DOI:
10.1039/D4QI00340C
(Research Article)
Inorg. Chem. Front., 2024,
11, 3263-3269
Stepwise synthesis of heterotrimetallic FeII/PdII/AuI coordination cages†
Received
4th February 2024
, Accepted 24th April 2024
First published on 29th April 2024
Abstract
The synthesis of heterotrimetallic molecular cages is reported. The assemblies contain three types of coordination compounds: FeII clathrochelate complexes, AuI3(pyrazolate)3 complexes, and [PdII(pyridine)4]2+ complexes. The cages were obtained in a stepwise fashion. A nanometer-sized metalloligand with three terminal 3-pyridyl donor groups was prepared by connection of three FeII clathrochelate complexes via a central Au3(pyrazolate)3 complex. A related strategy was employed for the synthesis of a ditopic N-donor ligand with two functionalized FeII clathrochelate complexes. Combining the tritopic ligand with [Pd(CH3CN)4](BF4)2 resulted in the clean formation of a spherical PdII6FeII24AuI24 coordination cage with a diameter of ∼4.1 nm and a molecular weight of 21 kDa. The ditopic metalloligand was used for the construction of a PdII2FeII8AuI12 cage.
Introduction
Heterometallic coordination cages are attractive synthetic targets because the presence of different metal centers within one assembly can potentially lead to interesting new functions and properties.1 For the preparation of heterometallic cages, two main strategies have been explored. The first strategy relies on the use of polydentate ligands with distinct binding sites for the respective metal ions. A chemoselective complexation can be achieved by presenting different donor groups (e.g., hard and soft donors for hard and soft metal ions), or by exploiting the preference of a metal ion for a certain coordination geometry.2 The second strategy circumvents selectivity problems by introducing different metal ions in a step-wise fashion under kinetic control.3 Metalloligands play an important role in this context. Metalloligands are coordination compounds with donor groups in their ligand periphery.4 Due to the inert character of metalloligands, reactions with other metal ions can be performed without ligand scrambling.
The above-mentioned strategies have been used with great success for the construction of heterobimetallic coordination cages.1–4 However, the synthesis of cages containing three different metal ions is still challenging, and only a few examples have been reported in the literature.1,4,5
Below, we describe the synthesis of a heterotrimetallic PdII6FeII24AuI24 coordination cage. This cage was obtained by an Fe2+-templated polycondensation reaction, followed by regioselective complexation with Au+, and a final self-assembly step with Pd2+ (Scheme 1). A related strategy was used to prepare a cage with two Pd2+ ions, 8 Fe2+ ions, and 12 Au+ ions.
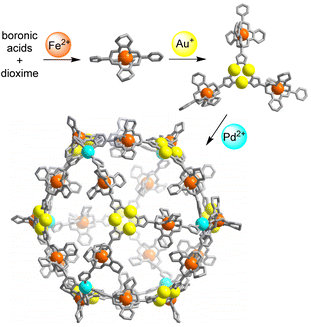 |
| Scheme 1 Stepwise synthesis of a coordination cage containing 6 Pd2+ ions, 24 Fe2+ ions, and 24 Au+ ions. | |
Results and discussion
Recently, we reported the synthesis of molecular cages containing trinuclear AuI3(pyrazolate)3 complexes.6 The cages were obtained by connection of pre-formed gold complexes via dynamic covalent imine chemistry. The incorporation of AuI3(pyrazolate)3 trimers into cages was motivated by the properties of these complexes: as highly π-basic compounds, they tend to form aggregates with π- or Lewis acids.7 Indeed, prismatic cages with two AuI3(pyrazolate)3 faces were found to encapsulate π-acidic aromatic compounds.6 Furthermore, we observed strong fullerene binding by a tetrahedral cage containing four AuI3(pyrazolate)3 complexes.6
For the present study, we explored the incorporation of AuI3(pyrazolate)3 trimers into heterometallic coordination cages. One challenge in building more complex nanostructures with AuI3(pyrazolate)3 complexes is the tendency of these trimers to form intermolecular Au⋯Au interactions. As a result, AuI3(pyrazolate)3 complexes often display low solubility.7,8 We hypothesized that solubility issues could be addressed by fusion of AuI3(pyrazolate)3 complexes to boronate ester-capped clathrochelate complexes.4c,9 The three-dimensional structure of the latter disfavors stacking interactions, and molecular nanostructures based on clathrochelates often display good solubility in organic solvents.4c,10
In order to connect clathrochelates with AuI3(pyrazolate)3 complexes, we first synthesized the FeII clathrochelate 1 (Scheme 2). This compound was obtained by a metal-templated polycondensation reaction involving four commercially available compounds: (1H-pyrazol-4-yl)boronic acid, 3-pyridylboronic acid, nioxime, and FeCl2. Due to the presence of two different boronic acids, symmetric clathrochelates with identical capping groups are obtained along with target 1. Chromatographic purification gave complex 1 in 26% yield.
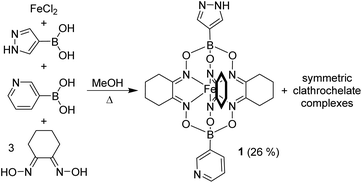 |
| Scheme 2 Synthesis of complex 1. | |
The solid-state structure of 1 was analyzed by single-crystal X-ray diffraction (XRD). The central FeII center in 1 displays a distorted trigonal prismatic coordination environment (Fig. 1), which is typical for such complexes.9 The Fe–N bond lengths are within the expected range (1.902(2)–1.913(2) Å).
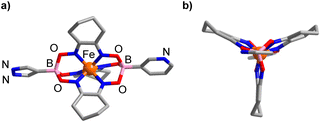 |
| Fig. 1 Molecular structure of complex 1 in the crystal with a view from the side (a) and along the B⋯B axis (b). Hydrogen atoms and co-crystallized solvent molecules are not shown. | |
Next, we have combined complex 1 with AuCl(SMe2) and NEt3 (Scheme 3). Based on literature reports,11 we expected a regioselective complexation of AuI to the pyrazole part of 1. Indeed, we were able to isolate the desired Au3(pyrazolate)3 complex 2 in high yield (87%).
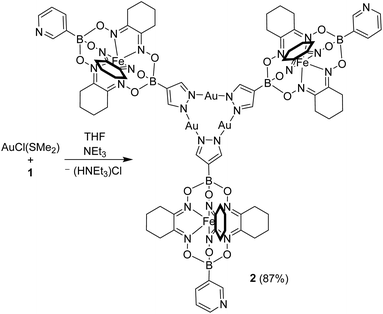 |
| Scheme 3 Synthesis of complex 2. | |
Complex 2 was found to be well soluble in dichloromethane and chloroform, but poorly soluble in acetonitrile or diethyl ether. The apparent C3 symmetry of 2 was reflected in the NMR spectra, which showed one set of signals for the three clathrochelate groups. The formation of a trimeric structure could be confirmed by high-resolution mass spectrometry.12
An XRD analysis of complex 2 confirmed that three clathrochelate complexes are connected via the pyrazolate N-atoms to three Au+ ions (Fig. 2a). In the solid state, two complexes form a closely packed dimer, with the AuI3(pyrazolate)3 complexes being positioned on top of each other (Fig. 2b). The arrangement seems to imply intermolecular aurophilic interactions. However, the shortest Au⋯Au distance is 3.8250(6) Å. This value is significantly larger than what is found for the solid-state structures of other Au3(pyrazolate)3 complexes, with typical Au⋯Au contacts in the range of 3.3 Å.7 Possibly, the bulky clathrochelates in 2 hamper a closer packing of the AuI3(pyrazolate)3 complexes. Another noteworthy feature of crystalline 2 is the non-planar arrangement of the three pyrazolate heterocycles. As a result, the plane defined by the three Fe2+ ions is inclined with respect to the plane defined by the three Au+ ions (Fig. 2c).
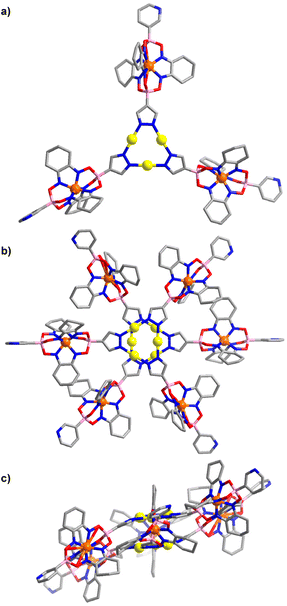 |
| Fig. 2 Molecular structure of complex 2 in the crystal (a), and two different views of the relative arrangement of two adjacent complexes (b and c). Hydrogen atoms are not shown. Color coding: C (gray), N (blue), O (red), B (pink), Au (yellow). | |
During previous studies, we had noted that AuI3(pyrazolate)3 complexes display a high kinetic inertness. This characteristic suggested that it might be possible to prepare low-symmetry complexes with different pyrazolate ligands. In order to investigate this point, we have examined the reaction between AuCl(SMe2) (3 eq.) and a mixture of complex 1 (2 eq.) and 3,5-dimethyl-1H-pyrazole (1 eq.) in the presence of NEt3 (Scheme 4). As expected, a mixture of products was obtained, including the symmetric trimer 2 (detected by mass spectrometry). Chromatographic purification gave the desired ditopic pyridyl ligand 3 in 15% yield.
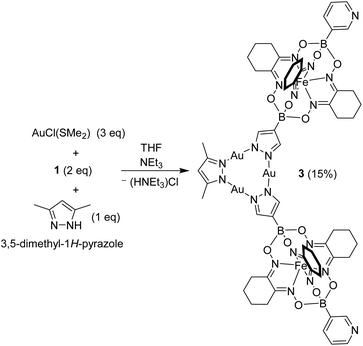 |
| Scheme 4 Synthesis of complex 3. | |
Complex 3 displays similar solubility properties as 2: it is soluble in dichloromethane and chloroform, but poorly soluble in acetonitrile. The NMR spectra were in line with the depicted structure, with one set of signals for the two clathrochelate groups, and one set of signals for the dimethylpyrazolate ligand. To the best of our knowledge, complex 3 represents the first example of a AuI3(pyrazolate)3 complex with two different pyrazolate ligands.13
The molecular structure of complex 3 in the crystal is depicted in Fig. 3. As in the case of 2, one can observe a stacked arrangement of the AuI3(pyrazolate)3 units of two adjacent complexes. Two close Au⋯Au contacts are observed (3.35(1) Å), indicating the presence of aurophilic interactions. Despite the presence of Au⋯Au interactions, complex 3 was not luminescent in the solid state.
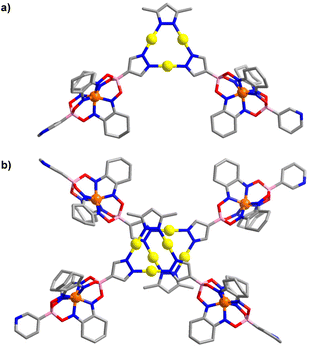 |
| Fig. 3 Molecular structure of complex 3 in the crystal (a), and the relative arrangement of two adjacent complexes (b). Hydrogen atoms are not shown. Color coding: C (gray), N (blue), O (red), B (pink), Au (yellow). | |
With the heterometallic ligands 2 and 3 in hand, we next studied reactions with Pd2+. Based on the geometry of ligand 2 (C3 symmetric ligand with terminal 3-pyridyl groups), we expected that a [Pd6L8]12+-type cage might form.3i,10d,14 When a solution of metalloligands 2 (4 eq.) and [Pd(CH3CN)4](BF4)2 in DMSO-d6 was heated to 60 °C for 15 h, a defined assembly with high apparent symmetry was obtained, as indicated by the NMR spectra (1H NMR and DOSY). The high-resolution mass spectrum confirmed that a [Pd6L8]12+-type cage (4) had formed (Scheme 5 and Fig. 4).
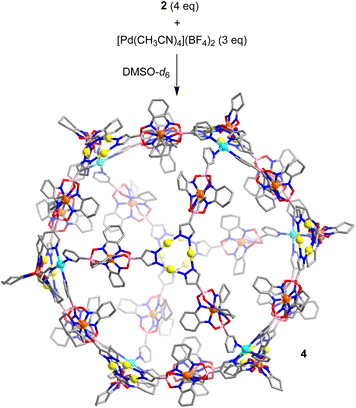 |
| Scheme 5 Synthesis of cage 4. The structure of the product is based on molecular modeling. Color coding: C (gray), N (blue), O (red), B (pink), Au (yellow), Pd (cyan). | |
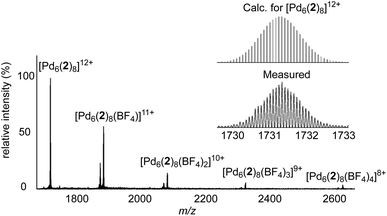 |
| Fig. 4 High-resolution ESI mass spectrum of cage 4. | |
The cationic cage 4 has a molecular weight of 21 kDa. This value is in the range found for small proteins. For example, myoglobin has a molecular weight of 17 kDa.15 Attempts to grow single crystals of 4 were not successful. Molecular modelling showed that cage 4 has a diameter of approximately 4.1 nm.16 This value is in agreement with the solvodynamic diameter of cage 4, which was deduced from the DOSY spectrum (4.3 nm).17
The ditopic N-donor ligand 3 is able to adopt a conformation with a parallel orientation of the coordinate vectors. As a result, it should be well suited to form [M2L4]4+-type cages.18 Thermal equilibration of a mixture of ligand 3 (2 eq.) and [Pd(CH3CN)4](BF4)2 (1 eq.) in DMSO-d6 resulted indeed in the formation of a dinuclear PdII complex with four bridging metalloligands (cage 5, Scheme 6). Cage 5 was characterized by NMR spectroscopy (1H NMR, DOSY) and mass spectrometry (see the ESI, Fig. S17†). According to the results of molecular modelling, the Pd2+ ions in cage 5 are ∼2.4 nm apart from each other. The width of the assembly, as defined by the maximum C⋯C distance, is ∼3.5 nm.
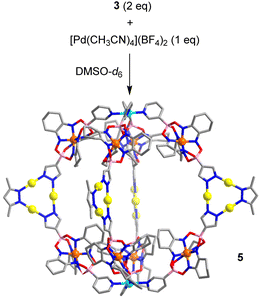 |
| Scheme 6 Synthesis of cage 5. The structure of the product is based on molecular modeling. Color coding: C (gray), N (blue), O (red), B (pink), Au (yellow), Pd (cyan). | |
Conclusions
We have reported the synthesis of heterometallic cages containing three types of coordination compounds: FeII clathrochelate complexes, AuI3(pyrazolate)3 complexes, and [PdII(pyridine)4]2+ complexes. The cages were obtained in a stepwise fashion. First, we prepared di- and tritopic metalloligands by connecting two or three functionalized FeII clathrochelates via AuI3(pyrazolate)3 complexes. The tritopic ligand (2) was then combined with [Pd(CH3CN)4](BF4)2 to give a spherical PdII6FeII24AuI24 coordination cage (4). With a weight of 21 kDa and a diameter of ∼4.1 nm, this complex is one of the largest [Pd6L8]12+-type cages reported to date. The ditopic ligand (3) was used to construct a PdII2FeII8AuI12 coordination cage (5).
In previous work, we had shown that functionalized FeII clathrochelate complexes are well-suited to build large and well-soluble metallosupramolecular structures.4c With the present work, we demonstrate that clathrochelate-based metalloligands can be combined with AuI3(pyrazolate)3 complexes. The inert character of the AuI trimers is important because it enables a final metal-based self-assembly step (here with Pd2+) without ligand scrambling. Furthermore, it is possible to prepare heteroleptic AuI3(pyrazolate)3 complexes containing different pyrazolate ligands. As a proof-of-concept, we have prepared the mixed-ligand complex 3 using a statistical synthesis followed by chromatographic separation. Other low-symmetry ligands based on AuI3(pyrazolate)3 trimers can likely be accessed in a similar fashion. In our opinion, AuI3(pyrazolate)3-based metalloligands have great potential as building blocks in supramolecular chemistry.
Author contributions
N. E. and K. S. initiated the study, N. E. performed the experiments and analyzed the data, F. F.-T. collected and processed the X-ray data, and N. E. and K. S. co-wrote the manuscript. All authors discussed the results and commented on the manuscript.
Conflicts of interest
There are no conflicts to declare.
Acknowledgements
The work was supported by the École Polytechnique Fédérale de Lausanne (EPFL). We thank Dr Ru-Jin Li for his help with the syntheses.
References
- For review articles, see:
(a) L. K. Moree, L. A. V. Faulkner and J. D. Crowley, Heterometallic cages: synthesis and applications, Chem. Soc. Rev., 2024, 53, 25–46 RSC;
(b) M. Hardy and A. Lützen, Better Together: Functional Heterobimetallic Macrocyclic and Cage-like Assemblies, Chem. – Eur. J., 2020, 26, 13332–13346 CrossRef CAS PubMed;
(c) W.-X. Gao, H.-N. Zhang and G.-X. Jin, Supramolecular catalysis based on discrete heterometallic coordination-driven metallacycles and metallacages, Coord. Chem. Rev., 2019, 386, 69–84 CrossRef CAS;
(d) Y.-Y. Zhang, W.-X. Gao, L. Lin and G.-X. Jin, Recent advances in the construction and applications of heterometallic macrocycles and cages, Coord. Chem. Rev., 2017, 344, 323–344 CrossRef CAS;
(e) H. Li, Z.-J. Yao, D. Liu and G.-X. Jin, Multi-component coordination-driven self-assembly toward heterometallic macrocycles and cages, Coord. Chem. Rev., 2015, 293–294, 139–157 CrossRef CAS.
- For examples, see:
(a) J. P. Carpenter, T. K. Ronson, F. J. Rizzuto, T. Héliot, P. Grice and J. R. Nitschke, Incorporation of a Phosphino(pyridine) Subcomponent Enables the Formation of Cages with Homobimetallic and Heterobimetallic Vertices, J. Am. Chem. Soc., 2022, 144, 8467–8473 CrossRef CAS PubMed;
(b) Y. Yang, Y. Wu, J.-H. Jia, X.-Y. Zheng, Q. Zhang, K.-C. Xiong, Z.-M. Zhang and Q.-M. Wang, Enantiopure Magnetic Heterometallic Coordination Cubic Cages [MII8CuII6] (M = Ni, Co), Cryst. Growth Des., 2018, 18, 4555–4561 CrossRef CAS;
(c) G. Liu, M. Zeller, K. Su, J. Pang, Z. Ju, D. Yuan and M. Hong, Controlled Orthogonal Self-Assembly of Heterometal-Decorated Coordination Cages, Chem. – Eur. J., 2016, 22, 17345–17350 CrossRef CAS PubMed;
(d) H. Li, Y.-F. Han, Y.-J. Lin, Z.-W. Guo and G.-X. Jin, Stepwise Construction of Discrete Heterometallic Coordination Cages Based on Self-Sorting Strategy, J. Am. Chem. Soc., 2014, 136, 2982–2985 CrossRef CAS PubMed;
(e) Y. Sakata, S. Hiraoka and M. Shionoya, Site-Selective Ligand Exchange on a Heteroleptic TiIV Complex Towards Stepwise Multicomponent Self-Assembly, Chem. – Eur. J., 2010, 16, 3318–3325 CrossRef CAS PubMed;
(f) S. Hiraoka, Y. Sakata and M. Shionoya, Ti(IV)-Centered Dynamic Interconversion between Pd(II), Ti(IV)-Containing Ring and Cage Molecules, J. Am. Chem. Soc., 2008, 130, 10058–10059 CrossRef CAS PubMed.
- For examples, see:
(a) A. C. Pearcy, L. S. Lisboa, D. Preston, N. B. Page, T. Lawrence, L. J. Wright, C. G. Hartinger and J. D. Crowley, Exploiting reduced-symmetry ligands with pyridyl and imidazole donors to construct a second-generation stimuli-responsive heterobimetallic [PdPtL4]4+ cage, Chem. Sci., 2023, 14, 8615–8623 RSC;
(b) Q.-Y. Zhu, L.-P. Zhou, L.-X. Cai, S.-J. Hu, X.-Z. Li and Q.-F. Sun, Stereocontrolled Self-Assembly of Ln(III)–Pt(II) Heterometallic Cages with Temperature-Dependent Luminescence, Inorg. Chem., 2022, 61, 16814–16821 CrossRef CAS PubMed;
(c) M. Hardy, M. Engeser and A. Lützen, A heterobimetallic tetrahedron from a linear platinum(II)-bis(acetylide) metalloligands, Beilstein J. Org. Chem., 2020, 16, 2701–2708 CrossRef CAS PubMed;
(d) L. S. Lisboa, J. A. Findlay, L. J. Wright, C. G. Hartinger and J. D. Crowley, A Reduced-Symmetry Heterobimetallic [PdPtL4]4+ Cage: Assembly, Guest Binding, and Stimulus-Induced Switching, Angew. Chem., Int. Ed., 2020, 59, 11101–11107 CrossRef CAS PubMed;
(e) A. A. Adeyemo and P. S. Mukherjee, Coordination-driven self-assembly of discrete Ru6–Pt6 prismatic cages, Beilstein J. Org. Chem., 2018, 14, 2242–2249 CrossRef CAS PubMed;
(f) A. J. Metherell and M. D. Ward, Imposing control on self-assembly: rational design and synthesis of a mixed-metal, mixed-ligand coordination cage containing four types of component, Chem. Sci., 2016, 7, 910–915 RSC;
(g) C. Heindl, E. V. Peresypkina, A. V. Virovets, W. Kremer and M. Scheer, Giant Rugby Ball [{CpBnFe(η5-P5)}24Cu96Br96] Derived from Pentaphosphaferrocene and CuBr2, J. Am. Chem. Soc., 2015, 137, 10938–10941 CrossRef CAS PubMed;
(h) S. Sanz, H. M. O'Connor, E. M. Pineda, K. S. Pedersen, G. S. Nichol, O. Mønsted, H. Weihe, S. Piligkos, E. J. L. McInnes, P. J. Lusby and E. K. Brechin, [CrIII8MII6]12+ Coordination Cubes (MII = Cu, Co), Angew. Chem., Int. Ed., 2015, 54, 6761–6764 CrossRef CAS PubMed;
(i) K. Li, L.-Y. Zhang, C. Yan, S.-C. Wei, M. Pan, L. Zhang and C.-Y. Su, Stepwise Assembly of Pd6(RuL3)8 Nanoscale Rhombododecahedral Metal–Organic Cages via Metalloligand Strategy for Guest Trapping and Protection, J. Am. Chem. Soc., 2014, 136, 4456–4459 CrossRef CAS PubMed;
(j) W. J. Ramsay, T. K. Ronson, J. K. Clegg and J. R. Nitschke, Bidirectional Regulation of Halide Binding in a Heterometallic Supramolecular Cube, Angew. Chem., Int. Ed., 2013, 52, 13439–13443 CrossRef CAS PubMed.
- For review articles, see:
(a) F. Li and L. F. Lindoy, Complementarity and Preorganisation in the Assembly of Heterometallic–Organic Cages via the Metalloligand Approach—Recent Advances, Chemistry, 2021, 4, 1439–1456 CrossRef;
(b) F. Li and L. F. Lindoy, Metalloligand Strategies for Assembling Heteronuclear Nanocages – Recent Developments, Aust. J. Chem., 2019, 72, 731–741 CrossRef CAS;
(c) S. M. Jansze and K. Severin, Clathrochelate Metalloligands in Supramolecular Chemistry and Materials Science, Acc. Chem. Res., 2018, 51, 2139–2147 CrossRef CAS PubMed;
(d) J. Gil-Rubio and J. Vicente, Chem. – Eur. J., 2018, 24, 32–46 CrossRef CAS PubMed;
(e) L. Li, D. J. Fanna, N. D. Shepherd and L. F. Lindoy, Constructing coordination nanocages: the metalloligand approach, J. Inclusion Phenom. Macrocyclic Chem., 2015, 82, 3–12 CrossRef CAS;
(f) E. Iengo, P. Cavigli, D. Milano and P. Tecilla, Metal mediated self-assembled porphyrin metallacycles: Synthesis and multipurpose applications, Inorg. Chim. Acta, 2014, 417, 59–78 CrossRef CAS;
(g) S. Durot, J. Taesch and V. Heitz, Multiporphyrinic Cages: Architectures and Functions, Chem. Rev., 2014, 114, 8542–8578 CrossRef CAS PubMed;
(h) G. Kumar and R. Gupta, Molecularly designed architectures – the metalloligand way, Chem. Soc. Rev., 2013, 42, 9403–9453 RSC;
(i) M. C. Das, S. Xiang, Z. Zhang and B. Chen, Functional Mixed Metal–Organic Frameworks with Metalloligands, Angew. Chem., Int. Ed., 2011, 50, 10510–10520 CrossRef CAS PubMed;
(j) E. C. Constable, Expanded ligands—An assembly principle for supramolecular chemistry, Coord. Chem. Rev., 2008, 252, 842–855 CrossRef CAS.
- For examples, see:
(a) H. Ube, K. Endo, H. Sato and M. Shionoya, Synthesis of Hetero-multinuclear Metal Complexes by Site-Selective Redox Switching and Transmetalation on a Homo-multinuclear Complex, J. Am. Chem. Soc., 2019, 141, 10384–10389 CrossRef CAS PubMed;
(b) J. Ferrando-Soria, A. Fernandez, D. Asthana, S. Nawaz, I. Vitorica-Yrezabal, G. F. S. Whitehead, C. A. Muryn, F. Tuna, G. A. Timco, N. D. Burton and R. E. P. Winpenny, A [13]rotaxane assembled via a palladium molecular capsule, Nat. Commun., 2019, 10, 3720 CrossRef PubMed;
(c) H. Brake, E. Peresypkina, C. Heindl, A. V. Virovets, W. Kremer and M. Scheer, From nano-balls to nano-bowls, Chem. Sci., 2019, 10, 2940–2944 RSC;
(d) J. Jiao, C. Tan, Z. Li, Y. Liu, X. Han and Y. Cui, Design and Assembly of Chiral Coordination Cages for Asymmetric Sequential Reactions, J. Am. Chem. Soc., 2018, 140, 2251–2259 CrossRef CAS PubMed;
(e) M. Chen, J. Wang, S. Chakraborty, D. Liu, Z. Jiang, Q. Liu, J. Yan, H. Zhong, G. R. Newcome and P. Wang, Metallosupramolecular 3D assembly of dimetallic Zn4[RuL2]2 and trimetallic Fe2Zn2[RuL2]2, Chem. Commun., 2017, 53, 11087–11090 RSC;
(f) K. Su, F. Jiang, J. Qian, L. Chen, J. Pang, S. M. Bawaked, M. Mokhtar, S. A. Al-Thabaiti and M. Hong, Stepwise Construction of Extra-Large Heterometallic Calixarene-Based Cages, Inorg. Chem., 2015, 54, 3183–3188 CrossRef CAS PubMed;
(g) D. M. Wood, W. Meng, T. K. Ronson, A. R. Stefankiewicz, J. J. M. Sanders and J. R. Nitschke, Guest-Induced Transformation of a Porphyrin-Edged FeII4L6 Capsule into a CuIFeII2L4 Fullerene Receptor, Angew. Chem., Int. Ed., 2015, 54, 3988–3992 CrossRef CAS PubMed;
(h) S. J. Lee, S.-H. Cho, K. L. Mulfort, D. M. Tiede, J. T. Hupp and S. T. Nguyen, Cavity-Tailored, Self-Sorting Supramolecular Catalytic Boxes for Selective Oxidation, J. Am. Chem. Soc., 2008, 130, 16828–16829 CrossRef CAS PubMed;
(i) A. K. Bar, R. Chakrabarty, G. Mostafa and P. S. Mukherjee, Self-Assembly of a Nanoscopic Pt12Fe12 Heterometallic Open Molecular Box Containing Six Porphyrin Walls, Angew. Chem., Int. Ed., 2008, 47, 8455–8459 CrossRef CAS PubMed.
- N. Eren, F. Fadaei-Tirani, R. Scopelliti and K. Severin, Molecular imine cages with π-basic Au3(pyrazolate) faces, Chem. Sci., 2024, 15, 3539–3544 RSC.
- For review articles, see:
(a) J. Zheng, Z. Lu, K. Wu, G.-H. Ning and D. Li, Coinage-Metal-Based Cyclic Trinuclear Complexes with Metal–Metal Interactions: Theories to Experiments and Structures, Chem. Rev., 2020, 120, 9675–9742 CrossRef CAS PubMed;
(b) J. Zheng, H. Yang, M. Xie and D. Li, The π-acidity/basicity of cyclic trinuclear units (CTUs): from a theoretical perspective to potential applications, Chem. Commun., 2019, 55, 7134–7146 RSC;
(c) M. A. Omray, A. A. Mohamed, M. A. Rawashdeh-Omary and J. P. Fackler, Jr., Photophysics of supramolecular binary stacks consisting of electron-rich trinuclear Au(I) complexes and organic electrophiles, Coord. Chem. Rev., 2005, 249, 1372–1381 CrossRef;
(d) A. Burini, A. A. Mohamed and J. P. Fackler, Cyclic Trinuclear Au(I) Compounds: Synthesis, Structures, and Supramolecuar Acid-Base π-Stacks, Comments Inorg. Chem., 2003, 24, 253–280 CrossRef CAS.
- For Au3(pyrazolate)3-based cages with low solubility, see:
(a) M. Veronelli, S. Dechert, A. Schober, S. Demeshko and F. Meyer, 1,1′-Bis(pyrazol-4-yl)ferrocenes: Potential Clip Ligands and Their Supramolecular Structures, Eur. J. Inorg. Chem., 2017, 446–453 CrossRef CAS;
(b) M. Veronelli, S. Dechert, S. Demeshko and F. Meyer, 1,1′-Bis(pyrazol-3-yl)ferrocene: A Clip Ligand That Forms Supramolecular Aggregates and Prismatic Hexanuclear Coinage Metal Complexes, Inorg. Chem., 2015, 54, 6917–6927 CrossRef CAS PubMed;
(c) T. Jozak, Y. Sun, Y. Schmitt, S. Lebedkin, M. Kappes, M. Gerhards and W. R. Thiel, New Hexanuclear Group 11 Pyrazolate Complexes: Synthesis and Photophysical Features, Chem. – Eur. J., 2011, 17, 3384–3389 CrossRef CAS PubMed.
-
Y. Voloshin, I. Belaya and R. Krämer, Cage Metal Complexes – Clathrochelates Revisited, Springer International Publishing, United States, 2017 Search PubMed.
-
(a) S. Sudan, R.-J. Li, S. M. Jansze, A. Platzek, R. Rudolf, G. H. Clever, F. Fadaei-Tirani, R. Scopelliti and K. Severin, Identification of a Heteroleptic Pd6L6L′6 Coordination Cage by Screening of a Virtual Combinatorial Library, J. Am. Chem. Soc., 2021, 143, 1773–1778 CrossRef CAS PubMed;
(b) O. M. Planes, S. M. Jansze, R. Scopelliti, F. Fadaei-Tirani and K. Severin, Two-Step Synthesis of Linear and Bent Dicarboxylic Acid Metalloligands with Lengths of up to 3 nm, Inorg. Chem., 2020, 59, 14544–14548 CrossRef CAS PubMed;
(c) G. Cecot, M. T. Doll, O. M. Planes, A. Ramorini, R. Scopelliti, F. Fadaei-Tirani and K. Severin, Cages vs. Prisms: Controlling the Formation of Metallosupramolecular Architectures with Ligand Side-Chains, Eur. J. Inorg. Chem., 2019, 2972–2976 CrossRef CAS;
(d) S. M. Jansze, D. Ortiz, F. Fadaei Tirani, R. Scopelliti, L. Menin and K. Severin, Inflating face-capped Pd6L8 coordination cages, Chem. Commun., 2018, 54, 9529–9532 RSC;
(e) G. Cecot, M. Marmier, S. Geremia, R. De Zorzi, A. V. Vologzhanina, P. Pattison, E. Solari, F. Fadaei Tirani, R. Scopelliti and K. Severin, The Intricate Structural Chemistry of MII2nLn-Type Assemblies, J. Am. Chem. Soc., 2017, 139, 8371–8381 CrossRef CAS PubMed;
(f) S. M. Jansze, M. D. Wise, A. V. Vologzhanina, R. Scopelliti and K. Severin, PdII2L4-type coordination cages up to three nanometers in size, Chem. Sci., 2017, 8, 1901–1908 RSC;
(g) G. Cecot, B. Alameddine, S. Prior, R. D. Zorzi, S. Geremia, R. Scopelliti, F. T. Fadaei, E. Solari and K. Severin, Large heterometallic coordination cages with gyrobifastigium-like geometry, Chem. Commun., 2016, 52, 11243–11246 RSC;
(h) M. D. Wise, J. J. Holstein, P. Pattison, C. Besnard, E. Solari, R. Scopelliti, G. Bricogne and K. Severin, Large, heterometallic coordination cages based on ditopic metallo-ligands with 3-pyridyl donor groups, Chem. Sci., 2015, 6, 1004–1010 RSC.
- T. Osuga, T. Murase, M. Hoshinoi and M. Fujita, A Tray-Shaped, PdII-Clipped Au3 Complex as a Scaffold for the Modular Assembly of [3×n] Au Ion Clusters, Angew. Chem., Int. Ed., 2014, 53, 11186–11189 CrossRef CAS PubMed.
- For examples of tetranuclear Au4(pyrazolate)4 complexes, see:
(a) R. A. Smith, R. Kulmaczewski and M. A. Halcrow, Ligand-Directed Metalation of a Gold Pyrazolate Cluster, Inorg. Chem., 2023, 62, 9300–9305 CrossRef CAS PubMed;
(b) K. Fujisawa, Y. Ishikawa, Y. Miyashita and K.-i. Okamoto, Pyrazolate-bridged group 11 metal(I) complexes: Substituent effects on the supramolecular structures and physicochemical properties, Inorg. Chim. Acta, 2010, 363, 2977–2989 CrossRef CAS;
(c) G. Yang and R. G. Raptis, Synthesis, structure and properties of tetrameric gold(I) 3,5-di-tert-butyl-pyrazolate, Inorg. Chim. Acta, 2003, 352, 98–104 CrossRef CAS.
- For heterometallic Au/Ag or Au/Cu trimers with different bridging ligands, see:
(a) R. Galassi, M. M. Ghimire, B. M. Otten, S. Ricci, R. N. McDougald, Jr., R. M. Almotawa, D. Alhmoud, J. F. Ivy, A.-M. M. Rawashdeh, V. N. Nesterov, E. W. Reinheimer, L. M. Daniels, A. Burini and M. A. Omary, Cupriphication of gold to sensitize d10–d10 metal–metal bonds and near-unity phosphorescence quantum yields, Proc. Natl. Acad. Sci. U. S. A., 2017, 114, E5042–E5051 CrossRef CAS PubMed;
(b) A. A. Mohamed, R. Galassi, F. Papa, A. Burini and J. P. Fackler, Jr., Gold(I) and Silver(I) Mixed-Metal Trinuclear Complexes: Dimeric Products from the Reaction of Gold(I) Carbeniates or Benzylimidazolates with Silver(I) 3,5-Diphenylpyrazolate, Inorg. Chem., 2006, 45, 7770–7776 CrossRef CAS PubMed;
(c) A. A. Mohamed, R. Galassi, A. Burini and J. P. Fackler, Jr., Mixed-Metal Triangular Trinuclear Complexes: Dimers of Gold-Silver Mixed-Metal Complexes from Gold(I) Carbeniates and Silver(I) 3,5-Diphenylpyrazolates, J. Am. Chem. Soc., 2005, 127, 5012–5013 CrossRef CAS PubMed.
-
(a) Y.-H. Huang, Y.-L. Lu, X.-D. Zhang, C.-H. Liu, J. Ruan, Y.-H. Qin, Z.-M. Cao, J. Jiang, H.-S. Xu and C.-Y. Su, Dynamic Stereochemistry of M8Pd6 Supramolecular Cages Based on Metal-Center Lability for Differential Chiral Induction, Resolution and Recognition, Angew. Chem., Int. Ed., 2024, 63, e202315053 CrossRef CAS PubMed;
(b) Y.-J. Hou, K. Wu, Z.-W. Wei, K. Li, Y.-L. Lu, C.-Y. Zhu, J.-S. Wang, M. Pan, J.-J. Jiang, G.-Q. Li and C.-Y. Su, Design and Enantioresolution of Homochiral Fe(II)–Pd(II) Coordination Cages from Stereolabile Metalloligands: Stereochemical Stability and Enantioselective Separation, J. Am. Chem. Soc., 2018, 140, 18183–18191 CrossRef CAS PubMed;
(c) T. Y. Kim, L. Digal, M. G. Gardiner, N. T. Lucas and J. D. Crowley, Octahedral [Pd6L8]12+ Metallosupramolecular Cages: Synthesis, Structures and Guest-Encapsulation Studies, Chem. – Eur. J., 2017, 23, 15089–15097 CrossRef CAS PubMed;
(d) K. Wu, K. Li, Y.-J. Hou, M. Pan, L.-Y. Zhang, L. Chen and C.-Y. Su, Homochiral D4-symmetric metal–organic cages from stereogenic Ru(II) metalloligands for effective enantioseparation of atropisomeric molecules, Nat. Commun., 2016, 7, 10487 CrossRef CAS PubMed;
(e) T. H. Noh, W. Hong, H. Lee and O.-S. Jung, Indistinguishability and distinguishability between amide and ester moieties in the construction and properties of M6L8 octahedral nanocages, Dalton Trans., 2015, 44, 787–794 RSC;
(f) X.-J. Li, F.-L. Jiang, M.-Y. Wu, S.-Q. Zhang, Y.-F. Zhou and M.-C. Hong, Self-Assembly of Discrete M6L8 Coordination Cages Based on a Conformationally Flexible Tripodal Phosphoric Triamide Ligand, Inorg. Chem., 2012, 51, 4116–4122 CrossRef CAS PubMed;
(g) D. Moon, S. Kang, J. Park, K. Lee, R. P. John, H. Won, G. H. Seong, Y. S. Kim, G. H. Kim, H. Rhee and M. S. Lah, Face-Driven Corner-Linked Octahedral Nanocages: M6L8 Cages Formed by C3-Symmetric Triangular Facial Ligands Linked via C4-Symmetric Square Tetratopic PdII Ions at Truncated Octahedron Corners, J. Am. Chem. Soc., 2006, 128, 3530–3531 CrossRef CAS PubMed.
- J. Zaia, R. S. Annan and K. Biemann, The Correct Molecular Weight for Myoglobin, a Common Calibrant for Mass Spectrometry, Rapid Commun. Mass Spectrom., 1992, 6, 32–36 CrossRef CAS PubMed.
- For a review article about large metal-based assemblies, see: A. V. Virovets, E. Peresypkina and M. Scheer, Structural Chemistry of Giant Metal Based Supramolecules, Chem. Rev., 2021, 121, 14485–14554 CrossRef CAS PubMed.
- S. Ivanova, P. Adamski, E. Köster, L. Schramm, R. Fröhlich and F. Beuerle, Size Determination of Organic Cages by Diffusion NMR Spectroscopy, Chem. – Eur. J., 2024, 30, e202303318 CrossRef CAS PubMed.
- M. Han, D. M. Engelhard and G. H. Clever, Self-assembled coordination cages based on banana-shaped ligands, Chem. Soc. Rev., 2014, 43, 1848–1860 RSC.
|
This journal is © the Partner Organisations 2024 |