DOI:
10.1039/D4QI00831F
(Research Article)
Inorg. Chem. Front., 2024,
11, 3458-3464
Fullerene rotation dictated by benzene–fullerene interactions†
Received
2nd April 2024
, Accepted 4th May 2024
First published on 8th May 2024
Abstract
The movement of metal ions inside the fullerene cage signifies the unique structural character of metallofullerenes; however, concrete details on this movement are extremely difficult to observe. In this work, we elucidated the structure of Dy2ScN@C80 using variable temperature single crystal X-ray diffraction. Dynamic disorders with the Dy2ScN and C80 rotations driven by temperature were precisely presented in detail. The position of the solvent molecule benzene in the crystal lattice proved to have a powerful impact on hindering fullerene rotation. These results present a deep understanding of the unique structural character of metallofullerenes, facilitating their high-accuracy structure–property relationship investigation.
The fullerene cage provides a robust confining arena for metal clusters that are otherwise unstable, allowing for the investigation of their peculiar structures/interactions and interesting properties. Versatile metal clusters have been discovered to be hosted by fullerene cages, and these fullerenes show application potential in biomedicine and materials science.1–4 Elucidating the structures of these metallofullerenes with single-crystal X-ray diffraction proved powerful in understanding these novel molecules.5–14 Determining the structure with high accuracy is fundamentally important to understand their properties such as bonding interactions between the entities of metallofullerenes and the crystal field around the metal ions.15–23 The ubiquitous disorders (more than 95%) in metallofullerene crystals originate from the round shape of fullerene cages, which tend to rotate in the crystal lattice, and the shallow potential energy surface supplied by the fullerene cage to hold the encapsulated metal ions.24–26 Co-crystallization with metal octaethylporphyrin or decapyrrylcorannulene significantly constrained the rotation of fullerene cages.27,28 However, the rotation of metal clusters in the fullerene cages persists as its intrinsic character.24–26,29,30 Trimetallic nitride clusterfullerenes have attracted broad interest since their discovery.6,31 A diversified combination of the metal ions from transition and rare-earth metals produces molecules with fascinating single-molecule magnetism represented by DyM2N@C80 and Dy2MN@C80 (M = Sc,32–36 Y,37,38 La,38 ErSc,39 Lu,40 or V
41).42 The combination of cluster rotation with mixed metals presents a great challenge in their structural elucidation by single crystal X-ray diffraction because the overlapping between different metal ions precludes their accurate assignment.33,38,39,43 Recently, we observed the snapshots of M3N rotation inside the C80 cage with spinning top character for heavy metal ions (Ho and Lu),26 while strong rotation for light metal ions (Sc).24 Interestingly, the C80 cage rotation in these crystals was blocked up to 280 K. On the other hand, it is notable that Sc3N@C70 shows fullerene cage rotation starting from 160 K.25 Since the symmetry of C70 (C2v) is lower than that of C80 (Ih), it is expected that C80 has a lower energy barrier for rotation, thus requiring a lower temperature to drive its rotation in the same environment. The driving force for fullerene cage rotation in the crystal lattice is fascinating and still unclear. Herein, we precisely determined the structure of Dy2ScN@C80 using variable temperature single crystal X-ray diffraction. The C80 cage and Dy2ScN cluster rotations driven by temperature were precisely recorded with great details. Surprising effects of solvent benzene–fullerene interactions on the fullerene rotations were disclosed, revealing the subtle driving force for fullerene cage rotation in the crystal lattice.
Single crystals were obtained by co-crystallization of Dy2ScN@C80 in benzene with nickel octaethylporphyrin (NiOEP) in benzene through solvent diffusion/evaporation. X-ray diffraction data collection was carried out at the BESSY storage ring (BL14.2, Berlin-Adlershof, Germany).44 The XDSAPP2.0 suite was employed for data processing.45,46 The structures were solved using direct methods and refined using SHELXL-2018.47 Hydrogen atoms were added geometrically and refined with a riding model. The crystal data are presented in Table S1 in the ESI.† The asymmetric unit contains one intact NiOEP, one intact fullerene, one intact benzene, and two halves of benzene. The fullerene cage (fully ordered Ih(7)-C80 at 100 K, (symmetry notation Ih(7) will be omitted hereafter)) sits onto the NiOEP as shown in Fig. 1. The packing characteristics show the typical packing character of fullerene–NiOEP co-crystals, in which one fullerene molecule is supported by one NiOEP, as shown in the DySc2N@C80-NiOEP co-crystals.32
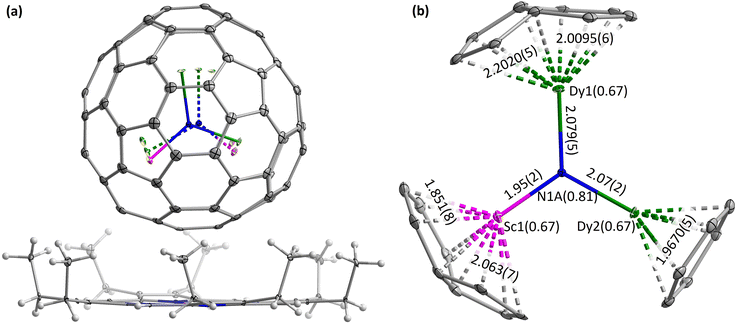 |
| Fig. 1 The structure of Dy2ScN@C80·NiOEP·2C6H6 with omitted benzene molecules (a) and the structure of Dy2ScN with coordinated fullerene cage fragments (b) observed at 100 K. The site occupancies with atom labels and bond lengths as well as the distance between metal ions and the coordinated hexagons/pentagons (in Å) are shown in the figure. Colour code: grey for C, white for H, blue for N, red for Ni, pink for Sc, and green for Dy. The thermal ellipsoids are set at 30% probability. | |
The encapsulated Dy2ScN cluster presents a slight disorder in the structure obtained at 100 K as shown in Fig. 1 and Fig. S1 in the ESI.† Three sites were refined with a site occupancy ratio of 0.67
:
0.29
:
0.04 for Dy2Sc. The significant size differences of Dy and Sc in the round C80 cage push the N out of the C80 centroid (vide infra); a similar disorder of N with three positions accordingly should be present along with the disordered Dy2Sc cluster. However, because of the rather short distances between the possible positions and the large differences of the site occupancies, it is difficult to refine all these positions accurately. Two N sites were refined with a site occupancy ratio of 0.81
:
0.19. The structure of Dy2ScN is evaluated with the main position Dy2ScN, which shows M–N bond lengths of 1.95(2), 2.07(2), and 2.079(5) Å, and bond angles of ∠Dy1N1ADy2 = 120.8(5)°, ∠Dy1N1ASc1 = 122.9(6)°, and ∠Dy2N1ASc1 = 116.3(3)°, indicating a planar Dy2ScN cluster. The N was pushed out of the centroid of C80 by the asymmetrical Dy2Sc cluster by 0.19(1) Å. The Dy ion far away from NiOEP is located under a hexagon/pentagon with a Dy–hexagon distance of 2.0095(6) Å (Dy–C bond lengths range from 2.345(4) to 2.599(4) Å), while the other Dy ion is located under a hexagon with a Dy–hexagon distance of 1.9670(5) Å (Dy–C bond lengths range from 2.433(4) to 2.464(4) Å). The Sc ion is located under a hexagon/pentagon with a Sc–hexagon distance of 1.851(8) Å (Sc–C bond lengths range from 2.238(7) to 2.492(7) Å). For comparison, the Dy–hexagon distance of DySc2N@C80 is 2.0241(8) Å, while the Dy–N and Sc–N bond lengths are 2.096(6), 1.965(6), and 1.978(6) Å,32 showing noticeable compression of Dy2ScN by the C80 cage.
Variable temperature single crystal X-ray diffraction was used to observe the detailed metal movement in the fullerene cage. In striking contrast to the previous reports on analogous M3N@C80 (M3 = Ho2Lu, Lu3, Sc3) without fullerene cage rotation until 280 K,24,26 the fullerene cage here presents rotation at 180 and 260 K as shown in Fig. 2, with 50% of them rotating to a new orientation, which shows the same interaction with the co-crystallized NiOEP. Both orientations are related by a mirror symmetry passing through NiOEP, as shown in Fig. S2 in the ESI.† The surprisingly different dynamic behaviours of C80 cages in a similar crystal lattice originate from the effects of benzene–fullerene interactions (π–π and C–H⋯π), as shown in Fig. 3, and Fig. S3 and Fig. S4 in the ESI.† The contribution of C–H⋯π is small compared to the π–π interactions,48 while the positions of two benzene molecules interacting with the fullerene through π–π interactions show a surprisingly powerful effect on the fullerene rotation, i.e., the fullerene starts rotation from 180 K when the two benzene molecules sit parallel, while the fullerene rotation is blocked until 280 K when the two benzene molecules sit with a magic angle of 125.1(8)° determined at 100 K.
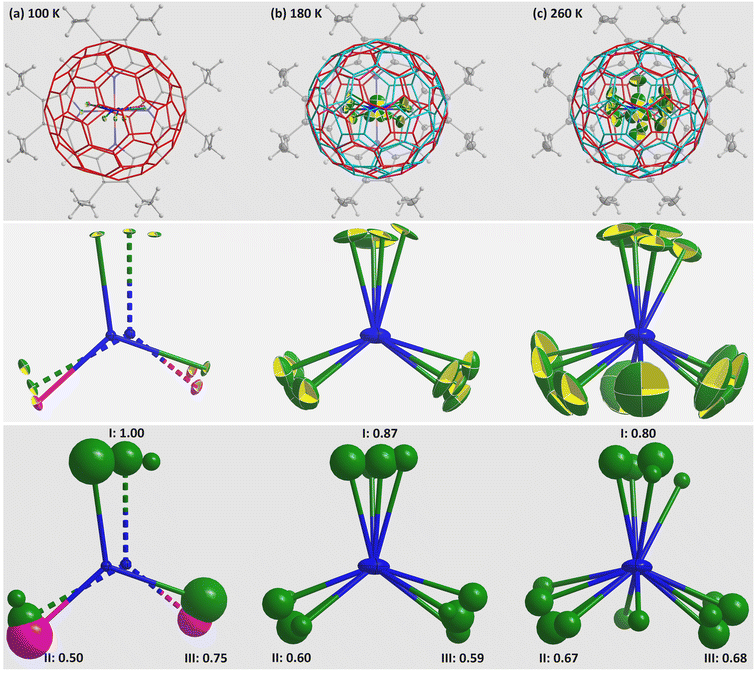 |
| Fig. 2 The structures of Dy2ScN@C80·NiOEP·2C6H6 viewed perpendicular to the NiOEP plane, observed at variable temperatures of (a) 100, (b) 180 and (c) 260 K. The structures with omitted benzene for clarity are shown in the upper panel, while the structures of the Dy2ScN cluster are highlighted in the middle panel, and the lower panel shows the Dy2ScN structure with a metal volume equal to the site occupancy, and the three main metal positions are labelled (I labels the position far away from NiOEP; II and III label the positions near the NiOEP, with the equivalent Dy occupancies noted in the figure) with their electron density gauged with equivalent Dy occupancies. Colour code: grey for C, white for H, blue for N, red for Ni, pink for Sc, and green for Dy. The thermal ellipsoids are set at 30% probability except for C80. C80 is highlighted with red/cyan to show the orientations. | |
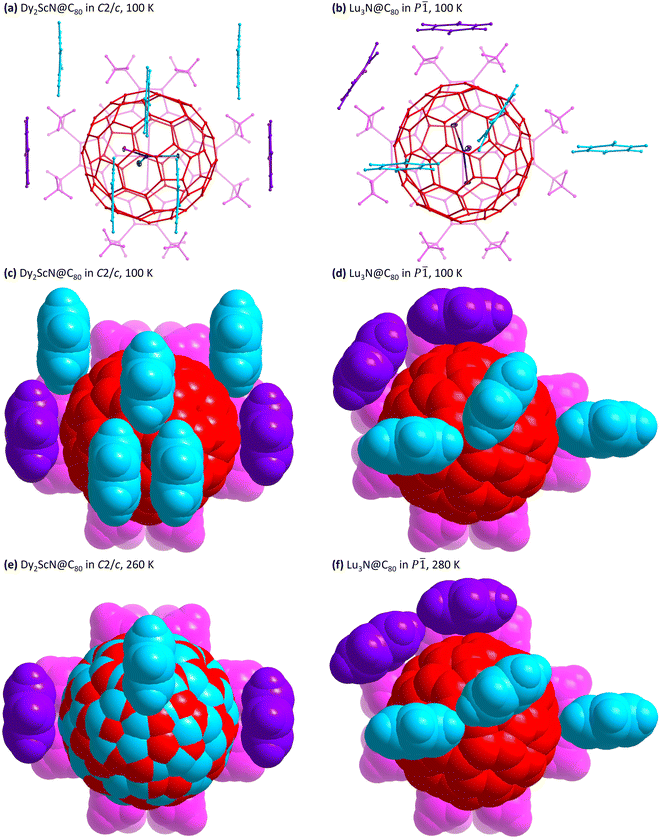 |
| Fig. 3 The structures of Dy2ScN@C80·NiOEP·2C6H6 in the C2/c space group (a, c and e) and Lu3N@C80·NiOEP·2C6H6 in the P space group (b, d, f, data from ref. 26) viewed perpendicular to the NiOEP plane. The ball-stick model is shown in the upper panel, while the space-filling structures are shown in the middle/lower panels. Colour code: red/cyan for the fullerene cage, pink for NiOEP, purple for benzene interacting with the fullerene cage through π–π interactions, and cyan for benzene interacting with the fullerene cage through C–H⋯π interactions. | |
The Dy2ScN cluster shows heavy disorder at 180 and 260 K, which hampers the assignment of Dy and Sc; thus, we applied heavier Dy with free occupancies to refine the positions of Dy/Sc.38 The positions of metal ions at 180 and 260 K show a tendency of “spinning top” rotation as observed for M3N@C80 (M3 = Ho2Lu, Lu3).26 The possibility of two metal ions near the NiOEP (labelled as II and III in Fig. 2) rotating to the far away position (labelled as I) is impossible to clarify in the case of M3N@C80 (M3 = Ho2Lu, Lu3) because of similar electron densities at the three positions (I, II, and III). Dy2ScN@C80 is ideal to clarify such a possibility with substantially different Dy and Sc electron densities (1Sc ≈ 0.25Dy in the refinement considering the effects of scattering power). The electron density imbalance of 0.50
:
0.75 (normalized with Dy) between II and III at 100 K is averaged off to 0.60
:
0.59 at 180 K, while the imbalance between I and II/III shows a tendency of averaging off with increasing temperature as shown in Fig. 2. Contrary to the disordered N at 100 K, the N is fully ordered at 180 and 260 K and is located at the centroid of C80. Because the N is pushed out of the centroid of C80 by the inequivalent Dy/Sc ions (vide supra), it does not occupy the crystallographically ordered N position. The flattened ellipsoids of N at 180 and 260 K hint at the rotation of N around the C80 centroid (see Fig. S5 in the ESI† for details). Thus, the assumption that the main position represents the real structure in single crystal X-ray diffraction involving disorder should be applied with caution. It is worth noting that the structures obtained at 180 and 260 K result in misrepresented Dy2SCN structures as shown in Table S2 in the ESI.† The Dy–N bond lengths of 2.07(2) and 2.079(5) Å determined at 100 K change to 2.052(5) and 1.949(6) Å at 180 K and to 2.019(9) and 1.935(11) Å at 260 K, showing remarkable discrepancies.
For most M3N@C80 co-crystallized with NiOEP, two metal atoms of M3N prefer to sit above the N–Ni–N bonds.24,26,49–51 The recently reported M2@C80-CF3 (M = Tb, Nd) co-crystallized with NiOEP also shows such tendency.5,52 The Dy2ScN cluster shown in Fig. S6 in the ESI† reveals that the encapsulated Dy2ScN sits above the N–Ni–N bonds at 100 K, while exhibiting rotation at 180 and 260 K, showing the subtle interaction between metal ions and the NiOEP molecule.
In conclusion, the structure of Dy2ScN@C80 was determined by variable temperature single crystal X-ray diffraction. The temperature-driven dynamics of Dy2ScN@C80 in the lattice was precisely recorded in great detail. The angle between the two benzenes interacting with the fullerene cage through π–π interaction shows a surprising ability to control the fullerene cage rotation. These results present a deep understanding of the metallofullerene structures, facilitating their high-accuracy structure–property relationship investigation.
Author contributions
Y. W. synthesized fullerenes, Y. W. and F. L. prepared the crystals and conducted the single crystal X-ray diffraction measurement and analysis. F. L. wrote the manuscript with a contribution from Y. W.
Conflicts of interest
There are no conflicts of interest to declare.
Acknowledgements
This research was financially supported by the Deutsche Forschungsgemeinschaft (LI 3055/3-1) and the Jiangsu Specially Appointed Professorship. The authors appreciate the great support of and fruitful discussion with Dr Alexey A. Popov. Diffraction data have been collected on BL14.2 at the BESSY II electron storage ring operated by the Helmholtz-Zentrum Berlin. The authors appreciate the help of Dr Manfred Weiss and his team during the experiments at BESSY II.
References
- A. A. Popov, S. Yang and L. Dunsch, Endohedral Fullerenes, Chem. Rev., 2013, 113, 5989–6113 CrossRef CAS PubMed.
- X. Lu, L. Feng, T. Akasaka and S. Nagase, Current status and future developments of endohedral metallofullerenes, Chem. Soc. Rev., 2012, 41, 7723–7760 RSC.
- S. Yang, T. Wei and F. Jin, When metal clusters meet carbon cages: endohedral clusterfullerenes, Chem. Soc. Rev., 2017, 46, 5005–5058 RSC.
- F. Liu, L. Spree, D. S. Krylov, G. Velkos, S. M. Avdoshenko and A. A. Popov, Single-Electron Lanthanide-Lanthanide Bonds Inside Fullerenes toward Robust Redox-Active Molecular Magnets, Acc. Chem. Res., 2019, 52, 2981–2993 CrossRef CAS PubMed.
- W. Yang, G. Velkos, M. Rosenkranz, S. Schiemenz, F. Liu and A. A. Popov, Nd─Nd Bond in Ih and D5h Cage Isomers of Nd2@C80 Stabilized by Electrophilic CF3 Addition, Adv. Sci., 2024, 11, 2305190 CrossRef CAS PubMed.
- W. Yang, M. Rosenkranz, G. Velkos, F. Ziegs, V. Dubrovin, S. Schiemenz, L. Spree, M. F. de Souza Barbosa, C. Guillemard, S. M. Valvidares, B. Büchner, F. Liu, S. Avdoshenko and A. A. Popov, Covalency versus magnetic axiality in Nd molecular magnets: Nd-photoluminescence, strong ligand-field, and unprecedented nephelauxetic effect in fullerenes NdM2N@C80 (M = Sc, Lu, Y), Chem. Sci., 2024, 15, 2141–2157 RSC.
- R. Guan, J. Huang, J. Xin, M. Chen, P. Du, Q. Li, Y.-Z. Tan, S. Yang and S.-Y. Xie, A stabilization rule for metal carbido cluster bearing μ3-carbido single-atom-ligand encapsulated in carbon cage, Nat. Commun., 2024, 15, 150 CrossRef CAS PubMed.
- P. Yu, M. Li, S. Hu, C. Pan, W. Shen, K. Guo, Y.-P. Xie, L. Bao, R. Zhang and X. Lu, Stabilizing non-IPR C,2(13333)-C74 cage with Lu2C2/Lu2O: the importance of encaged non-metallic elements, Chem. Commun., 2023, 59, 12990–12993 RSC.
- Y.-R. Yao, J. Zhao, Q. Meng, H.-S. Hu, M. Guo, Y. Yan, J. Zhuang, S. Yang, S. Fortier, L. Echegoyen, W. H. E. Schwarz, J. Li and N. Chen, Synthesis and Characterization of U≡C Triple Bonds in Fullerene Compounds, J. Am. Chem. Soc., 2023, 145, 25440–25449 CrossRef CAS PubMed.
- Y.-R. Yao, Z.-C. Chen, L. Chen, S.-Y. Zheng, S. Yang, S.-L. Deng, L. Echegoyen, Y.-Z. Tan, S.-Y. Xie and L.-S. Zheng, Two Metastable Endohedral Metallofullerenes Sc2C2@C,1(39656)-C82 and Sc2C2@C1(51383)-C84: Direct-C2-Insertion Products from Their Most Stable Precursors, J. Am. Chem. Soc., 2023, 145, 16778–16786 CrossRef CAS PubMed.
- Y. Yan, L. Abella, R. Sun, Y.-H. Fang, Y. Roselló, Y. Shen, M. Jin, A. Rodríguez-Fortea, C. de Graaf, Q. Meng, Y.-R. Yao, L. Echegoyen, B.-W. Wang, S. Gao, J. M. Poblet and N. Chen, Actinide-lanthanide single electron metal-metal bond formed in mixed-valence di-metallofullerenes, Nat. Commun., 2023, 14, 6637 CrossRef CAS PubMed.
- W. Xiang, Z. Hu, J. Xin, H. Jin, Z. Jiang, X. Han, M. Chen, Y.-R. Yao and S. Yang, Steering Single-Electron Metal–Metal Bonds and Hyperfine Coupling between a Transition Metal-Lanthanide Heteronuclear Bimetal Confined
in Carbon Cages, J. Am. Chem. Soc., 2023, 145, 22599–22608 CrossRef CAS PubMed.
- T.-X. Liu, X. Wang, S. Xia, M. Chen, M. Li, P. Yang, N. Ma, Z. Hu, S. Yang, G. Zhang and G.-W. Wang, Dearomative Ring-Fused Azafulleroids and Carbazole-Derived Metallofullerenes: Reactivity Dictated by Encapsulation in a Fullerene Cage, Angew. Chem., Int. Ed., 2023, 62, e202313074 CrossRef CAS PubMed.
- H. Jin, J. Xin, W. Xiang, Z. Jiang, X. Han, M. Chen, P. Du, Y.-R. Yao and S. Yang, Bandgap Engineering of Erbium-Metallofullerenes Toward Switchable Photoluminescence, Adv. Mater., 2023, 35, 2304121 CrossRef CAS PubMed.
- H. Jiang, X. Yu, M. Guo, Y.-R. Yao, Q. Meng, L. Echegoyen, J. Autschbach and N. Chen, USc2C2 and USc2NC Clusters with U–C Triple Bond Character Stabilized Inside Fullerene Cages, J. Am. Chem. Soc., 2023, 145, 5645–5654 CrossRef CAS PubMed.
- T. Cao, Q. Meng, Z. Fu, Y. Shen, Q. Wang, Y. Yan, B. Zhao, W. Wang, K. Merimi, A. Rodriguez-Fortea, Y.-R. Yao and N. Chen, Th@C,2(8)-C84 and Th@Cs(15)-C84: impact of actinide metal ions on the electronic structure of actinide endohedral metallofullerenes, Inorg. Chem. Front., 2023, 10, 6901–6908 RSC.
- P. Yu, M. Li, W. Shen, S. Hu, P.-Y. Yu, X. Tian, X. Zhao, L. Bao and X. Lu, An unprecedented C80 cage that violates the isolated pentagon rule, Inorg. Chem. Front., 2022, 9, 2264–2270 RSC.
- W. Yang, G. Velkos, S. Sudarkova, B. Büchner, S. M. Avdoshenko, F. Liu, A. A. Popov and N. Chen, Carbon cage isomers and magnetic Dy⋯Dy interactions in Dy2O@C88 and Dy2C2@C88 metallofullerenes, Inorg. Chem. Front., 2022, 9, 5805–5819 RSC.
- W. Xiang, X. Jiang, Y.-R. Yao, J. Xin, H. Jin, R. Guan, Q. Zhang, M. Chen, S.-Y. Xie, A. A. Popov and S. Yang, Monometallic Endohedral Azafullerene, J. Am. Chem. Soc., 2022, 144, 21587–21595 CrossRef CAS PubMed.
- G. Velkos, W. Yang, Y.-R. Yao, S. M. Sudarkova, F. Liu, S. M. Avdoshenko, N. Chen and A. A. Popov, Metallofullerene single-molecule magnet Dy2O@C2v(5)-C80 with a strong antiferromagnetic Dy⋯Dy coupling, Chem. Commun., 2022, 58, 7164–7167 RSC.
- Y. Shen, X. Yu, Q. Meng, Y.-R. Yao, J. Autschbach and N. Chen, ThC2@C82 versus Th@C84: unexpected formation of triangular thorium carbide cluster inside fullerenes, Chem. Sci., 2022, 13, 12980–12986 RSC.
- Q. Meng, L. Abella, Y.-R. Yao, D.-C. Sergentu, W. Yang, X. Liu, J. Zhuang, L. Echegoyen, J. Autschbach and N. Chen, A charged diatomic triple-bonded U≡N species trapped in C82 fullerene cages, Nat. Commun., 2022, 13, 7192 CrossRef CAS PubMed.
- R. Guan, Z.-C. Chen, J. Huang, H.-R. Tian, J. Xin, S.-W. Ying, M. Chen, Q. Zhang, Q. Li, S.-Y. Xie, L.-S. Zheng and S. Yang, Self-driven carbon atom implantation into fullerene embedding metal–carbon cluster, Proc. Natl. Acad. Sci. U. S. A., 2022, 119, e2202563119 CrossRef CAS PubMed.
- Y. Hao, Y. Wang, L. Spree and F. Liu, Rotation of fullerene molecules in the crystal lattice of fullerene/porphyrin: C60 and Sc3N@C80, Inorg. Chem. Front., 2021, 8, 122–126 RSC.
- Y. Hao, Y. Wang, V. Dubrovin, S. M. Avdoshenko, A. A. Popov and F. Liu, Caught in Phase Transition: Snapshot of the Metallofullerene Sc3N@C70 Rotation in the Crystal, J. Am. Chem. Soc., 2021, 143, 612–616 CrossRef CAS PubMed.
- F. Liu and L. Spree, Molecular spinning top: visualizing the dynamics of M3N@C80 with variable temperature single crystal X-ray diffraction, Chem. Commun., 2019, 55, 13000–13003 RSC.
- M. M. Olmstead, D. A. Costa, K. Maitra, B. C. Noll, S. L. Phillips, P. M. Van Calcar and A. L. Balch, Interaction of curved and flat molecular surfaces. The structures of crystalline compounds composed of fullerene (C60, C60O, C70, and C120O) and metal octaethylporphyrin units, J. Am. Chem. Soc., 1999, 121, 7090–7097 CrossRef CAS.
- Y.-Y. Xu, H.-R. Tian, S.-H. Li, Z.-C. Chen, Y.-R. Yao, S.-S. Wang, X. Zhang, Z.-Z. Zhu, S.-L. Deng, Q. Zhang, S. Yang, S.-Y. Xie, R.-B. Huang and L.-S. Zheng, Flexible decapyrrylcorannulene hosts, Nat. Commun., 2019, 10, 485 CrossRef CAS PubMed.
- F. Liu, G. Velkos, D. S. Krylov, L. Spree, M. Zalibera, R. Ray, N. A. Samoylova, C.-H. Chen, M. Rosenkranz, S. Schiemenz, F. Ziegs, K. Nenkov, A. Kostanyan, T. Greber, A. U. B. Wolter, M. Richter, B. Büchner, S. M. Avdoshenko and A. A. Popov, Air-stable redox-active nanomagnets with lanthanide spins radical-bridged by a metal–metal bond, Nat. Commun., 2019, 10, 571 CrossRef CAS PubMed.
- F. Liu, D. S. Krylov, L. Spree, S. M. Avdoshenko, N. A. Samoylova, M. Rosenkranz, A. Kostanyan, T. Greber, A. U. B. Wolter, B. Büchner and A. A. Popov, Single molecule magnet with an unpaired electron trapped between two lanthanide ions inside a fullerene, Nat. Commun., 2017, 8, 16098 CrossRef CAS PubMed.
- S. Stevenson, G. Rice, T. Glass, K. Harich, F. Cromer, M. R. Jordan, J. Craft, E. Hadju, R. Bible, M. M. Olmstead, K. Maitra, A. J. Fisher, A. L. Balch and H. C. Dorn, Small-bandgap endohedral metallofullerenes in high yield and purity, Nature, 1999, 401, 55–57 CrossRef CAS.
- D. S. Krylov, F. Liu, A. Brandenburg, L. Spree, V. Bon, S. Kaskel, A. U. B. Wolter, B. Büchner, S. M. Avdoshenko and A. A. Popov, Magnetization relaxation in the single-ion magnet DySc2N@C80: quantum tunneling, magnetic dilution, and unconventional temperature dependence, Phys. Chem. Chem. Phys., 2018, 20, 11656–11672 RSC.
- D. S. Krylov, F. Liu, S. M. Avdoshenko, L. Spree, B. Weise, A. Waske, A. U. B. Wolter, B. Büchner and A. A. Popov, Record-high thermal barrier of the relaxation of magnetization in the nitride clusterfullerene Dy2ScN@C80-Ih, Chem. Commun., 2017, 53, 7901–7904 RSC.
- R. Westerström, J. Dreiser, C. Piamonteze, M. Muntwiler, S. Weyeneth, K. Krämer, S.-X. Liu, S. Decurtins, A. Popov, S. Yang, L. Dunsch and T. Greber, Tunneling, remanence, and frustration in dysprosium-based endohedral single-molecule magnets, Phys. Rev. B: Condens.
Matter Mater. Phys., 2014, 89, 060406 CrossRef.
- R. Westerström, J. Dreiser, C. Piamonteze, M. Muntwiler, S. Weyeneth, H. Brune, S. Rusponi, F. Nolting, A. Popov, S. Yang, L. Dunsch and T. Greber, An Endohedral Single-Molecule Magnet with Long Relaxation Times: DySc2N@C80, J. Am. Chem. Soc., 2012, 134, 9840–9843 CrossRef PubMed.
- C. Schlesier, L. Spree, A. Kostanyan, R. Westerström, A. Brandenburg, A. Wolter, S. Yang, T. Greber and A. A. Popov, Strong carbon cage influence on the single molecule magnetism in Dy-Sc nitride clusterfullerenes, Chem. Commun., 2018, 54, 9730–9733 RSC.
- M. Nie, J. Liang, C. Zhao, Y. Lu, J. Zhang, W. Li, C. Wang and T. Wang, Single-Molecule Magnet with Thermally Activated Delayed Fluorescence Based on a Metallofullerene Integrated by Dysprosium and Yttrium Ions, ACS Nano, 2021, 15, 19080–19088 CrossRef CAS PubMed.
- Y. Hao, G. Velkos, S. Schiemenz, M. Rosenkranz, Y. Wang, B. Büchner, S. Avdoshenko, A. A. Popov and F. Liu, Using internal strain and mass to modulate Dy⋯Dy coupling and relaxation of magnetization in heterobimetallic metallofullerenes DyM2N@C80 and Dy2MN@C80 (M = Sc, Y, La, Lu), Inorg. Chem. Front., 2023, 10, 468–484 RSC.
- M. Nie, J. Xiong, C. Zhao, H. Meng, K. Zhang, Y. Han, J. Li, B. Wang, L. Feng, C. Wang and T. Wang, Luminescent single-molecule magnet of metallofullerene DyErScN@Ih,-C80, Nano Res., 2019, 12, 1727–1731 CrossRef CAS.
- L. Spree, C. Schlesier, A. Kostanyan, R. Westerström, T. Greber, B. Büchner, S. Avdoshenko and A. A. Popov, Single molecule magnets DyM2N@C80 and Dy2MN@C80 (M = Sc, Lu): The impact of diamagnetic metals on the Dy3+ magnetic anisotropy, Dy⋯Dy coupling, and mixing of molecular and lattice vibrations, Chem. – Eur. J., 2019, 26, 2436–2449 CrossRef PubMed.
- C. Huang, R. Sun, L. Bao, X. Tian, C. Pan, M. Li, W. Shen, K. Guo, B. Wang, X. Lu and S. Gao, A hard molecular nanomagnet from confined paramagnetic 3d-4f spins inside a fullerene cage, Nat. Commun., 2023, 14, 8443 CrossRef CAS PubMed.
- L. Spree and A. A. Popov, Recent advances in single molecule magnetism of dysprosium-metallofullerenes, Dalton Trans., 2019, 48, 2861–2871 RSC.
- C. Schlesier, F. Liu, V. Dubrovin, L. Spree, B. Büchner, S. M. Avdoshenko and A. A. Popov, Mixed dysprosium-lanthanide nitride clusterfullerenes DyM2N@C80-Ih and Dy2MN@C80-Ih (M = Gd, Er, Tm, and Lu): synthesis, molecular structure, and quantum motion of the endohedral nitrogen atom, Nanoscale, 2019, 11, 13139–13153 RSC.
- U. Mueller, R. Förster, M. Hellmig, F. U. Huschmann, A. Kastner, P. Malecki, S. Pühringer, M. Röwer, K. Sparta, M. Steffien, M. Ühlein, P. Wilk and M. S. Weiss, The macromolecular crystallography beamlines at BESSY II of the Helmholtz-Zentrum Berlin: Current status and perspectives, Eur. Phys. J. Plus, 2015, 130, 141 CrossRef.
- W. Kabsch, XDS, Acta Crystallogr., Sect. D: Biol. Crystallogr., 2010, 66, 125–132 CrossRef CAS PubMed.
- K. M. Sparta, M. Krug, U. Heinemann, U. Mueller and M. S. Weiss, XDSAPP2.0, J. Appl. Crystallogr., 2016, 49, 1085–1092 CrossRef.
- G. Sheldrick, Crystal structure refinement with SHELXL, Acta Crystallogr., Sect. C: Struct. Chem., 2015, 71, 3–8 Search PubMed.
- M.-M. Li, Y.-B. Wang, Y. Zhang and W. Wang, The Nature of the Noncovalent Interactions between Benzene and C60 Fullerene, J. Phys. Chem. A, 2016, 120, 5766–5772 CrossRef CAS PubMed.
- S. Stevenson, C. J. Chancellor, H. M. Lee, M. M. Olmstead and A. L. Balch, Internal and external factors in the structural organization in cocrystals of the mixed-metal endohedrals (GdSc2N@Ih,-C80, Gd2ScN@Ih-C80, and TbSc2N@Ih-C80) and nickel(II) octaethylporphyrin, Inorg. Chem., 2008, 47, 1420–1427 CrossRef CAS PubMed.
- M. M. Olmstead, T. Zuo, H. C. Dorn, T. Li and A. L. Balch, Metal Ion Size and the Pyramidalization of Trimetallic Nitride Units Inside a Fullerene Cage: Comparisons of the Crystal Structures of M3N@Ih,-C80 (M = Gd, Tb, Dy, Ho, Er, Tm, Lu, and Sc) and Some Mixed Metal Counterparts, Inorg. Chim. Acta, 2017, 468, 321–326 CrossRef CAS.
- V. Dubrovin, L.-H. Gan, B. Büchner, A. A. Popov and S. Avdoshenko, Endohedral metal-nitride cluster ordering in metallofullerene-NiII(OEP) complexes and crystals: a theoretical study, Phys. Chem. Chem. Phys., 2019, 21, 8197–8200 RSC.
- Y. Wang, G. Velkos, N. J. Israel, M. Rosenkranz, B. Büchner, F. Liu and A. A. Popov, Electrophilic Trifluoromethylation of Dimetallofullerene Anions en Route to Air-Stable Single-Molecule Magnets with High Blocking Temperature of Magnetization, J. Am. Chem. Soc., 2021, 143, 18139–18149 CrossRef CAS PubMed.
|
This journal is © the Partner Organisations 2024 |
Click here to see how this site uses Cookies. View our privacy policy here.