DOI:
10.1039/D4FO00414K
(Review Article)
Food Funct., 2024,
15, 5209-5223
Inhibitory effect of extracts from edible parts of nuts on α-amylase activity: a systematic review†
Received
24th January 2024
, Accepted 18th March 2024
First published on 8th May 2024
Abstract
Elevated blood glucose concentration is a risk factor for developing metabolic dysfunction and insulin resistance, leading to type 2 diabetes and cardiovascular diseases. Nuts have the potential to inhibit α-amylase activity, and so lower postprandial glucose, due to their content of polyphenols and other bioactive compounds. We conducted a systematic literature review to assess the ability of extracts from commonly consumed edible parts of nuts to inhibit α-amylase. Among the 31 included papers, only four utilised human α-amylases. These papers indicated that polyphenol-rich chestnut skin extracts exhibited strong inhibition of both human salivary and pancreatic α-amylases, and that a polyphenol-rich almond skin extract was a potent inhibitor of human salivary α-amylase. The majority of the reviewed studies utilised porcine pancreatic α-amylase, which has ∼86% sequence homology with the corresponding human enzyme but with some key amino acid variations located within the active site. Polyphenol-rich extracts from chestnut, almond, kola nut, pecan and walnut, and peptides isolated from cashew, inhibited porcine pancreatic α-amylase. Some studies used α-amylases sourced from fungi or bacteria, outcomes from which are entirely irrelevant to human health, as they have no sequence homology with the human enzyme. Given the limited research involving human α-amylases, and the differences in inhibition compared to porcine enzymes and especially enzymes from microorganisms, it is recommended that future in vitro experiments place greater emphasis on utilising enzymes sourced from humans to facilitate a reliable prediction of effects in intervention studies.
1. Introduction
Starch is the primary consituent of carbohydrates, which are recommended to make up 40 to 75% of dietary energy intake.1 Ingested starch undergoes an initial enzymatic breakdown into oligosaccharides through the action of α-amylases found in saliva and pancreatic juice. α-Glucosidases, membrane-bound enzymes located on the epithelium of the small intestine, further hydrolyse glucose from the resultant oligosaccharides and disaccharides, leading to the absorption of glucose and an increase in blood glucose concentration.2,3
Diabetes is one of the most prevalent chronic diseases in the world and is characterised by persistently elevated blood glucose levels.4,5 One therapeutic strategy for managing type 2 diabetes mellitus (T2DM) involves moderating postprandial glucose elevations.6 This can be achieved by delaying the uptake of glucose into the blood through the inhibition of enzymes responsible for carbohydrate hydrolysis, namely α-amylase and α-glucosidase. Inhibitors of these enzymes, such as acarbose, delay carbohydrate digestion, extending the overall duration of carbohydrate breakdown.7 This leads to a reduction in the rate of glucose absorption and, subsequently, attenuates the postprandial increase in blood glucose levels.8,9 Nevertheless, prolonged utilisation of these treatments may lead to the onset of side effects, including conditions such as irritable bowel syndrome, severe kidney or liver impairment, and the emergence of drug resistance.9 Hence, there is interest regarding the utilisation of food-based compounds with a comparable, but weaker, effect to acarbose to reduce the observed adverse effects.
Numerous foods and compounds derived from plants have been thoroughly documented for their capacity to inhibit α-amylase3 and so the association between the consumption of regular compounds from plants, including nuts, and a reduced incidence of T2DM10,11 may be attributed to this activity. Nuts are rich in nutrients and known for their complex nutritional composition, characterised by a high concentration of unsaturated fatty acids, proteins, vitamins, minerals, and a variety of bioactive chemicals, such as polyphenols and peptides.12–14 Literature indicates that peptides possess the capability to inhibit α-amylase activity by binding to the enzyme at multiple distinct active sites.15,16 Also, numerous polyphenolic compounds are able to inhibit α-amylases.3,17 The interaction between polyphenols and α-amylase involves hydrogen bonding, primarily hydroxyl groups interacting with the catalytic active site of the enzyme, as well as hydrophobic forces, mainly occurring between the aromatic rings of polyphenols and tryptophan residues within α-amylase.18 Findings have shown a substantial correlation between the inhibitory effectiveness and the number of hydroxyl groups located on the B ring of flavonoids, the most abundant type of polyphenols found in nuts.13,19
We recently conducted a systematic literature review exploring the inhibitory effect of extracts from edible parts of nuts on α-glucosidase activity in vitro but found no studies that tested the inhibitory potential against human α-glucosidases.20 Our objective in the current study was to systematically review the evidence concerning the impact of extracts of the most commonly consumed parts of nuts on α-amylase activity in vitro, which is the other enzyme central to carbohydrate digestion, and therefore regulation of postprandial glycaemia, in vivo.
2. Methods
2.1. Search strategy
This review was registered with the International Platform of Registered Systematic Review and meta-analysis Protocols (INPLASY, registration number: INPLASY202380030) and is reported following the Preferred Reporting Items for Systematic Reviews and meta-Analyses (PRISMA) statement.21 The literature search employed a pre-defined strategy to identify original peer-reviewed studies investigating the inhibitory effects of nut extracts on α-amylase activity. The study selection had no specific time frame restrictions, encompassing all pertinent studies published up to 22nd November 2023. The initial search was conducted on 2nd June 2023 and we repeated the search on 22nd November 2023. Articles were retrieved from four databases: PubMed, Scopus, Web of Science, and Cochrane. The search incorporated the following terms: (“Inhibit*”) AND (“Nuts” OR “*nut” OR “Almond*” OR “Pecan*” OR “Pistachio*” OR “Pistacia*” OR “Macadamia*” OR “Pine*” OR “Hazelnut*” OR “Walnut*” OR “Cashew*” OR “Prunus” OR “dulcis” OR “Bertholletia” OR “Brazil*” OR “Anacardium” OR “Corylus” OR “Carya” OR “Arachis” OR “Juglans”) AND (“*amylase”).
2.2. Eligibility criteria
To be eligible for inclusion, selected articles had to meet specific criteria: (1) be written in English; (2) report original in vitro research studies; (3) be focused on compounds extracted from commonly consumed parts of nuts (i.e. excluding components such as cashew testa, pistachio hull, chestnut shell and walnut diaphragm); (4) report on the inhibitory effects on α-amylase enzymes.
2.3 Screening
All the references from the search were imported into the Covidence Systematic Review Software (https://www.covidence.org; Veritas Health Innovation, Melbourne, Australia), which was used to screen studies. Duplicate records were removed automatically using Covidence. Article titles and abstracts were screened independently by two of the authors (MF and either MJH, BRC or MM) according to the eligibility criteria. Selected studies then underwent full-text screening, which was also conducted independently by two of the authors (MF and either MJH, BRC or MM). Differences in opinions in the two screening steps were discussed among all authors until a consensus was reached.
2.4. Data extraction
A single author (MF) conducted the data extraction, which was verified by a second author (MJH). Data regarding the name and part of the nut(s) and inhibitor compound(s) (e.g., polyphenols, proteins, fats, etc.,), the description of nut extraction methods, the source and type of enzyme (e.g., porcine pancreatic, human salivary, human pancreatic, bacteria/fungus), enzyme assay methods, the half-maximal inhibitory concentration (IC50) and/or inhibition percentage and range of inhibition, number of replicates, and statistical analyses were extracted from each paper. Where not clearly stated, the final concentrations of enzyme and substrate used in the assays were calculated from the descriptions of volumes and working concentrations described in the methods (where available). Further, where crucial information such as the source and type of enzyme utilised was not explicitly stated in the included text, but the method in another paper cited, the information (where available) was extracted from the secondary citation.
2.5. Quality assessment
A quality assessment of the studies was conducted independently by two authors, MF and MJH, using our previously published modified version of the ToxRTool,20 with minor modifications (ESI Table 1†). Briefly, the articles were assessed for quality based on a set of 17 criteria categorised into five assessment domains: test substance identification, test system characterisation, study design description, study results presentation, and the plausibility of the study design and results. Each criterion was given either a “yes” or “no” response (ESI Table 1†). Due to variations in the relative importance of individual criteria, assigning an overarching quality rating to each study was unfeasible.
2.6. BLAST analysis
A BLAST (Basic Local Alignment Search Tool) comparison analysis (conducted viahttps://www.uniprot.org/blast) was executed to assess the sequence homology among distinct α-amylase variants.
3. Results
3.1. Study selection
The primary aim of this systematic review was to evaluate the literature reporting the inhibition of human α-amylase activity through the utilisation of extracts rich in bioactive compounds from commonly consumed edible parts of nuts. It is noteworthy, however, that the search included all sources of α-amylase and was not limited exclusively to human enzymes. The initial search resulted in a total of 1156 papers, out of which 386 duplicate publications were excluded. Amongst the remaining 770 papers, 64 articles went through full-text screening in accordance with the pre-established inclusion criteria. A total of 31 papers met all the predetermined inclusion criteria and were included in the systematic review.16,22–51 In the second search, we identified 141 relevant publications published in 2023. After removing duplicates and carrying out a thorough screening, we identified and included two new studies,52,53 bringing the total included studies to 33. Fig. 1 illustrates the process of study selection. Out of the 33 papers, two studied the effect of areca nut (Areca catechu L.) extract, despite the fact that the use of areca nut in any form is considered unsafe for health.54–56 Therefore, these two studies were excluded, leaving a total of 31 papers.
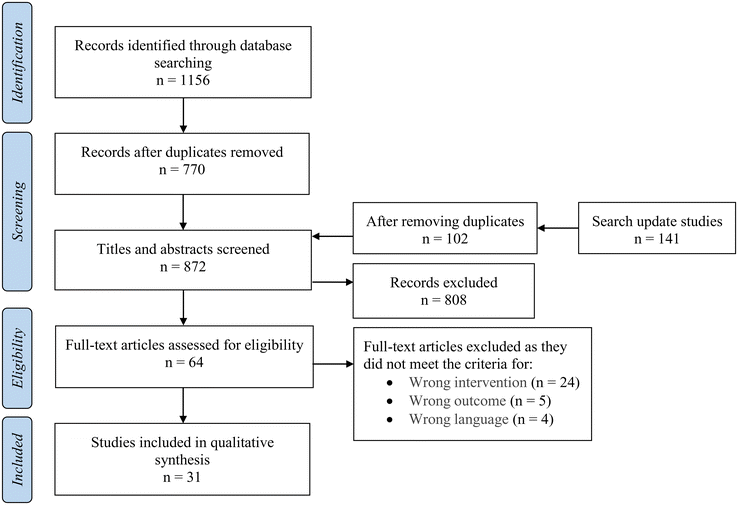 |
| Fig. 1 PRISMA flow diagram for the systematic review, detailing the database searches, the number of abstracts screened, and full texts retrieved. | |
3.2. Study characteristics
Among the 31 included papers, only two studies utilised human pancreatic α-amylase.44,45 These same two studies, along with Tsujita et al.42 and Göksu et al.,28 also used human salivary α-amylases in their enzyme inhibition assays (Table 1). All three studies by Tsujita et al., used porcine pancreatic α-amylases in addition to human α-amylases.42,44,45 Additionally, 17 papers explicitly mentioned or referenced the utilisation of porcine pancreatic α-amylases16,22,23,25–27,34–39,41,43,48,50,52 (Table 2). Four studies indicated or referenced the use of α-amylases derived from a bacterial24,31,47 or fungal source.32 However, the remaining six papers did not include information about the origin of the α-amylases used in their experiments, nor was information on the source included in any cited method29,33,40,46,49,53 (Table 3). Of the included studies, 24 reported the inhibitory effect of polyphenolic-rich extracts from edible parts of nuts on α-amylases.22,23,25–27,31–46,48–50 Three studies focused on proteins extracted from edible parts of nuts,16,28,47 and two studies evaluated the α-amylase inhibitory effect of oil extracts.29,53 Two studies did not extract specific compounds from nuts but used infusions of the whole kernel.24,52 All studies presented the results of enzyme activity as either a percentage of inhibition for a given concentration or reported the IC50, which represents the concentration of the extract required to inhibit enzyme activity by 50%. The assays were mostly conducted at least in triplicate, except for nine studies that did not specify the number of replicates performed.24,26,33,39,40,43,49,52,53
Table 1 Characteristics of included studies that examined inhibition of human α-amylases
Nut |
Extraction |
Enzyme assay |
Enzyme inhibition |
Ref. |
Name |
Part |
Solvent |
Inhibitor |
n
|
Substrate Conc. |
Enzyme Conc. |
Positive control, IC50 or inhibition% |
Source |
IC50 (μg mL−1)i or inhibitionb (%) |
Conc. range tested |
Abbreviations: NS: not specified; PP: polyphenols; Pro: proteins; ODS: octadecylsilyl. Not stated in the text and no method was cited so the enzyme concentration could not be determined even from a secondary citation. Percentage numbers represent the maximum % inhibition achieved, where IC50 was not reached or determined or reported for the respective extract or compound. If no concentration is specified along with % inhibition, it indicates that the concentration resulting in that inhibition was not reported. Mean ± standard error of the mean. Mean ± standard deviation. Estimated from the figure in the paper and not explicitly stated. The acetone extract exhibited the most potent α-amylase inhibition among the different extracts that were tested. Bromelain was used in the hydrolysis of hazelnut protein isolates, though the concentration of the sample used in the assay was not specified. Bromelain hydrolysate was further purified using fast protein liquid chromatography, and fraction 2 had the peptide sequence LEPTNRIEA, the concentration of which was not specified. μg dry weight of extract mL−1. |
Chestnut (Castanea sativa) |
Skin |
75% ethanol (v/v) |
PP |
6 |
5 mg mL−1 starch |
NSa |
NS |
Human pancreas |
7.5 ± 1.2c |
NS |
44
|
Human saliva |
9.4 ± 1.3c |
Porcine pancreas |
18.2 ± 3.2c |
Chestnut (Castanea sativa) |
Skin |
75% ethanol (v/v), centrifugation, dialysis, purification on Diaion HP-20 and Sephadex LH-20 columns |
PP |
3 |
5 mg mL−1 starch |
NSa |
Acarbose 11.2 μM (7.22 μg mL−1) |
Human pancreas |
1.89 |
≤100 μg mL−1e |
45
|
Human saliva |
3.17 |
Porcine pancreas |
5.71 |
Bacillus sp
|
23.9 |
Almond (Prunus dulcis) |
Roasted skin |
Water, methanol, ethanol, acetone, and/or acetonitrile; purification by ultrafiltration, Sephadex LH-20 and ODS columns |
PP |
3 |
5 mg mL−1 starch |
NSa |
NS |
|
Purified polyphenol fractionf |
≤50 μg mL−1e |
42
|
Human saliva |
2.74 |
Porcine pancreas |
2.20 |
Bacillus sp
|
49.5 |
Hazelnut (Corylus avellana L.) |
Protein isolates |
Alkali extraction-isoelectric precipitation, enzymatic proteolysis and fractionation |
Pro |
3 |
0.4% (w/v) starch |
0.8 U mL−1 |
Diprotin A |
Human saliva |
Bromelain hydrolysateg: 1.7 ± 0.1%d |
NS |
28
|
Bromelain hydrolysate fraction 2 (BRF2)h: 4.6 ± 0.1%d |
Table 2 Characteristics of included studies that examined inhibition of porcine α-amylases
Nut |
Extraction |
Enzyme assay |
Enzyme inhibition |
Ref. |
Name |
Part |
Solvent |
Inhibitor |
n
|
Substrate Conc. |
Enzyme Conc. |
Positive control, IC50 or inhibition% |
Source |
IC50 (μg mL−1)k or inhibitionc (%) |
Conc. range tested |
Abbreviations: NS: not specified; PP: polyphenols; Pro: proteins; ND: not detected. A search of the cited method revealed the source and/or concentration of α-amylase and/or substrate concentration, however the study did not specify explicitly. Not specified, even in secondary citation. Percentage numbers represent the maximum % inhibition achieved, where IC50 was not reached or determined or reported for the respective extract or compound. If no concentration is specified along with % inhibition, it indicates that the concentration resulting in that inhibition was not reported. Mean ± standard deviation. Mean ± standard error of the mean. Estimated from the figure in the paper and not explicitly stated. The study examined the impact of three rootstocks and three regulated deficit irrigation (RDI) treatments (T0 or control, T1 or moderate treatment, and T2 or strong treatment) on the functional quality of pistachios. No information is provided regarding the lowest concentration tested. Cashew nut globulin. Cashew nut albumin. μg dry weight of extract mL−1. |
Chestnut (Castanea sativa) |
Skin |
75% ethanol (v/v) |
PP |
NS |
5 mg mL−1 starcha |
NS |
NS |
Porcine pancreas |
7.5 |
≤100 μg mL−1f |
43
|
Chestnut (Castanea mollissima Blume) |
Inner skin |
70% ethanol (v/v) |
PP |
≥3 |
0.25% (w/v) starch |
0.94 U mL−1 |
Acarbose 29.4 μM |
Porcine pancreas |
27.2 ± 0.2d |
25 and 30 μg mL−1 |
50
|
(19 μg mL−1) |
Almond (Terminalia catappa L.) |
Drupe |
Methanol : 1 N HCl (1 : 1 w/v) |
PP |
3 |
0.5% (w/v) starch |
0.25 mg mL−1 |
NS |
Porcine pancreas |
10.1 ± 7.1d |
40–160 μg mL−1f |
22
|
Almond (Prunus dulcis cv. Casteltermini) |
Skin |
n-Hexane with ethanol |
PP |
3 |
5 mg mL−1 starch potatoa |
0.25 mg mL−1a |
Acarbose |
Porcine pancreasa |
73.8 ± 3.9d |
NS |
34
|
55.0 ± 1.4 μMd |
(35.5 ± 0.9 μg mL−1d) |
Kola nut (Cola acuminate) |
Kernel |
70% ethanol (v/v) |
PP |
3 |
0.02% (w/v) starcha |
0.04 mg mL−1a |
Acarbose 50.5 ± 1.2 μMe |
Porcine pancreas |
C. acuminata: 56.7 ± 0.7e |
NS |
25
|
Bitter kola (Garcinia kola) |
(32.6 ± 0.8 μg mL−1e) |
G. kola: 35.1 ± 0.6e |
Kola nut (Cola nitida) |
Kernel |
80% acetone (v/v) |
PP |
3 |
0.83–0.95% (w/v) starch |
0.42–0.48 mg mL−1 |
NS |
Porcine pancreas |
340 ± 10d |
100–400 μg mL−1 |
38
|
Atlantic terebinth (Pistacia terebinthus) |
Kernel |
n-Hexane |
PP |
3 |
0.33% (w/v) starch |
NS |
Acarbose |
Porcine pancreas |
Hexane extract: ≤ 33.5% at 160 000 μg mL−1 |
Hexane extract: 125–2000 μg mL−1 |
23
|
Acetone |
Acetone extract: ≤ 47.9% at 160 000 μg mL−1 |
Acetone and ethanol extracts: 100–1000 μg mL−1 |
Ethanol |
Ethanol extract: 100% at 1000 μg mL−1 |
|
Pistachio (Pistacia vera L.) cultivars: |
Kernel |
80% methanol (v/v) with 1% of HCl (v/v) |
PP |
3 |
0.33% (w/v) starcha |
0.167 mg mL−1a |
NS |
Porcine pancreas |
Aegina: 6710 |
NS |
37
|
Aegina
|
Avdat: 7410 |
Avdat
|
Napolitana: 1021 |
Sirora
|
Sirora: 9900 |
Mateur
|
Kastel: 8410 |
Napolitana
|
Larnaka: 6560 |
Mateur
|
Kerman: 5390 |
Kastel
|
Mateur: 5560 |
Mateur
|
|
Larnaka
|
|
Kerman
|
|
Pistachio (Pistacia vera) |
Kernel |
80% methanol (v/v) with 1% HCl (v/v) |
PP |
3 |
0.33% (w/v) starcha |
0.167 mg mL−1a |
NS |
Porcine pancreas |
Rootstocksg: |
NS |
36
|
P. integerrima: 24 000 |
P. atlantica: 23 900 |
P. terebinthus: 23 420 |
Regulated deficit irrigationg: |
T0: 19 730 |
T1: 26 420 |
T2: 25 170 |
Acorn (Quercus wutaishanica Blume) |
Kernel |
n-Hexane, 70% ethanol (v/v), AB-8 macroporous resin column |
PP |
≥3 |
Acorn, corn, potato, chestnut starches (Conc. NS) |
NS |
NS |
Porcine pancreas |
Free-polyphenol extract: 5250 |
NS |
35
|
Bound-polyphenol extract: 1370 |
Chilean hazelnut (Gevuina avellana) |
Kernel |
Methanol, chloroform, Amberlite XAD-7 resin |
PP |
NS |
0.33% (w/v) starcha |
2.67 U mL−1a |
Acarbose 44.2 μM (28.5 μg mL−1) |
Porcine pancreas |
Intensively roasted (coffee): 12.5% at 100 μg mL−1 |
50 and 100 μg mL−1h |
39
|
All other extracts < 10% |
Pecan (Carya illinoinensis) |
Kernel |
Acetone, water, acetic acid (70: 29.5 : 0.5, v/v/v) |
PP |
3 |
0.33% (w/v) potato starch |
12 U mL−1 |
NS |
Porcine pancreas |
77.9 |
10–1000 μg mL−1 |
27
|
Walnut (Juglans regia L) |
Kernel |
Methanolic maceration |
PP |
3 |
NS |
9.38 U mL−1 |
Acarbose 40.4 μM |
Porcine pancreasa |
37.4 ± 1.2d |
25–100 μg mL−1 |
26
|
(26.1 μg mL−1) |
Heckel (Ricinodendron heudelotii Baill.) |
Kernel |
Ethyl acetate |
PP |
3 |
0.05% (w/v) starcha |
NSb |
Acarbose |
Porcine pancreasa |
Ethyl acetate extract: 420 ± 40 μmol acarbose equivalent per gd |
NS |
41
|
Methanol |
Methanol extract: 550 ± 20 μmol acarbose equivalent per gd |
Water |
Water extract: 160 ± 0.00 μmol acarbose equivalent per gd |
Cashew (Anarcadium occidentale) |
Kernel |
1 M NaCl (1 : 10, w/v) |
Pro |
3 |
0.48% (w/v) starcha |
0.48 mg mL−1a |
|
Porcine pancreasa |
CNG i hydrolysates: |
500–2000 μg mL−1 |
16
|
|
Tryptic: 170 ± 10d |
|
Alcalase: 290 ± 10d |
Acarbose 387 ± 30 μMd |
Pancreatic: 390 ± 10d |
(250 ± 20 μg mL−1d) |
CNA j hydrolysates: tryptic: 61.1 ± 1.1% at 2000 μg mL−1 |
|
Alcalase: 290 ± 14d |
|
Pancreatic: 410 ± 21d |
Pine (Pinus halepensis) |
Kernel |
Deionised water |
Infusion from powdered kernel |
NS |
0.5% (w/v) starch |
0.00075% (w/v) |
NS |
Porcine pancreasa |
ND |
NS (supernatant from 100 g L−1) |
52
|
Pecan (Carya illinoinensis), Pine (Pinus pinea), Hazelnut (Corylus avellana), Pistachio (Pistacia vera), Almond (Prunus amygdalus), Cashew (Anacardium occidentale L.), Walnut (Juglans regia), Macadamia (Macadamia integrifolia) |
Kernel |
Methanol/water/HCl (80 : 19 : 1 v/v/v) |
PP |
3 |
NSb |
NSb |
|
Porcine pancreas |
Pecan: 148 900 ± 2600e |
NS |
48
|
|
Pine: 262 600 ± 3100e |
|
Hazelnut: 276 500 ± 2600e |
Acarbose |
Pistachio: 391 000 ± 1300e |
160 μM |
Almond: 262 100 ± 3400e |
(100 μg mL−1) |
Cashew: 318 900 ± 3300e |
|
Walnut: 281 000 ± 2400e |
|
Macadamia: 284 100 ± 2800e |
Table 3 Characteristics of included studies that examined inhibition of non-mammalian or non-defined α-amylases
Nut |
Extraction |
Enzyme assay |
Enzyme inhibition |
Ref. |
Name |
Part |
Solvent |
Inhibitor |
n
|
Substrate Conc. |
Enzyme Conc. |
Positive control, IC50 or inhibition% |
Source |
IC50 (μg mL−1)q or inhibitiond (%) |
Conc. range tested |
Abbreviations: NS: not specified; PP: polyphenols; Pro: proteins. A search of the cited method revealed the source and/or concentration of α-amylase and/or substrate concentration, however the study did not specify explicitly. Not specified, even in secondary citation. No further details on part of nut used, with no mention of skin left on seed or not, or identification of compounds present. Percentage numbers represent the maximum % inhibition achieved, where IC50 was not reached or determined or reported for the respective extract or compound. If no concentration is specified along with % inhibition, it indicates that the concentration resulting in that inhibition was not reported. SD or SEM not stated. Mean ± standard deviation. Mean ± standard error of the mean. Estimated from the figure in the paper and not explicitly stated. Nut collected from the Touila-Had Shary part of the Djelfa region, Algeria and not stated if SD or SEM. Nut collected from the Hassi El Ouch part of the Djelfa region, Algeria and not stated if SD or SEM. Nut collected from the Bouira–Al Hadab part of the Djelfa region, Algeria and not stated if SD or SEM. Nut collected from the Touila-Had Shary part of the Djelfa region, Algeria and not stated if SD or SEM. The IC50 values for other solvent extracts were not reached and therefore not specified. Reversed phase high performance liquid chromatography. A standard drug, containing 2 mg glimepiride and 500 mg metformin, used to treat type 2 diabetes. The α-amylase assay in this study was a part of a human in vitro digestive system, but the enzyme source was not explicitly stated. μg dry weight of extract mL−1. |
Atlantic terebinth (Pistacia atlantica) |
Dried unripe fruitc |
Ultrasonication in water |
PP |
NS |
Starch (Conc. NS) |
NS |
Acarbose 5660 ± 16.6 μMe |
Aspergillus oryzae
|
TRC4i: 32.6 ± 0.7e |
NS |
32
|
(3651 ± 10.7 μg mL−1e) |
HRC1j: 111 ± 3.0e |
|
SRC2k: 69.2 ± 3.1e |
|
BRC3l: 67.1 ± 1.7e |
Camus nut (acorn) (Q. floribunda) |
Kernel |
n-Hexane |
PP |
3 |
0.89 mg mL−1 starch |
0.04 U mL−1 |
Acarbose 52.3 ± 0.2 μMf |
Bacteriaa |
Ethyl acetate extract: 158.6m |
≤444 μg mL−1 |
31
|
Chloroform |
(33.7 ± 0.1 μg mL−1f) |
Acetone |
|
Ethylacetate |
|
Ethanol |
|
Methanol distilled water |
|
Sweet almond (Prunus amygdalus Stokes) |
Kernel |
Boiling distilled water |
Whole kernel infusion |
NS |
0.15% (w/v) starch |
0.25 U mL−1 |
NS |
Bacteriaa (Maxilase) |
2630 |
NS |
24
|
Bitter almond (Prunus amygdalus var. amara) |
Kernel |
Cold press, sedimentation and filtration |
Oil |
3 |
0.05% (w/v) starcha |
NSb |
Acarbose |
NSb |
1150 ± 594f |
NS |
29
|
5650 ± 16 600 μMf |
(3650 ± 10 700 μg mL−1f) |
Almond oil (Prunus dulcis) |
Kernel |
NA |
Oil |
NS |
2.5 mg mL−1 (0.1 M HCl) |
12.5 μg mL−1 |
Acarbose 93.4 ± 2.8 μMe (60.3 ± 1.8 μg mL−1e) |
NSb |
120.6 ± 2.6e |
NS |
53
|
Walnut (Juglans mandshurica Maxim.) |
Purified peptidesc |
Alkaline protease hydrolysis, ultrafiltration and purification on a Sephadex G-25 column and by RP-HPLCn |
Pro |
5 |
0.94% (w/v) starch |
0.02 U mL−1 |
Acarbose |
Bacteriaa |
39.1% for 2000 μM LPLLR peptide |
100–2000 μM |
47
|
Fever nut (Caesalpinia bonducella L.) |
Kernel |
Water |
PP |
NS |
0.25% (w/v) starch |
NS |
Glycomet GP2o |
NSb |
47.4% at 1000 μg mL−1 |
500 and 1000 μg mL−1 |
40
|
59.2% at 500 μg mL−1 |
81.0% at 1000 μg mL−1 |
Pecan (Carya illinoinensis) |
Kernel |
Hexane (1 : 10, w/v), 80% acetone (v/v), 95% ethanol (v/v), sonication, Sephadex LH-20 |
PP |
3 |
4-Nitrophenyl α-D-maltoside (Conc. NS) |
0.75 ng mL−1 |
NS |
NSp |
45.9 ± 0.3% for 2.5 μg mL−1f proanthocyanidin-rich extract |
NS |
46
|
Kola nut (Cola nitida) |
Kernel |
Boiling distilled water |
PP |
NS |
0.25% (w/v) starch |
NS |
Acarbose |
NSb |
120.22 |
15–240 μg mL−1 |
33
|
2.34 μM |
(1.51 μg mL−1) |
Pine nut (Pinus gerardiana) |
Kernel |
Methanol |
PP |
NS |
1% (w/v) starcha |
NSb |
Acarbose 1550–2480 μM |
NSb |
Methanolic extract 88.7 ± 5.1% at 16 000 μg mL−1g |
1000–16 000 μg mL−1 |
49
|
Ethyl acetate |
(1000–16 000 μg mL−1) |
Ethyl acetate extract 82.3 ± 5.5% at 16 000 μg mL−1h |
3.3. Inhibition of human α-amylases by nut extracts
Table 1 presents the characteristics of the studies included in this systematic review that used human α-amylase. The inhibition by chestnuts was examined in detail in two studies.44,45 When a dialysis-based purification process with Diaion HP-20 and Sephadex LH-20 columns was used to isolate polyphenolic compounds from chestnut skin, there was a significant improvement (∼3.4-fold) in α-amylase inhibition.45 The size exclusion fraction contained approximately 65% total polyphenols, primarily composed of flavanol-type tannins and procyanidins, and IC50 values were 5.71, 3.17, and 1.89 μg mL−1 for porcine pancreatic, human salivary and human pancreatic α-amylase, respectively (acarbose IC50 for porcine pancreatic: 7.22 μg mL−1). Interestingly, these results indicate inhibition in the order: human pancreatic > human salivary > porcine pancreatic α-amylase inhibition. Comparable results were reported in another study by the same group,44 where polyphenolic extracts from chestnut skin exerted stronger inhibitory effects on human pancreatic and salivary α-amylase activity (IC50: 7.5 ± 1.2 μg mL−1 and 9.4 ± 1.3 μg mL−1, respectively) when compared to porcine pancreatic α-amylase activity (IC50: 18.2 ± 3.2 μg mL−1). However, a direct comparison with acarbose as a positive control was lacking.44
A comprehensive investigation was conducted by the same group again to analyse the inhibitory effect of almond skin polyphenolic extracts on human salivary, porcine pancreatic, and Bacillus α-amylases.42 The data revealed subtle differences in the inhibitory effects on human salivary α-amylase (IC50: 2.74 μg mL−1) and porcine pancreatic α-amylase (IC50: 2.20 μg mL−1). Notably, this extract exhibited considerably weaker inhibition, ∼25-fold less effective, when assayed against Bacillus α-amylase activity (IC50: 49.5 μg mL−1). These findings underscore the effectiveness of almond skin extract as an inhibitor of human salivary and porcine pancreatic α-amylase. Moreover, the isolation and purification of polyphenolic compounds from almond skin was meticulously conducted through a sequential process involving ultrafiltration, Sephadex LH-20 chromatography, and ODS (octadecylsilyl) column chromatography. The total polyphenol content of the purified fraction was ∼62% of the dry weight, as estimated by the Folin–Ciocalteu assay with catechin as the standard, and the fraction was characterised by the presence of ∼34% flavanol-type tannins and ∼30% procyanidins of the dry weight. On the other hand, protein extracted from hazelnuts has minimal inhibitory effects on human salivary α-amylase.28 In this study, proteins were isolated by alkali extraction-isoelectric precipitation and then enzymatically hydrolysed by papain, bromelain or pepsin. The hydrolysates exhibited no significant α-amylase inhibitory activity, with the authors suggesting that the lack of lysine, phenylalanine, tyrosine and tryptophan residues in the isolated peptides was responsible for the lack of binding to α-amylase.
3.4. Inhibition of porcine pancreatic and non-mammalian α-amylases by nut extracts
The effects of chestnut and almond skin extracts on human α-amylases are complemented by effects on porcine pancreatic α-amylase. Chestnuts have been found to possess a significant amount of dietary fibre and a variety of essential vitamins and minerals,57–60 and an abundance of polyphenols.13,45 Chestnut inner skin extracts inhibited porcine pancreatic α-amylase with an IC50 value of 27.2 ± 0.2 μg mL−1, which was comparable to, but less potent than, acarbose (IC50: 19 μg mL−1).50 Another study reported a lower IC50 value of 7.5 μg mL−1 for chestnut skin extract, but the absence of a reference inhibitor in this assay leaves the effectiveness of this extract uncertain.43 Almonds are nutrient-dense foods that contain vitamins, minerals, fibre, and bioactive compounds.61,62 Consuming almonds has been associated with several health benefits, including a reduction in postprandial glycemia.63,64 Studies included in the current systematic review show that polyphenolic extracts from almond drupe (IC50: 10.1 ± 7.1 μg mL−1) or skin (IC50: 73.8 ± 3.9 μg mL−1), but less so kernel (IC50: 262
100 ± 3400 μg mL−1), effectively inhibit porcine pancreatic α-amylase activity.22,34,48
Kola nuts are the seed pods from evergreen trees indigenous to Africa. Kola nut extracts are increasingly recognised as a natural or complementary remedy for diabetes management.65 The active constituents within kola nuts primarily consist of phenolic compounds and purine alkaloids.25 Polyphenolic extracts from two varieties of kola nut, Cola acuminate and Garcinia kola, demonstrated inhibition of porcine pancreatic α-amylase with IC50 values of 56.7 ± 0.7 μg mL−1 and 35.1 ± 0.6 μg mL−1, respectively (acarbose IC50: 32.6 ± 0.8 μg mL−1).25 In a separate investigation, the observed IC50 value for kola nut was nearly ten-fold higher, though it is important to note that a positive control was not utilised so the reliability of the enzyme assay system cannot be ascertained.38
In this systematic review, among the 17 studies that utilised porcine pancreatic α-amylase as the only enzyme,16,22,23,25–27,34–39,41,43,48,50,52 four of them specifically investigated the inhibitory potential of polyphenolic extracts from the edible parts of nuts belonging to the Anacardiaceae family, including pistachio (Pistacia vera),36,37,48 and Atlantic terebinth (Pistacia terebinthus).23 The Pistacia genus predominantly inhabits the northern hemisphere, encompasses both evergreen and deciduous varieties, and has long been recognised for medicinal properties.66 The ethanol extract of Pistacia terebinthus, at a concentration of 1 mg mL−1, exhibited complete inhibition of porcine pancreatic α-amylase,23 while the Sirora cultivar of pistachio exhibited a 50% inhibition of porcine pancreatic α-amylase activity at the same concentration.37 In contrast, the mean IC50 value for different pistachio crops in the study by Noguera-Artiaga et al. was 23.8 ± 2.3 mg mL−1.36 Unfortunately, none of these studies utilised or presented the inhibition data for a positive control. In the study by Wojdyło et al., pistachio (Pistacia vera) exhibited much lower inhibition against porcine pancreatic α-amylase, with an IC50 of 391 ± 1.3 mg mL−1, whereas acarbose showed 74% inhibition at a concentration of 0.1 mg mL−1.48 This suggests that pistachio extracts possess relatively lower inhibitory activity against porcine pancreatic α-amylase compared to acarbose.
Acorn belongs to the oak genus within the Fagaceae family.67 Raw acorns are inedible but they are used in the food industry to produce many different products such as noodles, bread, muffins, and drinks.68–70 The primary constituents of acorns encompass starches, lipids, proteins, and bioactive compounds, including polyphenols.35,71 Polyphenolic extracts from acorn kernels of the Quercus variabilis Blume species, also known as Chinese cork oak, demonstrated inhibitory effects on porcine pancreatic α-amylase, with IC50 values of 5.25 mg mL−1 for the free-polyphenol extract and 1.37 mg mL−1 for the bound-polyphenol extract.35 However, detail on the α-amylase assay was lacking since this was conducted to support an investigation into the hydrolysis rates of various starches in vitro, rather than being the main focus of the study.
The Chilean hazelnut, Gevuina avellana Mol., stands as the southernmost representative within the Proteaceae family. This botanical family also encompasses the macadamia nut species.72 The nomenclature “Chilean hazelnut” was assigned to G. avellana owing to its morphological resemblance to the European hazelnut, Corylus avellana L., which belongs to the Betulaceae family.39 Compared to acarbose (IC50: 28.5 μg mL−1), Chilean hazelnut extracts are not potent inhibitors of porcine pancreatic α-amylase (<12.5% inhibition at 100 μg mL−1).39 This observation may be attributed to the relatively low polyphenol content present in these extracts.39 A similar result was demonstrated for hazelnuts (Corylus avellana) (IC50: 276
500 ± 2600 μg mL−1),48 indicating that neither Chilean hazelnut nor hazelnut extracts are effective inhibitors of porcine pancreatic α-amylase activity compared to acarbose.
Both walnuts (Juglans regia L.) and pecans (Carya illinoinensis) have attracted global recognition as nutritionally-rich foods and feature prominently within the Mediterranean diet.62,73 Notably, two polyphenolic-rich walnut extracts (Juglans regia L.), prepared in a very similar way using a methanol-based extraction, exhibited markedly variable inhibition of porcine pancreatic α-amylase activity, with IC50 values ranging from 37.4 ± 1.2 μg mL−1 in the study by Bourais et al., to 281 ± 2.4 mg mL−1 in the study by Wojdyło et al., denoting a ∼7500-fold difference between the two.26,48 The latter study did not state the amount of enzyme used in the assay and lacked a lot of detail on the assay in general, highlighting the difficulty in drawing conclusions from these studies. While not described in the paper, the inhibition data presented by Wojdyło et al., seems to have been calculated to give equivalent values for the raw kernels, rather than for the polyphenolic extract, which may explain this discrepancy. A similar situation is apparent for polyphenol extracts of pecan, with reported IC50 values showing significant variation across different studies (IC50 values 78–148
900 μg mL−1), thus the effect of pecan on porcine pancreatic α-amylase activity remains inconclusive.27,48
Nuts such as cashews, macadamias and pine nuts are highly favoured as snacks, owing to their distinctive flavours, nutritional composition, and health-related benefits.13,62 However, cashew nuts contain <100 mg of polyphenols per 100 g, and macadamia and pine nuts have polyphenol contents below detectable levels.48 Polyphenol extracts of pine nuts (Pinus pinea),48,52 macadamia (Macadamia integrifolia)48 and cashew48 showed no significant inhibition of porcine pancreatic α-amylase. Conversely, peptides isolated from cashew (Anacardium occidentale L.) inhibited porcine pancreatic α-amylase with a mean IC50 of 310 ± 96 μg mL−1.16
Polyphenol extracts from Atlantic terebinth (Pistacia atlantica) demonstrated greater inhibition of Aspergillus oryzae α-amylase activity compared to acarbose.32 Furthermore, inhibition of α-amylase from a bacterial source was observed with various extracts from Camus nuts (Q. floribunda, another species of acorn),31 walnut (Juglans mandshurica Maxim.),47 and sweet almond (Prunus amygdalus Stokes).24 However, the IC50 values for the inhibition of α-amylases from Bacillus sp. by the same inhibitors (chestnut and almond skin extracts) was a mean of 13-fold higher than those reported for the mammalian α-amylases.42,45
3.5. Quality assessment
The results of the quality assessment conducted on the included studies are presented in ESI Table 1.† During this assessment, it came to light that several crucial elements were absent in the reviewed studies. Among the 31 included papers, two of them failed to provide explicit details concerning the part of the nut from which the test compounds were extracted.37,47 Additionally, nine investigations did not provide sufficient details regarding the purity or compositional analyses of the test inhibitors employed.24,27,32,35,40,43,44,52,53 Notably, two studies did not mention the origin/supplier of the nuts used,24,53 and 16 studies did not specify the supplier(s) for all chemicals used.22,25,33,37,38,40–45,47–49,52,53 Eight of them lacked important information on the specific characteristics of the extraction procedure, such as duration, temperature, and pH levels;25,29,33,37,40,49,52,53 for example, two studies failed to specify the temperature conditions for the rotatory evaporator25 or water bath.40 Furthermore, two studies subjected nuts to prolonged immersion in a solvent for over a week; however, there is no explicit mention of whether the nuts were stored in a dark environment during this experimental process.29,49 It is noteworthy that the polyphenol content can be influenced by both temperature and light.74
Over half of the studies failed to specify the type or source of the enzyme used.16,24,26,28,29,31,33,34,37,40,41,46–49,52,53 For Göksu et al., the use of human salivary enzyme was inferred based on the catalogue number, however this information was not explicitly stated.28 Most studies lacked significant information on the enzyme assay, with crucial factors such as pH, buffer composition, incubation period, and temperature absent.16,25,29,33–37,39–45,48,49 It is important to acknowledge that these factors have a significant influence on the kinetics of the enzyme reaction and therefore both enzyme activity and inhibition.75 Furthermore, 22 studies failed to include data regarding the concentration of enzymes utilised.16,22,23,25,29,32–37,39–45,48,49,52,53 In nearly half of the studies, the substrate concentration was not specified,16,22,25,26,28,29,32–37,39,41,43,48,49,53 and the inhibitor concentration range was not provided in 15 of the studies.16,24,25,28,29,32,34–37,41,44,48,52,53 To determine the final concentration of the substrate and/or enzyme (Tables 1–3), we had to refer to secondary papers cited in the studies. Moreover, 12 studies did not specify the time at which the endpoint measurement was recorded at the end of the enzyme assay.16,25,29,33–37,39,41,43,48 There were 24 studies that did not mention using negative controls (such as substrate- and enzyme-free controls),16,22,24–27,29,33–48 and 12 lacked a positive control (such as acarbose).22,24,27,35–38,42–44,46,52 While most studies stated the method used to measure the enzyme activity or inhibition endpoint (e.g. via iodine or dinitrosalicylic acid), a significant limitation observed in several studies was the absence of key information relating to this measurement, such as incubation time and temperature.16,25,29,33–37,39,41,43,46,48
There was a lack of comprehensive reporting of all examined endpoints in some studies.28,35,37,39–41,44,48,52,53 All papers, except for three,40,52,53 provided details on the statistical methodologies employed for data analysis. The quantitative findings in 15 investigations were deemed questionable due to the variation in the data not being presented and/or as a result of atypical calculations and data presentation techniques.23,24,29,35–37,39–43,45,48,52,53 Notably, two studies did not specify whether they reported variation in α-amylase inhibition by standard deviation (SD) or standard error of the mean (SEM),32,53 and the study by Guici El Kouacheur et al., reported standard deviations that were 3-fold larger than the mean when reporting IC50 values.29 The study by Sut et al., investigating the inhibition of porcine pancreatic α-amylase by heckel, presented their inhibition data as acarbose equivalents,41 which were challenging to interpret. While certain studies may have had an appropriate study design, all were missing at least some information to adequately determine this. For instance, in two studies,35,46 the α-amylase assay was part of a human in vitro digestive system and not conducted independently. In the study by Wojdyło et al., a different extraction method was used for polyphenol analysis compared to that for the test inhibitor used in the α-amylase assay.48 It is noteworthy that Zhang et al.50 presented a very well-described enzyme assay but only two inhibitor concentrations were tested, which is insufficient to determine a reliable IC50 value.
4. Discussion
Our review primarily focused on evaluating the existing literature regarding the inhibition of human α-amylases by extracts from commonly consumed nuts. It is noteworthy that only four studies specifically utilised this enzyme source. These studies revealed that chestnut skin extract inhibits both human salivary and pancreatic α-amylases and that almond skin extract can inhibit the salivary enzyme, while peptides isolated from hazelnut were not effective. Phenolic compounds are mostly concentrated in the skins of nuts76 and this may explain why, from the studies conducted on human enzymes to date, nut skins in particular appear to be promising α-amylase inhibitors. The remaining studies mainly employed porcine pancreatic α-amylases, with four studies utilising α-amylases sourced from bacteria or fungi, and six studies failing to specify the source of the enzyme. The findings suggest that polyphenol-rich extracts from nuts, particularly chestnut, almond, kola nut, pecan and walnut, as well as peptides isolated from cashew, inhibit porcine pancreatic α-amylase, while polyphenols in pistachio, Atlantic terebinth, acorn, hazelnut, Chilean hazelnut, pine, cashew and macadamia exhibit milder inhibition. Polyphenols inhibit α-amylase through hydrogen bonding between their hydroxyl groups and the enzyme active site and through hydrophobic forces between the aromatic rings of polyphenols and tryptophan residues within α-amylase.18 Consequently, the inhibitory impact of polyphenols is augmented by an increased number of hydroxyl groups on the B ring of flavonoids, the primary class of polyphenols found in nuts.13,19
4.1. Differences between human salivary and human pancreatic α-amylases
α-Amylases are endo-acting enzymes found in both salivary and pancreatic secretions, and they play a pivotal role in catalysing the initial hydrolysis of starch into shorter oligosaccharides.77,78 Our findings suggest that extracts obtained from chestnut skin exhibit a greater inhibitory effect on the activity of human pancreatic α-amylase when compared to human salivary α-amylase.44,45 Both human α-amylase isozymes consist of 511 amino acids within a single polypeptide chain. Human salivary and pancreatic α-amylases share a significant degree of amino acid sequence homology, with 97% identical residues in their overall sequences and 92% identified within their respective catalytic domains.79,80 However, despite this, there are 15 differences in amino acid composition between the sequences of these two enzymes,79 and this could explain the enhanced inhibition of human pancreatic α-amylase by chestnut skin extracts compared to the salivary enzyme.44,45 It is important to note, however, that our conclusion should be considered in the context of the limited number of studies available on chestnut skin extract and that other research suggests, for example, that (–)-epigallocatechin-3-gallate (EGCG) may be a more effective inhibitor of human salivary α-amylase compared to the pancreatic enzyme.77,81,82 Therefore, it cannot be definitively concluded that nut extracts consistently exhibit superior inhibition of human pancreatic α-amylase, since inhibition is dependent on the interaction between the specific polyphenols present in each extract and the amino acids in each enzyme.
4.2. Differences between human and porcine α-amylases
The findings from the current review highlight the potential of nut extracts to inhibit porcine pancreatic α-amylase activity. Among the 31 studies included in this systematic review, only two investigations utilised both human α-amylases and porcine pancreatic α-amylase, allowing for a direct comparison of the inhibitory effects of nut extracts on these two enzyme sources.44,45 Results of these two studies show that chestnut skin extract was a stronger inhibitor of human α-amylases compared to porcine pancreatic α-amylase.44,45 A separate study investigated the α-amylase inhibitory effect of various Indian medicinal plant extracts, renowned for their hypoglycaemic properties.83 In this study, among ten extracts showing α-amylase inhibition, half of them showed superior inhibition of human pancreatic α-amylase, while three of them exhibited greater inhibition of porcine pancreatic α-amylase, and the remaining extracts were similar on both enzymes.83 Compared to acarbose, four of these plant extracts showed significantly enhanced inhibition of human pancreatic α-amylase, whereas none of the extracts exhibited significant inhibition of porcine pancreatic α-amylase when compared with acarbose.83
Compared to the human pancreatic α-amylase, the polypeptide chain of porcine pancreatic α-amylase contains four distinct regions (residues 237–250, 304–310, 346–354, and 458–461) comprising 70 amino acids, which exhibit different conformations.79,84 Among these regions, the first (residues 237–250) entails multiple mutations within a segment of the polypeptide chain situated adjacent to a highly conserved region of the primary sequence, which includes the catalytic residue Glu 233.79 The second region (residues 304–310) is located within the active site and is known to interact directly with the bound substrate.79 These distinctions in amino acid sequences are responsible for the functional differences between porcine and human pancreatic α-amylases. For example, the Km values of the porcine enzyme for long oligosaccharides are similar to those of the human enzyme. However, the kcat values (maximum rate of substrate conversion into product per enzyme molecule) appear to be significantly higher for long oligosaccharides in the case of porcine α-amylase, although a comprehensive assessment of the specific cleavage mechanisms was lacking.85 Due to differences in enzyme structure and variations in research findings when comparing human and porcine enzymes, it is not appropriate to extrapolate the effect on the porcine enzyme to the effect of an inhibitor on human amylase.
4.3. Differences between human, bacterial, and fungal α-amylases
Even though BLAST analysis (https://www.uniprot.org) confirmed the absence of sequence homology between bacterial or fungal α-amylases, such as Bacillus subtilis (659 amino acids) and Aspergillus oryzae α-amylase (498 amino acids), in comparison to human α-amylase (511 amino acids), some studies have utilised these sources of α-amylase. It is significant to highlight that while these studies have shown the potential of nut extracts to inhibit α-amylases sourced from fungi and bacteria, their outcomes are entirely irrelevant to human health.
4.4. Limitations
The primary limitation is the restricted availability of studies that specifically assess the inhibitory effects of extracts from edible parts of nuts on human α-amylases. Our investigation identified only four relevant studies, with most of the research in this field relying on porcine α-amylase. Another significant consideration is the methodological approach employed in the studies included in this review. The evaluation of α-amylase activity was predominantly conducted indirectly, utilising colorimetric techniques such as the measurement of reducing sugars through dinitrosalicylic acid (DNSA) or chromophore-linked substrates (e.g., starch-iodine). It is crucial to note that in many of these colorimetric methods, redox and UV-visible light-absorbing compounds, such as phenolics, have the potential to directly interfere with the analytical process.75,77,86 Moreover, it has been reported that peptides in the extracts can lead to an overestimation in measurements when using DNSA.87 Since DNSA is used to estimate the reducing ability of sugar products and estimate carbohydrate hydrolysis, the presence of endogenous sugars in plant extracts can influence the accuracy of the reducing sugars method for enzyme activity.86 Additionally, the exclusive use of starch as the substrate across all studies included in this review is another limitation to be acknowledged. Certain bioactive compounds, particularly polyphenols, have been found to directly interact with starch, especially potato-derived starch, through hydrophobic interactions and hydrogen bonding and then affect the kinetics.88–90 The reaggregation of amylose molecules through hydrogen bond-mediated complexation with phenolic compounds limits substrate accessibility to α-amylase, consequently reducing starch digestion.77 This is due to the fact that polyphenols have a greater affinity for forming complexes with amylose as opposed to amylopectin.77,88 Considering these factors, it appears that the mechanism underlying the inhibition of starch digestion by polyphenols, including those found in nuts, extends beyond solely interaction with the active site or binding site of α-amylase. Direct interaction between polyphenols and starch also contributes significantly to this inhibitory effect.77 Additionally, the absence of a positive control such as acarbose in many of the studies complicates any comparison between studies. While polyphenol-rich extracts from chestnut and almond skins demonstrated significant inhibition of human α-amylases, translating these findings to the in vivo context and quantifying the consumption of actual nuts required to see an effect presents considerable challenges. Notably, the precise quantity of nuts utilised for extraction was unspecified in the studies on human α-amylase inhibition.42,44,45 Further, while we can estimate the volume of digestive fluids within the intestine, this would be an approximation only and is highly variable between individuals and contexts. Additionally, there is a lack of literature addressing the bioaccessibility of polyphenols from chestnut and almond skins, further complicating the extrapolation of these results to α-amylase inhibition in vivo.
5. Conclusion
Within the extensive research dedicated to the study of nuts and their impact on health outcomes, only four studies on a limited range of nuts have specifically examined the inhibitory effects of compounds extracted from commonly consumed edible parts on the activity of human α-amylases. These investigations have demonstrated that polyphenol-rich chestnut skin extracts are potent inhibitors of both human salivary and pancreatic α-amylases, and that polyphenol-rich almond skin extract is also a potent inhibitor of salivary α-amylase. Notably, over half of the studies included in the present systematic review utilised porcine pancreatic α-amylase. It is crucial to highlight that while extracts from nuts have shown inhibitory effects on porcine pancreatic α-amylase activity, these cannot be confidently extrapolated to human subjects. This limitation arises due to recognised functional distinctions between human and porcine α-amylases, owing to variations in the amino acid sequences of their catalytic residues and active sites. It is worth mentioning again that non-mammalian sources of α-amylases have no relevance to human health and disease. For future in vitro studies to be relevant to human health and disease, it is important to use human α-amylase enzyme sources. It is evident that the primary mechanism through which certain bioactive compounds, notably polyphenols, inhibit α-amylase activity appears to involve complex formation with starch, particularly amylose, as well as direct inhibition of the enzyme. Therefore, future research should take this factor into consideration when selecting the substrate and should also incorporate direct assessments of α-amylase activity, potentially utilising chromatographic quantification of product formation in the assays for this purpose.7 Also, these precise in vitro studies will help to explain the mechanism behind any effects seen in humans related to the potential role of nut consumption in the prevention and management of chronic diseases.10–12,62,64
Author contributions
MJH developed the concept and GW supervised the research. MF, MJH, BRC and MM screened the full texts. Data were extracted and the quality of included papers assessed independently by MF and MJH. MF, MJH and GW collated and interpreted the data. MF wrote the first version of the manuscript and MJH, BRC, MM and GW edited the manuscript. All the authors contributed to the writing of the manuscript and approved the final version.
Conflicts of interest
The authors report no current relevant conflict of interest.
Acknowledgements
We thank Monash University for an International PhD Scholarship to MGF and for a research establishment fund to GW.
References
- A. K. Buyken, D. J. Mela, P. Dussort, I. T. Johnson, I. A. Macdonald, J. D. Stowell and F. J. P. H. Brouns, Dietary carbohydrates: A review of international recommendations and the methods used to derive them, Eur. J. Clin. Nutr., 2018, 72, 1625–1643 CrossRef CAS PubMed
.
- H. Bischoff, Pharmacology of alpha-glucosidase inhibition, Eur. J. Clin. Invest., 1994, 24(Suppl 3), 3–10 CAS
.
- P. M. Sales, P. M. Souza, L. A. Simeoni and D. Silveira, α-Amylase inhibitors: a review of raw material and isolated compounds from plant source, J. Pharm. Pharm. Sci., 2012, 15, 141–183 Search PubMed
.
- Y. Khajebishak, L. Payahoo, M. Alivand and B. Alipour, Punicic acid: A potential compound of pomegranate seed oil in Type 2 diabetes mellitus management, J. Cell Physiol., 2019, 234, 2112–2120 CrossRef CAS PubMed
.
- J. C. Brand-Miller, Glycemic load and chronic disease, Nutr. Rev., 2003, 61, S49–S55 CrossRef PubMed
.
- A. T. Kharroubi and H. M. Darwish, Diabetes mellitus: The epidemic of the century, World J. Diabetes, 2015, 6, 850–867 CrossRef PubMed
.
- J. A. Balfour and D. McTavish, Acarbose. An update of its pharmacology and therapeutic use in diabetes mellitus, Drugs, 1993, 46, 1025–1054 CrossRef CAS PubMed
.
- F. A. Van de Laar, P. L. Lucassen, R. P. Akkermans, E. H. Van de Lisdonk, G. E. Rutten and C. Van Weel, Alpha-glucosidase inhibitors for type 2 diabetes mellitus, Cochrane Database Syst. Rev., 2005, 2005, CD003639 Search PubMed
.
- A. Y. Cheng and I. G. Fantus, Oral antihyperglycemic therapy for type 2 diabetes mellitus, CMAJ, 2005, 172, 213–226 Search PubMed
.
- A. Pan, Q. Sun, J. E. Manson, W. C. Willett and F. B. Hu, Walnut consumption is associated with lower risk of type 2 diabetes in women, J. Nutr., 2013, 143, 512–518 CrossRef CAS PubMed
.
- R. Jiang, J. E. Manson, M. J. Stampfer, S. Liu, W. C. Willett and F. B. Hu, Nut and peanut butter consumption and risk of type 2 diabetes in women, J. Am. Med. Assoc., 2002, 288, 2554–2560 CrossRef PubMed
.
- E. Ros, Health benefits of nut consumption, Nutrition, 2010, 2, 652–682 CAS
.
- C. Aneklaphakij, T. Saigo, M. Watanabe, T. Naake, A. R. Fernie, S. Bunsupa, V. Satitpatipan and T. Tohge, Diversity of chemical structures and biosynthesis of polyphenols in nut-bearing species, Front. Plant Sci., 2021, 12, 642581 CrossRef PubMed
.
- M. Venkatachalam and S. K. Sathe, Chemical composition of selected edible nut seeds, J. Agric. Food Chem., 2006, 54, 4705–4714 CrossRef CAS PubMed
.
- R. O. Arise, J. J. Idi, I. M. Mic-Braimoh, E. Korode, R. N. Ahmed and O. Osemwegie, In vitro Angiotesin-1-converting enzyme, α-amylase and α-glucosidase inhibitory and antioxidant activities of Luffa cylindrical (L.) M. Roem seed protein hydrolysate, Heliyon, 2019, 5, e01634 CrossRef PubMed
.
- R. O. Arise, O. O. Taofeek, K. Babaita, R. I. Adeoye and O. Osemwegie, Blood pressure and sugar regulating potentials of Anarcadium occidentale nut globulin and albumin hydrolysates, Heliyon, 2021, 7, e06384 CrossRef CAS PubMed
.
- G. Williamson, Possible effects of dietary polyphenols on sugar absorption and digestion, Mol. Nutr. Food Res., 2013, 57, 48–57 CrossRef CAS PubMed
.
- E. Lo Piparo, H. Scheib, N. Frei, G. Williamson, M. Grigorov and C. J. Chou, Flavonoids for controlling starch digestion: structural requirements for inhibiting human alpha-amylase, J. Med. Chem., 2008, 51, 3555–3561 CrossRef CAS PubMed
.
- K. Tadera, Y. Minami, K. Takamatsu and T. Matsuoka, Inhibition of alpha-glucosidase and alpha-amylase by flavonoids, J. Nutr. Sci. Vitaminol., 2006, 52, 149–153 CrossRef CAS PubMed
.
- M. Farazi, M. J. Houghton, M. Murray and G. Williamson, A systematic review of the inhibitory effect of extracts from edible parts of nuts on α-glucosidase activity, Food Funct., 2023, 14, 5962–5976 RSC
.
- D. Moher, A. Liberati, J. Tetzlaff and D. G. Altman, Preferred reporting items for systematic reviews and meta-analyses: the PRISMA Statement, Open Med., 2009, 3, e123–e130 Search PubMed
.
- S. A. Adefegha, G. Oboh, S. I. Oyeleye and I. Ejakpovi, Erectogenic, antihypertensive, antidiabetic, anti-oxidative properties and phenolic compositions of almond fruit (Terminalia catappa L.) parts (hull and drupe) – in vitro, J. Food Biochem., 2017, 41, e12309–e12320 CrossRef
.
- M. Akyuz, L. Yabo-Dambagi, T. Kilic and A. Cakir, Antidiabetic, neuroprotective and antioxidant potentials of different parts of Pistacia terebinthus fruits, S. Afr. J. Bot., 2022, 147, 443–456 CrossRef CAS
.
- R. Attaallah, D. Elfadil and A. Amine, Screening study of enzymatic inhibition of medicinal plants for the treatment of diabetes using a glucometer biosensor approach and optical method, J. Herb. Med., 2021, 28, 100441–100448 CrossRef
.
- S. Boudiba, S. Kucukaydin, A. N. Tamfu, K. Blaise, A. M. Munvera, Y. Arab, O. Ceylan and R. M. Dinica, HPLC-DAD phenolic composition, antioxidant, anticholinesterase, antidiabetic and anti-quorum sensing properties of bitter kola (Garcinia kola) and kolanut (Cola acuminata), Pharm. Res., 2023, 15, 373–383 CAS
.
- I. Bourais, S. Elmarrkechy, D. Taha, B. Badaoui, Y. Mourabit, N. Salhi, M. M. Alshahrani, A. A. Al Awadh, A. Bouyahya, K. W. Goh, C. S. Tan, S. El Hajjaji, N. Dakka and N. Iba, Comparative investigation of chemical constituents of kernels, leaves, husk, and bark of Juglans regia L., using HPLC-DAD-ESI-MS/MS analysis and evaluation of their antioxidant, antidiabetic, and anti-inflammatory activities, Molecules, 2022, 27, 1420–3049 CrossRef PubMed
.
- J. Feng and F. Kong, Enzyme inhibitory activities of phenolic compounds in pecan and the effect on starch digestion, Int. J. Biol. Macromol., 2022, 220, 117–123 CrossRef CAS PubMed
.
- A. G. Göksu, B. Çakır and İ. Gülseren, Sequence alterations affect the antidiabetic attributes of hazelnut peptide fractions during the industrial manufacture and simulated digestion of hazelnut paste, J. Food Sci. Technol., 2023, 60, 171–180 CrossRef PubMed
.
- K. Guici El Kouacheur, H. S. Cherif, F. Saidi, C. Bensouici and M. L. Fauconnier, Prunus amygdalus var. amara (bitter almond) seed oil: fatty acid composition, physicochemical parameters, enzyme inhibitory activity, antioxidant and anti-inflammatory potential, J. Food Meas. Charact., 2023, 17, 371–384 CrossRef
.
- T. A. Kumosani, Effect of areca nut extracts on some digestive enzymes in vitro, Pak. J. Sci. Ind. Res., 2003, 46, 188–192 Search PubMed
.
- M. Ahmed, M. Adil, I. U. Haq, M. K. Tipu, M. Qasim and B. Gul, RP-HPLC-based phytochemical analysis and diverse pharmacological evaluation of Quercus floribunda Lindl. ex A. camus nuts extracts, Nat. Prod. Res., 2021, 35, 2257–2262 CrossRef CAS PubMed
.
- M. Benmohamed, H. Guenane, M. Messaoudi, W. Zahnit, C. Egbuna, M. Sharifi-Rad, A. Chouh, B. B. Seghir, A. Rebiai, S. Boubekeur, T. Azli, M. Harrat, B. Sawicka, M. Atanassova and M. Yousfi, Mineral profile, antioxidant, anti-inflammatory, antibacterial, anti-urease and anti-α-amylase activities of the unripe fruit extracts of Pistacia atlantica, Molecules, 2023, 28, 1420–3049 CrossRef PubMed
.
- O. L. Erukainure, O. A. Oyebode, M. K. Sokhela, N. A. Koorbanally and M. S. Islam, Caffeine - rich infusion from Cola nitida (kola nut) inhibits major carbohydrate catabolic enzymes; abates redox imbalance; and modulates oxidative dysregulated metabolic pathways and metabolites in Fe(2+)-induced hepatic toxicity, Biomed. Pharmacother., 2017, 96, 1065–1074 CrossRef CAS PubMed
.
- M. R. Loizzo, R. Tundis, M. Leporini, G. D'Urso, R. Gagliano Candela, T. Falco, S. Piacente, M. Bruno and F. Sottile, Almond (Prunus dulcis cv. Casteltermini) skin confectionery by-products: New opportunity for the development of a functional blackberry (Rubus ulmifolius Schott) jam, Antioxidants, 2021, 10, 2076–3921 CrossRef PubMed
.
- M. Liu, Q. Yang, Y. Wu and J. Ouyang, Effects of endogenous polyphenols in acorn (Quercus wutaishanica Blume) kernels on the physicochemical properties of starch, Starch/Staerke, 2022, 74, 2200005 CrossRef CAS
.
- L. Noguera-Artiaga, D. Pérez-López, A. Burgos-Hernández, A. Wojdyło and Á. A. Carbonell-Barrachina, Phenolic and triterpenoid composition and inhibition of α-amylase of pistachio kernels (Pistacia vera L.) as affected by rootstock and irrigation treatment, Food Chem., 2018, 261, 240–245 CrossRef CAS PubMed
.
- L. Noguera-Artiaga, M. D. Salvador, G. Fregapane, J. Collado-González, A. Wojdyło, D. López-Lluch and Á. A. Carbonell-Barrachina, Functional and sensory properties of pistachio nuts as affected by cultivar, J. Sci. Food Agric., 2019, 99, 6696–6705 CrossRef CAS PubMed
.
- G. Oboh, K. E. Nwokocha, A. J. Akinyemi and A. O. Ademiluyi, Inhibitory effect of polyphenolic-rich extract from Cola nitida (Kolanut) seed on key enzyme linked to type 2 diabetes and Fe(2+) induced lipid peroxidation in rat pancreas in vitro, Asian Pac. J. Trop. Biomed., 2014, 4, S405–S412 CrossRef PubMed
.
- L. L. Pino Ramos, F. Jiménez-Aspee, C. Theoduloz, A. Burgos-Edwards, R. Domínguez-Perles, C. Oger, T. Durand, Á. Gil-Izquierdo, L. Bustamante, C. Mardones, K. Márquez, D. Contreras and G. Schmeda-Hirschmann, Phenolic, oxylipin and fatty acid profiles of the Chilean hazelnut (Gevuina avellana): Antioxidant activity and inhibition of pro-inflammatory and metabolic syndrome-associated enzymes, Food Chem., 2019, 298, 125026 CrossRef CAS PubMed
.
- V. Subbiah, P. Nagaraja, P. Narayan and H. G. R. Nagendra, Evaluation of pharmacological properties of Caesalpinia bonducella seed and shell extract, Pharmacogn. J., 2019, 11, 150–154 CrossRef CAS
.
- S. Sut, S. Dall'Acqua, K. Bene, S. B. di Marco, K. I. Sinan, M. F. Mahomoodally, M. C. N. Picot-Allain and G. Zengin, Ricinodendron heudelotii, (Baill.) heckelstem barks and seed extracts, a native food plant from Africa: Characterization by NMR and HPLC-DAD-ESI-MS(n), Food Res. Int., 2020, 129, 108877 CrossRef CAS PubMed
.
- T. Tsujita, T. Shintani and H. Sato, α-Amylase inhibitory activity from nut seed skin polyphenols. 1. Purification and characterization of almond seed skin polyphenols, J. Agric. Food Chem., 2013, 61, 4570–4576 CrossRef CAS PubMed
.
- T. Tsujita and T. Takaku, Mechanism of the inhibitory action of chestnut astringent skin extract on carbohydrate absorption, J. Nutr. Sci. Vitaminol., 2008, 54, 416–421 CrossRef CAS PubMed
.
- T. Tsujita, T. Takaku and T. Suzuki, Chestnut astringent skin extract, an alpha-amylase inhibitor, retards carbohydrate absorption in rats and humans, J. Nutr. Sci. Vitaminol., 2008, 54, 82–88 CrossRef CAS PubMed
.
- T. Tsujita, M. Yamada, T. Takaku, T. Shintani, K. Teramoto and T. Sato, Purification and characterization of polyphenols from chestnut astringent skin, J. Agric. Food Chem., 2011, 59, 8646–8654 CrossRef CAS PubMed
.
- A. A. Vazquez-Flores, J. E. Wong-Paz, M. A. Lerma-Herrera, A. I. Martinez-Gonzalez, F. J. Olivas-Aguirre, C. N. Aguilar, A. Wall-Medrano, G. A. Gonzalez-Aguilar, E. Alvarez-Parrilla and L. A. de la Rosa, Proanthocyanidins from the kernel and shell of pecan (Carya illinoinensis): Average degree of polymerization and effects on carbohydrate, lipid, and peptide hydrolysis in a simulated human digestive system, J. Funct. Foods, 2017, 28, 227–234 CrossRef CAS
.
- J. Wang, T. Wu, L. Fang, C. Liu, X. Liu, H. Li, J. Shi, M. Li and W. Min, Anti-diabetic effect by walnut (Juglans mandshurica Maxim.)-derived peptide LPLLR through inhibiting α-glucosidase and α-amylase, and alleviating insulin resistance of hepatic HepG2 cells, J. Funct. Foods, 2020, 69, 103944 CrossRef CAS
.
- A. Wojdyło, I. P. Turkiewicz, K. Tkacz, P. Nowicka and Ł. Bobak, Nuts as functional foods: Variation of nutritional and phytochemical profiles and their in vitro bioactive properties, Food Chem.: X, 2022, 15, 100418 Search PubMed
.
- F. Zulfqar, M. F. Akhtar, A. Saleem, B. Akhtar, A. Sharif and U. Saleem, Chemical characterization, antioxidant evaluation, and antidiabetic potential of Pinus gerardiana (pine nuts) extracts, J. Food Biochem., 2020, 44, e13199 CrossRef CAS PubMed
.
- Y. Zhang, Z. Yang, G. Liu, Y. Wu and J. Ouyang, Inhibitory effect of chestnut (Castanea mollissima Blume) inner skin extract on the activity of α-amylase, α-glucosidase, dipeptidyl peptidase IV and in vitro digestibility of starches, Food Chem., 2020, 324, 126847 CrossRef CAS PubMed
.
- M. Haseena, P. Viswanathan, C. Sangavai and M. K. Sudha, Antioxidant and antidiabetic potential of areca nut extract, Int. J. Life Sci. Pharma Res., 2021, 319–323 Search PubMed
.
- A. Abbou, N. Kadri, G. Giovanetti, G. Morel, O. Aoun, A. Servent, K. Madani, M. Dornier and N. Achir, Reduction of antinutritional factors during Pinus halepensis seeds beverage processing, a focus on phytates and oxalates, J. Food Compos. Anal., 2023, 124, 105635 CrossRef CAS
.
- A. Kumar, P. Arora and V. Kumar, Antidiabetic and antioxidant potential of Perunus dulcis (Mill.), J. Young Pharm., 2023, 15, 257–263 CrossRef CAS
.
- T. Y. Lin, H. C. Chang and K. H. Hsu, Areca nut chewing is associated with common mental disorders: a population-based study, Soc. Psychiatry Psychiatr. Epidemiol., 2018, 53, 393–401 CrossRef PubMed
.
- C. R. Trivedy, G. Craig and S. Warnakulasuriya, The oral health consequences of chewing areca nut, Addict. Biol., 2002, 7, 115–125 CrossRef CAS PubMed
.
-
N. S. Ali and A. K. Khuwaja, Betel nut (Areca catechu) usage and its effects on health, in Nuts and Seeds in Health and Disease Prevention, eds. V. R. Preedy, R. R. Watson and V. B. Patel, Academic Press, San Diego, 2011, pp. 197–204 Search PubMed
.
- L. Neri, G. Dimitri and G. Sacchetti, Chemical composition and antioxidant activity of cured chestnuts from three sweet chestnut (Castanea sativa Mill.) ecotypes from Italy, J. Food Compos. Anal., 2010, 23, 23–29 CrossRef CAS
.
- M. J. Santos, T. Pinto and A. Vilela, Sweet chestnut (Castanea sativa Mill.) nutritional and phenolic composition interactions with chestnut flavor physiology, Foods, 2022, 11, 2304–8158 CrossRef PubMed
.
- I. A. Wani, H. Hamid, A. M. Hamdani, A. Gani and B. A. Ashwar, Physico-chemical, rheological and antioxidant properties of sweet chestnut (Castanea sativa Mill.) as affected by pan and microwave roasting, J. Adv. Res., 2017, 8, 399–405 CrossRef CAS PubMed
.
- M. Mota, T. Pinto, A. Vilela, T. Marques, A. Borges, J. Caço, J. Ferreira-Cardoso, F. Raimundo and J. Gomes-Laranjo, Irrigation positively affects the chestnut's quality: The chemical composition, fruit size and sensory attributes, Sci. Hortic., 2018, 238, 177–186 CrossRef CAS
.
- D. Barreca, S. M. Nabavi, A. Sureda, M. Rasekhian, R. Raciti, A. S. Silva, G. Annunziata, A. Arnone, G. C. Tenore, İ. Süntar and G. Mandalari, Almonds (Prunus Dulcis Mill. D. A. Webb): A source of nutrients and health-promoting compounds, Nutrition, 2020, 12, 2072–6643 Search PubMed
.
- T. Gervasi, D. Barreca, G. Laganà and G. Mandalari, Health benefits related to tree nut consumption and their bioactive compounds, Int. J. Mol. Sci., 2021, 22, 1422–0067 CrossRef PubMed
.
- A. R. Josse, C. W. C. Kendall, L. S. A. Augustin, P. R. Ellis and D. J. A. Jenkins, Almonds and postprandial glycemia—a dose-response study, Metabolism, 2007, 56, 400–404 CrossRef CAS PubMed
.
- S.-C. Li, Y.-H. Liu, J.-F. Liu, W.-H. Chang, C.-M. Chen and C. Y. O. Chen, Almond consumption improved glycemic control and lipid profiles in patients with type 2 diabetes mellitus, Metabolism, 2011, 60, 474–479 CrossRef CAS PubMed
.
- A. Sofowora, Research on medicinal plants and traditional medicine in Africa, J. Altern. Complementary Med., 1996, 2, 365–372 CrossRef CAS PubMed
.
-
S. K. Pell, J. D. Mitchell, A. J. Miller and T. A. Lobova, Anacardiaceae in Flowering Plants. Eudicots: Sapindales, Cucurbitales, Myrtaceae, ed. K. Kubitzki, Springer Berlin Heidelberg, Berlin, Heidelberg, 2011, 3, pp. 7–50 Search PubMed
.
- M. Wu, Q. Yang, Y. Wu and J. Ouyang, Inhibitory effects of acorn (Quercus variabilis Blume) kernel-derived polyphenols on the activities of α-amylase, α-glucosidase, and dipeptidyl peptidase IV, Food Biosci., 2021, 43, 101224 CrossRef CAS
.
- R. Sardão, R. A. Amaral, E. M. C. Alexandre, J. A. Saraiva and M. Pintado, Effect of high-pressure processing to improve the safety and quality of an Quercus acorn beverage, LWT, 2021, 149, 111858 CrossRef
.
- P. Claudia, Acorn bread: A traditional food of the past in Sardinia (Italy), J. Cult. Heritage, 2013, 14, S71–S74 CrossRef
.
- M. Masmoudi, S. Besbes, M. A. Bouaziz, M. Khlifi, D. Yahyaoui and H. Attia, Optimization of acorn (Quercus suber L.) muffin formulations: Effect of using hydrocolloids by a mixture design approach, Food Chem., 2020, 328, 127082 CrossRef CAS PubMed
.
- D. Tejerina, S. García-Torres, M. Cabeza de Vaca, F. M. Vázquez and R. Cava, Acorns (Quercus rotundifolia Lam.) and grass as natural sources of antioxidants and fatty acids in the “montanera” feeding of Iberian pig: Intra- and inter-annual variations, Food Chem., 2011, 124, 997–1004 CrossRef CAS
.
- E. Uquiche, M. Jeréz and J. Ortíz, Effect of pretreatment with microwaves on mechanical extraction yield and quality of vegetable oil from Chilean hazelnuts (Gevuina avellana Mol), Innovative Food Sci. Emerging Technol., 2008, 9, 495–500 CrossRef CAS
.
- M. Bulló, R. Lamuela-Raventós and J. Salas-Salvadó, Mediterranean diet and oxidation: nuts and olive oil as important sources of fat and antioxidants, Curr. Top. Med. Chem., 2011, 11, 1797–1810 CrossRef PubMed
.
- A. Antony and M. Farid, Effect of temperatures on polyphenols during extraction, Appl. Sci., 2022, 12(4), 2107 CrossRef CAS
.
- E. Barber, M. J. Houghton, R. Visvanathan and G. Williamson, Measuring key human carbohydrate digestive enzyme activities using high-performance anion-exchange chromatography with pulsed amperometric detection, Nat. Protoc., 2022, 17, 2882–2919 CrossRef CAS PubMed
.
- N. G. Taş and V. Gökmen, Phenolic compounds in natural and roasted nuts and their skins: a brief review, Curr. Opin. Food Sci., 2017, 14, 103–109 CrossRef
.
- R. Visvanathan, M. J. Houghton and G. Williamson, Maltoheptaoside hydrolysis with chromatographic detection and starch hydrolysis with reducing sugar analysis: Comparison of assays allows assessment of the roles of direct α-amylase inhibition and starch complexation, Food Chem., 2021, 343, 128423 CrossRef CAS PubMed
.
-
E. Bertoft, Analyzing starch molecular structure, in Starch in Food, eds M. Sjöö and L. Nilsson, Woodhead Publishing, (Second Edition), 2018, pp. 97–149 Search PubMed
.
- G. D. Brayer, Y. Luo and S. G. Withers, The structure of human pancreatic alpha-amylase at 1.8 A resolution and comparisons with related enzymes, Protein Sci., 1995, 4, 1730–1742 CrossRef CAS PubMed
.
- N. Ramasubbu, V. Paloth, Y. Luo, G. D. Brayer and M. J. Levine, Structure of human salivary alpha-amylase at 1.6 A resolution: implications for its role in the oral cavity, Acta Crystallogr., Sect. D: Biol. Crystallogr., 1996, 52, 435–446 CrossRef CAS PubMed
.
- Q. Fei, Y. Gao, X. Zhang, Y. Sun, B. Hu, L. Zhou, S. Jabbar and X. Zeng, Effects of oolong tea polyphenols, EGCG, and EGCG3′′Me on pancreatic α-amylase activity in vitro, J. Agric. Food Chem., 2014, 62, 9507–9514 CrossRef CAS PubMed
.
- W. Zhan, Y. Liu, D. Li and Y. Liu, Advancing insights on the anti-obesity biochemical mechanism of (−)-epigallocatechin gallate (EGCG) by inhibiting α-amylase activity, RSC Adv., 2016, 6, 96918–96927 RSC
.
- S. Ponnusamy, R. Ravindran, S. Zinjarde, S. Bhargava and A. Ravi Kumar, Evaluation of traditional indian antidiabetic medicinal plants for human pancreatic amylase inhibitory effect in vitro, J. Evidence-Based Complementary Altern. Med., 2011, 2011, 515647 Search PubMed
.
- V. Nahoum, G. Roux, V. Anton, P. Rougé, A. Puigserver, H. Bischoff, B. Henrissat and F. Payan, Crystal structures of human pancreatic alpha-amylase in complex with carbohydrate and proteinaceous inhibitors, Biochem. J., 2000, 346(Pt 1), 201–208 CrossRef CAS PubMed
.
- G. D. Brayer, G. Sidhu, R. Maurus, E. H. Rydberg, C. Braun, Y. Wang, N. T. Nguyen, C. M. Overall and S. G. Withers, Subsite mapping of the human pancreatic alpha-amylase active site through structural, kinetic, and mutagenesis techniques, Biochemistry, 2000, 39, 4778–4791 CrossRef CAS PubMed
.
- R. Visvanathan, M. Qader, C. Jayathilake, B. C. Jayawardana, R. Liyanage and R. Sivakanesan, Critical review on conventional spectroscopic α-amylase activity detection methods: merits, demerits, and future prospects, J. Sci. Food Agric., 2020, 100, 2836–2847 CrossRef CAS PubMed
.
- R. S. Teixeira, A. S. da Silva, V. S. Ferreira-Leitão and E. P. da Silva Bon, Amino acids interference on the quantification of reducing sugars by the 3,5-dinitrosalicylic acid assay mislead carbohydrase activity measurements, Carbohydr. Res., 2012, 363, 33–37 CrossRef CAS PubMed
.
- D. Amoako and J. M. Awika, Polyphenol interaction with food carbohydrates and consequences on availability of dietary glucose, Curr. Opin. Food Sci., 2016, 8, 14–18 CrossRef
.
- Y. Chai, M. Wang and G. Zhang, Interaction between amylose and tea polyphenols modulates the postprandial glycemic response to high-amylose maize starch, J. Agric. Food Chem., 2013, 61, 8608–8615 CrossRef CAS PubMed
.
- Y. Lv, L. Zhang, M. Li, X. He, L. Hao and Y. Dai, Physicochemical properties and digestibility of potato starch treated by ball milling with tea polyphenols, Int. J. Biol. Macromol., 2019, 129, 207–213 CrossRef CAS PubMed
.
|
This journal is © The Royal Society of Chemistry 2024 |