amino Acids via Difluorocarbene, J. Am. Chem. Soc., 2021, 143, 6376–6381 CrossRef CAS;
(i) H. Sheng, J. Su, X. Li, X. Li and Q. Song, Double Capture of Difluorocarbene by 2-Aminostyrenes Enables the Construction of 3-(2,2-Difluoroethyl)-2-fluoroindoles, Org. Lett., 2021, 23, 7781–7786 CrossRef CAS;
(j) H. Sheng, Z. Chen, X. Li, J. Su and Q. Song, Construction and transformations of 2,2-difluoro-2,3-dihydrofurans from enaminones and difluorocarbene, Org. Chem. Front., 2022, 9, 3000–3005 RSC;
(k) F. Yuan, H. Sun, C. Yang, H. Yang, L. Pan, X. Zhang, R. Tian, L. Li, W. Chen, X. Wu and H. Wu, Difluorocarbene-derived rapid late-stage trifluoromethylation of 5-iodotriazoles for the synthesis of 18F-labeled radiotracers, Chin. Chem. Lett., 2023, 34, 107960 CrossRef CAS;
(l) A. Liu, C. Ni, Q. Xie and J. Hu, Transition-Metal-Free Controllable Single and Double Difluoromethylene Formal Insertions into C-H Bonds of Aldehydes with TMSCF2Br, Angew. Chem., Int. Ed., 2023, 62, e202217088 CrossRef CAS.
-
(a) P. J. Brothers and W. R. Roper, Transition-metal dihalocarbene complexes, Chem. Rev., 1988, 88, 1293–1326 CrossRef CAS;
(b) X.-P. Fu, X.-S. Xue, X.-Y. Zhang, Y.-L. Xiao, S. Zhang, Y.-L. Guo, X. Leng, K. N. Houk and X. Zhang, Controllable catalytic difluorocarbene transfer enables access to diversified fluoroalkylated arenes, Nat. Chem., 2019, 11, 948–956 CrossRef CAS.
-
(a) D. J. Harrison, G. M. Lee, M. C. Leclerc, I. Korobkov and R. T. Baker, Cobalt Fluorocarbenes: Cycloaddition Reactions with Tetrafluoroethylene and Reactivity of the Perfluorometallacyclic Products, J. Am. Chem. Soc., 2013, 135, 18296–18299 CrossRef CAS;
(b) G. M. Lee, D. J. Harrison, I. Korobkov and R. T. Baker, Stepwise addition of difluorocarbene to a transition metal centre, Chem. Commun., 2014, 50, 1128–1130 RSC;
(c) Y. Takahira and Y. Morizawa, Ruthenium-Catalyzed Olefin Cross-Metathesis with Tetrafluoroethylene and Analogous Fluoroolefins, J. Am. Chem. Soc., 2015, 137, 7031–7034 CrossRef CAS.
-
(a) Z. Feng, Q.-Q. Min and X. Zhang, Access to Difluoromethylated Arenes by Pd-Catalyzed Reaction of Arylboronic Acids with Bromodifluoroacetate, Org. Lett., 2016, 18, 44–47 CrossRef CAS;
(b) X.-Y. Deng, J.-H. Lin and J.-C. Xiao, Pd-Catalyzed Transfer of Difluorocarbene, Org. Lett., 2016, 18, 4384–4387 CrossRef CAS;
(c) K. Fuchibe, T. Aono, J. Hu and J. Ichikawa, Copper(I)-Catalyzed [4 + 1] Cycloaddition of Silyl Dienol Ethers with Sodium Bromodifluoroacetate: Access to β,β-Difluorocyclopentanone Derivatives, Org. Lett., 2016, 18, 4502–4505 CrossRef CAS;
(d) Z. Feng, Q. Q. Min, X. P. Fu, L. An and X. Zhang, Chlorodifluoromethane-triggered formation of difluoromethylated arenes catalysed by palladium, Nat. Chem., 2017, 9, 918–923 CrossRef CAS;
(e) Z.-W. Xu, W. Zhang, J.-H. Lin, C. M. Jin and J.-C. Xiao, Pd–catalyzed transfer of difluorocarbene for three component cross–coupling, Chin. J. Chem., 2020, 38, 1647–1650 CrossRef CAS;
(f) X.-Y. Zhang, X.-P. Fu, S. Zhang and X. Zhang, Palladium Difluorocarbene Involved Catalytic Coupling with Terminal Alkynes, CCS Chem., 2020, 2, 293–304 CrossRef CAS;
(g) X. Zeng, Y. Li, Q.-Q. Min, X.-S. Xue and X. Zhang, Copper-catalysed difluorocarbene transfer enables modular synthesis, Nat. Chem., 2023, 15, 1064–1073 CrossRef CAS;
(h) X.-Y. Zhang, S.-P. Sun, Y.-Q. Sang, X.-S. Xue, Q.-Q. Min and X. Zhang, Reductive Catalytic Difluorocarbene Transfer via Palladium Catalysis, Angew. Chem., Int. Ed., 2023, 62, e202306501 CrossRef CAS.
-
(a) Y. Kim, J. Heo, D. Kim, S. Chang and S. Seo, Ring-opening functionalizations of unstrained cyclic amines enabled by difluorocarbene transfer, Nat. Commun., 2020, 11, 4761 CrossRef CAS;
(b) J. Su, X. Ma, Z. Ou and Q. Song, Deconstructive Functionalizations of Unstrained Carbon-Nitrogen Cleavage Enabled by Difluorocarbene, ACS Cent. Sci., 2020, 6, 1819–1826 CrossRef CAS;
(c) X. Liu, H. Sheng, Y. Zhou and Q. Song, Pd-Catalyzed Assembly of Fluoren-9-ones by Merging of C-H Activation and Difluorocarbene Transfer, Org. Lett., 2021, 23, 2543–2547 CrossRef CAS;
(d) A. Liu, C. Ni, Q. Xie and J. Hu, TMSCF2Br-Enabled Fluorination-Aminocarbonylation of Aldehydes: Modular Access to α-Fluoroamides, Angew. Chem., Int. Ed., 2022, 61, e202115467 CrossRef CAS;
(e) S. Wang, X. Li, S. Jin, K. Liu, C. Dong, J. Su and Q. Song, Difluorocarbene-enabled access to 1,3-oxazin-6-ones from enamides, Org. Chem. Front., 2022, 9, 1282–1287 RSC;
(f) G. Zhang, Q. Shi, M. Hou, K. Yang, S. Wang, S. Wang, W. Li, C. Li, J. Qiu, H. Xu, L. Zhou, C. Wang, S.-J. Li, Y. Lan and Q. Song, Atom recombination of difluorocarbene enables 3-fluorinated oxindoles from 2-aminoarylketones, CCS Chem., 2022, 4, 1671–1679 CrossRef CAS;
(g) S. Chen, H. Huang, X. Li, X. Ma, J. Su and Q. Song, Difluorocarbene-Enabled Synthesis of 3-Alkenyl-2-oxindoles from ortho-Aminophenylacetylenes, Org. Lett., 2023, 25, 1178–1182 CrossRef CAS.
-
(a) M. Zhang, J.-H. Lin, C.-M. Jin and J.-C. Xiao, Difluorocarbene-based cyanodifluoromethylation of alkenes induced by a dual-functional Cu-catalyst, Chem. Commun., 2021, 57, 2649–2652 RSC;
(b) M. Zhang, J.-H. Lin and J.-C. Xiao, Photocatalyzed Cyanodifluoromethylation of Alkenes, Angew. Chem., Int. Ed., 2019, 58, 6079–6083 CrossRef CAS.
-
(a) Y.-X. Si, P.-F. Zhu and S.-L. Zhang, Synthesis of Isocyanides by Reacting Primary Amines with Difluorocarbene, Org. Lett., 2020, 22, 9086–9090 CrossRef CAS;
(b) B.-J. Jiang and S.-L. Zhang, Synthesis of Quinolines and 2-Functionalized Quinolines by Difluorocarbene Incorporation, Adv. Synth. Catal., 2022, 364, 2157–2162 CrossRef CAS.
- J. Su, X. Hu, H. Huang, Y. Guo and Q. Song, Difluorocarbene enables to access 2-fluoroindoles from ortho-vinylanilines, Nat. Commun., 2021, 12, 4986 CrossRef CAS.
- J. Zheng, J. Cai, J.-H. Lin, Y. Guo and J.-C. Xiao, Synthesis and decarboxylative Wittig reaction of difluoromethylene phosphobetaine, Chem. Commun., 2013, 49, 7513–7515 RSC.
-
(a) J. Zheng, L. Wang, J.-H. Lin, J.-C. Xiao and S. H. Liang, Difluorocarbene-Derived Trifluoromethylthiolation and [18F]Trifluoromethylthiolation of Aliphatic Electrophiles, Angew. Chem., Int. Ed., 2015, 54, 13236–13240 CrossRef CAS;
(b) J. Yu, J.-H. Lin and J.-C. Xiao, Reaction of Thiocarbonyl Fluoride Generated from Difluorocarbene with Amines, Angew. Chem., Int. Ed., 2017, 56, 16669–16673 CrossRef CAS;
(c) J. Zheng, R. Cheng, J.-H. Lin, D. H. Yu, L. Ma, L. Jia, L. Zhang, L. Wang, J.-C. Xiao and S. H. Liang, An Unconventional Mechanistic Insight into SCF3 Formation from Difluorocarbene: Preparation of 18F-Labeled alpha-SCF3 Carbonyl Compounds, Angew. Chem., Int. Ed., 2017, 56, 3196–3200 CrossRef CAS;
(d) J. Yu, J.-H. Lin, D. Yu, R. Du and J.-C. Xiao, Oxidation of difluorocarbene and subsequent trifluoromethoxylation, Nat. Commun., 2019, 10, 5362 CrossRef.
-
(a) Y. Qiao, T. Si, M.-H. Yang and R. A. Altman, Metal-Free Trifluoromethylation of Aromatic and Heteroaromatic Aldehydes and Ketones, J. Org. Chem., 2014, 79, 7122–7131 CrossRef CAS;
(b) V. V. Levin, A. L. Trifonov, A. A. Zemtsov, M. I. Struchkova, D. E. Arkhipov and A. D. Dilman, Difluoromethylene Phosphabetaine as an Equivalent of Difluoromethyl Carbanion, Org. Lett., 2014, 16, 6256–6259 CrossRef CAS;
(c) Y. Liu, K. Zhang, Y. Huang, S. Pan, X.-Q. Liu, Y. Yang, Y. Jiang and X.-H. Xu, Synthesis of 3-fluoroalkenyl-3-trifluoromethyl-2-oxindoles by the reaction of indoline-2,3-diones with difluoromethylene phosphabetaine, Chem. Commun., 2016, 52, 5969–5972 RSC;
(d) L. I. Panferova, A. V. Tsymbal, V. V. Levin, M. I. Struchkova and A. D. Dilman, Reactions of gem-Difluorinated Phosphonium Salts Induced by Light, Org. Lett., 2016, 18, 996–999 CrossRef CAS;
(e) L. I. Panferova, V. V. Levin, M. I. Struchkova and A. D. Dilman, Light-mediated copper-catalyzed phosphorus/halogen exchange in 1,1-difluoroalkylphosphonium salts, Chem. Commun., 2019, 55, 1314 RSC–1317;
(f) C. W. Kee, O. Tack, F. Guibbal, T. C. Wilson, P. G. Isenegger, M. Imiolek, S. Verhoog, M. Tilby, G. Boscutti, S. Ashworth, J. Chupin, R. Kashani, A. W. J. Poh, J. K. Sosabowski, S. Macholl, C. Plisson, B. Cornelissen, M. C. Willis, J. Passchier, B. G. Davis and V. Gouverneur,
18F-Trifluoromethanesulfinate Enables Direct C-H 18F-Trifluoromethylation of Native Aromatic Residues in Peptides, J. Am. Chem. Soc., 2020, 142, 1180–1185 CrossRef CAS;
(g) Y. Jia, Y. Yuan, J. Huang, Z.-X. Jiang and Z. Yang, Synthesis of Difluorinated Heterocyclics through Metal-Free [8 + 1] and [4 + 1] Cycloaddition of Difluorocarbene, Org. Lett., 2021, 23, 2670–2675 CrossRef CAS.
-
(a) L. Le Bozec and C. J. Moody, Naturally Occurring NitrogenSulfur Compounds. The Benzothiazole Alkaloids, Aust. J. Chem., 2009, 62, 639–647 CrossRef CAS;
(b) V. Facchinetti, R. d. R. Reis, C. R. B. Gomes and T. R. A. Vasconcelos, Chemistry and biological activities of 1,3-benzothiazoles, Mini-Rev. Org. Chem., 2012, 9, 44–53 CrossRef CAS;
(c) M. Singh and S. K. Singh, Benzothiazoles: How Relevant in Cancer Drug Design Strategy?, Anti-Cancer Agents Med. Chem., 2014, 14, 127–146 CrossRef CAS;
(d) A. Kamal, M. A. H. Syed and S. M. Mohammed, Therapeutic potential of benzothiazoles: a patent review (2010–2014), Expert Opin. Ther. Pat., 2015, 25, 335–349 CrossRef CAS;
(e) R. S. Keri, M. R. Patil, S. A. Patil and S. Budagupi, A comprehensive review in current developments of benzothiazole-based molecules in medicinal chemistry, Eur. J. Med. Chem., 2015, 89, 207–251 CrossRef CAS;
(f) S. Agarwal, D. Gandhi and P. Kalal, Benzothiazole: A Versatile and Multitargeted Pharmacophore in the Field of Medicinal Chemistry, Lett. Org. Chem., 2017, 14, 729–742 CrossRef CAS;
(g) M. Gjorgjieva, T. Tomasic, D. Kikelj and L. P. Masic, Benzothiazole-based Compounds in Antibacterial Drug Discovery, Curr. Med. Chem., 2018, 25, 5218–5236 CrossRef CAS;
(h) C. Liao, U.-J. Kim and K. Kannan, A Review of Environmental Occurrence, Fate, Exposure, and Toxicity of Benzothiazoles, Environ. Sci. Technol., 2018, 52, 5007–5026 CrossRef CAS;
(i) A. Irfan, F. Batool, S. A. Zahra Naqvi, A. Islam, S. M. Osman, A. Nocentini, S. A. Alissa and C. T. Supuran, Benzothiazole derivatives as anticancer agents, J. Enzyme Inhib. Med. Chem., 2020, 35, 265–279 CrossRef CAS;
(j) N. Pathak, E. Rathi, N. Kumar, S. G. Kini and C. M. Rao, A Review on Anticancer Potentials of Benzothiazole Derivatives, Mini-Rev. Med. Chem., 2020, 20, 12–23 CrossRef CAS.
-
(a) M. Arisawa, S. Tanii, T. Tazawa and M. Yamaguchi, Rhodium-catalyzed transformation of heteroaryl aryl ethers into heteroaryl fluorides, Chem. Commun., 2016, 52, 11390–11393 RSC;
(b) N. Yoneda and T. Fukuhara, Facile preparation of aromatic fluorides by deaminative fluorination of aminoarenes using hydrogen fluoride combined with bases, Tetrahedron, 1996, 52, 23–36 CrossRef CAS;
(c) F. G. Simeon, M. T. Wendahl and V. W. Pike, The [18F]2-fluoro-1,3-thiazolyl moiety-an easily-accessible structural motif for prospective molecular imaging radiotracers, Tetrahedron Lett., 2010, 51, 6034–6036 CrossRef CAS;
(d) C. M. Hong, A. M. Whittaker and D. M. Schultz, Nucleophilic Fluorination of Heteroaryl Chlorides and Aryl Triflates Enabled by Cooperative Catalysis, J. Org. Chem., 2021, 86, 3999–4006 CrossRef CAS;
(e) C. Ghiazza, T. Faber, A. Gomez-Palomino and J. Cornella, Deaminative chlorination of aminoheterocycles, Nat. Chem., 2022, 14, 78–84 CrossRef CAS.
- E. A. Castro, M. E. Aliaga, M. Gazitúa and J. G. Santos, The nucleofuge in the pyridinolysis of O-(4-nitrophenyl) S-aryl thio and dithiocarbonates, J. Phys. Org. Chem., 2012, 25, 994–997 CrossRef CAS.
- C. S. Thomoson and W. R. Dolbier Jr., Use of Fluoroform as a Source of Difluorocarbene
in the Synthesis of Difluoromethoxy- and Difluorothiomethoxyarenes, J. Org. Chem., 2013, 78, 8904–8908 CrossRef CAS.
Footnote |
† Electronic supplementary information (ESI) available: Materials and methods, experimental procedures, and 1H NMR, 19F NMR, 13C NMR, IR, and MS data, and NMR spectra (PDF). CCDC 2283499 (compound 3y). For ESI and crystallographic data in CIF or other electronic format see DOI: https://doi.org/10.1039/d3qo01422c |
|
This journal is © the Partner Organisations 2024 |
Click here to see how this site uses Cookies. View our privacy policy here.