DOI:
10.1039/D3FO05292C
(Paper)
Food Funct., 2024,
15, 4122-4139
Potential application of green extracts rich in phenolics for innovative functional foods: natural deep eutectic solvents as media for isolation of biocompounds from berries†
Received
30th November 2023
, Accepted 5th February 2024
First published on 4th April 2024
Abstract
The health-promoting effects of berries have attracted attention due to the possible application of their extracts as functional ingredients in food products. Natural deep eutectic solvents (NADESs) are a new generation of environmentally friendly solvents for the extraction of natural products, and they are green alternatives to organic solvents, and they can improve the solubility, stability, and bioavailability of isolated biocompounds. In this study, an efficient eco-friendly method was used for the extraction of phenolic compounds from different berries: chokeberries, blueberries, and black goji berries with a range of eutectic solvents consisting of hydrogen bond acceptors (HBAs) such as choline chloride, L-proline, L-glycine, and L-lysine and hydrogen bond donors (HBDs) such as malic, citric, tartaric, lactic and succinic acids, glucose and glycerol. The obtained results indicated the ability of NADESs towards selective extraction of phenolics; the eutectic system choline chloride
:
malic acid showed selective extraction of anthocyanins, while choline chloride
:
glycerol and choline chloride
:
urea showed selectivity towards flavonoids and phenolic acids. The methodology for screening of the NADES extraction performance, which included chromatographic profiling via high-performance thin layer chromatography combined with chemometrics and spectrophotometric essays, allowed effective assessment of optimal eutectic solvents for isolation of different groups of phenolics. Great antioxidant and antimicrobial activities of extracts, along with the green nature of eutectic solvents, enable NADES berry extracts to be used as “green-labelled” functional foods or ingredients.
1. Introduction
In recent years, awareness of the importance of diet in relation to human health has increased and food rich in bioactive compounds has received growing interest. Berries have been recognized as fruit beneficial for human nutrition and health since they are some of the richest sources of natural antioxidants.1–3 In general, berries contain bioactive components, such as anthocyanins, flavonols, procyanidins, phenolic acids, tannins and triterpenoids, as well as numerous vitamins and minerals.4 Numerous studies have shown that berry fruits have diverse beneficial effects on health, including anti-inflammatory, antioxidant, anti-hypertensive, anti-hyperglycemic, cell-protective and potential prebiotic-like effects; they also have health-promoting effects on cardiovascular diseases, diabetes, cancer, and other diseases.5,6 Recently, berries like chokeberries and black goji berries have been gaining more attention, and they have been widely consumed in traditional medicine.7–9 Berries such as blueberries, strawberries, raspberries, cranberries and blackcurrants are widely consumed by the general population in fresh or processed forms.10 The health-promoting effects of berries have led to increasing attention on the possible application of their extracts as functional ingredients in formulations and food products. Preparation of extracts as concentrated solutions of bioactive compounds would overcome the problem of low bioavailability of phenolic compounds from berries, especially anthocyanins.6,11
Natural deep eutectic solvents (NADESs) are a new green alternative to organic solvents for the extraction of bioactive compounds from natural products. NADESs are mixtures of two or more natural products (individually solid at room temperature) in a certain molar ratio which can form a liquid at room temperature. NADESs are composed of hydrogen bond acceptors (HBAs) such as quaternary ammonium salts or amino acids and hydrogen bond donors (HBDs) such as organic acids, sugars, polyalcohols and urea. NADESs represent a new approach in green chemistry that aims to overcome the drawbacks of conventional organic solvents such as toxicity, low flash points and high flammability. In recent years, NADESs have been extensively used for the extraction of biocompounds, phenolics, anthocyanins, natural colorants, alkaloids, etc., from various sources.12–15
Besides them being proved to be favourable solvents for green extractions, NADESs have demonstrated improvement in the solubility,16,17 stability,18–20 or bioavailability21,22 of targeted compounds. Higher solubility of phytochemicals in eutectic systems, together with the stabilizing effect of NADESs, could be explained by strong hydrogen bonding or dipole–dipole interactions between solutes and solvent molecules (phytochemicals and NADESs). The low bioavailability of phenolic compounds, along with a short shelf-life, represents the downside of the consumption of fresh berries, which can be overcome by NADES extraction and subsequent preparation of formulations. These rich-in-phenolics and eco-friendly extracts could have promising applications as functional foods, food preservatives or supplements, due to improved solubility, stability and bioaccessibility of phenolic compounds via NADES surroundings.
The aim of this study was to develop an efficient eco-friendly method for the extraction of phenolic compounds from berries (blueberries, chokeberries, and black goji berries) using NADESs. This goal was achieved through investigation of the extraction efficiency of the applied NADES based on three different approaches: (a) chromatographic – through high-performance thin layer chromatography (HPTLC) fingerprint analysis, ultra-high-performance liquid chromatography with a diode array detector and triple-quadrupole mass spectrometry (UHPLC-DAD-MS/MS) target analysis, (b) spectroscopic – through quality control parameters: total phenolic content (TPC), total flavonoid content (TFC), total anthocyanin content (TAC) and radical scavenging activity (RSA), and (c) microbiological – through a well diffusion method and bioautography.
2. Materials and methods
2.1. Berry materials
Chokeberries and blueberries were collected in summer 2021 in Kraljevo, Central Serbia and Valjevo, West Serbia, respectively, while dried black goji berries were bought from a local shop in Belgrade, Serbia and they were declared to be originated from China. The fresh samples of chokeberries and blueberries were dried in a stream of hot air for 5 days, at a temperature of 40 °C (Gorenje food dehydrator FDK500GCW). All samples were milled into a powder using a coffee grinder (Bosch coffee electric grinder TSM6A017C). Raw materials of dried berries were stored in sealed containers at room temperature in the dark until further analysis.
2.2. Chemicals and reagents
Aluminium chloride and maleic acid were ordered from Acros Organics (Geel, Belgium). Urea was purchased from Betahem (Belgrade, Serbia). Hydrochloric acid, acetic acid, sulfuric acid, 2-butanone, glucose, and fructose were purchased from Centrohem (Stara Pazova, Serbia). The Folin–Ciocalteu (FC) reagent was provided by Carlo Erba Reagents (Milan, Italy). Lactic acid and tartaric acid were bought from ICN Biomedicals Inc. (Aurora, OH, USA). Succinic acid, sodium hydroxide, polyethylene glycol 400 (PEG) and sodium dihydrogen phosphate were purchased from Merck (Darmstadt, Germany). Formic acid was provided by Lach-Ner (Neratovice, Czech Republic). Ethyl acetate, toluene and glycerol were purchased from Zorka Pharma (Sabac, Serbia). Choline chloride (ChCl), L-proline, L-glycine, L-lysine, L-tyrosine, citric acid, 2,2-diphenyl-1-picrylhydrazyl (DPPH), 2-aminoethyl diphenylborinate (NEU reagent), anisaldehyde, methanol, Triton X-100, 3-(4,5-dimethylthiazol-2-yl)-2,5-diphenyltetrazolium bromide (MTT), potassium chloride, sodium acetate, sodium carbonate, sodium nitrite, Trolox, gallic acid, chlorogenic acid, caffeic acid, p-coumaric acid, quercetin, rutin, quercetin-3-O-rhamnoside, quercitrin, hyperoside, isorhamnetin, isorhamnetin-3-O-rutinoside, isorhamnetin-3-O-glucoside, epicatechin, naringin, kaempferol-3-O-glucoside, myricetin, aesculetin, galangin, delphidin-3-O-glucoside, cyanidin-3-O-sophoroside, cyanidin-3,5-di-O-glucoside, malvidin-3,5-di-O-glucoside, cyanidin-3-O-glucoside, cyanidin-3-O-rutinoside, cyanidin-3-O-sambubioside, cyanidin-3-O-arabinoside, pelargonidin-3-O-glucoside, peonidin-3-O-glucoside and malvidin-3-O-glucoside were provided by Sigma-Aldrich Chemical Co. (St Louis, MO, USA). Nutrient agar slants were supplied by Lab M (Bury, UK), and Tripton LP0042 and the yeast extract LP0021 were supplied by Oxoid Ltd (Basingstoke, UK).
2.3. Preparation of NADESs
NADESs were synthesized by the heating and stirring method, and HBAs and HBDs were weighed at a certain molar ratio and 20% w/w water was added to reduce the viscosity. The mixtures were constantly stirred by magnetic stirring in an oil bath at 80 °C until homogeneous and transparent solvents were formed. The compositions of the studied NADESs are listed in Table 1, while the list of the attempted combinations that did not form an eutectic system or were too viscous to use for extractions is given in Table S1.†
Table 1 The compositions of the studied NADESs and their abbreviations
Abbreviation |
Eutectic mixture |
Molar ratio |
E1 |
Choline chloride : fructose |
1 : 1 |
E2 |
Choline chloride : fructose |
5 : 2 |
E3 |
Choline chloride : glucose |
1 : 1 |
E4 |
Choline chloride : glucose |
5 : 2 |
E5 |
Choline chloride : malic acid |
1 : 1 |
E6 |
Choline chloride : citric acid |
1 : 1 |
E7 |
Choline chloride : tartaric acid |
1 : 1 |
E8 |
Choline chloride : lactic acid |
1 : 1 |
E9 |
Choline chloride : lactic acid |
1 : 2 |
E10 |
Choline chloride : lactic acid |
1 : 3 |
E11 |
Choline chloride : glycerol |
1 : 1 |
E12 |
Choline chloride : glycerol |
1 : 2 |
E13 |
Choline chloride : glycerol |
1 : 3 |
E14 |
Choline chloride : urea |
1 : 1 |
E15 |
Choline chloride : urea |
1 : 2 |
E16 |
Urea : glycerol |
1 : 1 |
E17 |
Urea : glycerol |
1 : 2 |
E18 |
Urea : glycerol |
1 : 3 |
E19 |
L-Proline : glycerol |
1 : 1 |
E20 |
L-Proline : glycerol |
1 : 3 |
E21 |
L-Proline : glycerol |
5 : 2 |
E22 |
L-Proline : citric acid |
1 : 1 |
E23 |
L-Proline : malic acid |
1 : 1 |
E24 |
L-Proline : glucose |
1 : 1 |
E25 |
L-Proline : urea |
1 : 1 |
E26 |
L-Proline : lactic acid |
1 : 1 |
E27 |
L-Proline : lactic acid |
1 : 3 |
E28 |
L-Lysine : lactic acid |
1 : 1 |
E29 |
L-Lysine : lactic acid |
1 : 3 |
E30 |
L-Lysine : malic acid |
1 : 1 |
E31 |
L-Glycine : lactic acid |
1 : 3 |
E32 |
L-Glycine : tartaric acid |
1 : 1 |
E33 |
L-Glycine : malic acid |
1 : 1 |
E34 |
Sodium acetate : lactic acid |
1 : 3 |
E35 |
Glucose : lactic acid |
1 : 3 |
E36 |
Citric acid : fructose |
1 : 1 |
Abbreviation
|
Conventional solvents
|
K1 |
0.01% v/v hydrochloric acid in methanol |
K2 |
1% w/w citric acid in water |
2.4. Extraction of phenolic compounds from berries
Extractions were performed using a laboratory ultrasonic bath. Ground berry materials were weighed in conical centrifuge tubes (10 mL) and NADESs or conventional solvents were added at a solid/liquid ratio of 100
:
1 mg mL−1. Before the extraction, the samples were vortexed for 30 s to homogenize the mixtures, and extractions were carried out for 30 minutes at a temperature of 50 °C. The resulting mixtures were centrifuged at 10
000 rpm for 15 min (Thermo Scientific SL 16 centrifuge, Waltham, USA) to separate the solid and liquid phases, and prior to centrifugation, water was added to ease the separation of the phases.23 The supernatants were purified using solid-phase extraction (SPE) to recover phenolics from the NADESs. Reversed-phase sorbents (Cartridge Bond Elut C18, Agilent Technologies) were used as the solid phase, and cartridges were conditioned with methanol and acidified water (0.01% v/v hydrochloric acid). After the samples were loaded, the cartridges were washed of non-adsorbed berry compounds and NADES compounds with acidified water (0.01% v/v hydrochloric acid) and phenolic compounds were eluted with acidified methanol (0.01% v/v hydrochloric acid).24 The obtained extracts were used without further processing for all analyses, except in microbiological experiments, where the solvent was evaporated using a vacuum evaporator (IKA-Werke HB4 Basic, Staufen, Germany) and dissolved in acidified methanol (0.01% v/v hydrochloric acid) to prepare extracts with a final concentration of 20 mg mL−1. All obtained extracts were stored in dark glass vials at −20 °C until usage. Acidified methanol (0.01% v/v hydrochloric acid) was used as a conventional solvent for extraction of phenolics and anthocyanins, together with 1% w/w solution of citric acid in water, which is known as a GRAS (generally recognized as safe) solvent.25
2.5. HPTLC analysis
NADESs and conventional extracts of berries (15 μL) were applied to a HPTLC glass plate (20 × 10 cm) precoated with silica gel 60 F254 (serial number: 1056420001; Merck, Darmstadt, Germany) using Linomat 5 (CAMAG, Muttenz, Switzerland) and a 100 μL Hamilton syringe (Bonaduz, Switzerland) as a 6 mm long band. The composition of the mobile phase was optimized to separate simultaneously all phenolic compounds, including anthocyanins, flavonoids, and phenolic acids. In total, nine mobile phases were tested (Table S2†).26–31 The best separation of all targeted compounds was achieved using the mobile phase ethyl acetate
:
water
:
acetic acid
:
formic acid (100/27/11/11, v/v/v/v). The saturation time of the solvent vapor in the chromatographic chamber (CAMAG Twin Trough Chamber) was 10 minutes and the solvent front was 80 mm. After the development, the plates were dried under hot air for 5 minutes to remove the mobile phase and then heated at 120 °C for 5 minutes. The plates were derivatized with the NEU reagent (0.5% w/v methanolic solution), followed by 5% w/v PEG solution in methanol using a TLC chromatogram immersion device (CAMAG). Derivatized HPTLC chromatograms were dried in a stream of warm air, visualized and documented under UV light at 366 nm and visible light using a mobile phone camera (64 MPs) and stored in JPEG format for further image processing.
2.6. Spectrophotometric assays
All spectrophotometric assays were measured on a GBC UV-Visible Cintra 6 spectrophotometer (Dandenong, VIC, Australia) and carried out in triplicate.
2.6.1. Total phenolic content.
The total phenolic content (TPC) was determined in all berry extracts using the Folin–Ciocalteu method. The extracts (0.5 mL) were diluted with distilled water (0.5 mL) and mixed with 2.5 mL of Folin–Ciocalteu reagent (10% w/v). After 5 minutes, 2.0 mL of sodium carbonate solution (7.5% w/w) was added, and the mixtures were incubated for 2 h at room temperature. The absorbance was measured at 765 nm and gallic acid was used as the standard in the range of 20–120 mg L−1. TPC values were expressed as gram of gallic acid equivalent (GAE) per kg of dry sample.32
2.6.2. Total flavonoid content.
The total flavonoid content (TFC) in the extracts was determined using the aluminium chloride method. The extracts (0.3 mL) were diluted with 3.4 mL of 30% v/v methanol solution and then mixed with 0.15 mL of 0.5 mol L−1 sodium nitrite solution and 0.15 mL of 0.3 mol L−1 aluminium chloride solution. After incubation for 5 minutes at room temperature, 1.0 mL of 1 mol L−1 sodium hydroxide solution was added. The absorbance was measured at 506 nm and quercetin was used as the standard in the range of 10–500 mg L−1. TFC values were expressed as gram of quercetin equivalent (QUE) per kg of dry sample.33
2.6.3. Total anthocyanin content.
The total anthocyanin content (TAC) in the berry extracts was determined by the pH differential method. The extracts (0.3 mL) were diluted with 1.2 mL of pH 1.0 buffer solution (potassium chloride, 0.025 mol L−1) or pH 4.5 buffer solution (sodium acetate, 0.4 mol L−1) and incubated for 10 minutes. The absorbance of the test portion diluted with pH 1.0 buffer and pH 4.5 buffer was measured at both 520 and 700 nm. The diluted test portions were read versus a blank cell filled with distilled water. The total anthocyanin content was expressed as cyanidin-3-glucoside equivalents and calculated as:
where A = (A520nm − A700nm) pH 1.0 − (A520nm − A700nm) pH 4.5; MW (molecular weight) = 449.2 g mol−1 for cyanidin-3-glucoside; DF = dilution factor; l = path length in cm and ε = 26
900 molar extinction coefficient, in L mol−1 cm−1 for cyanidin-3-glucoside.34 TAC values were expressed as gram of cyanidin-3-glucoside equivalent (cyd-3-glu) per kg of dry sample.
2.6.4. Radical scavenging activity.
Radical scavenging activity (RSA) was determined using DPPH radical solution. The extracts (0.1 mL) were mixed with 4 mL of 79 μmol L−1 methanolic solution of DPPH. After incubation for 1 h at room temperature in the dark, the absorbance was measured at 517 nm. Trolox was used as the standard in the range of 100–600 μmol L−1. The results were expressed as millimoles of Trolox equivalents (TEs) per kg of dry sample.35
2.7. HPTLC-antioxidant assays
2.7.1. HPTLC-FC assay.
The same chromatographic conditions used for the determination of the phenolic profile were applied for this assay. After development, the plate was heated at 120 °C for 5 minutes and immersed in an aqueous solution of the Folin–Ciocalteu reagent (3
:
2, v/v). After derivatization, the HPTLC chromatogram was dried under hot air for 5 minutes and documented under visible light. The active compounds appeared as a navy-blue colour zone against a yellow-coloured background.36
2.7.2. HPTLC-DPPH assay.
For the HPTLC-DPPH assay, the same chromatographic conditions were applied and after development, the plate was immersed in a 0.1% w/v methanolic solution of DPPH. The chromatograms were documented under visible light every 15 minutes for 2 h. The active compounds appeared as yellow bands against a violet background.36
2.8. Quantification of phenolic compounds
Separation of phenolics and anthocyanins was performed using an ultrahigh-performance liquid chromatography (UHPLC) system that consisted of a quaternary Accela 600 pump and an Accela autosampler (Thermo Fisher Scientific, Bremen, Germany) as previously described in the literature.37 The UHPLC system was coupled to a TSQ Quantum Access Max triple-quadrupole mass spectrometer equipped with a heated electrospray ionization (HESI) source, with the vaporizer temperature kept at 200 °C. The ion source settings were as follows: spray voltage – 5000 V, sheet gas (N2) pressure – 40 AU, ion sweep gas pressure – 1 AU, and auxiliary gas (N2) pressure – 8 AU, capillary temperature – 300 °C, and skimmer offset – 0 V. The mass spectrometry data were acquired in the negative mode for phenolics and in the positive mode for anthocyanin analysis. A Syncronis C18 column (100 × 2.1 mm, 1.7 μm particle size) at 40 °C was used for separation, the flow rate was set at 0.250 mL min−1, and the mobile phase consisted of (A) water + 0.1% formic acid and (B) acetonitrile. The injection volume was 5 μL, and the linear gradient program was as follows: 0.0–1.0 min 5% B, 1.0–14.0 min from 5% to 95% (B), 14.0–14.1 min from 95% to 5% (B), and 5% (B) for 6 min. The phenolics and anthocyanins were identified via direct comparison with commercial standards. The total amounts of each compound were evaluated via calculation of the peak areas, and they are expressed as μg L−1.
2.9. Antimicrobial activity
The antimicrobial potential of the selected berry extracts was tested against Bacillus subtilis ATCC 6633, Bacillus cereus ATCC 1177, Staphylococcus aureus ATCC 6538, methicillin-resistant Staphylococcus aureus (MRSA) ATCC 33591, Staphylococcus epidermidis ATCC 12228, Listeria monocytogenes ATCC 13932, Escherichia coli ATCC 35218, Enterobacter cloacae ATCC 13047, Klebsiella pneumoniae ATCC 29665, Salmonella typhimurium ATCC 14028, Salmonella enterica ATCC 13076 and Pseudomonas aeruginosa ATCC 10145, using the well diffusion method.38 Sterile molds were placed on LA agar plates (LB medium with addition of 15 g L−1 of agar) and 7 mL LA soft agar, previously inoculated with 7 or 70 μL of the corresponding bacterial suspension, was uniformly spread on the LA agar. The molds were removed after solidification of the soft agar and 20 μL of the corresponding extracts was added to the wells. As a negative control, 20 μL of acidified methanol (0.01% v/v hydrochloric acid) was used, while the antibiotic vancomycin (400 μg mL−1) was used as a positive control. The plates were incubated at 37 °C for 24 hours. The activity of the extract was evaluated by the appearance of a zone of inhibition of bacterial growth around the well. The diameters of the inhibition zones were measured and expressed in mm.
2.10. HPTLC-direct bioautography
Bioautography was performed against five bacterial strains, encompassing both Gram-negative strains, E. coli ATCC 35218 and K. pneumoniae ATCC 29665, and Gram-positive strains, S. aureus ATCC 6538, MRSA ATCC 33591 and B. subtilis ATCC 6633 according to a modified procedure described in the literature.39 The standard bacterial strains were cultivated on nutrient agar slants for 24 hours at 37 °C. Each well-grown culture was subsequently suspended in 5 mL of sterile physiological solution. Afterward, 10 mL of Luria Bertani broth (LB) was inoculated with 0.1 mL of the bacterial cell suspension, and then it was incubated for 18 hours using a Biosan Orbital Shaker-Incubator ES-20, maintained at 37 °C and 220 rpm. The resulting inoculums were combined with fresh LB in a 1
:
1000 ratio, and the incubation was continued as described. Bacterial growth was tracked by measuring the optical density (OD) of the suspensions using a Biosan DEN-1B McFarland densitometer. The inoculums were permitted to grow until reaching an OD of 4.30 McF, which corresponds to the logarithmic growth phase of the tested strains. At this moment, the developed HPTLC chromatograms were immersed in the bacterial broth cultures briefly and subsequently incubated in humid thermostat plastic containers at 37 °C for 90 minutes. Antibacterial zones were made visible by immersing plates in a thermostat 0.1% MTT solution in a phosphate buffer (0.1 mol L−1, pH 7.2). For the Gram-negative bacteria, Triton X-100 was added into the MTT solution at a proportion of one drop per one mL of the solution. Additional incubation was continued under the same conditions until the active zones became visible.
Composition of the mobile phase used for direct bioautography was optimized to achieve the best separation of compounds with antimicrobial activity and the eight studied mobile phases are listed in Table S3.†29,40–43 The best separation was achieved using the mobile phase toluene
:
ethyl acetate
:
formic acid (6/4/1, v/v/v). Prior to the application of the extracts, the plates were washed with methanol and dried under hot air and the extracts (concentration: 20 mg mL−1, volume: 12 μL) were applied as 8 mm long bands. Chemical derivatization was performed by immersing the plates in an anisaldehyde sulfuric acid reagent (anisaldehyde
:
acetic acid
:
methanol
:
sulfuric acid, 0.5/10/85/5, w/v/v/v) and heated at 110 °C until the development of a zone, which was visualized under visible light.44
2.11. Image processing
Images of the HPTLC chromatograms were processed using the Image J processing program (https://imagej.net/Downloads).45 Before signal acquisition and analysis, each image was split-filtered through red, green, and blue channels. Data was denoised by means of the median filter function with a 3 pixels width filter, without further background subtraction. The line profile plots were generated with the Plot Profile option for each sample. The profile plot displays a two-dimensional graph of the intensities of pixels along a chromatographic line.
2.12. Statistical analysis
Principal component analysis (PCA) was performed using the PLS ToolBox statistical package (Eigenvectors Inc., v. 5.7) within MATLAB version 7.4.0.287 (R2007a) (MathWorks Inc., Natick, MA, USA). It was carried out as an exploratory data analysis using a singular value decomposition algorithm (SVD) and a 0.95 confidence level for Q and T2 Hotelling limits for outliers. Chemometric analysis was performed on a HPTLC fingerprint dataset consisting of 36 NADES extract samples × 670 variables, which represent the pixel intensities along the 670 length lines. Data were preprocessed to improve the multivariate models and PCA was applied on the results obtained for each channel, separately. The best PCA models were achieved for chokeberries using the green channel and following preprocessing: variable alignment (correlation optimized warping (COW), slack = 5), standard normal variate and mean center; for blueberries using the green channel and following preprocessing: variable alignment (COW, slack = 5), normalize (1-Norm, area = 1) and mean center; and for black goji berries using the blue channel and following preprocessing: variable alignment (COW, slack = 5), normalize (1-Norm, area = 1) and mean center.
Descriptive statistics and a paired t-test were performed using Analysis ToolPak, Excel for Microsoft 365.
3. Results and discussion
3.1. Selection of NADES systems
The chemical composition of NADESs determines their physicochemical properties, such as polarity, viscosity, and pH, and consequently greatly influences the extraction efficiency of bioactive compounds.46,47 Therefore, the selection of the extraction solvent was a crucial step in this study. Choline chloride is a very common hydrogen bond acceptor used in the preparation of NADESs when the target compounds are phenolics.48 Choline chloride combined with organic acids proved to be good solvents for the extraction of anthocyanins, due to the lower pH values of the mixture compared to NADESs based on polyols and sugars.49 Therefore, in the current study, primarily choline chloride was chosen as a HBA in combination with five organic acids (malic, citric, tartaric, lactic, and succinic), glucose, glycerol, and urea as a HBD, for the preparation of eutectic mixtures. In order to further evaluate different NADESs, four amino acids (L-proline, L-glycine, L-lysine and L-tyrosine) were employed as acceptors with the previously mentioned HBD. Not all combinations of HBAs and HBDs were able to form eutectic mixtures (Table 1 shows the successfully synthesized mixtures and Table S1† shows other attempts). Additionally, NADESs commonly studied in the extraction of phenolics, such as sodium acetate
:
lactic acid, glucose
:
lactic acid, citric acid
:
fructose, urea
:
glycerol and L-proline
:
glycerol, were also synthesized.50–52 The viscosity of NADESs is a major drawback that limits their extraction efficiency due to the lower mass transport efficiency. Water content and temperature are the main factors that influence viscosity. The optimal content of water in terms of the change of interactions between the target compounds and NADESs and the miscibility of the mixture were established to be approximately 20% (w/w), whereas an increase of temperature to 50 °C decreased the viscosity noticeably.50,53 Based on these facts, all NADESs were diluted with 20% (w/w) deionized water before use, while the extraction temperature was set at 50 °C. All other extraction parameters were held constant in an attempt to study only the influence of NADES composition on extraction. As conventional solvents acidified methanol (0.01% v/v HCl), along with 1% w/v citric acid in water, was used.
3.2. High-performance thin layer chromatography fingerprint analysis
HPTLC was used as a screening method in the evaluation of the extraction efficiency of NADES extracts, since it is a simple, accessible, and inexpensive method and the most important environmentally friendly alternative, for fingerprint analysis. Herein, HPTLC analysis was combined with PCA to estimate the extraction selectivity among the different eutectic mixtures.
Concerning the complex and diverse chemical composition of berries which include, among others, phenolic compounds of different polarities (phenolic acids, flavonoids, anthocyanins, etc.), the goal was to achieve the maximum separation efficiency of all compounds present in the berry species included in the study. Different mobile phases were evaluated (Table S2†), and optimal results with numerous sharp bands and good separation of anthocyanins from other phenolic classes were achieved using the mobile phase ethyl acetate
:
water
:
acetic acid
:
formic acid (100/27/11/11, v/v/v/v). Visual examination of the HPTLC chromatograms (Fig. 1) revealed different phenolic profiles depending on the botanical origin and extraction solvent, indicating the specific chemical composition of certain species and different extraction performances of NADESs. The chokeberry samples showed the richest phenolic profiles (Fig. 1A) with noticeable differences in the number and intensity of bands depending on the applied solvent mixture. Contrarily, the extracts of blueberry had a low number of bands, but variation in the phenolic profile was present (Fig. 1B), especially in L-proline (E19–21)- and L-lysine (E28–29)-based NADES extracts. The phenolic profiles of black goji berries (Fig. 1C) were particularly rich in zones at a lower hRF, and blue band at hRF = 55 (identified as chlorogenic acid, Fig. S1†), characteristic of this botanical origin as well as the particular NADES extract.
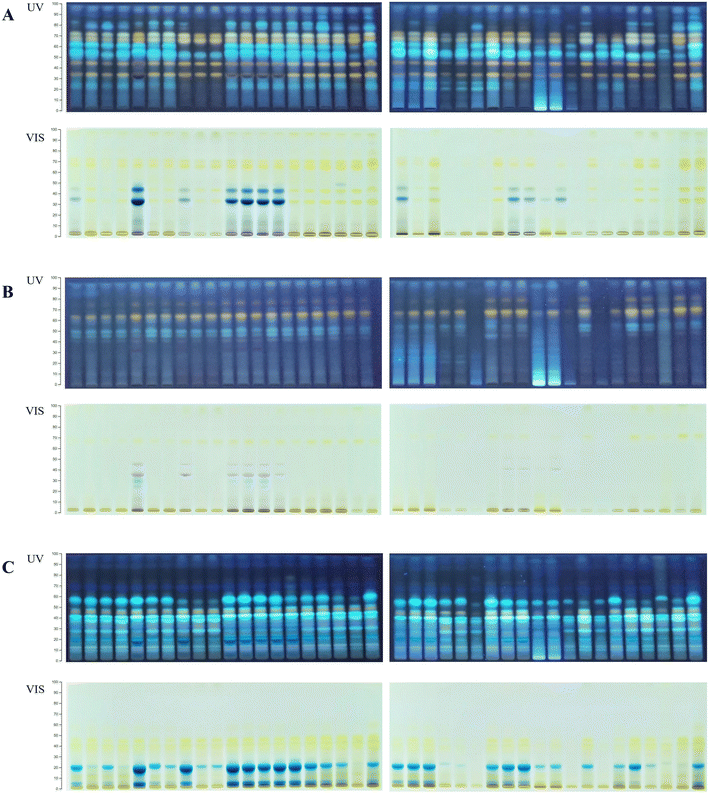 |
| Fig. 1 HPTLC profiles of the NADES and conventional extracts for (A) chokeberry: first plate extracts: E1–E18 and K1 and K2 and second plate extracts: E19–E38 and K1 and K2; (B) blueberry: first plate extracts: E1–E18 and K1 and K2 and second plate extracts: E19–E38 and K1 and K2; and (C) black goji berry: first plate extracts: E1–E18 and K1 and K2 and second plate extracts: E19–E38 and K1 and K2. Abbreviations of the extracts are listed in Table 1. | |
The HPTLC chromatograms of all berries revealed richer phenolic profiles of NADES extracts compared to acidified methanol (K1), while compared to acidified water (K2), the extraction efficiency was mainly similar. The chokeberry NADES extracts E1–7, E11–21 and E25 had comparable profiles to acidified water concerning the intensity of bands, while E8–10, E23–24, E26–29, E31–34 and E36 showed a weaker extraction efficiency compared to acidified water, but like acidified methanol. Only a few extracts (E22, E30 and E35) showed poorer profiles compared to both conventional solvents. The majority of blueberry NADES extracts had similar phenolic profiles to conventional solvents, with E6–7, E11, E14, E25 and E34 having a more intensive profile and E24, E30, E32 and E36 having very poor profiles. HPTLC profiles under visible light showed that the NADES extracts E5, E8 and E11–14 have more anthocyanin bands compared to conventional solvents. All NADES extracts of black goji berries showed similar profiles to acidified water, except for E8–10, E30, E32, and E34–36, which had profiles more comparable to acidified methanol. Visual comparison of the HPTLC chromatograms indicated that a vast majority of NADESs expressed equal or even better extraction efficiency compared to conventional solvents; however, the low toxicity, biodegradability and tuneable properties of NADESs give them a considerable advantage for application and creation of green extracts.
Visual assessment of the HPTLC data suggests that the composition of eutectic solvents has a considerable influence on phenolic profiles, and although most extracts exhibit similar profiles, the differences could be observed. To investigate possible grouping patterns among the extracts and similarities/dissimilarities between profiles according to the composition of the applied NADESs, PCA was employed on a multivariate space of chromatographic profiles. The matrix consisted of 36 objects (tested NADES extracts) and 670 variables representing the intensity of pixels along the 670 length lines obtained by digitization of HPTLC chromatograms. Three PCA models are graphically presented as mutual projections of factor scores for the selected principal components (PCs) (Fig. 2 and Fig. S2†).
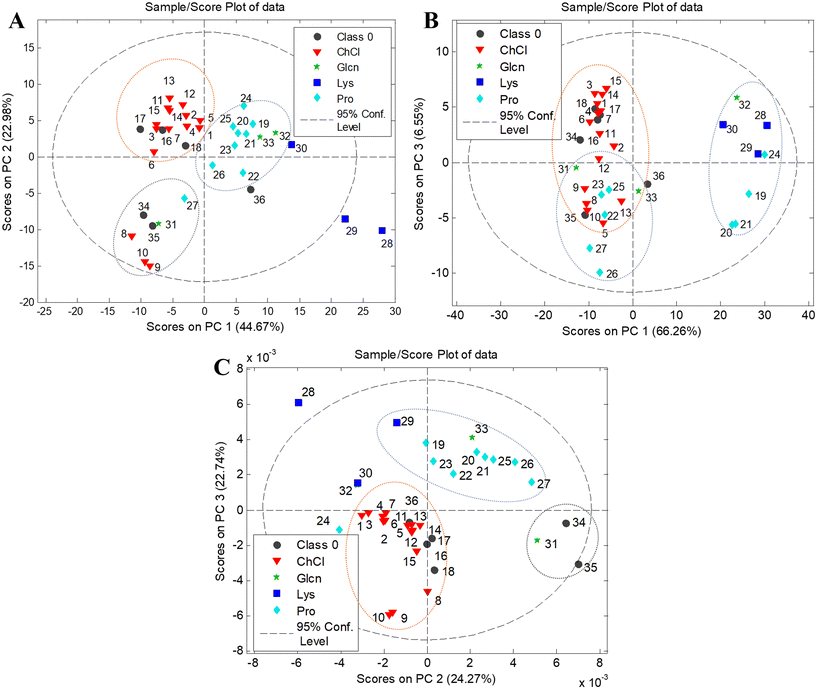 |
| Fig. 2 Principal component analysis of the NADES extracts for the HPTLC phenolic profile score plots for (A) chokeberry; (B) blueberry; and (C) black goji berry. Abbreviations: ChCl: choline chloride, Glcn: L-glycine, Lys: L-lysine, Pro: L-proline. | |
The five-component model obtained for the HPTLC phenolic profiles of chokeberries explained 89.28% of total variance; 44.67% of the overall data variance was accounted for by PC1, and 22.98% by PC2. The score plot revealed the existence of three clusters corresponding to different NADES extracts (choline chloride-, amino acid- and lactic acid (LA)-based extracts) (Fig. 2A). Alongside the PC1 axis, choline chloride-based NADES extracts were separated from the group composed of three amino acids (L-glycine (Glcn)-, L-proline (Pro)-, and L-lysine (Lys)-based extracts), showing that the choice of HBA has an essential influence on the extraction performance of eutectic solvents. Additionally, lactic acid-based extracts E8–E10, E27, E31 and E34–E35 clustered as an individual group, although they contained choline chloride or amino acids as the HBA, indicating the predominant role of lactic acid in these eutectic solvents. Different phenolic profiles of LA-based extracts are best reflected in the lack of a blue zone at hRF = 61 (Fig. 1A). The loading plot for the PC2 axis (Fig. S2A†) also showed that the presence/absence of this zone in the extracts has the most positive influence on separation in the obtained PCA model.
The PCA model obtained for the HPTLC phenolic profiles of black goji berries had 5 components and explained 90.60% of total variance (PC1 – 35.89%, PC2 – 24.27%, PC3 – 22.74%). A similar classification, as with chokeberry extracts, could be observed on the score plot of black goji berries (Fig. 2C). Choline chloride-based NADES extracts were separated from the amino acid-based group along the PC3 axis, while E31, E34 and E35 formed an individual LA-based group. Furthermore, the score plot revealed further separation of L-lysine extracts from the amino acid-based cluster along the PC2 axis, which displays the impact of the structural differences of amino acids on the extraction profiles, as L-lysine has positive charge on the side chain and consequently basic properties, compared to nonpolar amino acids such as L-proline and L-glycine. This separation of L-lysine-based extracts was also observed in the chokeberry profiles.
Regarding the HPTLC phenolic profiles of blueberries, the obtained PCA model had 5 components and explained 91.50% of the total variance (PC1 – 66.26%, PC2 – 11.27%, PC3 – 6.55%). The PCA model resulted in inferior classification according to the composition of the eutectic mixtures. Two clusters separated along the PC1 axis could be observed; the first cluster contains choline chloride- and amino acid-based extracts, which are partially separated along the PC3 axis, while the second cluster contains L-lysine extracts (E28–30) and several other amino acid-based extracts (E19–21, E24 and E32). The separation of the second group was consistent with the richer phenolic profiles of these extracts, especially at a lower hRF.
The PCA models resulted in a specific classification of NADES extracts for each berry species, indicating that NADESs could be used as designer solvents. The properties of eutectic systems and their ability to extract compounds could be selectively tuned to target specific bioactive components, giving them great potential for application in food processing.
Additionally, chemometric evaluation revealed the importance of HBA components, choline chloride or amino acids, in the extraction performances of NADESs. A high influence of HBAs was expected due to the differences in structures, which affect the number and strength of the hydrogen bonds formed in the eutectic systems. The hydrogen atom of the HBD forms a hydrogen bond with the Cl atom of choline chloride,54 while in the case of amino acids, it was formed with the amino and carboxyl groups, which could affect the properties of the eutectic system, and thus the performance of extraction.55 Additionally, it has been reported that L-proline-based NADESs are promising solvents for the isolation of flavonoids, since they could improve the solubility and bioavailability of certain flavonoids.23 Clustering of lactic acid-based NADESs as an individual group seen in the chokeberry and black goji berry extracts can be due to several different reasons. The presence of liquid compounds (lactic acid, etc.) in the formation of eutectic mixtures could lower the viscosity of the system and improve extraction.47 On the other hand, Macchioni and co-authors56 suggested that lactic acid-based NADESs have high structural organization, in which water may act as a critical component, which can lead to differences in the extraction performance. In the work of Bakirtzi and co-authors,57 it was stated that phenolics can act as a type of HBD and interact competitively with lactic acid for the anion. They assumed that anions such as chloride from choline chloride, could be enveloped in the hydroxyl and carboxyl groups from lactic acid, and therefore obstruct the interactions of phenolics with eutectic solvents.
Therefore, combination of HPTLC, used as non-targeted chromatographic profiling, with PCA analysis gave a comprehensive approach in the assessment of the extraction performance of NADESs.
3.3. Spectroscopic assays
For further evaluation of the extraction efficiency, spectroscopic assays were performed; a total of 36 NADESs and 2 conventional extracts were evaluated, comparing the values of four quality control parameters: total phenolic content (TPC), total flavonoid content (TFC), total anthocyanin content (TAC) and radical scavenging activity (RSA) (Fig. 3 and Table S4†).
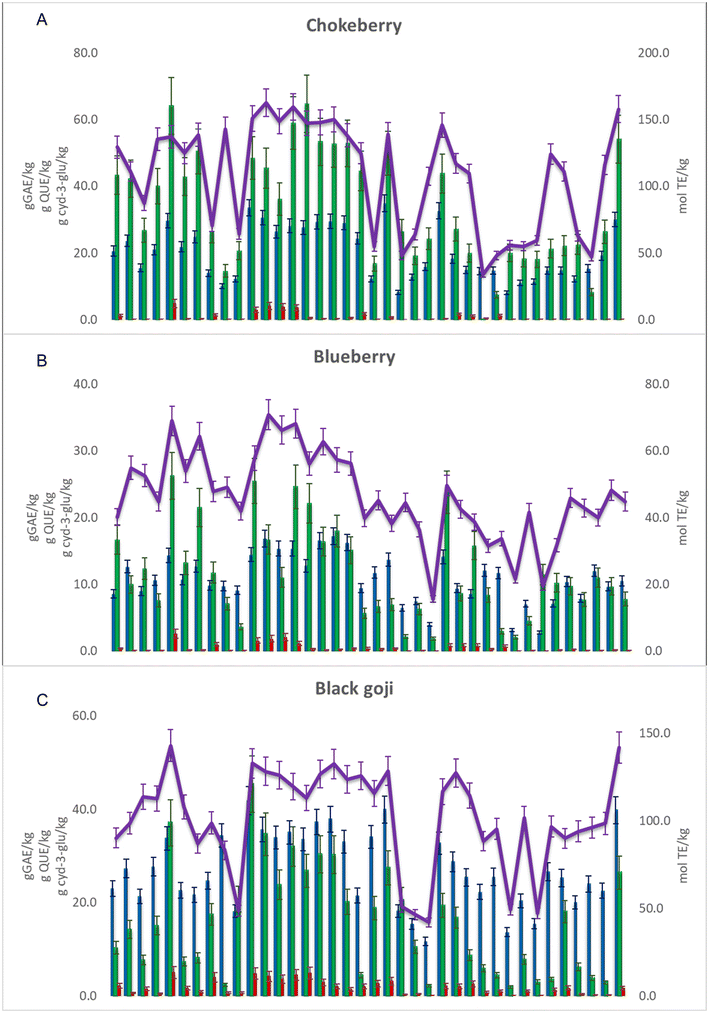 |
| Fig. 3 TPC: blue bar, TFC: green bar, TAC: red bar and RSA: purple line for the NADES and conventional extracts – (A) chokeberry; (B) blueberry; and (C) black goji berry (abbreviations are listed in Table 1). | |
The NADES extracts of chokeberries had TPC, TFC, TAC and RSA values in the range 8.1–34.9 g GAE per kg, 0.1–64.8 g QUE per kg, 0.02–4.84 g cyd-3-glu per kg and 33.9–162.6 mol TE per kg, respectively. Extract E21 showed the highest TPC value (34.9 ± 2.5 g GAE per kg), while E14 showed the highest TFC value (59.0 ± 7.8 g QUE per kg), E5 the highest TAC value (4.84 ± 1.25 g cyd-3-glu per kg) and E12 the highest RSA value (163 ± 11 mol TE per kg). The high value of Pearson's correlation coefficient was observed between RSA and TPC values (r = 0.8157), as well as between RSA and TFC values (r = 0.8119), while between RSA and TAC values it was considerably lower (r = 0.4236), implying that phenolics, especially flavonoids, are the main compounds responsible for the antioxidant activity of these extracts.
The blueberry extracts had lower values of TPC, TFC, TAC and RSA compared to the other two studied berry species and they ranged between 2.8–17.2 g GAE per kg−1, 1.9–26.3 g QUE per kg, 0.02–2.62 g cyd-3-glu per kg and 15.6–70.9 mol TE per kg, respectively. Extract E5 showed the highest TFC (26.3 ± 3.5 g QUE per kg) and TAC values (2.63 ± 0.68 cyd-3-glu per kg), while E17 showed the highest TAC value (17.2 ± 1.3 GAE per kg) and extract E12 had the highest RSA value (71 ± 5 mol TE per kg). A high value of Pearson's correlation coefficient was observed between RSA and TPC values (r = 0.8119), while it was lower between RSA and TFC values (r = 0.6789) and RSA and TAC values (r = 0.5538), showing that phenolics, particularly phenolic acids, are responsible for the antioxidant activity of these extracts.
The NADES extracts of black goji berries had TPC, TFC, TAC and RSA values in the range 11.7–41.8 g GAE per kg, 1.9–45.4 g QUE per kg, 0.06–4.98 g cyd-3-glu per kg and 42.0–143.1 mol TE per kg, respectively. Extract E11 showed the highest TPC (41.8 ± 3.0 g GAE per kg) and TFC values (45.4 ± 6.0 g QUE/kg), while E5 exhibited the highest TAC (4.98 ± 1.28 g cyd-3-glu per kg) and RSA values (143 ± 9 mol TE per kg). A high value of Pearson's correlation coefficient was observed between RSA and TPC values (r = 0.8244), while between RSA and TFC or RSA and TAC values it was lower (r = 0.5806 and r = 0.6730, respectively).
An eutectic mixture composed of choline chloride
:
malic acid in a molar ratio of 1
:
1 was appointed as the best medium for the extraction of anthocyanins from all studied berries, according to the results of TAC assays. Organic acid-based NADESs usually exhibit a higher efficiency in anthocyanin extraction compared to sugar- or polyalcohol-based NADESs, due to their lower pH values.58 Anthocyanins are highly polar compounds and their chemical structure and stability depend on the pH value. They are predominantly in the form of flavylium ion (stable at pH < 2) and their structure changes with increasing pH values and degrades at pH > 7. Since the flavylium ion is stable under acidic conditions, the positive effect of acidic NADESs on anthocyanin extraction is expected. Moreover, several studies59,60 suggested that eutectic solvents with malic acid used as HBDs were the most promising in the extraction of anthocyanins, while others suggested that oxalic61 or citric acid was better.62
The results of the TPC assay indicated that NADESs with glycerol as the HBD (urea
:
glycerol: 1
:
2 and 1
:
3, choline chloride
:
glycerol: 1
:
1 and 1
:
2 and L-proline
:
glycerol: 5
:
2) were the best solvents for the extraction of phenolics. NADESs could be highly viscous as a consequence of the extensive hydrogen-bonding network between the compounds. It can be a drawback in the application of NADESs as media for extraction, due to the reduced mass transfer which can result in lower extraction yields in general. The viscosity of eutectic mixtures is mainly affected by the chemical nature of NADES components (type of HBA, type of HBD, molar ratio), but also temperature and water content, as previously mentioned. According to the study of Zhang and co-authors,63 viscosity of choline chloride-based eutectic mixtures depend on the nature of the HBD and choline chloride
:
ethylene glycol mixtures exhibit the lowest viscosity. However, when sugar alcohols or carboxylic acids are used as HBDs, the corresponding NADESs have high viscosity as a result of a more rigid hydrogen-bond network. Choline chloride
:
glycerol mixtures display greater viscosity compared to those containing ethylene glycol, but much lower than sugar alcohols or carboxyl acid ones. A possible lower viscosity of glycerol-based NADESs can contribute to their better performance as media for extraction and thus higher values of TPC were obtained in this study.
The highest TFC values were determined in the eutectic systems choline chloride
:
malic acid – 1
:
1, choline chloride
:
urea – 1
:
1 and 1
:
2, and choline chloride
:
glycerol – 1
:
1, similar to the findings reported in our previous studies, where different NADESs were employed for the extraction of phenolics from medicinal plants.46,47 In one of the studies,47 activity coefficients at infinite dilution were calculated by using a conductor-like screening model for realistic solvation (COSMO-RS) and transformed into the solvent-solute affinity parameter β∞, which is proportional to the solubility of the compound. The β∞ values indicate that the mixture choline chloride
:
glycerol
:
water in a molar ratio 1
:
1
:
3 (which is equivalent to 20% w/w of water) has greater affinity than methanol towards flavonoids, especially quercetin and luteolin. Besides that, in another study,46 COSMO-RS was employed for calculating σ-profiles, which showed that isoquercetin and quercitrin have σ-profiles similar to the system choline chloride
:
glycerol – 1
:
1. Similarities between the σ-profiles of solvents and solutes could explain the results of TFC, since they suggest that high solubility of some flavonoids is expected in eutectic solvents with the best TFC values.
Statistical evaluation of the results (paired t-test) of spectroscopic analysis revealed equal or higher extraction efficiency of NADES mixtures compared to conventional solvents in the case of all studied samples, demonstrating their possibility to be better alternatives for green isolation of bioactive compounds. Looking at the difference between each pair of the results (Table S5†), no statistically significant differences between acidified methanol and other NADESs, as well as acidified water and NADESs, were observed, indicating an equal extraction efficiency. Exception was observed in the case of black goji berries, where significantly higher values of spectroscopic parameters showed E15, E20 and E21 compared to methanol (P = 0.04, 0.04 and 0.05, respectively), making them more efficient extraction media in comparison with organic solvents.
The results of spectroscopic assays (TPC, TFC, TAC and RSA) were in agreement with non-targeted HPTLC profiling, pointing out the strong influence of NADES composition on extraction performances.
Additionally, these two approaches proved to be valuable in the preliminary assessment of extraction efficiency since they provided two different views: HPTLC combined with chemometrics could be used for the evaluation of similarities between NADES extracts, while the results of spectroscopic assays could give concrete values that showed which eutectic mixtures are the best for the extraction of a specific group of phenolics. Considering the results of these two approaches, five NADES extracts and one conventional extract, for each berry species, were chosen for further analysis and a more detailed study of the NADES extraction performance (HPTLC-antioxidant assays, quantification of phenolic compounds, antimicrobial activity and HPTLC-direct bioautography).
For further analysis of the chokeberry extracts, the following eutectic systems were chosen: E5 (choline chloride
:
malic acid in a molar ratio 1
:
1) with the maximum TAC value; E12 (choline chloride
:
glycerol in a molar ratio 1
:
2) with the maximum RSA value; E14 (choline chloride
:
urea in a molar ratio 1
:
1) with the maximum TFC value; E21 (L-proline
:
glycerol in a molar ratio 5
:
2) with the maximum TPC value; and E8 (choline chloride
:
lactic acid in a molar ratio 1
:
1), which belongs to a separate group of LA-based extracts according to the HPTLC profile (Fig. 2A). In the case of blueberry extracts, the selected eutectic systems were: E5 (choline chloride
:
malic acid in a molar ratio 1
:
1) with the maximum TAC and TFC values; E12 (choline chloride
:
glycerol in a molar ratio 1
:
2) with the maximum RSA value; E17 (urea
:
glycerol in a molar ratio 1
:
2) with the maximum TPC value; and E29 (L-lysine
:
lactic acid in a molar ratio 1
:
3) and E25 (L-proline
:
urea in a molar ratio 1
:
1), which belong to a separate group of amino acid-based extracts according to the HPTLC profile (Fig. 2B). For the black goji berry extracts, the selected eutectic systems were: E5 (choline chloride
:
malic acid in a molar ratio 1
:
1) with the maximum TAC and RSA values; E11 (choline chloride
:
glycerol in a molar ratio 1
:
1) with the maximum TPC and TFC values; E15 (choline chloride
:
urea in a molar ratio 1
:
2) with the maximum TAC value; and E21 (L-proline
:
glycerol in a molar ratio 5
:
2) and E34 (sodium-acetate
:
lactic acid in a molar ratio 1
:
3), which belong to a separate group of amino acid-based and LA-based NADES extracts according to the HPTLC profile, respectively (Fig. 2C). Acidified methanol (0.01% v/v hydrochloric acid) was chosen as the conventional system for further analysis.
The extraction of the blueberry sample was carried out in six replicates with the eutectic system choline chloride
:
malic acid, 1
:
1 n/n in order to assess the repeatability of extraction (Table S6 and Fig. S3†). The results of the spectroscopic assays showed low values of relative standard deviation: 7.3%, 13.3%, 25.8% and 6.3%, respectively, for TPC, TFC, TAC and RSA. The HPTLC analysis of the six replicates of NADES blueberry extracts also showed great repeatability of the extraction procedure; the relative standard deviation of the peak area was 18.8% for the anthocyanin band and 11.0% for the phenolic band.
3.4. HPTLC-antioxidant assays
Since the results of the spectrophotometric assays showed strong antioxidant activity of the NADES samples, selected extracts of each berry were further analysed by HPTLC-FC and HPTLC-DPPH assays. The combination of antioxidant assays and HTPLC provides a more detailed study of antioxidant activity, since individual compounds present in the samples are separated and visualised, in contrast to spectrophotometric assays, where the overall activity is studied.36 The HPTLC-FC assay resulted in the formation of blue zones against a yellow background (Fig. 4C), while in the HPTLC-DPPH assay active compounds appeared as yellow bands against a purple background (Fig. 4D). The black goji berry extracts showed the richest antioxidant profiles, followed by the chokeberry extracts, while the blueberry extracts had poor profiles, which was expected considering the spectrophotometric assays and chromatographic profiles. Among the two applied antioxidant assays, HPTLC-DPPH exhibited a higher number of active zones, indicating higher sensitivity and possibility of detection of a wider spectrum of compounds. However, the HPTLC-FC profiles revealed a band at hRF ∼ 97, present only in the NADES extracts based on lactic acid (chokeberry E8, blueberry E29 and black goji berry E34), additionally pointing out the predominant role of lactic acid in the extraction performances.
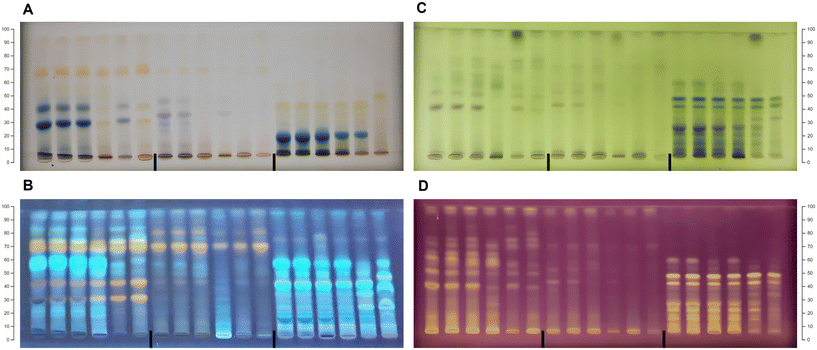 |
| Fig. 4 HPTLC profiles of the NADES and conventional extracts for (A) the phenolic profile under visible light and (B) the phenolic profile under ultraviolet light. (C) HPTLC-FC assay. (D) HPTLC-DPPH assay. Order of the samples on each plate: chokeberry: E5, E12, E14, E21, E8 and K1; blueberry: E5, E12, E17, E29, E25 and K1; and black goji berry: E5, E11, E15, E21, E34 and K1. | |
3.5. Quantification of phenolic compounds
In order to further evaluate the efficiency of NADESs to extract phenolic compounds, UHPLC-DAD-MS/MS analysis was performed on selected extracts of each berry species. In total, twenty-two phenolic compounds were determined in the NADES extracts of chokeberry (Tables S7 and S8† and Fig. 5), and the most abundant compounds were chlorogenic acid, cyanidin-3-O-glucoside and cyanidin-3-O-arabinoside. The eutectic systems E12, E14 and E21 extracted chlorogenic acid in an amount nearly two times higher than acidified methanol (1055, 1023, 1008 and 557 mg kg−1, respectively), while the anthocyanins cyanidin-3-O-glucoside and cyanidin-3-O-arabinoside were extracted in approximately nine- and ten-time higher amounts with E5 compared to the studied conventional solvents (C3G: 1390 and 157 mg kg−1 and C3A: 2375 and 235 mg kg−1, respectively). The great ability of the eutectic systems E12 and E14 to extract the two mentioned anthocyanins was in accordance with the high TAC values. Additionally, the system E5 was seen to be an excellent extraction medium for targeting kaempferol-3-O-glucoside and isorhamnetin, extracting these compounds approximately six and ten times, respectively, more than acidified methanol (K3G: 105 and 17.2 mg kg−1, ISR: 2.01 and 0.21 mg kg−1, respectively). Quercetin was extracted with E21 approximately twenty-two times more than with acidified methanol (92 and 4.1 mg kg−1, respectively).
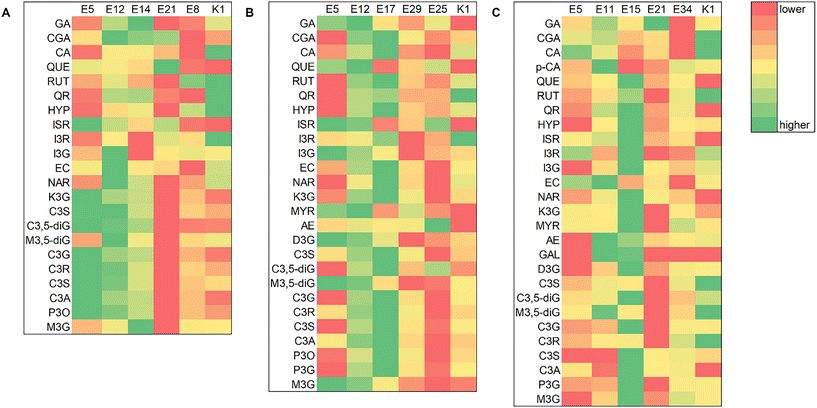 |
| Fig. 5 Heatmap of the quantified phenolic compounds in the selected extracts of (A) chokeberry; (B) blueberry and (C) black goji berry. Abbreviations: GA: gallic acid, CGA: chlorogenic acid, CA: caffeic acid, p-CA: p-coumaric acid, QUE: quercetin, RUT: rutin, QR: quercetin-3-O-rhamnoside quercitrin, HYP: hyperoside, ISR: isorhamnetin, I3R: isorhamnetin-3-O-rutinoside, I3G: isorhamnetin-3-O-glucoside, EC: epicatechin, NAR: naringin, K3G: kaempferol-3-O-glucoside, MYR: myricetin, AE: aesculetin, GAL: galangin, D3G: delphidin-3-O-glucoside, C3S: cyanidin-3-O-sophoroside, C3,5-diG: cyanidin-3,5-di-O-glucoside, M3,5-diG: malvidin-3,5-di-O-glucoside, C3G: cyanidin-3-O-glucoside, C3R: cyanidin-3-O-rutinoside, C3S: cyanidin-3-O-sambubioside, C3A: cyanidin-3-O-arabinoside, P3O: pelargonidin-3-O-glucoside, P3G: peonidin-3-O-glucoside, M3G: malvidin-3-O-glucoside. | |
Twenty-six phenolic compounds were determined in the NADESs of blueberry (Tables S7 and S8† and Fig. 5), and the most abundant compounds were malvidin-3-O-glucoside, delphidin-3-O-glucoside and chlorogenic acid. The eutectic mixture E17 extracted phenolics, such as chlorogenic acid, rutin, hyperoside, epicatechin, naringin and kaempferol-3-O-glucoside, in noticeable higher yields compared to methanol. The system E17 extracted chlorogenic acid three times more than acidified methanol (CGA: 191 and 64.6 mg kg−1, respectively), while naringin was extracted approximately two times more (39.7 and 17.1 mg kg−1, respectively). The eutectic system E12 extracted higher amounts of gallic acid, quercetin and myricetin compared to the studied conventional solvent, that too approximately four, seventeen and five times more, respectively (GA: 21.6 and 6.4 mg kg−1, QUE: 61.4 and 3.49 mg kg−1, and MYR: 29.6 and 5.61 mg kg−1, respectively). For the extraction of blueberry anthocyanins, the eutectic system E5 was seen to be the most effective, extracting delphidin-3-O-glucoside, malvidin-3,5-di-O-glucoside and malvidin-3-O-glucoside in higher yields compared to methanol (especially malvidin-3-O-glucoside, which was extracted with a twenty-four times higher yield, 917 and 38.2 mg kg−1, respectively). Additionally, the system E5 extracted isorhamnetin and isorhametin-3-O-glucoside better than methanol.
The most abundant phenolics in the black goji berry extracts were chlorogenic acid, rutin and delphidin-3-O-glucoside (Tables S7 and S8† and Fig. 5). The eutectic system E15 was an excellent extraction medium for the majority of the studied phenolics, especially for quercetin, hyperoside, naringin, and delphidin-3-O-glucoside, with four to seven times higher extractability power (QUE: 10.7 and 1.44, HYP: 24.6 and 4.99, NAR: 49.8 and 11.9, and D3G: 164.7 and 30.2 mg kg−1, respectively). The mixture E11 was seen to be a great solvent for targeting p-coumaric acid and epicatechin, while E21 extracted gallic acid in the highest yield compared to methanol and other eutectic solvents.
The extraction efficiency of each NADES in relation to acidified methanol was analysed by looking at the difference between each pair of results (presented by 28 quantified compounds) given by the two solvents, using a paired t-test (Table S9†). Significantly higher amounts of phenolic compounds were extracted by using E17 (urea
:
glycerol in a molar ratio 1
:
2) in the case of blueberry compared with methanol (P = 0.003), making it a more efficient extraction medium than organic solvents. No statistically significant differences between acidified methanol and other green extracts were observed, indicating that paired results are drawn from the same population. Such results of the statistical test implied that an equal amount of phenolic compounds was extracted by the organic solvent and NADES, i.e., suggesting an equal extraction efficiency. However, since NADESs are environmentally friendly media, this gives them an advantage over conventional solvents.
3.6. Antimicrobial activity
Literature data64–66 indicate that phenolics have potential antimicrobial properties. In that sense, the antimicrobial activity of selected NADES extracts, rich in phenolics, was additionally investigated. Screening was performed against six strains of Gram-positive and six strains of Gram-negative bacteria using the well diffusion method (see Table 2).
Table 2 Antimicrobial activity of the selected extracts evaluated by the well diffusion method
Tested stains |
Chokeberry |
Blueberry |
Black goji berry |
VAN |
E5 |
E12 |
E14 |
E21 |
E8 |
K1 |
E5 |
E12 |
E17 |
E29 |
E25 |
K1 |
E5 |
E11 |
E15 |
E21 |
E34 |
K1 |
VAN: vancomycin. The results are presented as zone of inhibition expressed in mm. “*” indicates bactericidal activity and “—” indicates no inhibition. |
B. subtilis ATCC 6633 |
15.5* |
18* |
16* |
12.5* |
— |
14.0 |
7.0* |
7.0 |
— |
— |
— |
— |
— |
— |
— |
— |
— |
— |
21.0* |
B. cereus ATCC 11778 |
13.5 |
14.5 |
15.5 |
14.0 |
12.0 |
19.0 |
— |
— |
— |
— |
— |
19.0 |
14.0 |
— |
— |
— |
— |
— |
15.0* |
S. aureus ATCC 6538 |
12.0 |
16.0 |
15.0 |
14.5 |
— |
12.0 |
— |
— |
18.0 |
— |
— |
— |
15.0 |
11.5 |
— |
— |
— |
— |
19.0* |
MRSA ATCC 33591 |
14.0 |
18.0 |
16.0 |
12.0 |
— |
12.0 |
— |
— |
12.0 |
— |
— |
— |
— |
— |
— |
— |
— |
— |
20.0* |
S. epidermidis ATCC 12228 |
14.0 |
17.5 |
15.0 |
14.0 |
— |
12.0 |
— |
— |
— |
— |
— |
— |
— |
— |
— |
— |
— |
— |
20.0* |
L. monocytogenes ATCC 13932 |
14.5 |
15.0 |
15.0 |
15.5 |
— |
14.5 |
— |
— |
— |
— |
— |
— |
— |
— |
— |
— |
— |
— |
22.0* |
E. coli ATCC 35218 |
14.5 |
16.5 |
14.0 |
15.0 |
11.0 |
11.5 |
12.0 |
15.5 |
— |
— |
— |
— |
— |
— |
— |
14.5 |
15.0 |
— |
— |
E. cloacae ATCC 13047 |
11.0 |
13.0 |
12.0 |
— |
— |
— |
— |
— |
— |
— |
— |
— |
— |
— |
— |
— |
— |
— |
— |
K. pneumoniae ATCC 29665 |
12.0 |
15.0 |
12.5 |
11.0 |
— |
11.5 |
— |
11.0 |
12.5 |
— |
— |
— |
— |
— |
— |
— |
— |
— |
— |
S. typhimurium ATCC 14028 |
12.5 |
13.0 |
13.0 |
14.0 |
— |
10.0 |
12.0 |
13.0 |
— |
— |
— |
— |
— |
— |
— |
— |
— |
— |
— |
S. enterica ATCC 13076 |
12.0 |
14.5 |
11.0 |
11.5 |
— |
11.0 |
— |
— |
— |
— |
— |
— |
— |
— |
— |
— |
— |
— |
— |
P. aeruginosa ATCC 10145 |
15.5 |
14.0 |
14.0 |
— |
12.0 |
15.5 |
14.0 |
13.5 |
17.5 |
— |
— |
— |
9.0 |
— |
— |
10.5 |
— |
— |
— |
The chokeberry extracts E5, E12 and E14 showed high antibacterial activity against all the tested strains, while the extract E21 was active against all tested Gram-positive strains and some negative strains. Chlorogenic acid was the most abundant phenolic compound in these extracts, followed by cyanidin-3-O-glucoside and cyanidin-3-O-arabinoside. Chlorogenic acid has been reported to exhibit inhibitor activity against B. subtilis, E. coli, S. Typhimurium67 and S. aureus.67,68 Studies also suggest that anthocyanins extracted from different plants69,70 have great antimicrobial activity against different strains, which could explain the lower antimicrobial activity of E21, compared to E5, E12 and E14, as this extract has a lower content of anthocyanins. It has also been reported that Gram-positive bacteria are generally more sensitive to anthocyanins than Gram-negative ones.70 The extract E8 only showed activity against B. cereus, E. coli, and P. aeruginosa. The conventional chokeberry extract (K1) had good antibacterial activity against all the strains tested, except against E. cloacae, which was lower compared to the green extracts E5, E12 and E14. The activity of these extracts was consistent with the phenolic content. Noticeably, the stronger antibacterial activity of the chokeberry extracts compared to the other berry extracts might be due to the presence of proanthocyanidins in the sample.71
The blueberry extracts showed different antibacterial activities. The extracts E5, E12 and E17 showed activity mainly against Gram-negative strains, while E29 and E26 were not active against any of the strains tested. This biological activity could be correlated with the phenolic composition, as the extracts E5, E12 and E17 were rich in phenolics. Only extract E17 showed activity against S. aureus and MRSA, which could be related to the higher naringin concentration, as several studies have reported its inhibitory effect against these strains.72,73 The extracts E5 and E12 showed similar activity against B. subtilis, E. coli, S. typhimurium and P. aeruginosa, and both have high levels of malvidin-3-O-glucoside, but to our knowledge, there are no reports on the antimicrobial activity of this anthocyanin. The conventional blueberry extract showed the potential to inhibit only Gram-positive bacteria such as B. cereus.
In the case of black goji berries, the extract E5 had the highest antimicrobial potential among the analysed extracts and showed activity against B. cereus, S. aureus and P. aeruginosa. Antibacterial activity against E. coli was marked by the extracts E21 and E34, but since these extracts had a very different phenolic profile, it was not possible to suggest a compound that could be responsible for their activity. The extracts E11 and E15 were the richest in phenolics, but they showed no antimicrobial activity, except E11 against S. aureus. The conventional black goji berry extract showed no activity against any of the strains tested.
3.7. HPTLC-direct bioautography
In order to further evaluate antibacterial activity and to identify compounds responsible for the inhibitory activity,74 direct bioautography assays were developed against the selected strains (B. subtilis, S.aureus, MRSA, E. coli and K. pneumoniae). Initially, chromatographic conditions for the development of a phenolic profile were applied; however, antibacterial zones were eluted with the solvent front, implying that the mobile phase was too polar. Therefore, the composition of the mobile phase was optimized (Table S3†) to achieve separation of compounds with antimicrobial activity, and the mobile phase toluene
:
ethyl acetate
:
formic acid (6/4/1, v/v/v) was selected. Since the selected chromatographic conditions led to separation of weakly and medium polar compounds, for chemical derivation (Fig. 6A), an anisaldehyde sulfuric acid (ANS) reagent was used.
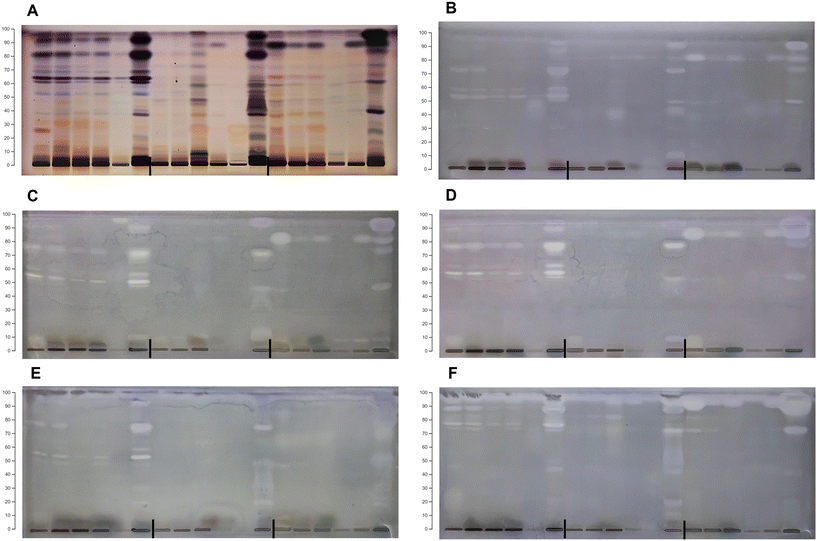 |
| Fig. 6 HPTLC-direct bioautography of the NADES and conventional extracts for (A) the metabolic profile; (B) Bacillus subtilis; (C) Staphylococcus aureus; (D) methicillin-resistant S. aureus (MRSA); (E) Escherichia coli; and (F) Klebsiella pneumoniae. Order of the samples on each plate: chokeberry: E5, E12, E14, E21, E8 and K1; blueberry: E5, E12, E17, E29, E25 and K1; and black goji berry: E5, E11, E15, E21, E34 and K1. | |
The bioautograms of Gram-positive strains showed a similar pattern (Fig. 6B–D), with the most active zones at hRF >50. The extracts of chokeberries and black goji berries confirmed the differences in antibacterial activity according to the extraction solvent, while the blueberry extracts showed weak activity. The methanolic extracts of each berry showed the greatest sensitivity towards the analysed bacteria. The bioautograms of the Gram-negative strains (Fig. 6E and F) revealed different antimicrobial profiles with the highest activity against K. pneumoniae.
These results suggest that compounds responsible for the antimicrobial activity are not phenolics, since the bioautograms revealed non-polar active zones. In addition, lipophilic compounds can exhibit higher antimicrobial potential against various microorganisms, since they can disrupt microbial cell membranes, interfere with metabolic processes, or hinder enzymatic activity.75 Active zones could correspond to monoterpenes based on chromatographic behaviour (non-polar properties) and according to the metabolic profile obtained with the ANS reagent, where they appeared as blue zones.44,76
Application of HPTLC-direct bioautography and HPTLC-antioxidant assays enabled parallel comparison of chromatographic data and biological activities, which enabled bioprofiling of the selected extracts. The obtained bioprofiles indicated a higher antioxidant activity of the analysed samples compared to its antimicrobial activity. Additionally, different compounds present in the samples were responsible for each biological activity – phenolics for antioxidant activity, and lipophilic components, most probably monoterpenes, for antimicrobial activity.
4. Conclusion
In this study, a range of eutectic solvents consisting of HBAs, such as choline chloride, L-proline, L-glycine and L-lysine and HBDs such as malic, citric, tartaric, lactic and succinic acids, glucose, glycerol and urea, were used for the extraction of phenolic compounds from three different berry species. Choline chloride-based NADESs in combination with malic acid, glycerol or urea were the most efficient. The results indicated the great potential of NADESs towards selective extraction of phenolics and showed that NADESs are designer solvents. Appropriate selection of components for NADES preparation can result in selective extraction of specific phenolic compounds; however, the optimal solvent needs to be adjusted for each matrix. The eutectic system choline chloride
:
malic acid showed selective extraction of anthocyanins, while choline chloride
:
glycerol or choline chloride
:
urea showed selectivity toward flavonoids and phenolic acids. The suggested methodology for the screening of NADES extraction performance, which included chromatographic profiling via HPTLC combined with chemometrics and spectrophotometric tests, allowed quick and effective assessment of optimal eutectic solvents for the isolation of different groups of phenolics. The chokeberry extract had the highest antioxidant and antimicrobial activity, followed by the black goji berry and blueberry extracts. Great antioxidant and antimicrobial activities of the studied extracts along with the natural origin of NADES components endow NADES extracts with a significant advantage for application as functional foods, food preservatives or supplements and give the opportunity for the creation of “green-labelled” products.
Author contributions
Mila Lazović: methodology, investigation, formal analysis, software, and writing – original draft; Marko Jović: methodology, investigation, and writing – original draft; Marija Petrović: investigation; Ivica Dimkić: writing – review and editing; Uroš Gašić: investigation; Dušanka Milojković-Opsenica: writing – review and editing; Petar Ristivojević: conceptualization and writing – review and editing; Jelena Trifković: methodology, conceptualization, supervision, and writing – review and editing.
Conflicts of interest
There are no conflicts to declare.
Acknowledgements
This work has been supported by the Ministry of Science, Technological Development and Innovation of Republic of Serbia Contract number: 451-03-47/2023-01/200168, 451-03-47/2023-01/200288.
References
- C. D. Bo’, D. Martini, M. Porrini, D. Klimis-Zacas and P. Riso, Berries and oxidative stress markers: an overview of human intervention studies, Food Funct., 2015, 6, 2890–2917 RSC.
- J. Higbee, P. Solverson, M. Zhu and F. Carbonero, The emerging role of dark berry polyphenols in human health and nutrition, Food Front., 2022, 3, 3–27 CrossRef CAS.
- O. Paredes-López, M. L. Cervantes-Ceja, M. Vigna-Pérez and T. Hernández-Pérez, Berries: Improving Human Health and Healthy Aging, and Promoting Quality Life—A Review, Plant Foods Hum. Nutr., 2010, 65, 299–308 CrossRef PubMed.
- S. Barkaoui, J. Madureira, N. Boudhrioua and S. C. Verde, Berries: effects on health, preservation methods, and uses in functional foods: a review, Eur. Food Res. Technol., 2023, 249, 1689–1715 CrossRef CAS.
- N. Pap, M. Fidelis, L. Azevedo, M. A. V. do Carmo, D. Wang, A. Mocan, E. P. R. Pereira, D. Xavier-Santos, A. S. Sant'Ana, B. Yang and D. Granato, Berry polyphenols and human health: evidence of antioxidant, anti-inflammatory, microbiota modulation, and cell-protecting effects, Curr. Opin. Food Sci., 2021, 42, 167–186 CrossRef CAS.
- L. Lavefve, L. R. Howard and F. Carbonero, Berry polyphenols metabolism and impact on human gut microbiota and health, Food Funct., 2020, 11, 45–65 RSC.
- B. B. Vidović, D. D. Milinčić, M. D. Marčetić, J. D. Djuriš, T. D. Ilić, A. Ž. Kostić and M. B. Pešić, Health Benefits and Applications of Goji Berries in Functional Food Products Development: A Review, Antioxidants, 2022, 11, 248 CrossRef PubMed.
- Y. Zhang, Y. Zhao, X. Liu, X. Chen, C. Ding, L. Dong, J. Zhang, S. Sun, Q. Ding, S. Khatoom, Z. Cheng, W. Liu, L. Shen and F. Xiao, Chokeberry (Aronia melanocarpa) as a new functional food relationship with health: an overview, J. Future Foods, 2021, 1, 168–178 CrossRef.
- N. Ćujić, K. Trifković, B. Bugarski, S. Ibrić, D. Pljevljakušić and K. Šavikin, Chokeberry (Aronia melanocarpa L.) extract loaded in alginate and alginate/inulin system, Ind. Crops Prod., 2016, 86, 120–131 CrossRef.
- S. Skrovankova, D. Sumczynski, J. Mlcek, T. Jurikova and J. Sochor, Bioactive Compounds and Antioxidant Activity in Different Types of Berries, Int. J. Mol. Sci., 2015, 16, 24673–24706 CrossRef CAS PubMed.
- C. Di Lorenzo, F. Colombo, S. Biella, C. Stockley and P. Restani, Polyphenols and Human Health: The Role of Bioavailability, Nutrients, 2021, 13, 273 CrossRef PubMed.
- B. Socas-Rodríguez, M. V. Torres-Cornejo, G. Álvarez-Rivera and J. A. Mendiola, Deep Eutectic Solvents for the Extraction of Bioactive Compounds from Natural Sources and Agricultural By-Products, Appl. Sci., 2021, 11, 4897 CrossRef.
- C. Cannavacciuolo, S. Pagliari, J. Frigerio, C. M. Giustra, M. Labra and L. Campone, Natural Deep Eutectic Solvents (NADESs) Combined with Sustainable Extraction Techniques: A Review of the Green Chemistry Approach in Food Analysis, Foods, 2023, 12, 56 CrossRef CAS PubMed.
- M. Ivanović, M. Islamčević Razboršek and M. Kolar, Innovative Extraction Techniques Using Deep Eutectic Solvents and Analytical Methods for the Isolation and Characterization of Natural Bioactive Compounds from Plant Material, Plants, 2020, 9, 1428 CrossRef PubMed.
- S. Kaoui, B. Chebli, S. Zaidouni, K. Basaid and Y. Mir, Deep eutectic solvents as sustainable extraction media for plants and food samples: A review, Sustainable Chem. Pharm., 2023, 31, 100937 CrossRef CAS.
- J. Cao, J. Cao, H. Wang, L. Chen, F. Cao and E. Su, Solubility improvement of phytochemicals using (natural) deep eutectic solvents and their bioactivity evaluation, J. Mol. Liq., 2020, 318, 113997 CrossRef CAS.
- N. P. E. Hikmawanti, D. Ramadon, I. Jantan and A. Mun'im, Natural Deep Eutectic Solvents (NADES): Phytochemical Extraction Performance Enhancer for Pharmaceutical and Nutraceutical Product Development, Plants, 2021, 10, 2091 CrossRef CAS PubMed.
- Y. Dai, R. Verpoorte and Y. H. Choi, Natural deep eutectic solvents providing enhanced stability of natural colorants from safflower (Carthamus tinctorius), Food Chem., 2014, 159, 116–121 CrossRef CAS PubMed.
- G. Grillo, V. Gunjević, K. Radošević, I. R. Redovniković and G. Cravotto, Deep Eutectic Solvents and Nonconventional Technologies for Blueberry-Peel Extraction: Kinetics, Anthocyanin Stability, and Antiproliferative Activity, Antioxidants, 2020, 9, 1069 CrossRef CAS PubMed.
- Y. Dai, E. Rozema, R. Verpoorte and Y. H. Choi, Application of natural deep eutectic solvents to the extraction of anthocyanins from Catharanthus roseus with high extractability and stability replacing conventional organic solvents, J. Chromatogr. A, 2016, 1434, 50–56 CrossRef CAS PubMed.
- D. T. da Silva, F. A. Smaniotto, I. F. Costa, J. Baranzelli, A. Muller, S. Somacal, C. S. Monteiro, M. Vizzotto, E. Rodrigues, M. T. Barcia and T. Emanuelli, Natural deep eutectic solvent (NADES): A strategy to improve the bioavailability of blueberry phenolic compounds in a ready-to-use extract, Food Chem., 2021, 364, 130370 CrossRef CAS PubMed.
- B. M. Popović, D. Uka, O. Alioui, R. Ždero Pavlović and Y. Benguerba, Experimental and COSMO-RS theoretical exploration of rutin formulations in natural deep eutectic solvents: Solubility, stability, antioxidant activity, and bioaccessibility, J. Mol. Liq., 2022, 359, 119266 CrossRef.
- B. Pavlić, Ž. Mrkonjić, N. Teslić, A. C. Kljakić, M. Pojić, A. Mandić, A. Stupar, F. Santos, A. R. C. Duarte and A. Mišan, Natural Deep Eutectic Solvent (NADES) Extraction Improves Polyphenol Yield and Antioxidant Activity of Wild Thyme (Thymus serpyllum L.) Extracts, Molecules, 2022, 27, 1508 CrossRef PubMed.
- B. Gordillo, G. T. Sigurdson, F. Lao, M. L. González-Miret, F. J. Heredia and M. M. Giusti, Assessment of the color modulation and stability of naturally copigmented anthocyanin-grape colorants with different levels of purification, Food Res. Int., 2018, 106, 791–799 CrossRef CAS PubMed.
- L. F. Ferreira, N. M. Minuzzi, R. F. Rodrigues, R. Pauletto, E. Rodrigues, T. Emanuelli and V. C. Bochi, Citric acid water-based solution for blueberry bagasse anthocyanins recovery: Optimization and comparisons with microwave-assisted extraction (MAE), LWT, 2020, 133, 110064 CrossRef CAS.
- V. Lebot, S. Michalet and L. Legendre, Identification and quantification of phenolic compounds responsible for the antioxidant activity of sweet potatoes with different flesh colours using high performance thin layer chromatography (HPTLC), J. Food Compos. Anal., 2016, 49, 94–101 CrossRef CAS.
- E. Łata, A. Fulczyk, T. Kowalska and M. Sajewicz, Thin-layer chromatographic method of screening the anthocyanes containing alimentary products and precautions taken at the method development step, J. Chromatogr. A, 2017, 1530, 211–218 CrossRef PubMed.
- S. Krüger, M. Mirgos and G. E. Morlock, Effect-directed analysis of fresh and dried elderberry (Sambucus nigra L.) via hyphenated planar chromatography, J. Chromatogr. A, 2015, 1426, 209–219 CrossRef PubMed.
- R. Yao, M. Heinrich, Y. Zou, E. Reich, X. Zhang, Y. Chen and C. S. Weckerle, Quality Variation of Goji (Fruits of Lycium spp.) in China: A Comparative Morphological and Metabolomic Analysis, Front. Pharmacol., 2018, 9, 151 CrossRef PubMed.
- L. Krenn, M. Steitz, C. Schlicht, H. Kurth and F. Gaedcke, Anthocyanin- and proanthocyanidin-rich extracts of berries in food supplements
– analysis with problems, Pharmazie, 2007, 62, 803–812 CAS.
- H. G. Cutler, J. M. Jacyno, R. S. Phillips, R. L. VonTersch, P. D. Cole and N. Montemurro, Cyclonerodiol from a Novel Source, Trichoderma koningii: Plant Growth Regulatory Activity, Agric. Biol. Chem., 1991, 55, 243–244 CAS.
-
V. L. Singleton, R. Orthofer and R. M. Lamuela-Raventós, in Methods in Enzymology, Academic Press, 1999, vol. 299, pp. 152–178 Search PubMed.
- J. Zhishen, T. Mengcheng and W. Jianming, The determination of flavonoid contents in mulberry and their scavenging effects on superoxide radicals, Food Chem., 1999, 64, 555–559 CrossRef CAS.
- J. Lee, R. W. Durst, R. E. Wrolstad and Collaborators, Determination of Total Monomeric Anthocyanin Pigment Content of Fruit Juices, Beverages, Natural Colorants, and Wines by the pH Differential Method: Collaborative Study, J. AOAC Int., 2005, 88, 1269–1278 CrossRef CAS PubMed.
- M. S. Blois, Antioxidant Determinations by the Use of a Stable Free Radical, Nature, 1958, 181, 1199–1200 CrossRef CAS.
- M. Jankov, P. Ristivojević, I. Cvijetić and D. Milojković-Opsenica, Assessing radical scavenging capacity of Sempervivum tectorum L. leaf extracts: An integrated high-performance thin-layer chromatography/in silico/chemometrics approach, J. Chromatogr. A, 2023, 1703, 464082 CrossRef CAS PubMed.
- M. B. Pešić, D. D. Milinčić, A. Ž. Kostić, N. S. Stanisavljević, G. N. Vukotić, M. O. Kojić, U. M. Gašić, M. B. Barać, S. P. Stanojević, D. A. Popović, N. R. Banjac and Ž. Lj. Tešić, In vitro digestion of meat- and cereal-based food matrix enriched with grape extracts: How are polyphenol composition, bioaccessibility and antioxidant activity affected?, Food Chem., 2019, 284, 28–44 CrossRef PubMed.
- I. Dimkić, P. Ristivojević, T. Janakiev, T. Berić, J. Trifković, D. Milojković-Opsenica and S. Stanković, Phenolic profiles and antimicrobial activity of various plant resins as potential botanical sources of Serbian propolis, Ind. Crops Prod., 2016, 94, 856–871 CrossRef.
- M. D. Jović, S. Agatonovic-Kustrin, P. M. Ristivojević, J. Đ. Trifković and D. W. Morton, Bioassay-Guided Assessment of Antioxidative, Anti-Inflammatory and Antimicrobial Activities of Extracts from Medicinal Plants via High-Performance Thin-Layer Chromatography, Molecules, 2023, 28, 7346 CrossRef PubMed.
- G. C. Cretu and G. E. Morlock, Analysis of anthocyanins in powdered berry extracts by planar chromatography linked with bioassay and mass spectrometry, Food Chem., 2014, 146, 104–112 CrossRef CAS PubMed.
- S. Krüger, O. Urmann and G. E. Morlock, Development of a planar chromatographic method for quantitation of anthocyanes in pomace, feed, juice and wine, J. Chromatogr. A, 2013, 1289, 105–118 CrossRef PubMed.
- S. Krüger and G. E. Morlock, Fingerprinting and characterization of anthocyanins in 94 colored wheat varieties and blue aleurone and purple pericarp wheat crosses, J. Chromatogr. A, 2018, 1538, 75–85 CrossRef PubMed.
- S. Krüger, L. Hüsken, R. Fornasari, I. Scainelli and G. E. Morlock, Effect-directed fingerprints of 77 botanical extracts via a generic high-performance thin-layer chromatography method combined with assays and mass spectrometry, J. Chromatogr. A, 2017, 1529, 93–106 CrossRef PubMed.
- A. da C. L. Gerlach, A. Gadea, R. M. B. da Silveira, P. Clerc and F. L. Dévéhat, The Use of Anisaldehyde Sulfuric Acid as an Alternative Spray Reagent in TLC Analysis Reveals Three Classes of Compounds in the Genus Usnea Adans. (Parmeliaceae, lichenized Ascomycota), Preprints, 2018, 2018020151 Search PubMed.
- P. Ristivojević, J. Trifković, I. Vovk and D. Milojković-Opsenica, Comparative study of different approaches for multivariate image analysis in HPTLC fingerprinting of natural products such as plant resin, Talanta, 2017, 162, 72–79 CrossRef PubMed.
- M. Lazović, I. Cvijetić, M. Jankov, D. Milojković-Opsenica, J. Trifković and P. Ristivojević, Efficiency of Natural Deep
Eutectic Solvents to Extract Phenolic Compounds from Agrimonia eupatoria: Experimental Study and In Silico Modelling, Plants, 2022, 11, 2346 CrossRef PubMed.
- M. Lazović, I. Cvijetić, M. Jankov, D. Milojković-Opsenica, J. Trifković and P. Ristivojević, COSMO-RS in prescreening of Natural Eutectic Solvents for phenolic extraction from Teucrium chamaedrys, J. Mol. Liq., 2023, 387, 122649 CrossRef.
- A. Ali Redha, Review on Extraction of Phenolic Compounds from Natural Sources Using Green Deep Eutectic Solvents, J. Agric. Food Chem., 2021, 69, 878–912 CrossRef CAS PubMed.
- L. Benvenutti, A. Sánchez-Camargo, A. A. F. Zielinski and S. R. S. Ferreira, NADES as potential solvents for anthocyanin and pectin extraction from Myrciaria cauliflora fruit by-product: In silico and experimental approaches for solvent selection, J. Mol. Liq., 2020, 315, 113761 CrossRef CAS.
- Y. Dai, J. van Spronsen, G.-J. Witkamp, R. Verpoorte and Y. H. Choi, Natural deep eutectic solvents as new potential media for green technology, Anal. Chim. Acta, 2013, 766, 61–68 CrossRef CAS PubMed.
- M. H. Zainal-Abidin, M. Hayyan, A. Hayyan and N. S. Jayakumar, New horizons in the extraction of bioactive compounds using deep eutectic solvents: A review, Anal. Chim. Acta, 2017, 979, 1–23 CrossRef CAS PubMed.
- T. Alishlah, A. Mun'im and M. Jufri, Optimization of Urea-Glycerin Based NADES-UAE for Oxyresveratrol Extraction from Morus alba Roots for Preparation of Skin Whitening Lotion, J. Young Pharm., 2019, 11, 155–160 CrossRef CAS.
- L. K. Savi, M. C. G. C. Dias, D. Carpine, N. Waszczynskyj, R. H. Ribani and C. W. I. Haminiuk, Natural deep eutectic solvents (NADES) based on citric acid and sucrose as a potential green technology: a comprehensive study of water inclusion and its effect on thermal, physical and rheological properties, Int. J. Food Sci. Technol., 2019, 54, 898–907 CrossRef CAS.
- H. Wang, S. Liu, Y. Zhao, J. Wang and Z. Yu, Insights into the Hydrogen Bond Interactions in Deep Eutectic Solvents Composed of Choline Chloride and Polyols, ACS Sustainable Chem. Eng., 2019, 7, 7760–7767 CrossRef CAS.
- M. S. Rahman, R. Roy, C. Montoya, M. A. Halim and D. E. Raynie, Acidic and basic amino acid-based novel deep eutectic solvents and their role in depolymerization of lignin, J. Mol. Liq., 2022, 362, 119751 CrossRef CAS.
- V. Macchioni, K. Carbone, A. Cataldo, R. Fraschini and S. Bellucci, Lactic acid-based deep natural eutectic solvents for the extraction of bioactive metabolites of Humulus lupulus L.: Supramolecular organization, phytochemical profiling and biological activity, Sep. Purif. Technol., 2021, 264, 118039 CrossRef CAS.
- C. Bakirtzi, K. Triantafyllidou and D. P. Makris, Novel lactic acid-based natural deep eutectic solvents: Efficiency in the ultrasound-assisted extraction of antioxidant polyphenols from common native Greek medicinal plants, J. Appl. Res. Med. Aromat. Plants, 2016, 3, 120–127 Search PubMed.
- K. Radošević, N. Ćurko, V. Gaurina Srček, M. Cvjetko Bubalo, M. Tomašević, K. Kovačević Ganić and I. Radojčić Redovniković, Natural deep eutectic solvents as beneficial extractants for enhancement of plant extracts bioactivity, LWT, 2016, 73, 45–51 CrossRef.
- B. M. Popovic, N. Micic, A. Potkonjak, B. Blagojevic, K. Pavlovic, D. Milanov and T. Juric, Novel extraction of polyphenols from sour cherry pomace using natural deep eutectic solvents – Ultrafast microwave-assisted NADES preparation and extraction, Food Chem., 2022, 366, 130562 CrossRef CAS PubMed.
- T. Bosiljkov, F. Dujmić, M. Cvjetko Bubalo, J. Hribar, R. Vidrih, M. Brnčić, E. Zlatic, I. Radojčić Redovniković and S. Jokić, Natural deep eutectic solvents and ultrasound-assisted extraction: Green approaches for extraction of wine lees anthocyanins, Food Bioprod. Process., 2017, 102, 195–203 CrossRef CAS.
- M. Cvjetko Bubalo, N. Ćurko, M. Tomašević, K. Kovačević Ganić and I. Radojčić Redovniković, Green extraction of grape skin phenolics by using deep eutectic solvents, Food Chem., 2016, 200, 159–166 CrossRef CAS PubMed.
- M. Panić, V. Gunjević, G. Cravotto and I. Radojčić Redovniković, Enabling technologies for the extraction of grape-pomace anthocyanins using natural deep eutectic solvents in up-to-half-litre batches extraction of grape-pomace anthocyanins using NADES, Food Chem., 2019, 300, 125185 CrossRef PubMed.
- Q. Zhang, K. D. O. Vigier, S. Royer and F. Jérôme, Deep eutectic solvents: syntheses, properties and applications, Chem. Soc. Rev., 2012, 41, 7108–7146 RSC.
- M. C. Lima, C. Paiva de Sousa, C. Fernandez-Prada, J. Harel, J. D. Dubreuil and E. L. de Souza, A review of the current evidence of fruit phenolic compounds as potential antimicrobials against pathogenic bacteria, Microb. Pathog., 2019, 130, 259–270 CrossRef CAS PubMed.
- A. Lobiuc, N.-E. Pavăl, I. I. Mangalagiu, R. Gheorghiţă, G.-C. Teliban, D. Amăriucăi-Mantu and V. Stoleru, Future Antimicrobials: Natural and Functionalized Phenolics, Molecules, 2023, 28, 1114 CrossRef CAS PubMed.
- N. Oulahal and P. Degraeve, Phenolic-Rich Plant Extracts With Antimicrobial Activity: An Alternative to Food Preservatives and Biocides?, Front. Microbiol., 2022, 12, 753518 CrossRef PubMed.
- Z. Lou, H. Wang, S. Zhu, C. Ma and Z. Wang, Antibacterial Activity and Mechanism of Action of Chlorogenic Acid, J. Food Sci., 2011, 76, M398–403 CrossRef CAS PubMed.
- G. Li, X. Wang, Y. Xu, B. Zhang and X. Xia, Antimicrobial effect and mode of action of chlorogenic acid on Staphylococcus aureus, Eur. Food Res. Technol., 2014, 238, 589–596 CrossRef CAS.
- Y. Ma, S. Ding, Y. Fei, G. Liu, H. Jang and J. Fang, Antimicrobial activity of anthocyanins and catechins against foodborne pathogens Escherichia coli and Salmonella, Food Control, 2019, 106, 106712 CrossRef CAS.
- A. Cisowska, D. Wojnicz and A. B. Hendrich, Anthocyanins as Antimicrobial Agents of Natural Plant Origin, Nat. Prod. Commun., 2011, 6, 149–156 CrossRef CAS PubMed.
- P. Denev, M. Číž, M. Kratchanova and D. Blazheva, Black chokeberry (Aronia melanocarpa) polyphenols reveal different antioxidant, antimicrobial and neutrophil-modulating activities, Food Chem., 2019, 284, 108–117 CrossRef CAS PubMed.
- S. S. Imam, S. J. Gilani, A. Zafar, M. N. Bin Jumah, R. Ali, M. M. Ahmed and S. Alshehri, Preparation and Optimization of Naringin Oral Nanocarrier: In Vitro Characterization and Antibacterial Activity, Coatings, 2022, 12, 1230 CrossRef CAS.
- N. Jaradat, N. Shawarb, F. Hussein, M. Al-Masri, I. Warad, A. Khasati, M. Shehadeh, M. Qneibi, A. M. A. Hussein and S. Makhamreh, Antibacterial and Antioxidant Screening of Semi-Synthetic Naringin Based Hydrazone and Oxime Derivatives, Jundishapur J. Microbiol., 2018, 11, e65496 Search PubMed.
-
I. Choma and W. Jesionek, Effects-Directed Biological Detection in Instrumental Thin-Layer Chromatography, ed. C. F. Poole, Elsevier, Boston, 2015, ch. 11, pp. 279–312 Search PubMed.
- L. J. McGaw, A. K. Jäger and J. van Staden, Antibacterial effects of fatty acids and related compounds from plants, S. Afr. J. Bot., 2002, 68, 417–423 CrossRef CAS.
- S. Agatonovic-Kustrin, S. Wong, A. V. Dolzhenko, V. Gegechkori, H. Ku, J. Tucci and D. W. Morton, Evaluation of bioactive compounds from Ficus carica L. leaf extracts via high-performance thin-layer chromatography combined with effect-directed analysis, J. Chromatogr. A, 2023, 1706, 464241 CrossRef CAS PubMed.
|
This journal is © The Royal Society of Chemistry 2024 |