Amide-derived enols in enol–Ugi reactions: expanding horizons for peptidomimetic scaffold synthesis†
Received
23rd July 2024
, Accepted 17th September 2024
First published on 18th September 2024
Abstract
A highly efficient enol–Ugi reaction of β,β-diketoamides has been developed using a novel non-heterocyclic amide-stabilised enol. This approach enables a broad reaction scope, affording β-enaminoamide peptidomimetics with constrained conformations due to CH–π interaction and C(sp3)H⋯O hydrogen bonding. Notably, the use of a five-membered cyclic enol is crucial for achieving stable products in excellent yields. This work highlights the potential of the enol–Ugi reaction for constructing diverse peptidomimetic scaffolds.
Introduction
Enols are crucial intermediates in many organic reactions and have been employed to synthesise a wide range of complex molecules, including natural products and pharmaceuticals.1 The reaction of the α position of enols with electrophiles has been widely used to form new C–C and C–heteroatom bonds. However, the reaction of enols through the oxygen atom has been relatively unexplored and is almost exclusively limited to protection reactions and the synthesis of enol ethers.2
In a recent study, we reported an Ugi-type multicomponent reaction that employs electron-deficient heterocyclic enols as both acids and oxygen nucleophiles, resulting in the synthesis of heterocyclic enamine peptidomimetic derivatives.3–8 This reaction leads to the formation of various new bonds, including a C–N bond in the enol position that originally carried the OH group. The extension of this approach to other types of enols could facilitate a wider exploration of the space of biologically relevant privileged structures.9 In particular, the use of amide-stabilised enols could lead to the synthesis of β-enaminoamide peptidomimetics that pose a synthetic challenge.10–13 For example, 3-aminocyclohex-2-en-1-onederivatives have revealed as potent chemokine receptor 2 (CXCR2) antagonists,14 and indeno[1,2-d]pyrido[1,2-a]pyrimidines are inhibitors of receptor tyrosine kinase15 (Fig. 1).
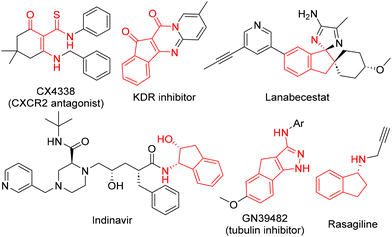 |
| Fig. 1 Biologically active β-enaminoamides and indane derivatives. | |
In contrast with the relatively high number of known stable enols of aldehydes and ketones, enols of carboxylic acids and their derivatives are usually very unstable. In fact, only a few amide-derived enols are known. Rappoport described several enols derived from amides that contain two electron-withdrawing groups, such as β,β-diketoamides and β,β-diester amides.16,17 In these cases, the enol tautomer is stabilised by both electron delocalisation and the formation of intramolecular hydrogen bonds. Cyclic systems, as 1 and 2, are also more prone to exhibit stable enol tautomers, due to better negative charge delocalisation,16 and were found to exist in solution as their more stable tautomeric forms 1a–2a, although enolic forms 1b–2b may be also present in minor proportions (Fig. 2).16,17
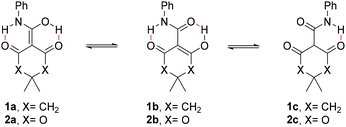 |
| Fig. 2 Six-membered cyclic amide-derived enols. | |
Unfortunately, our prior investigations into the reactions of these enols (1, 2) with imines (3) and isocyanides (4) demonstrated a narrow substrate scope. The reactions of dimedone-derived enol (1) typically afforded complex mixtures containing various degradation products and, in rare instances, the desired enamines (5) in low yields (Fig. 3). Similarly, Medrum's acid-derived enol (2) exhibited no viable reactivity in either dichloromethane or methanol. However, in isopropanol, the reaction with participation of the solvent molecule and subsequent loss of acetone yielded five-component triamide adducts (7), albeit also in low yields.18
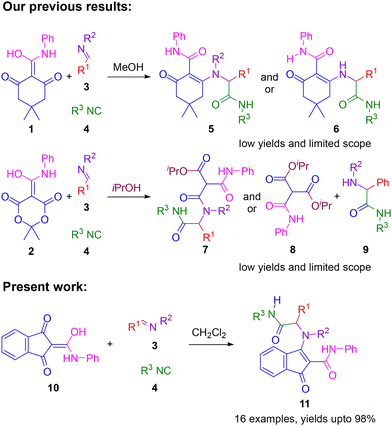 |
| Fig. 3 Enol–Ugi reactions with amide-derived enols. | |
The limited scope and low yields observed in these previous attempts are likely attributable to the inherent instability of the targeted adducts (5, 7). This hypothesis is supported by the isolation of hydrolysis products, such as amides (6, 8, 9), in several instances (unpublished results). Notably, enamines (5) derived from enol (1) exhibited significant instability, which is consistent with the established notion that five-membered cyclic enamines are generally more stable than their six-membered counterparts.19 Based on this understanding, this work focuses on investigating the reactivity of enols derived from five-membered cyclic amides, particularly indandione-derived enol (10). This enol is expected to yield more stable enamines, enabling further exploration of the desired reaction pathway. Importantly, indane is the core structure of many bioactive natural products and pharmaceuticals,20,21 as tubulin polymerisation inhibitor GN39482,22 anti-Parkinson agent rasagiline23 and HIV protease inhibitor indinavir24 (Fig. 1).
Results and discussion
In order to investigate the potential of five-membered cyclic amide-derived enols to participate in enol–Ugi multicomponent reactions, indandione derivative (10) was reacted with (E)-N-benzyl-1-phenylmethanimine (3a) and cyclohexyl isocyanide (4a) in methanol (Scheme 1). After stirring 48 hours at room temperature, the desired enamine (11a) was successfully obtained in a 43% yield, as confirmed by its spectroscopic data. Notably, enol–Ugi adduct (11a) exhibits remarkable stability, both as a solid and in solution.
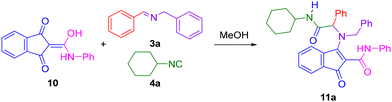 |
| Scheme 1 Enol–Ugi condensation of indanodione-derived enol 10. | |
To further improve the yield of the reaction, different solvents, reaction temperatures and reagents’ molar ratios were examined (Table 1). The reaction in CH2Cl2 at rt with an excess (1.7 equivalents) of enol (10) and imine (3a) resulted in the enol–Ugi adduct 11a almost quantitatively (Table 1, entry 7).
Table 1 Optimisation of enol–Ugi reaction of indandione-derived enol 10
|
|
10 + 3a + 4a → 11a |
|
|
Entry |
Solvent |
10
: 3a : 4a |
Temp. |
Time |
Yield |
N.R.: no reaction.
|
1 |
MeOH |
1 : 1 : 1 |
rt |
48 h |
43% |
2 |
THF |
1 : 1 : 1 |
rt |
48 h |
N.R.a |
3 |
DCM |
1 : 1 : 1 |
rt |
48 h |
49% |
4 |
DCE |
1 : 1.2 : 1 |
50 °C (μW) |
5 min |
39% |
5 |
DCM |
1 : 1.7 : 1 |
rt |
24 h |
78% |
6 |
DCM |
1.7 : 1 : 1 |
rt |
24 h |
25% |
7 |
DCM |
1.7 : 1.7 : 1 |
rt |
24 h |
99% |
According to the accepted mechanism,3,8 the first step in the enol–Ugi reaction of enols, imines and isocyanides is the protonation of the imine by the enol, resulting in formation of the corresponding enolate anion. The iminium cation is attacked by the isocyanide to give a nitrilium cation intermediate that then suffers the nucleophilic attack of the enolate, resulting in a primary adduct. In the case of enol 10 a single enolate (12) is formed, irrespective of which tautomeric species (10a–b) participates in the reaction. The most reactive enolate position reacts with the intermediate nitrilium cation (14) to yield the primary adduct (15). Density functional theory (DFT) calculations on enolate 12 reveal that the oxygen atoms directly bonded to the five-membered ring present greater nucleophilic character than the amide oxygen, as evidenced by their higher nucleophilic Fukui indices (f−)25 (see, ESI, Table S1†). This suggests that these oxygen atoms are more susceptible to nucleophilic attack. The primary adduct (15) then undergoes a conjugate addition – β-elimination rearrangement, ending in the formation of the diamide–enamine adduct (11; Scheme 2). While sharing some similarities with the Mumm rearrangement in the Ugi reaction and the Smiles rearrangement in the Ugi–Smiles condensation,26 this transformation exhibits distinct features. It involves the direct attack of the amine nitrogen to the indandione ring, leading to the formation of a stable five-membered ring enamine (11), possibly through a spirocyclic intermediate (16). The electron-withdrawing effects of the carbonyl and amide groups in the primary adduct (15) facilitate this conjugate addition – β-elimination pathway. DFT calculations reveal a significantly low electron density at C3 and a negative charge on the attacking nitrogen atom (see ESI, Fig. S3†), supporting the feasibility of this nucleophilic attack. Additionally, C3 exhibits the highest electrophilic Fukui index (f+)25 in the molecule (see ESI, Table S3†), further confirming its propensity to undergo nucleophilic attack. This observation is consistent with previous reports on the mechanism of the Michael reaction.27 Moreover, DFT energy calculations indicate that both the spirocyclic intermediate (16) and the final adduct (11) are thermodynamically more stable than the primary adduct (15; see ESI, Table S4†), consistent with a favourable reaction pathway.
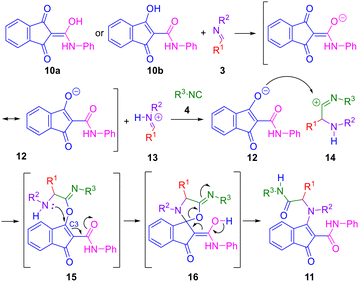 |
| Scheme 2 Proposed mechanism for the enol–Ugi condensation of indandione-derived enol 10. | |
The formation of the spirocyclic intermediate (16) likely results from the interaction of the lowest unoccupied molecular orbital (LUMO) and the next-to-highest occupied molecular orbital (HOMO−1) of the primary adduct (15; Fig. 4). These orbitals have a small energy gap (Table 2) and appropriate geometries and density distribution, making them suitable for forming the spirocyclic intermediate (16). While HOMO and HOMO−1 are close in energy, HOMO−1 has a high electron density localised on the amine nitrogen, suggesting that this nitrogen is well-positioned to act as a nucleophile (see ESI, Table S2†). This observation is confirmed by the highly positive nucleophilic Fukui index (f−) on the amine nitrogen (see ESI, Table S3†) and is consistent with the behaviour of similar molecular systems.3,8
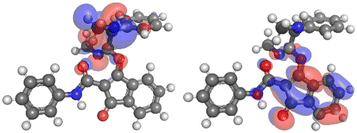 |
| Fig. 4 Orbitals HOMO−1 (left) and LUMO (right) calculated for the primary adduct (15). | |
Table 2 Energy differences between primary adduct FMOs
|
HOMO−1–HOMO |
HOMO−1–LUMO |
HOMO–LUMO |
Energy gap (eV) |
0.53 |
3.45 |
2.92 |
The amide group plays a crucial role in the rearrangement, not only enhancing the electrophilic character of the indandione ring, but also likely facilitating the proton transfer from the attacking amine. A control experiment with the indandione without the amide group (17) resulted in no reaction products, further supporting the importance of the amide in this mechanism (Scheme 3).
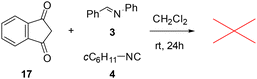 |
| Scheme 3 Control experiment with 1,3-indandione (17). | |
With the optimised conditions established, we explored the reaction's scope using enol 10 with various combinations of imines (3a–l) and isocyanides (4a–c). Gratifyingly, the reaction afforded the desired adducts (11a–p) as the sole product in all cases, with good to excellent yields (Table 3). This demonstrates a broad scope for diverse aliphatic and aromatic amines and isocyanides. However, a limitation emerged with aliphatic aldehydes. While aromatic aldehyde-derived imines participated effectively, aliphatic counterparts either failed to react or resulted in complex reaction mixtures (results not shown). We additionally investigated a four-component reaction variant, omitting the preformation of the imine. Unfortunately, this approach did not lead to the desired products in consistent manner.
Table 3 Synthesis of indanone adducts 11a–pa,b
Procedure for the synthesis of 11a–p: a mixture of enol 10 (1.7 equiv.), imine (3, 1.7 equiv.) and isocyanide (4, 1 equiv.) in CH2Cl2 was stirred at rt for 24 h.
All yields refer to isolated products.
|
|
The spectroscopic analysis of the adducts (11a–p) unveiled intriguing features. Notably, in adduct 11h the H4 aromatic proton signal of the indanone ring exhibited an unexpected upfield shift, appearing as a doublet at 5.81 ppm. Conversely, the signal of the proton on the asymmetric carbon experienced a downfield shift, appearing within the aromatic protons’ region at 7.48 ppm. These unusual chemical shifts can be attributed to the constrained conformation of 11h, which is stabilised by potent intramolecular forces. A hydrogen bond between the indanone carbonyl and the NH of the amide group at indanone position 2 dictates the amide's orientation. Furthermore, an edge-to-face π–π interaction between the indanone aromatic ring and the amine phenyl substituent enforces close proximity between these two aromatic moieties (Fig. 5). The shielding effect on indanone H4 can be attributed to the CH–π interaction. This type of interaction is a well-stablished phenomenon in small molecules.28 Similar outcomes have been observed in other enamino indanones, as reported by Malamidou-Xenikaki.29 Additionally, the unusual chemical shift of the proton on the asymmetric carbon is likely a consequence of a second hydrogen bond between the C
O of the amide group at indanone position 2 and both the NH of the second amide and the proton on the asymmetric carbon.30,31 The acidic character of the proton on the asymmetric carbon in Ugi and enol–Ugi adducts is a well-known feature that has been harnessed for diverse post-condensation transformations.32,33 This relative acidity explains its involvement here in a C(sp3)H⋯O hydrogen bond. Similar chemical shifts for H4 and the proton on the asymmetric carbon were observed for indandione adducts (11i–p) derived from the imines of aromatic amines (3f–l). Bidimensional COSY and HSQC spectra of 11o provided unambiguous assignment of NMR signals (see ESI†).
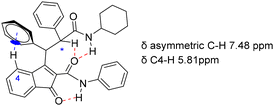 |
| Fig. 5 Intramolecular forces determining 11h1H NMR chemical shifts. | |
The post-condensation transformations of the enol–Ugi adducts may open up new avenues of reactivity, potentially leading to novel applications. To illustrate this concept, indanone adducts 11a,e were efficiently converted to corresponding spiroimidazoleindenes 18a,e with total diastereoselectivity upon treatment with excess base (Scheme 4). A ROESY NMR experiment of 18a was employed to elucidate the relative stereochemistry of the product (ESI†). The observed through-space interaction between the H4 protons of the indandione core and the proton from the asymmetric carbon atom generated in the enol–Ugi reaction suggests an R,R/S,S configuration for 18a. This conclusion is supported by the stronger interaction expected in the transition state leading to R,R/S,S diastereomer compared to the R,S/S,R diastereomer due to the closer proximity of the interacting protons. Similarly, the observed interaction between the proton from the asymmetric carbon and the methylene group is also indicative of the R,R/S,S configuration, as this interaction would be less favourable in the R,S/S,R diastereoisomer (detailed information provided in the ESI†). Interestingly, a similar spirocyclic structure34 is found in the core of β-secretase (BACE1) inhibitor Lanabecestat (Fig. 1). This drug developed by AstraZeneca and Eli Lilly initially showed promise in halting Alzheimer's disease progression during phase II/III clinical trials but was ultimately discontinued due to lack of efficacy and psychiatric side effects.35
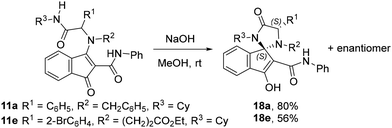 |
| Scheme 4 Spirocyclisation of enol–Ugi adducts 18a,e. | |
Experimental
General methods
All reagents were purchased from commercial sources and used without further purification. Aldehydes, amines, isocyanides, 1,3-indandione and phenyl isocyanate are commercially available. Imines were synthesised according to known procedures.
Liquid reagents were measured using positive-displacement micropipettes with disposable tips and pistons. Organic solvents were evaporated in a rotary evaporator.
Thin layer chromatography (TLC) analyses were performed on Merck silica gel 60 F254 (230–400 mesh) plates, using 254 nm UV light or a mixture of p-anisaldehyde (2.5%), acetic acid (1%) and H2SO4 (3.4%) in 95% ethanol as developer. Flash column chromatography was carried out using a CombiFlash chromatographer from Teledyne Isco.
1H, 13C NMR and bidimensional NMR spectra were acquired on a 500 MHz spectrometer. Chemical shifts (δ) are provided in parts per million (ppm). Multiplicity of signals is abbreviated as: s = singlet, bs = broad singlet, d = doublet, dd = doublet of doublets, t = triplet, dt = doublet of triplets, q = quartet, dq = doublet of quartets, m = multiplet. The assignments of quaternary C, CH, CH2, and CH3 signals were made by using DEPT spectra. IR spectra were recorded in an ATR-IR Affinity-1S Shimadzu. HRMS were recorded on an Agilent LC-QTOF 6520 mass spectrometer. Melting points were determined on melting point apparatus Stuart Scientific SMP3 and are uncorrected.
Computational calculations
All quantum chemical calculations were performed with GAUSSIAN16 series of programs.36 Geometries were optimised in the gas-phase using the B3LYP/6-31G+(d) level of theory. The B3LYP functional combines the Becke's three-parameter non-local hybrid exchange potential with the non-local correlation functional of Lee, Yang, and Parr.37,38 Frequency calculations (at 298.15 K) at the same level of theory were used to confirm the nature of all stationary points as minima and provided values for compute free energies. The most stable conformation was employed to visualise the frontiers orbitals HOMO−1, HOMO and LUMO with Gaussview and Pymol. Moreover, this conformation was employed to carry out NBO with NPA charge calculations39–41 and Fukui indices25 estimation at B3LYP/6-31+g(d,p) level of theory.
Enol–Ugi reactions of 3-hydroxy-1-oxo-N-phenyl-1H-indene-2-carboxamide (10)
To a solution of corresponding imine (3a–l, 0.33 mmol.) in CH2Cl2 (2 mL), isocyanide (4a–c, 0.2 mmol) and enol (10, 0.33 mmol) were successively added. The reaction mixture was stirred at room temperature for 24 hours. Then 10% HCl (1 mL) and water (20 mL) were added, and the mixture was extracted with CH2Cl2 (3 × 20 mL) and dried over Na2SO4. Removal of the solvent and purification by column chromatography (SiO2, gradient from 100% hexanes to hexanes–EtOAc 7
:
3) gave the corresponding enamines 11a–p.
3-(Benzyl(2-(cyclohexylamino)-2-oxo-1-phenylethyl)amino)-1-oxo-N-phenyl-1H-indene-2-carboxamide (11a)
Obtained from 3-hydroxy-1-oxo-N-phenyl-1H-indene-2-carboxamide (10, 0.33 mmol), cyclohexyl isocyanide (4a, 0.2 mmol) and imine (3a, 0.33 mmol) as a red solid (99%); mp 97–99 °C; IR (cm−1) 3279, 3059, 3026, 2926, 2853, 1659, 1593, 1555, 1497, 1443, 1414, 1306, 1248, 1215, 1130, 1078, 1028, 752, 690; 1H NMR (500 MHz, CDCl3) δ 10.43 (s, 1H), 8.00 (s, 1H), 7.70 (d, J = 7.9 Hz, 2H), 7.60 (d, J = 7.1 Hz, 1H), 7.56 (d, J = 7.7 Hz, 1H), 7.47–7.27 (m, 9H), 7.21–7.13 (m, 3H), 7.12–7.04 (m, 3H), 6.72 (s, 1H), 5.42 (d, J = 16.8 Hz, 1H), 5.03 (d, J = 16.9 Hz, 1H), 3.79–3.69 (m, 1H), 1.84–1.73 (m, 2H), 1.72–1.64 (m, 2H), 1.59–1.50 (m, 1H), 1.37–1.12 (m, 5H); 13C NMR (126 MHz, CDCl3) δ 192.10 (C), 173.29 (C), 171.31 (C), 167.00 (C), 162.18 (C), 138.95 (C), 137.98 (C), 136.42 (C), 134.92 (C), 134.84 (C), 132.97 (CH), 132.03 (CH), 129.12 (CH), 129.01 (CH), 128.87 (CH), 128.79 (CH), 128.74 (CH), 127.58 (CH), 126.69 (CH), 124.92 (CH), 123.80 (CH), 122.37 (CH), 120.29 (CH), 70.59 (CH), 55.12 (CH2), 48.78 (CH), 32.59 (CH2), 32.52 (CH2), 25.64 (CH2), 24.76 (CH2), 24.71 (CH2); HRMS (qTOF) calcd for C37H36N3O3: 570.2757. Found: 570.2759.
3-(Benzyl(2-(tert-butylamino)-2-oxo-1-phenylethyl)amino)-1-oxo-N-phenyl-1H-indene-2-carboxamide (11b)
Obtained from 3-hydroxy-1-oxo-N-phenyl-1H-indene-2-carboxamide (10, 0.33 mmol), tert-butyl isocyanide (4b, 0.2 mmol) and imine (3a, 0.33 mmol) as a red solid (75%); mp 102–104 °C; IR (cm−1) 3279, 3026, 2959, 2854, 1656, 1597, 1533, 1494, 1444, 1409, 1306, 1248, 1211, 1123, 1074, 1028, 756, 687; 1H NMR (500 MHz, CDCl3) δ 10.64 (s, 1H), 7.71 (d, J = 8.0 Hz, 2H), 7.64 (bs, 1H), 7.61 (d, J = 7.0 Hz, 1H), 7.48–7.26 (m, 10H), 7.23 (dd, J = 14.1, 6.9 Hz, 2H), 7.19 (d, J = 6.9 Hz, 1H), 7.14 (d, J = 7.4 Hz, 2H), 7.09 (t, J = 7.4 Hz, 1H), 6.74 (s, 1H), 5.45 (d, J = 17.2 Hz, 1H), 5.15 (d, J = 17.3 Hz, 1H), 1.24 (s, 9H); 13C NMR (126 MHz, CDCl3) δ 191.89 (C), 173.72 (C), 167.02 (C), 162.44 (C), 139.09 (C), 137.86 (C), 136.46 (C), 135.18 (C), 135.00 (C), 132.79 (CH), 132.04 (CH), 129.02 (CH), 128.99 (CH), 128.83 (CH), 128.76 (CH), 128.66 (CH), 127.60 (CH), 126.51 (CH), 125.40 (CH), 123.71 (CH), 122.32 (CH), 120.23 (CH), 71.29 (CH), 55.20 (CH2), 51.84 (C), 28.38 (CH3); HRMS (qTOF) calcd for C35H34N3O3: 544.2600. Found: 544.2597.
3-(Benzyl(2-(cyclohexylamino)-1-(furan-2-yl)-2-oxoethyl)amino)-1-oxo-N-phenyl-1H-indene-2-carboxamide (11c)
Obtained from 3-hydroxy-1-oxo-N-phenyl-1H-indene-2-carboxamide (10, 0.33 mmol), cyclohexyl isocyanide (4a, 0.2 mmol) and imine (3b, 0.33 mmol) as a red solid (50%); mp 142–144 °C; IR (cm−1) 3262, 3061, 3030, 2924, 2853, 1668, 1640, 1595, 1537, 1517, 1497, 1443, 1412, 1350, 1323, 1248, 1132, 1014, 889, 750, 736.1H NMR (500 MHz, CDCl3) δ 9.92 (s, 1H), 8.84 (s, 1H), 7.97 (d, J = 7.2 Hz, 1H), 7.73 (d, J = 7.9 Hz, 2H), 7.55 (dd, J = 6.6, 1.7 Hz, 1H), 7.50–7.42 (m, 3H), 7.37 (t, J = 7.9 Hz, 2H), 7.17–7.05 (m, 4H), 6.98 (dd, J = 7.0, 2.8 Hz, 2H), 6.79 (d, J = 3.3 Hz, 1H), 6.52 (s, 1H), 6.43 (dd, J = 3.4, 1.8 Hz, 1H), 5.42 (d, J = 16.1 Hz, 1H), 4.69 (d, J = 15.8 Hz, 1H), 3.88–3.73 (m, 1H), 2.13–0.72 (m, 10H); 13C NMR (126 MHz, CDCl3) δ 192.73 (C), 171.34 (C), 164.74 (C), 161.64 (C), 148.33 (C), 143.31 (CH), 138.80 (C), 138.25 (C), 136.00 (C), 133.48 (CH), 132.01 (CH), 129.09 (CH), 128.52 (CH), 127.68 (CH), 127.56 (CH), 123.99 (CH), 122.37 (CH), 120.11 (CH), 113.11 (CH), 111.21 (CH), 64.56 (CH), 54.00 (CH2), 49.57 (CH), 32.88 (CH2), 32.55 (CH2), 25.72 (CH2), 25.26 (CH2), 25.17 (CH2); HRMS (qTOF) calcd for C35H34N3O4: 560.2549. Found: 560.2530.
3-((Benzo[d][1,3]dioxol-5-ylmethyl)(2-(tert-butylamino)-2-oxo-1-phenylethyl)amino)-1-oxo-N-phenyl-1H-indene-2-carboxamide (11d)
Obtained from 3-hydroxy-1-oxo-N-phenyl-1H-indene-2-carboxamide (10, 0.33 mmol), tert-butyl isocyanide (4b, 0.2 mmol) and imine (3c, 0.33 mmol) as a red solid (79%); mp 100°–102 °C; IR (cm−1) 3285, 2966, 2926, 1661, 1593, 1516, 1499, 1487, 1441, 1414, 1362, 1325, 1308, 1238, 1128, 1036, 925, 804, 752, 691; 1H NMR (500 MHz, CDCl3) δ 10.57 (s, 1H), 7.70 (d, J = 7.7 Hz, 2H), 7.61 (dd, J = 7.3, 1.3 Hz, 1H), 7.58 (s, 1H), 7.49 (d, J = 7.6 Hz, 1H), 7.43 (t, J = 7.4 Hz, 1H), 7.40–7.28 (m, 8H), 7.09 (t, J = 6.8 Hz, 1H), 6.65–6.63 (m, 2H), 6.62 (s, 1H), 6.59 (d, J = 8.0 Hz, 1H), 5.89 (q, J = 1.5 Hz, 2H), 5.34 (d, J = 16.8 Hz, 1H), 5.00 (d, J = 16.9 Hz, 1H), 1.28 (s, 9H); 13C NMR (126 MHz, CDCl3) δ 192.04 (C), 173.56 (C), 167.12 (C), 162.30 (C), 148.12 (C), 147.07 (C), 139.05 (C), 137.94 (C), 135.07 (C), 135.01 (C), 132.88 (CH), 132.04 (CH), 130.31 (C), 129.03 (CH), 128.80 (CH), 128.73 (CH), 125.28 (CH), 123.74 (CH), 122.33 (CH), 120.25 (CH), 120.13 (CH), 108.44 (CH), 107.05 (CH), 101.23 (CH2), 71.19 (CH), 54.97 (CH2), 51.91 (C), 28.45 (CH3); HRMS (qTOF) calcd for C36H34N3O5: 588.2498. Found: 588.2506.
Ethyl 3-((1-(2-bromophenyl)-2-(cyclohexylamino)-2-oxoethyl)(1-oxo-2-(phenylcarbamoyl)-1H-inden-3-yl)amino)propanoate (11e)
Obtained from 3-hydroxy-1-oxo-N-phenyl-1H-indene-2-carboxamide (10, 0.33 mmol), cyclohexyl isocyanide (4a, 0.2 mmol) and imine (3d, 0.33 mmol) as a red solid (53%); mp 97–99 °C; IR (cm−1) 3296, 3065, 2930, 2851, 1726, 1666, 1593, 1518, 1497, 1441, 1306, 1246, 1186, 1130, 1088, 1043, 1024, 943, 891, 752, 690; 1H NMR (500 MHz, CDCl3) δ 10.48 (s, 1H), 7.74–7.55 (m, 5H), 7.54–7.40 (m, 4H), 7.38–7.12 (m, 4H), 7.06 (t, J = 7.4 Hz, 1H), 6.50 (s, 1H), 4.25–3.99 (m, 4H), 3.98–3.87 (m, 1H), 3.10–2.98 (m, 1H), 2.65–2.56 (m, 1H), 2.08–1.97 (m, 1H), 1.90–1.81 (m, 1H), 1.79–1.65 (m, 2H), 1.63–1.53 (m, 2H), 1.50–0.92 (m, 7H); 13C NMR (126 MHz, CDCl3) δ 192.83 (C), 172.97 (C), 171.38 (C), 167.25 (C), 161.46 (C), 139.09 (C), 138.45 (C), 134.84 (C), 134.35 (C), 133.84 (CH), 133.34 (CH), 131.96 (CH), 130.89 (CH), 130.58 (CH), 128.90 (CH), 127.97 (CH), 125.82 (C), 124.16 (CH), 123.55 (CH), 122.36 (CH), 120.18 (CH), 105.46 (C), 71.75 (CH), 61.09 (CH2), 48.94 (CH), 47.17 (CH2), 32.80 (CH2), 32.71 (CH2), 25.61 (CH2), 24.96 (CH2), 24.89 (CH2), 14.12 (CH3); HRMS (qTOF) calcd for C35H3781BrN3O5: 660.1896. Found: 660.1896.
Ethyl 3-((1-(2-bromophenyl)-2-(tert-butylamino)-2-oxoethyl)(1-oxo-2-(phenylcarbamoyl)-1H-inden-3-yl)amino)propanoate (11f)
Obtained from 3-hydroxy-1-oxo-N-phenyl-1H-indene-2-carboxamide (10, 0.33 mmol), tert-butyl isocyanide (4b, 0.2 mmol) and imine (3d, 0.33 mmol) as a red solid (54%); mp 72–73 °C; IR (cm−1) 3288, 3061, 2963, 2924, 2853, 1728, 1688, 1666, 1593, 1520, 1499, 1443, 1364, 1306, 1246, 1186, 1130, 1024, 943, 897, 752, 690, 561; 1H NMR (500 MHz, CDCl3) δ 10.52 (s, 1H), 7.70 (d, J = 7.6 Hz, 2H), 7.65–7.62 (m, 1H), 7.62–7.59 (m, 2H), 7.55 (dd, J = 7.9, 1.7 Hz, 1H), 7.49–7.44 (m, 2H), 7.37 (dt, J = 7.7, 1.3 Hz, 1H), 7.32 (t, J = 7.8 Hz, 2H), 7.23 (dt, J = 7.7, 1.7 Hz, 1H), 7.07 (t, J = 7.4 Hz, 1H), 6.78 (s, 1H), 6.57 (s, 1H), 4.29–4.16 (m, 2H), 4.09–4.01 (m, 2H), 3.04–2.96 (m, 1H), 2.66–2.58 (m, 1H), 1.37 (s, 9H), 1.17 (t, J = 7.1 Hz, 3H); 13C NMR (126 MHz, CDCl3) δ 192.68 (C), 173.14 (C), 170.96 (C), 167.11 (C), 161.71 (C), 139.17 (C), 138.47 (C), 135.10 (C), 134.50 (C), 133.80 (CH), 133.30 (CH), 131.97 (CH), 130.48 (CH), 128.96 (CH), 128.05 (CH), 125.61 (C), 124.23 (CH), 123.55 (CH), 122.40 (CH), 120.10 (CH), 105.56 (C), 71.82 (CH), 61.05 (CH2), 52.04 (C), 47.20 (CH2), 34.63 (CH2), 28.54 (CH3), 14.17 (CH3); HRMS (qTOF) calcd for C33H3581BrN3O5: 634.1740. Found: 634.1737.
Ethyl 3-((2-(cyclohexylamino)-2-oxo-1-(p-tolyl)ethyl)(1-oxo-2-(phenylcarbamoyl)-1H-inden-3-yl)amino)propanoate (11g)
Obtained from 3-hydroxy-1-oxo-N-phenyl-1H-indene-2-carboxamide (10, 0.33 mmol), cyclohexyl isocyanide (4a, 0.2 mmol) and imine (3e, 0.33 mmol) as a red solid (85%); mp 177–180 °C; IR (cm−1) 3289, 2924, 2851, 1732, 1666, 1638, 1595, 1529, 1499, 1443, 1414, 1364, 1306, 1246, 1186, 1157, 1186, 1020, 858, 750, 690; 1H NMR (500 MHz, CDCl3) δ 10.57 (s, 1H), 7.71–7.66 (m, 3H), 7.66–7.62 (m, 1H), 7.51–7.45 (m, 3H), 7.36–7.30 (m, 4H), 7.18 (d, J = 7.8 Hz, 2H), 7.08 (t, J = 7.4 Hz, 1H), 6.42 (s, 1H), 4.37–4.29 (m, 1H), 4.12–4.05 (m, 1H), 4.01 (qd, J = 7.2, 2.3 Hz, 2H), 3.88 (dtq, J = 10.8, 7.0, 3.5 Hz, 1H), 2.84–2.71 (m, 1H), 2.49 (ddd, J = 15.7, 9.5, 4.9 Hz, 1H), 2.35 (s, 3H), 2.04–1.48 (m, 5H), 1.39–1.18 (m, 5H), 1.13 (t, J = 7.1 Hz, 3H); 13C NMR (126 MHz, CDCl3) δ 192.49 (C), 173.49 (C), 172.67 (C), 170.90 (C), 167.40 (C), 161.89 (C), 139.02 (C), 138.04 (C), 133.29 (CH), 132.17 (CH), 131.93 (C), 129.98 (C), 129.85 (CH), 129.26 (C), 129.03 (CH), 128.99 (CH), 124.44 (CH), 123.73 (CH), 122.47 (CH), 120.23 (CH), 70.99 (CH), 61.03 (CH2), 48.90 (CH), 47.21 (CH2), 32.83 (CH2), 32.72 (CH2), 29.85 (CH2), 25.66 (CH2), 24.95 (CH2), 21.32 (CH3), 14.12 (CH3); HRMS (qTOF) calcd for C36H40N3O5: 594.2968. Found: 594.2973.
3-((2-(Cyclohexylamino)-2-oxo-1-phenylethyl)(phenyl)amino)-1-oxo-N-phenyl-1H-indene-2-carboxamide (11h)
Obtained from 3-hydroxy-1-oxo-N-phenyl-1H-indene-2-carboxamide (10, 0.33 mmol), cyclohexyl isocyanide (4a, 0.2 mmol) and imine (3f, 0.33 mmol) as a red solid (95%); mp 160–161 °C; IR (cm−1) 3275, 3063, 3030, 2928, 2851, 1666, 1593, 1537, 1506, 1487, 1445, 1381, 1308, 1248, 1153, 1086, 1023, 804, 750, 710, 690; 1H NMR (500 MHz, CDCl3) δ 10.80 (s, 1H), 7.72 (d, J = 7.9 Hz, 2H), 7.57 (d, J = 7.2 Hz, 1H), 7.51 (s, 1H), 7.39 (d, J = 7.9 Hz, 1H), 7.36–7.27 (m, 8H), 7.27–7.19 (m, 5H), 7.10 (t, J = 7.4 Hz, 1H), 7.06 (dt, J = 7.7, 1.3 Hz, 1H), 5.81 (d, J = 7.7 Hz, 1H), 3.75–3.64 (m, 1H), 1.91–1.85 (m, 1H), 1.73–1.65 (m, 1H), 1.54–1.47 (m, 2H), 1.46–1.40 (m, 1H), 1.37–1.04 (m, 4H), 0.82–0.76 (m, 1H); 13C NMR (126 MHz, CDCl3) δ 193.55 (C), 170.85 (C), 166.54 (C), 162.32 (C), 141.69 (C), 139.46 (C), 138.89 (C), 135.57 (C), 134.27 (C), 132.67 (CH), 131.41 (CH), 129.06 (CH), 128.95 (CH), 128.93 (CH), 128.48 (CH), 128.38 (CH), 128.25 (CH), 127.96 (CH), 125.22 (CH), 123.95 (CH), 121.90 (CH), 120.39 (CH), 105.12 (C), 71.19 (CH), 48.41 (CH), 32.56 (CH2), 32.43 (CH2), 25.61 (CH2), 24.61 (CH2), 24.52 (CH2); HRMS (qTOF) calcd for C36H34N3O3: 556.2600. Found: 556.2603.
3-((2-(tert-Butylamino)-2-oxo-1-phenylethyl)(phenyl)amino)-1-oxo-N-phenyl-1H-indene-2-carboxamide (11i)
Obtained from 3-hydroxy-1-oxo-N-phenyl-1H-indene-2-carboxamide (10, 0.33 mmol), tert-butyl isocyanide (4b, 0.2 mmol) and imine (3f, 0.33 mmol) as a red solid (94%); mp 125–127 °C; IR (cm−1) 3050, 2920, 2851, 1666, 1620, 1585, 1535, 1495, 1439, 1416, 1308, 1242, 1134, 1078, 887, 746, 688; 1H NMR (500 MHz, CDCl3) δ 10.77 (s, 1H), 7.73(d, J = 7.3, 2H), 7.56 (dd, J = 7.3, 1.2 Hz, 1H), 7.39 (s, 1H), 7.38–7.29 (m, 10H), 7.29–7.19 (m, 4H), 7.11 (t, J = 7.4 Hz, 1H), 7.06 (dt, J = 7.7, 1.3 Hz, 1H), 5.81 (d, J = 7.8 Hz, 1H), 1.15 (s, 9H); 13C NMR (126 MHz, CDCl3) δ 193.49 (C), 170.76 (C), 166.41 (C), 162.36 (C), 141.85 (C), 139.44 (C), 138.91 (C), 135.70 (C), 134.28 (C), 132.67 (CH), 131.41 (CH), 129.42 (C), 129.09 (CH), 128.96 (CH), 128.87 (CH), 128.48 (CH), 128.36 (CH), 127.89 (CH), 125.20 (CH), 123.97 (CH), 121.91 (CH), 120.35 (CH), 105.16 (C), 71.73 (CH), 51.45 (C), 28.36 (CH3); HRMS (qTOF) calcd for C34H32N3O3: 530.2444. Found: 530.2425.
3-((2-(Cyclohexylamino)-2-oxo-1-(p-tolyl)ethyl)(phenyl)amino)-1-oxo-N-phenyl-1H-indene-2-carboxamide (11j)
Obtained from 3-hydroxy-1-oxo-N-phenyl-1H-indene-2-carboxamide (10, 0.33 mmol), cyclohexyl isocyanide (4a, 0.2 mmol) and imine (3g, 0.33 mmol) as a red solid (89%); mp 98–99 °C; IR (cm−1) 3306, 3057, 2928, 2851, 1666, 1639, 1593, 1537, 1510, 1487, 1443, 1383, 1348, 1308, 1248, 1153, 1086, 1020, 869, 752, 690; 1H NMR (500 MHz, CDCl3) δ 10.79 (s, 1H), 7.71 (d, J = 7.7 Hz, 2H), 7.56 (dd, J = 7.3, 1.2 Hz, 1H), 7.47 (s, 1H), 7.38–7.27 (m, 7H), 7.27–7.21 (m, 4H), 7.10 (t, J = 6.8 Hz, 1H), 7.07–7.02 (m, 3H), 5.77 (d, J = 7.8 Hz, 1H), 3.74–3.64 (m, 1H), 2.27 (s, 3H), 1.91–183 (m, 1H), 1.74–1.60 (m, 2H), 1.55–1.38 (m, 2H), 1.38–1.05 (m, 4H), 0.91–0.76 (m, 1H); 13C NMR (126 MHz, CDCl3) δ 193.49 (C), 170.83 (C), 166.71 (C), 162.34 (C), 141.74 (C), 139.44 (C), 138.94 (C), 137.78 (C), 134.34 (C), 132.60 (CH), 132.48 (C), 131.37 (CH), 129.08 (CH), 129.05 (CH), 128.91 (CH), 128.46 (CH), 128.30 (CH), 125.21 (CH), 123.90 (CH), 121.85 (CH), 120.37 (CH), 105.02 (C), 71.06 (CH), 48.38 (CH), 32.59 (CH2), 32.45 (CH2), 25.61 (CH2), 24.62 (CH2), 24.53 (CH2), 21.18 (CH3); HRMS (qTOF) calcd for C37H36N3O3: 570.2757. Found: 570.2760.
3-((2-(tert-Butylamino)-1-(4-methoxyphenyl)-2-oxoethyl)(phenyl)amino)-1-oxo-N-phenyl-1H-indene-2-carboxamide (11k)
Obtained from 3-hydroxy-1-oxo-N-phenyl-1H-indene-2-carboxamide (10, 0.33 mmol), tert-butyl isocyanide (4b, 0.2 mmol) and imine (3h, 0.33 mmol) as a red solid (76%); mp 167–169 °C; IR (cm−1) 3354, 3065, 2959, 2926, 2849, 1678, 1645, 1589, 1533, 1508, 1491, 1452, 1435, 1300, 1182, 1155, 1132, 1045, 962, 750, 731, 532; 1H NMR (500 MHz, CDCl3) δ 10.80 (s, 1H), 7.73 (d, J = 8.0 Hz, 2H), 7.56 (d, J = 7.2 Hz, 1H), 7.45 (s, 1H), 7.38–7.21 (m, 10H), 7.10 (t, J = 7.4 Hz, 1H), 7.05–6.97 (m, 2H), 6.75 (d, J = 8.4 Hz, 2H), 5.66 (d, J = 7.8 Hz, 1H), 3.74 (s, 3H), 1.16 (s, 9H); 13C NMR (126 MHz, CDCl3) δ 193.26 (C), 170.49 (C), 166.97 (C), 162.53 (C), 159.29 (C), 141.83 (C), 139.27 (C), 139.05 (C), 134.49 (C), 132.50 (CH), 131.37 (CH), 130.65 (CH), 129.08 (CH), 128.94 (CH), 128.69 (CH), 128.59 (CH), 127.26 (C), 125.22 (CH), 123.86 (CH), 121.81 (CH), 120.34 (CH), 113.76 (CH), 104.49 (C), 71.20 (CH), 55.37 (CH3), 51.50 (C), 28.38 (CH3); HRMS (qTOF) calcd for C35H34N3O4: 560.2549. Found: 560.2543.
3-((1-(Benzo[d][1,3]dioxol-5-yl)-2-(tert-butylamino)-2-oxoethyl)(phenyl)amino)-1-oxo-N-phenyl-1H-indene-2-carboxamide (11l)
Obtained from 3-hydroxy-1-oxo-N-phenyl-1H-indene-2-carboxamide (10, 0.33 mmol), tert-butyl isocyanide (4b, 0.2 mmol) and imine (3i, 0.33 mmol) as a red solid (64%); mp 178–179 °C; IR (cm−1) 3321, 3281, 3248, 1692, 1668, 1633, 1595, 1546, 1514, 1485, 1379, 1312, 1251, 1155, 1037, 914, 887, 804, 750, 731, 687; 1H NMR (500 MHz, CDCl3) δ 10.80 (s, 1H), 7.73 (d, J = 8.0 Hz, 2H), 7.56 (dd, J = 7.3, 1.2 Hz, 1H), 7.40 (s, 1H), 7.38–7.33 (m, 4H), 7.33–7.27 (m, 2H), 7.11 (tt, J = 7.3, 1.2 Hz, 1H), 7.06 (s, 1H), 7.03 (dt, J = 7.7, 1.3 Hz, 1H), 6.85–6.76 (m, 2H), 6.65 (d, J = 8.1 Hz, 1H), 5.89 (d, J = 1.4 Hz, 1H), 5.88 (d, J = 1.5 Hz, 2H), 5.69 (d, J = 7.8 Hz, 1H), 1.15 (s, 9H); 13C NMR (126 MHz, CDCl3) δ 193.35 (C), 170.54 (C), 166.69 (C), 162.46 (C), 147.69 (C), 147.36 (C), 141.73 (C), 139.29 (C), 138.98 (C), 134.36 (C), 132.59 (CH), 131.42 (CH), 129.09 (CH), 129.08 (CH), 128.97 (CH), 128.63 (CH), 125.21 (CH), 123.92 (CH), 122.81 (CH), 121.86 (CH), 120.35 (CH), 109.95 (CH), 108.05 (CH), 104.66 (C), 101.27 (CH2), 71.33 (CH), 51.52 (C), 28.36 (CH3); HRMS (qTOF) calcd for C35H32N3O5: 574.2342. Found: 574.2349.
3-((2-(tert-Butylamino)-2-oxo-1-(4-(trifluoromethyl)phenyl)ethyl)(phenyl)amino)-1-oxo-N-phenyl-1H-indene-2-carboxamide (11m)
Obtained from 3-hydroxy-1-oxo-N-phenyl-1H-indene-2-carboxamide (10, 0.33 mmol), tert-butyl isocyanide (4b, 0.2 mmol) and imine (3j, 0.33 mmol) as a red solid (98%); mp 129–130 °C; IR (cm−1) 3325, 3065, 2963, 2920, 2851, 1703, 1668, 1643, 1599, 1545, 1514, 1485, 1383, 1323, 1113, 1067, 870, 746, 712, 687, 555; 1H NMR (500 MHz, CDCl3) δ 10.74 (s, 1H), 7.81 (s, 1H), 7.72 (d, J = 8.0 Hz, 2H), 7.59 (d, J = 7.2 Hz, 1H), 7.53 (d, J = 8.1 Hz, 2H), 7.44 (d, J = 8.1 Hz, 2H), 7.39–7.23 (m, 9H), 7.12 (t, J = 8.4 Hz, 2H), 5.99 (d, J = 7.7 Hz, 1H), 1.12 (s, 9H); 13C NMR (126 MHz, CDCl3) δ 193.74 (C), 170.91 (C), 165.36 (C), 162.06 (C), 141.71 (C), 140.37 (C), 139.62 (C), 138.64 (C), 133.85 (C), 133.02 (CH), 131.64 (CH), 130.06 (C), 129.81 (C), 129.21 (CH), 129.15 (CH), 128.59 (CH), 128.52 (CH), 127.55 (CH), 125.33 (CH), 125.30 (CH), 125.27 (CH) 125.01 (CH), 124.24 (CH), 122.22 (CH), 120.37 (CH), 106.15 (C), 71.42 (CH), 51.45 (C), 28.30 (CH3); HRMS (qTOF) calcd for C35H31F3N3O3: 598.2318. Found: 598.2321.
3-((2-((2,6-Dimethylphenyl)amino)-2-oxo-1-phenylethyl)(phenyl)amino)-1-oxo-N-phenyl-1H-indene-2-carboxamide (11n)
Obtained from 3-hydroxy-1-oxo-N-phenyl-1H-indene-2-carboxamide (10, 0.33 mmol), 2,6-dimethylphenyl isocyanide (4c, 0.2 mmol) and imine (3f, 0.33 mmol) as a red solid (68%); mp 166–178 °C; IR (cm−1) 3269, 3061, 2922, 2853, 1672, 1638, 1595, 1568, 1545, 1492, 1381, 1310, 1155, 1078, 900, 754, 691, 557.1H NMR (500 MHz, CDCl3) δ 10.92 (s, 1H), 9.49 (s, 1H), 7.72 (d, J = 8.5 Hz, 2H), 7.71 (s, 1H), 7.60 (d, J = 7.1 Hz, 1H), 7.44 (d, J = 8.1 Hz, 2H), 7.40–7.26 (m, 11H), 7.15–7.08 (m, 2H), 7.06–6.96 (m, 3H), 5.95 (d, J = 7.7 Hz, 1H), 1.99 (s, 6H); 13C NMR (126 MHz, CDCl3) δ 193.86 (C), 171.24 (C), 165.54 (C), 162.14 (C), 141.87 (C), 139.69 (C), 138.58 (C), 136.08 (C), 135.16 (C), 134.41 (C), 134.05 (C), 132.95 (CH), 131.57 (CH), 129.38 (CH), 129.05 (CH), 128.66 (CH), 128.53 (CH), 128.21 (CH), 128.15 (CH), 128.01 (CH), 127.91 (CH), 126.98 (CH), 125.32 (CH), 124.21 (CH), 122.13 (CH), 120.53 (CH), 105.89 (C), 71.47 (CH), 18.50 (CH3); HRMS (qTOF) calcd for C38H32N3O3: 578.2444. Found: 578.2432.
3-((4-Chlorophenyl)(2-(cyclohexylamino)-2-oxo-1-phenylethyl)amino)-1-oxo-N-phenyl-1H-indene-2-carboxamide (11o)
Obtained from 3-hydroxy-1-oxo-N-phenyl-1H-indene-2-carboxamide (10, 0.33 mmol), cyclohexyl isocyanide (4a, 0.2 mmol) and imine (3k, 0.33 mmol) as a red solid (48%); mp 89–92 °C; IR (cm−1) 3302, 3059, 2924, 2851, 1666, 1639, 1595, 1537, 1510, 1485, 1445, 1377, 1307, 1246, 1087, 1012, 752, 690; 1H NMR (500 MHz, CDCl3) δ 10.76 (s, 1H), 7.71 (d, J = 8.0 Hz, 2H), 7.58 (d, J = 7.2 Hz, 1H), 7.46 (s, 1H), 7.39 (d, J = 7.6 Hz, 1H), 7.37–7.33 (m, 3H), 7.33–7.31 (m, 2H), 7.30–7.25 (m, 3H), 7.25–7.20 (m, 4H), 7.17–7.07 (m, 2H), 5.95 (d, J = 7.7 Hz, 1H), 3.77–3.70 (m, 1H), 1.95–0.77 (m, 10H); 13C NMR (126 MHz, CDCl3) δ 193.64 (C), 170.51 (C), 166.56 (C), 162.12 (C), 140.31 (C), 139.33 (C), 138.76 (C), 135.30 (C), 134.16 (C), 134.00 (C), 132.94 (CH), 131.59 (CH), 129.33 (CH), 129.10 (CH), 129.07 (CH), 128.85 (CH), 128.56 (CH), 128.18 (CH), 125.10 (CH), 124.11 (CH), 122.17 (CH), 120.42, (CH) 105.73 (C), 71.09 (CH), 48.48 (CH), 32.60 (CH2), 32.54 (CH2), 29.85 (CH2), 25.60 (CH2), 24.59 (CH2), 24.53 (CH2); HRMS (qTOF) calcd for C36H33ClN3O3: 590.2210. Found: 590.2203.
3-((2-(Cyclohexylamino)-2-oxo-1-phenylethyl)(4-methoxyphenyl)amino)-1-oxo-N-phenyl-1H-indene-2-carboxamide (11p)
Obtained from 3-hydroxy-1-oxo-N-phenyl-1H-indene-2-carboxamide (10, 0.33 mmol), cyclohexyl isocyanide (4a, 0.2 mmol) and imine (3l, 0.33 mmol) as a red solid (90%); mp 104–106 °C; IR (cm−1) 3296, 3059, 2926, 2851, 1663, 1639, 1593, 1545, 1504, 1492, 1440, 1384, 1298, 1247, 1174, 1107, 839, 804, 754, 692; 1H NMR (500 MHz, CDCl3) δ 10.86 (s, 1H), 7.72 (d, J = 7.4 Hz, 2H), 7.57 (d, J = 7.2 Hz, 1H), 7.54 (s, 1H), 7.38–7.30 (m, 6H), 7.26–7.18 (m, 5H), 7.13–7.05 (m, 2H), 6.75 (d, J = 9.1 Hz, 2H), 5.81 (d, J = 7.8 Hz, 1H), 3.79 (s, 3H), 3.76–3.78 (m, 1H), 1.94–1.84 (m, 2H), 1.73–1.65 (m, 1H), 1.58–1.46 (m, 2H), 1.36–1.08 (m, 4H), 0.96–0.85 (m, 1H); 13C NMR (126 MHz, CDCl3) δ 193.33 (C), 170.58 (C), 166.67 (C), 162.53 (C), 159.71 (C), 139.32 (C), 139.02 (C), 135.53 (C), 134.60 (C), 134.42 (C), 132.63 (CH), 131.36 (CH), 129.67 (CH), 129.03 (CH), 128.43 (CH), 128.00 (CH), 125.30 (CH), 123.82 (CH), 121.80 (CH), 120.36 (CH), 114.00 (CH), 104.08 (C), 71.23 (CH), 60.53 (CH3), 55.63 (CH), 48.38 (CH2), 32.63 (CH2), 32.56 (CH2), 25.62 (CH2), 24.56 (CH2); HRMS (qTOF) calcd for C37H36N3O4: 586.2706. Found: 586.2713.
Synthesis of spiroimidazolidines 18a and 18e
To a stirred solution of (11a,e, 0.1 mmol) in EtOH (2 mL), 1.5 equivalents of an ethanolic solution of 0.1 M NaOH were added and the reaction mixture was kept at room temperature for 1 hour. An additional 0.5 equivalents of 0.1 M NaOH were added and stirring continued for another 30 minutes. The reaction was quenched with 1 mL 10% HCl and 20 mL of H2O, followed by extraction with EtOAc (3 × 15 mL). The combined organic phases were dried over Na2SO4 and the solvent was eliminated in the rotatory evaporator. Purification by column chromatography (SiO2, gradient from 100% hexanes to hexanes–EtOAc 6
:
4) gave the corresponding spiroimidazolidines (18a,e).
1-Benzyl-3-cyclohexyl-3′-hydroxy-4-oxo-N,5-diphenylspiro[imidazolidine-2,1′-indene]-2′-carboxamide (18a)
Obtained from 11a as a green solid (80%); mp 95–96 °C; IR (cm−1) 3288, 3063, 3030, 2922, 2853, 1697, 1538, 1595, 1576, 1537, 1445, 1415, 1361, 1294, 1242, 1141, 1076, 1028, 804, 752; 1H NMR (500 MHz, CDCl3) δ 8.77 (s, 1H), 7.66–7.61 (m, 1H), 7.58–7.50 (m, 3H), 7.46 (d, J = 6.8 Hz, 2H), 7.43–7.33 (m, 7H), 7.17 (t, J = 7.2 Hz, 1H), 7.03 (t, J = 7.3 Hz, 1H), 6.95 (t, J = 7.5 Hz, 2H), 6.59 (d, J = 7.4 Hz, 2H), 4.84 (s, 1H), 3.80 (d, J = 13.3 Hz, 1H), 3.41 (d, J = 13.4 Hz, 1H), 2.74 (tt, J = 12.2, 3.9 Hz, 1H), 2.24 (qd, J = 12.5, 3.8 Hz, 1H), 1.97 (qd, J = 12.3, 4.0 Hz, 1H), 1.65–1.47 (m, 3H), 1.10–0.97 (m, 3H), 0.91–0.82 (m, 2H); 13C NMR (126 MHz, CDCl3) δ 173.00 (C), 170.52 (C), 166.30 (C), 143.93 (C), 137.18 (C), 136.86 (C), 136.27 (C), 136.22 (C), 130.62 (CH), 130.43 (CH), 129.25 (CH), 129.11 (CH), 128.86 (CH), 128.82 (CH), 128.79 (CH), 128.00 (CH), 127.73 (CH), 124.72 (CH), 124.46 (CH), 121.99 (CH), 120.70 (CH), 102.33 (C), 87.56 (C), 69.51 (CH), 54.15 (CH), 53.16 (CH2), 30.08 (CH2), 29.41 (CH2), 26.23 (CH2), 26.12 (CH2), 25.11 (CH2); HRMS (qTOF) calcd for C37H36N3O5: 570.2757. Found: 570.2726.
Ethyl 3-(4-(2-bromophenyl)-1-cyclohexyl-3′-hydroxy-5-oxo-2′-(phenylcarbamoyl)spiro[imidazolidine-2,1′-inden]-3-yl)propanoate (18e)
Obtained from 11e as a green solid (56%); mp 89–90 °C; IR (cm−1) 3289, 3057, 2926, 2851, 1732, 1705, 1635, 1595, 1576, 1535, 1443, 1414, 1362, 1298, 1240, 1190, 1141, 1026, 690, 632, 534; 1H NMR (500 MHz, CDCl3) δ 8.93 (s, 1H), 7.69 (d, J = 8.3 Hz, 1H), 7.56–7.49 (m, 3H), 7.47–7.41 (m, 3H), 7.40–7.34 (m, 3H), 7.29 (dt, J = 7.6, 1.8 Hz, 1H), 7.16 (t, J = 7.4 Hz, 1H), 5.48 (s, 1H), 3.81–3.73 (m, 2H), 2.92–2.84 (m, 1H), 2.85–2.77 (m, 1H), 2.71 (dt, J = 13.7, 7.6 Hz, 1H), 2.26–2.15 (m, 1H), 2.11–1.90 (m, 4H), 1.64 (dq, J = 12.6, 2.7 Hz, 1H), 1.60–1.47 (m, 2H), 1.47 (d, J = 12.3 Hz, 1H), 1.14–1.03 (m, 1H), 0.99 (t, J = 7.2 Hz, 3H), 0.94–0.83 (m, 2H); 13C NMR (126 MHz, CDCl3) δ 173.69 (C), 171.16 (C), 170.21 (C), 166.54 (C), 144.20 (C), 137.55 (C), 136.80 (C), 135.64 (C), 133.59 (CH), 131.09 (CH), 130.55 (CH), 130.47 (CH), 130.00 (CH), 129.26 (CH), 128.60 (CH), 126.30 (C), 124.80 (CH), 124.13 (CH), 122.14 (CH), 120.62 (CH), 101.52 (C), 88.10 (C), 68.11 (CH), 60.72 (CH2), 54.69 (CH), 45.14 (CH2), 33.59 (CH2), 30.14 (CH2), 29.42 (CH2), 26.24 (CH2), 26.11 (CH2), 25.09 (CH2), 21.19 (CH2), 13.98 (CH3); HRMS (qTOF) calcd for C35H37BrN3O5: 658.1917. Found: 658.1888.
Conclusions
In conclusion, the reaction of 1,3-dioxo-N-phenyl-2,3-dihydro-1H-indene-2-carboxamide (10) with imines (3a–l) and isocyanides (4a–c) readily leads to the selective formation of indano β-enaminoamides (11a–p) in high yields. This highly convergent process occurs in a single reaction vessel, at ambient temperature, with excellent atom economy. Importantly, this represents the first successful documented example of an enol–Ugi reaction involving a non-heterocyclic amide-stabilised enol. Overall, this methodology provides an efficient approach for synthesising conformationally restrained retro-peptidic subunits that are challenging to obtain using other synthetic methods.
Ongoing research will focus on exploring the scope of the reaction by employing indandiones with different substituents on the aromatic ring. Additionally, the incorporation of various amide groups into the indanone scaffold will be investigated to modulate the reactivity and properties of the resulting products. Furthermore, post-condensation transformations aimed at generating polycyclic compounds with potential biological interest will be explored. These studies will contribute to further expanding the applicability of this reaction and unlocking its full potential for the synthesis of complex and biologically relevant molecules.
Data availability
Data will be made available on request.
Conflicts of interest
There are no conflicts to declare.
Acknowledgements
We thank financial support from Junta de Extremadura and the European Regional Development Fund (ERDF) (GR21123). J. L. R. thanks Fundación Valhondo for a predoctoral fellowship.
References
-
Enols, ed. Z. Rappoport, John Wiley & Sons Ltd, 1990. DOI:10.1002/9780470772294.
-
Organic Mechanisms, ed. M. Harmata, Springer Berlin Heidelberg, 2010, pp. 487–517. DOI:10.1007/978-3-642-03651-4_12.
- T. G. Castellano, A. G. Neo, S. Marcaccini and C. F. Marcos, Org. Lett., 2012, 14, 6218–6221, DOI:10.1021/ol302976g.
- J. L. Ramiro, A. G. Neo and C. F. Marcos, Org. Biomol. Chem., 2022, 20, 5293–5307, 10.1039/d2ob00518b.
- A. Bornadiego, A. G. Neo and C. F. Marcos, Molecules, 2021, 26, 1287–1305, DOI:10.3390/molecules26051287.
- A. G. Neo and C. F. Marcos, Proceedings, 2019, 9, 6, DOI:10.3390/ecsoc-22-05864.
- A. G. Neo, T. G. Castellano and C. F. Marcos, ARKIVOC, 2017, 21–31, DOI:10.3998/ark.5550190.p009.775.
- A. G. Neo, T. G. Castellano and C. F. Marcos, Synthesis, 2015, 2431–2438, DOI:10.1055/s-0034-1380436.
- T. B. Poulsen, Acc. Chem. Res., 2021, 54, 1830–1842, DOI:10.1021/acs.accounts.0c00851.
- S. Perrone, M. Capua, G. Cannazza, A. Salomone and L. Troisi, Tetrahedron Lett., 2016, 57, 1421–1424, DOI:10.1016/j.tetlet.2016.02.035.
- P. Neupane, X. Li, J. H. Jung, Y. R. Lee and S. H. Kim, Tetrahedron, 2012, 68, 2496–2508, DOI:10.1016/j.tet.2012.01.060.
- Y. O. Bouone, A. Bouzina, N.-E. Aouf and M. Ibrahim-Ouali, Res. Chem. Intermed., 2023, 49, 1349–1368, DOI:10.1007/s11164-022-04939-8.
- Z. Y. Gu, X. Wang, J. J. Cao, S. Y. Wang and S. J. Ji, Eur. J. Org. Chem., 2015, 4699–4709, DOI:10.1002/ejoc.201500458.
- W. Dai, W. Chen, B. Debnath, Y. Wu and N. Neamati, ChemMedChem, 2018, 13, 916–930, DOI:10.1002/cmdc.201800027.
- M. Tsanakopoulou, T. Cottin, A. Buttner, V. Sarli, E. Malamidou-Xenikaki, S. Spyroudis and A. Giannis, ChemMedChem, 2008, 3, 429–433, DOI:10.1002/cmdc.200700306.
- J. K. Mukhopadhyaya, S. Sklenák and Z. Rappoport, J. Am. Chem. Soc., 2000, 122, 1325–1336, DOI:10.1021/ja992059f.
- J. Song, M. Mishima and Z. Rappoport, Org. Lett., 2007, 9, 4307–4310, DOI:10.1021/ol7018554.
-
A. G. Neo, T. G. Castellano, J. Ramiro and C. F. Marcos, Amide-stabilized enols in the enol-Ugi reaction: a five-component synthesis of triamides, in 25th International Electronic Conference on Synthetic Organic Chemistry (Published in Chemistry Proceedings 2022, 8, 102), MDPI2021. DOI:10.3390/ecsoc-25-11665.
- Y. Li, L. Zhang and S. Luo, ACS Omega, 2022, 7, 6354–6374, DOI:10.1021/acsomega.1c06945.
- N. Ahmed, Nat. Prod. Chem., 2016, 51, 383–434, DOI:10.1016/b978-0-444-63932-5.00008-5.
- P. Prasher and M. Sharma, ChemistrySelect, 2021, 6, 2658–2677, DOI:10.1002/slct.202100177.
- H. Minegishi, Y. Futamura, S. Fukashiro, M. Muroi, M. Kawatani, H. Osada and H. Nakamura, J. Med. Chem., 2015, 58, 4230–4241, DOI:10.1021/acs.jmedchem.5b00035.
- V. Oldfield, G. M. Keating and C. M. Perry, Drugs, 2007, 67, 1725–1747, DOI:10.2165/00003495-200767120-00006.
- T. R. Cressey, N. Plipat, F. Fregonese and K. Chokephaibulkit, Expert Opin. Drug Metab. Toxicol., 2007, 3, 347–361, DOI:10.1517/17425255.3.3.347.
- R. G. Parr and W. Yang, J. Am. Chem. Soc., 1984, 106, 4049–4050, DOI:10.1021/ja00326a036.
- N. Cheron, R. Ramozzi, L. El Kaim, L. Grimaud and P. Fleurat-Lessard, J. Org. Chem., 2012, 77, 1361–1366, DOI:10.1021/jo2021554.
- C. Giraldo, S. Gomez, F. Weinhold and A. Restrepo, ChemPhysChem, 2016, 17, 2022–2034, DOI:10.1002/cphc.201600166.
- J. Z. Liu, H. D. Sun, L. Chen and G. Ding, J. Nat. Prod., 2023, 86, 2238–2245, DOI:10.1021/acs.jnatprod.3c00434.
- E. Malamidou-Xenikaki, S. Spyroudis, M. Tsanakopoulou and H. Krautscheid, J. Org. Chem., 2008, 73, 8392–8397, DOI:10.1021/jo8014903.
- C. R. Jones, P. K. Baruah, A. L. Thompson, S. Scheiner and M. D. Smith, J. Am. Chem. Soc., 2012, 134, 12064–12071, DOI:10.1021/ja301318a.
- A. M. Vibhute, U. Deva Priyakumar, A. Ravi and K. M. Sureshan, Chem. Commun., 2018, 54, 4629–4632, 10.1039/c8cc01653d.
- A. G. Neo, J. L. Ramiro, M. Garcia-Valverde, J. Diaz and C. F. Marcos, Mol. Divers., 2024, 28, 335–418, DOI:10.1007/s11030-023-10641-7.
- A. Ghoshal, M. D. Ambule, A. Yadav and A. K. Srivastava, Asian J. Org. Chem., 2021, 10, 315–333, DOI:10.1002/ajoc.202000648.
- U. Grošelj, A. Beck, W. B. Schweizer and D. Seebach, Helv. Chim. Acta, 2014, 97, 751–796, DOI:10.1002/hlca.201400110.
- A. M. Wessels, P. N. Tariot, J. A. Zimmer, K. J. Selzler, S. M. Bragg, S. W. Andersen, J. Landry, J. H. Krull, A. M. Downing, B. A. Willis, S. Shcherbinin, J. Mullen, P. Barker, J. Schumi, C. Shering, B. R. Matthews, R. A. Stern, B. Vellas, S. Cohen, E. Macsweeney, M. Boada and J. R. Sims, JAMA Neurol., 2020, 77, 199, DOI:10.1001/jamaneurol.2019.3988.
-
M. J. Frisch, G. W. Trucks, H. B. Schlegel, G. E. Scuseria, M. A. Robb, J. R. Cheeseman, G. Scalmani, V. Barone, G. A. Petersson, H. Nakatsuji, X. Li, M. Caricato, A. V. Marenich, J. Bloino, B. G. Janesko, R. Gomperts, B. Mennucci, H. P. Hratchian, J. V. Ortiz, A. F. Izmaylov, J. L. Sonnenberg, D. Williams-Young, F. Ding, F. Lipparini, F. Egidi, J. Goings, B. Peng, A. Petrone, T. Henderson, D. Ranasinghe, V. G. Zakrzewski, J. Gao, N. Rega, G. Zheng, W. Liang, M. Hada, M. Ehara, K. Toyota, R. Fukuda, J. Hasegawa, M. Ishida, T. Nakajima, Y. Honda, O. Kitao, H. Nakai, T. Vreven, K. Throssell, J. A. Montgomery Jr., J. E. Peralta, F. Ogliaro, M. J. Bearpark, J. J. Heyd, E. N. Brothers, K. N. Kudin, V. N. Staroverov, T. A. Keith, R. Kobayashi, J. Normand, K. Raghavachari, A. P. Rendell, J. C. Burant, S. S. Iyengar, J. Tomasi, M. Cossi, J. M. Millam, M. Klene, C. Adamo, R. Cammi, J. W. Ochterski, R. L. Martin, K. Morokuma, O. Farkas, J. B. Foresman and D. J. Fox, Gaussian 16 Rev. C.01, Wallingford, CT, 2016 Search PubMed.
- A. D. Becke, J. Chem. Phys., 1993, 98, 5648–5652, DOI:10.1063/1.464913.
- C. T. Lee, W. T. Yang and R. G. Parr, Phys. Rev. B: Condens. Matter Mater. Phys., 1988, 37, 785–789, DOI:10.1103/PhysRevB.37.785.
- F. Weinhold and C. R. Landis, Chem. Educ. Res. Pract., 2001, 2, 91–104, 10.1039/b1rp90011k.
- A. E. Reed, R. B. Weinstock and F. Weinhold, J. Chem. Phys., 1985, 83, 735–746, DOI:10.1063/1.449486.
- A. E. Reed and F. Weinhold, J. Chem. Phys., 1983, 78, 4066–4073, DOI:10.1063/1.445134.
Footnote |
† Electronic supplementary information (ESI) available: Experimental procedures and 1H and 13C NMR spectra for new compounds (PDF). See DOI: https://doi.org/10.1039/d4ob01216j |
|
This journal is © The Royal Society of Chemistry 2024 |
Click here to see how this site uses Cookies. View our privacy policy here.