DOI:
10.1039/D3FO05059A
(Paper)
Food Funct., 2024,
15, 4983-4999
A novel black poplar propolis extract with promising health-promoting properties: focus on its chemical composition, antioxidant, anti-inflammatory, and anti-genotoxic activities†
Received
16th November 2023
, Accepted 25th March 2024
First published on 26th March 2024
Abstract
Propolis is a resinous mixture produced by honeybees which has been used since ancient times for its useful properties. However, its chemical composition and bioactivity may vary, depending on the geographical area of origin and the type of tree bees use for collecting pollen. In this context, this research aimed to investigate the total phenolic content (using the Folin–Ciocalteu assay) and the total antioxidant capacity (using the FRAP, DPPH, and ABTS assays) of three black poplar (Populus nigra L.) propolis (BPP) solutions (S1, S2, and S3), as well as the chemical composition (HPLC-ESI-MSn) and biological activities (effect on cell viability, genotoxic/antigenotoxic properties, and anti-inflammatory activity, and effect on ROS production) of the one which showed the highest antioxidant activity (S1). The hydroalcoholic BPP solution S1 was a prototype of an innovative, research-type product by an Italian nutraceutical manufacturer. In contrast, hydroalcoholic BPP solutions S2 and S3 were conventional products purchased from local pharmacy stores. For the three extracts, 50 phenolic compounds, encompassing phenolic acids and flavonoids, were identified. In summary, the results showed an interesting chemical profile and the remarkable antioxidant, antigenotoxic, anti-inflammatory and ROS-modulating activities of the innovative BPP extract S1, paving the way for future research. In vivo investigations will be a possible line to take, which may help corroborate the hypothesis of the potential health benefits of this product, and even stimulate further ameliorations of the new prototype.
1. Introduction
Propolis, generally known as bee glue, is a resinous mixture produced by honeybees (Apis mellifera L.) for the construction and maintenance of their hives. Honeybees produce propolis by mixing the enzymes contained in their saliva and beeswax with exudates gathered mainly from leaf and flower buds, stems, and bark cracks of numerous species of trees.1 Propolis is mostly used by bees for sealing holes and cracks, smoothing the inner surfaces, retaining the internal temperature of the beehive, as well as for preventing weathering and invasion of predators.2,3 Propolis also possesses a marked antimicrobial activity, thus contributing to an aseptic internal environment. Moreover, propolis is used by bees to mummify (i.e., to prevent decay) the body of dead pests that have invaded the hives (e.g., shrews and mice), which are too big to be removed outside.4 Propolis is not a bee food and the name derives from the Greek ‘pro’ = ‘in defence’ or ‘in favour’ and ‘polis’ = ‘city’, thus meaning ‘defence of the hive’.5
Raw propolis is typically composed of resins and balms (including phenolic compounds) (50–60%), waxes and fatty acids (30–40%), essential oils (5–10%), pollen (∼5%), and other substances, including amino acids, micronutrients, and vitamins (thiamine, riboflavin, pyridoxine, vitamins C and E) (∼5%).6,7 In general, all propolis have similar organoleptic characteristics; however, the chemical composition of propolis—and consequently, colours and biological activities—depends on the race of bees, the geographical area of origin, the type of plant or tree used by bees for collecting pollen, nectar and/or exudates, and the sampling season.8,9
Propolis from the temperate zone all over the world (Europe, non-tropical regions of Asia, North America, and continental Australia) is classified as the poplar type propolis since it originates mainly from the bud exudates of poplar (Populus spp., most often Populus nigra L.), whose main biologically active components are flavonoids (flavones and flavanones), phenolic acids (e.g., cinnamic acid), and their esters.1,7,10,11 Propolis from Russia mainly originates from birch (Betula verrucosa Ehrh.) and contains flavones and flavonols (different from those present in poplar propolis).11 Propolis from the Mediterranean area (e.g., Greece and Greek islands, Sicily, Malta, Cyprus, Croatia, and Algeria) originates mainly from the resin of cypress (Cupressus sempervirens L.) and it is characterized by relatively high amounts of diterpenes.1,10,11 Several types of propolis originate in tropical zones, deriving from many different sources. Brown propolis is mainly produced in north-eastern Brazil from bushmints (Hyptis divaricata Pohl ex Benth).12,13 Green propolis—probably the most popular one among tropical propolis—owes its colour to the chlorophyll occurring in young tissues and nonexpanded leaves of Baccharis dracunculifolia DC (popularly known in Brazil as “alecrim-do-campo”) and collected by the bees;13 this type of propolis is rich in derivatives of phenylpropanoids (e.g., artepillin C) and diterpenes, although flavonoids arise in small amounts.11 Red propolis is characterized by the presence of numerous flavonoids (e.g., formononetin, liquiritigenin, pinobanksin, luteolin, rutin, quercetin, pinocembrin), which are found in the resinous exudates from the surface of coinvine (Dalbergia ecastaphyllum L.), a plant typically present in Brazil,12,14 but also characteristic of Cuba and Mexico.15 Pacific propolis originates from the tropical tree Macaranga tanarius L. (known by many common names which include parasol leaf tree, blush macaranga, David's heart and heart leaf), typically growing on Pacific Ocean tropical islands (e.g., Taiwan, Okinawa, Indonesia); Pacific propolis is typically rich in prenylated flavonoids, such as nymphaeol, 3′-geranyl-naringenin, propolin, and prokinawan.11,15,16
Propolis has been extensively used as an antiseptic and a potent herbal and dietary supplement since ancient times of human civilization, as well as raw material in numerous preparations, perfumes, and beverages.17,18 Ancient Egyptians extensively employed propolis to embalm the dead and preserve corpses from decomposition and heal wounds.19,20 The healing activities of propolis were also described by Roman and Greek physicians,21 as well as by other scientists, such as Aristotle, Pliny the Elder, Dioscorides, and Galen.22,23 Hippocrates (known as the father of modern medicine) also proposed the use of propolis to cure wounds and ulcers, both external and internal.21 More recently, during the Boer War in South Africa, propolis was used as vaseline-based ointment preparation for healing war wounds.24 Propolis was recognized for use in both human and veterinary medicine in the former Union of Soviet Socialist Republics (USSR); during World War II propolis – nicknamed as “Russian Penicillin” – was used in first aid to reduce wound infection and speed healing.25
In modern times, propolis is applied for various purposes in addition to human medicine, such as cosmetics, the food industry and aquaculture as well as in livestock farming.5
Overall, the reported biological activities of propolis include antibacterial,26,27 antiviral,28 antifungal,29 anti-parasitic,30 anti-oxidant,31,32 anti-inflammatory,33 and anti-cancer.34,35
The first scientific study involving propolis, which covered its chemical properties and composition, was published in 1908.36 However, given the high variability that actually characterises this product, reasoning through its general health potentialities without contemplating each peculiar case is definitely inappropriate. Each specific propolis should be separately considered as a distinct entity, which has to be investigated for its peculiar chemical features and biological activity. Analogously, novel propolis-based products prepared to further ameliorate health-related properties should also be accurately evaluated in terms of their chemical and functional characteristics.
In light of the above, this study aimed at defining the chemical composition and the antioxidant activity of three black poplar propolis (BPP) extracts, including a new, research-type product by an Italian nutraceutical manufacturer and two commercial preparations followed by the evaluation of some selected biological properties. Through the comparison with two largely diffused commercial products, the study intends to provide evidence that it is still possible to ameliorate the beneficial properties of BPP extracts, and that there is still space for research on this precious resinous mixture. Details on the interesting and copious results collected during the study are reported in the following sections.
2. Materials and methods
2.1. Chemicals, solvents, reagents, and media
2.1.1. Chemical analyses.
The following products were employed to carry out the UV-Vis and LC-MS/MS analyses. As far as the UV-Vis analyses are concerned, these refer to the protocols applied to appraise the total phenol content (TPC, through the Folin–Ciocalteu assay) and the total antioxidant capacity (TAC, through the FRAP, ABTS and DPPH assays) of the three extracts S1–S3. LC-MS/MS analyses were performed on the three BPP extracts for their qualitative characterization, as well. Folin–Ciocalteu (FC) reagent, 2,4,6-tris(2-pyridyl)-s-triazine (TPTZ), 6-hydroxy-2,5,7,8-tetramethyl-2-carboxylic acid (Trolox), 2,2′-azino-bis(3-ethylbenzothiazoline-6-sulfonic acid) (ABTS), hydrochloric acid (HCl), ferric chloride (FeCl3 × 6H2O), sodium acetate (NaOAc), sodium carbonate (Na2CO3), gallic acid (GA), potassium persulfate (K2S2O8), HPLC grade methanol (MeOH), HPLC grade ethanol (EtOH), HPLC grade acetonitrile (ACN) and formic acid (HCOOH) were purchased from Merck Life Science (Merck KGaA, Darmstadt, Germany). Water (H2O) was purified using a Milli-Q Plus185 system from Millipore (Milford, MA, USA).
2.1.2. Biological tests.
Dimethyl sulfoxide (DMSO), ethidium bromide, hydrocortisone hemisuccinate, insulin, lipopolysaccharide (LPS), low- and normal melting-point agarose (LMPA and NMPA, respectively), 4-nitroquinoline N-oxide (4NQO), tris(hydroxymethyl)-aminomethane (Tris base), and Triton X-100 were purchased from Merck Life Science (Merck KGaA, Darmstadt, Germany). Ethanol, ethylenediaminetetracetic acid disodium (Na2EDTA) and tetrasodium (Na4EDTA) salt, sodium chloride (NaCl), and sodium hydroxide (NaOH) were purchased from Carlo Erba Reagents Srl (Milan, Italy). Acridine orange (AO), 6,4′-diamidino-2-phenylindole (DAPI), and Via1-Cassette™ were purchased from ChemoMetec A/S (Allerød, Denmark). Eagle's Minimum Essential Medium (MEM) and Dulbecco's phosphate-buffered saline, pH 7.4 (PBS), were purchased from Invitrogen Srl (Milan, Italy). Ammonium–Chloride–Potassium (ACK) lysing buffer, Ficoll-Paque™ Plus, Gibco™ William's E and RPMI-1640 culture media, and GlutaMAX™ supplement were obtained from Thermo Fisher Scientific (Waltham, MA, USA). Antibiotics (penicillin and streptomycin), foetal bovine serum (FBS), MEM non-essential amino acids (NEAA), sodium pyruvate, and trypsin were purchased from Euroclone SpA (Milan, Italy). ViaLight™ Plus Kit was purchased from Lonza, Rockland, ME, USA. TNFα, IL1-β and IL-10 ELISA assay kits were purchased from U-CyTech BV, Utrecht, The Netherlands. COX Activity Assay Kit was obtained from BioVision Incorporated, Milpitas, CA, USA. Conventional microscope slides and coverslips were supplied by Knittel-Glaser GmbH (Braunschweig, Germany).
2.1.3. Black poplar propolis solutions.
The hydroalcoholic black poplar (Populus nigra L.) propolis (BPP) solution S1 (sample S1) was a prototype of a new (research) product by an Italian manufacturer of nutraceutical, herbal, and other health and medical products. Hydroalcoholic BPP solutions S2 and S3 were largely diffused preparations purchased in local pharmacy stores and analysed to identify possible remarkable differences with the innovative prototype S1. Being aware of the limited number of selected comparative products (S2 and S3), we however deem that these are sufficient to prove the intended concept that it is still possible to ameliorate the characteristics of the natural extract. BPP samples S1, S2, and S3 have been tested for total phenolic content, total antioxidant capacity, radical scavenging capacity and characterized by HPLC-ESI-MSn analyses. The extract which displayed the highest antioxidant activity (i.e., BPP S1) was further characterized for its biological features by cytotoxicity testing, genotoxicity/antigenotoxicity testing, anti-inflammatory activity, and analysis of ROS production.
2.2. Preparation of black poplar propolis (BPP) dry extracts
2.2.1. Chemical analyses.
One mL of each BPP hydroalcoholic solution (samples S1, S2, and S3) was evaporated to dryness under a gentle stream of nitrogen. A specific treatment was applied to S1 before the drying step. Indeed, being a prototype and still unrefined product, S1 was characterized by the presence of a solid suspension (large-sized debris), which was precipitated via centrifugation. Accordingly, 10 mL of S1 was placed in a 15 mL Falcon tube and centrifuged for 10 min at 2000 rpm, setting the temperature at 20 °C. One mL of the supernatant was then dried as described above.
The solid residues obtained after drying were weighed and re-dissolved in MeOH to obtain a 0.45 mg mL−1 concentration. For each sample, five solid extracts were independently analysed in terms of their total phenolic content and TAC values, according to the procedures described in sections 2.3–2.6.
2.2.2. Biological tests.
Because of the high concentration of ethanol present in the hydroalcoholic BPP solutions, solvent removal procedures and resuspension into solvents compatible with in vitro tests were necessary. After removing the ethanol by using a vacuum rotary evaporator (15 min, 30 °C), the remaining residue was frozen and then freeze-dried for 15 h, until the liquid fraction was completely removed. Once the dry BPP extract was obtained, the choice of solvent or vehicle was based on obtaining the maximum solubility of the test material without interacting with the test system. Following the protocol for solubility determination proposed by the US National Toxicology Program Interagency Center for the Evaluation of Alternative Toxicological Methods (NICEATM),37 the BPP extract was first resuspended in complete MEM and a sequence of mixing methods (i.e., vortexing, sonicating, and heating at 37 °C) was followed. The BPP extract was always completely insoluble, with the rapid formation of an evident precipitate. The BPP extract was then resuspended in a solvent specifically proposed for propolis and consisted of 98
:
2 (v/v) DMSO
:
EtOH.38 Solubility was considered to be achieved when, upon visual observation, the solution was clear and showed no signs of cloudiness or precipitation. The highest concentration soluble in DMSO
:
EtOH 98
:
2 (v/v) was 50 mg mL−1. The stock solution was then further diluted in complete MEM until the desired highest concentrations, namely 500 μg mL−1 (see section 2.9 – cytotoxicity testing). Solvent concentration in cell cultures was always equal to or less than 1% (v/v).
2.3. Determination of total phenolic content (TPC) and total antioxidant capacity (TAC)
The TPC of each BPP extract was determined with the Folin–Ciocalteu (FC) method as described in refs. 39 and 40 with only a few modifications. The TAC of each BPP extract was determined with the FRAP, DPPH and ABTS methods as described elsewhere,39,40 with only a few modifications. More details are reported in the ESI.†
2.4. HPLC-ESI-MSn for the identification of propolis secondary metabolites
HPLC-ESI-MSn analyses were carried out using an Agilent Technologies modular 1200 system, equipped with a vacuum degasser, a binary pump, an autosampler, a thermostatted column compartment and an ion trap mass analyzer with an ESI ion source. An Ascentis Express C18 column (150 mm × 3.0 mm I.D., 2.7 μm; Supelco, Bellefonte, PA, USA) was used for the separation of phenolic compounds in BPP extracts.41 The mobile phase was composed of 0.1% (v/v) HCOOH in H2O (A) and ACN (B). The gradient elution was set as follows: 0–3 min 20% B, 3–10 min from 20 to 30% B, 10–40 min from 30 to 40% B, 40–50 min from 40 to 60% B, 50–60 min from 60 to 80% B, and 60–65 min from 80 to 50% B. The gradient was back to 20% B in the post-running time for column reconditioning. The post-running time was 10 min. The flow rate was set at 0.6 mL min−1, while the column temperature was set to 30 °C. The sample injection volume was 2 μL.
The flow rate was split 3
:
1 before the ESI source. The experimental parameters were set as follows: the capillary voltage was 3.5 kV, the nebulizer (N2) pressure was 32 psi, the drying gas temperature was 350 °C, the drying gas flow was 11 L min−1 and the skimmer voltage was 40 V. Data were acquired by using Agilent 6300 Series Ion Trap LC/MS system software (version 6.2). The mass spectrometer was operated in the full-scan negative ion mode in the m/z range 100–1000. MS2 spectra were automatically performed with helium as the collision gas by using the SmartFrag function.
2.5. Cell culture isolation or maintenance
2.5.1. Human peripheral blood mononuclear cells (PBMCs) and polymorphonuclear (PMN) cells.
PBMC were isolated from heparinized venous blood, obtained from a buffy coat, kindly provided by the Blood Bank of the Ospedale Santa Maria della Misericordia of Perugia. All donors signed the consensus form (MO-SIT_06), approved by the CEAS (Comitato Etico Aziende Sanitarie) Ethics Committee, authorizing their sample for research use. Heparinized venous blood (10 mL) was diluted with RPMI 1640 (25 mL). The interface layer of the PBMCs was obtained by gradient centrifugation over Ficoll-Paque Plus (density = 1.077; 35 mL of diluted blood layered over 10 mL of Ficoll-Paque solution). The pellet containing PMN and erythrocytes was treated with 40 mL of hypotonic saline (ACK lysing buffer) to lyse the erythrocytes. PBMC and PMN were collected and counted and the concentration was adjusted for use.
2.5.2. HepG2 and HepaRG liver cells.
Cytotoxicity/genotoxicity tests comprised in the study design were performed in two preclinical hepatic models of human origin,42,43 namely HepG2 hepatoblastoma44,45 and HepaRG™ immortalized46 liver cells. The HepG2 cells were obtained from Istituto Zooprofilattico Sperimentale della Lombardia e dell'Emilia Romagna “Bruno Ubertini” (Brescia, Italy). The cells were maintained in MEM supplemented with 10% (v/v) FBS, 1% NEAA, 1 mM sodium pyruvate, 100 U mL−1 penicillin and 0.1 mg mL−1 streptomycin, in an incubator at 37 °C and 5% CO2. The HepaRG cells were purchased from Thermo Fisher Scientific (Waltham, MA, USA). The cells were maintained in William's E medium supplemented with 10% FBS, 1% glutamax, 5 μg mL−1 human insulin and 50 μM hydrocortisone hemisuccinate, in an incubator at 37 °C and 5% CO2.
Experiments were carried out using HepG2 at passages 24–28 and undifferentiated HepaRG at passages 8–14.
2.6. Cytotoxicity testing
For cytotoxicity/genotoxicity testing, the top test concentration used was 500 μg mL−1, according to the European Food Safety Authority (EFSA) guidelines on genotoxicity testing strategies for food and feed safety assessment.47 Cytotoxicity testing was then performed by evaluating five scalar concentrations (i.e., 25, 50, 100, 250, and 500 μg mL−1) of the tested BPP extract.
2.6.1. ATP assay.
The number of living PBMCs after treatment with BPP extract S1 was measured using the luminescent cell viability assay (ViaLight Plus Kit), according to the manufacturer's instructions. This assay determines the viability of metabolically active cells based on the quantification of ATP concentration. The kit includes a detergent to break the cell membrane causing ATP release. The dosage is based on the conversion of luciferin to oxyluciferin from recombinant luciferase in the presence of ATP. The observed luminescence is proportional to the amount of ATP in the cells. The experiments were performed on 96-well white plates. Additional controls were included in the test to exclude auto-luminescence. For the ATP assay, 1 × 105 PBMC cells per well were plated and treated with different concentrations (0, 25, 50, 100, 250, 500 μg mL−1) of BPP extract S1 and incubated at 37 °C for 24 h (the same incubation time used for cytokine determination). Then, the cell lysis reagent was added to each well to extract ATP from cells. Next, the ATP Monitoring Reagent Plus was added, and after 2 minutes, the luminescence was read using a microplate luminometer (Infinite® M200pro, Tecan, Männedorf, Switzerland). Results were expressed as 50% cytotoxic concentration (CC50), which is the concentration required to reduce the cell viability by 50% compared with the untreated controls.
2.6.2. Acridine orange/DAPI double staining.
After exposure of HepG2 and HepaRG cells to scalar concentrations of the BPP extract S1, the number of total and viable cells was estimated by staining cell populations with AO and DAPI fluorophores. For the test, the cells (5 × 105 per well) were dispensed within six-well culture plates (Becton Dickinson Italia SpA, Milan, Italy) in 5 mL volumes and exposed for 4 or 24 h (37° C, 5% CO2) to the BPP extracts. After cell treatment, aliquots of cell suspensions were loaded into Via1-Cassette that were then read in a NucleoCounter® NC-3000™ (Chemometec, Allerød, Denmark), a fluorescence-based image cytometer.48,49
2.7. Genotoxicity/antigenotoxicity testing
To avoid conditions that would lead to false-positive results arising from DNA damage associated with cytotoxicity,50 non-cytotoxic concentrations of the BPP extract S1 (i.e., 3.125, 6.25, 12.5, 25, 50, and 100 μg mL−1) were processed in the comet assay. Treatments were performed by following the protocol proposed by Munari et al.51 (treatment scheme depicted in ESI Fig. S1†).
2.7.1. Co-exposure treatment.
Briefly, 24 h before the treatment with the BPP extract S1, HepG2 and HepaRG cells were seeded (approximately 2.5 × 105 cells per well) in 12-well plates. The culture medium was replaced by fresh, complete MEM or William's E medium and the cells were then incubated further for 4 h according to the following scheme:
(i) Challenge cultures: serial dilutions of BPP extract S1 (i.e., 3.125, 6.25, 12.5, 25, 50, and 100 μg mL−1) plus 1 μM 4NQO;
(ii) BPP cultures: serial dilutions of BPP extract S1 (see above);
(iii) Known mutagen cultures (positive control): 1 μM 4NQO;
(iv) Solvent control: 1% DMSO
:
EtOH 98
:
2, v/v;
(v) Negative: untreated cells.
At the end of treatments, the cells were processed for the comet assay as described elsewhere.52
2.7.2. Pre-exposure treatment.
After 24 h culture, for both control and challenge cultures, the culture medium was replaced by fresh complete MEM or William's E medium containing the test concentrations of the BPP extract S1 (i.e., 3.125, 6.25, 12.5, 25, 50, and 100 μg mL−1). The cells were then incubated further for 4 h. After that, the culture medium containing the BPP extract was removed, and the cells were washed in PBS and, after adding fresh, complete MEM or William's E medium, incubated further for 4 h according to the following scheme:
(i) Challenge cultures: 1 μM 4NQO.
(ii) BPP cultures: fresh, complete MEM/William's E medium.
Appropriate positive (i.e., 1 μM 4NQO), solvent (i.e., 1% DMSO
:
EtOH 98
:
2, v/v), and negative (i.e., untreated cells) controls were included in each experimental set. At the end of treatments, the cells were washed and harvested as described elsewhere.52
2.7.3. Post-exposure treatment.
After 24 h culture, the culture medium was replaced by fresh complete MEM/William's E medium containing 1 μM 4NQO and the cells were incubated further for 4 h. The culture medium was then removed; the cells were washed in PBS and, after adding fresh, complete MEM or William's E medium, incubated further for 4 h according to the following scheme:
(i) Challenge cultures: fresh, complete MEM/William's medium containing serial dilutions of the BPP extract S1 (see above);
(ii) Known mutagen cultures (positive control): fresh, complete MEM/William's medium.
Appropriate extract (i.e., serial dilutions of BPP extract S1), positive (i.e., 1 μM 4NQO), solvent (i.e., 1% DMSO
:
EtOH 98
:
2, v/v), and negative (i.e., untreated cells) controls were included in each experimental set. At the end of treatments, the cells were washed and harvested as described elsewhere.52
2.7.4. Alkaline single-cell microgel electrophoresis (comet) assay.
At the end of treatments, the comet assay was conducted under alkaline conditions (alkaline unwinding/alkaline electrophoresis, pH > 13) following the original three-layer procedure53 as described in detail elsewhere.42,43 Briefly, the cells were washed twice with ice-cold PBS (pH 7.4) and detached with trypsin. The cells were then collected by centrifugation, and cell pellets were gently resuspended in 0.7% (w/v) LMPA and layered onto conventional microscope slides precoated with 1% (w/v) NMPA. After brief agarose solidification, the embedded cells were protected with a top layer of 0.7% (w/v) LMPA. After brief agarose solidification, the slides were immersed in cold, freshly prepared lysing solution (2.5 M NaCl, 100 mM Na4EDTA, 10 mM Tris-HCl; pH 10 with NaOH; 1% (v/v) Triton X-100 added just before use) for at least 60 min at 4 °C. The slides were then placed in a horizontal electrophoresis box (HU20, Scie-Plas, Cambridge, UK) filled with a freshly prepared solution (10 mM Na4EDTA, 300 mM NaOH); pH > 13. Before electrophoresis, the slides were left in the alkaline buffer for 20 min to allow DNA unwinding and expression of alkali-labile damage. Electrophoresis runs were then performed in an ice bath for 20 min by applying an electric field of 34 V (1 V cm−1) and adjusting the current to 300 mA (Power Supply PS250, Hybaid, Chesterfield, MO, USA). After the electrophoresis, the microgels were neutralized with 0.4 M Tris-HCl buffer (pH 7.5), fixed for 10 min in EtOH, allowed to air-dry and stored in slide boxes at room temperature until analysis. All the steps of the comet assay were conducted in yellow light to prevent the occurrence of additional DNA damage.
Immediately before scoring, the air-dried slides were stained with 50 mL of 20 mg mL−1 ethidium bromide and covered with a coverslip. The comets in each microgel were analyzed (blind), at ×500 magnification with an epi-fluorescence microscope (BX41, Olympus, Tokyo, Japan), equipped with a high sensitivity black and white charge-coupled device (CCD) camera (PE2020, Pulnix, UK), under a 100 W high-pressure mercury lamp (HSH-1030-L, Ushio, Japan), using appropriate optical filters (excitation filter 510–550 nm and emission filter 590 nm). Images were elaborated by using Comet Assay III software (Perceptive Instruments, UK). One hundred randomly selected comets (50 cells per replicate slide) were evaluated for each experimental point. The percentage of DNA in the comet tail (i.e., tail intensity %) was used as a measure of the extent of DNA damage.54
2.8. Anti-inflammatory activity
2.8.1. Cyclooxygenase activity.
The anti-inflammatory activity of BPP S1 was first evaluated through a COX assay in HepG2 cells.
Although COX-1 is constitutively expressed in many tissues including the liver, conversely, COX-2 is usually undetectable (or a very low expression can be observed at least) in tissues under normal conditions. Particularly, high COX-2 expression has been identified in hepatocarcinoma tumours, as well as in HepG2 and other hepatoma cell lines.55–57
As the inhibition of COX—in particular COX-2—and its downstream pathways have a potential role in cancer therapy we explored, in the HepG2 cell line, the COX-inhibiting activity of BPP S1.58–61
Briefly, HepG2 cells were seeded in 25 cm2 flasks (3 × 106 cells per flask in 5 mL of complete medium) and maintained in culture for 24 h to form a semi-confluent monolayer. After that, cells were treated with the three highest non-cytotoxic concentrations of the BPP extract S1 (i.e., 25, 50, and 100 μg mL−1). The COX inhibiting activity was assessed basically as previously described using the COX Activity Assay Kit.62
2.8.2. Cytokine determination.
To evaluate cytokine production, PBMCs (2 × 106 cells per mL) were incubated in the presence of 1 μg mL−1 LPS and/or BPP extract (50 μg mL−1). In the pre-treatment protocol, the cells were first incubated for 4 h at 37 °C and 5% CO2 with the BPP extract S1 and then stimulated with LPS for a further 24 h. In the post-treatment protocol, the cells were first challenged with LPS and then treated with the BPP extract. In the co-treatment protocol, cells were treated with LPS and BPP extract for 24 h. The concentration of secreted cytokines TNFα, IL1-β and IL-10 was determined in cell supernatants by the ELISA assay (U-CyTech kit) according to the manufacturer's instructions.
2.9. Analysis of ROS production
ROS production was evaluated by a chemiluminescence assay according to ref. 63 with some modifications. Chemiluminescence measurements were performed by stimulating 100 μL of PMN suspension (1.25 × 106 cells) with 50 μL of BPP extract S1 (50 and 5 μg mL−1, respectively) in the presence of 50 μL of Luminol (0.28 mM). The mixture was incubated for 3 min at 37 °C. The cells were then stimulated with 50 μL of 10–7 phorbol-12-myristate-13-acetate (PMA) (500 ng mL−1). The chemiluminescence produced by the cells was monitored for 60 minutes in a luminometer (Infinite M200pro, Tecan). The light output was recorded as RLU (relative photons light units). Each measure was performed in triplicate in four individual experiments for each concentration.
2.10. Statistical analysis
Biological assays were carried out at least in triplicate (except for the AO/DAPI test in HepaRG cells). After testing the normal distribution of data with the Kolmogorov–Smirnov test, the results were expressed as the mean ± standard error of the mean (SEM) and differences were investigated by one-way analysis of variance (ANOVA) followed by Dunnett's post hoc analysis for pairwise comparisons; the level of significance was set at p < 0.05. For antigenotoxicity testing, the remaining 4NQO-induced genotoxic activity (RGA%) and genotoxic inhibition rate (GIR%) were calculated as specified in detail elsewhere.52
The SPSS statistical package (SPSS Inc., Chicago, IL, USA) was used for statistical analyses.
3. Results
3.1. Total phenolic content (TPC)/total antioxidant capacity (TAC)
TPC was evaluated with the FC method using GA as the reference standard for the construction of the calibration curve. TPC values are here reported as mg equivalents of GA (referred to as GAE) per g of dried methanolic extract (Table 1).
Table 1 Summary of the measured Folin–Ciocalteu (FC), FRAP, DPPH and ABTS values of the three phenolic extracts (BPP S1–S3) from the three investigated BPP hydro-alcoholic solutions
Assay |
BPP extracta |
S1b |
S2 |
S3 |
Data are expressed as mean ± SD. Analyses were performed in triplicate for each extract.
Only the most effective sample (i.e., BPP extract S1) was further characterized for its biological features.
|
FC (mg GAE per g d.e.) |
282.4 ± 4.6 |
122.2 ± 5.4 |
127.8 ± 8.2 |
FRAP (μmol TE per g d.e.) |
887 ± 44 |
744 ± 25 |
800 ± 15 |
DPPH (μmol TE per g d.e.) |
1400 ± 72 |
1176 ± 19 |
1205 ± 52 |
ABTS (μmol TE per g d.e.) |
1946 ± 146 |
1482 ± 101 |
1558 ± 61 |
The TPC of BPP extract S1 was found to be among the highest measured so far for alcoholic and hydro-alcoholic extracts from black poplar-type propolis.64–66 Moreover, the BPP extract S1 exhibited a TPC more than twice higher than that measured in our laboratory in two similar extracts available on the Italian market (BPP extracts S2 and S3).
TAC was determined through the FRAP, DPPH and ABTS methods,39,40 using Trolox as the reference standard for the construction of the calibration curves. TAC values are here reported as mg equivalents of Trolox (referred to as TE) per g of the methanolic extract (Table 1).
In analogy to TPC values, the results of FRAP, DPPH, and ABTS assays on BPP extract S1 were among the highest found in the literature for the analysis of BPP extracts,64,65 Moreover, these evaluations revealed that TACs of the extracts from BPP extracts S2 and S3 were sensitively lower than that of BPP extract S1 (Table 1), and in line with literature data.
3.2. Identification of propolis secondary metabolites (HPLC-ESI-MSn)
The BPP hydro-alcoholic extracts S1–S3 were analyzed by means of HPLC-ESI-MSn.41 The identification of the compounds was achieved by comparing MS and MS2 data of each chromatographic peak with those described in the literature for the same analytes.67–73 All phenolic compounds occurring in the propolis sample showed a better fragmentation when the mass spectrometer was operated in the negative ion mode, which allowed us to also keep a good intensity of the pseudo-molecular ions [M − H]−. For this reason, the negative ion mode only was selected to be applied for compound characterization in this work.
The base peak chromatogram obtained using HPLC-ESI-MS2 analysis is shown in Fig. 1, which highlights a very complex chemical composition. Compound identity was initially hypothesized based on the molecular weight. Then, MS2 spectrum was recorded to study the fragmentation pathway of the compounds belonging to the different classes of phenolics.
 |
| Fig. 1 Base peak HPLC-MS chromatogram of propolis samples S1 (blue line), S2 (red line) and S3 (green line). For peak identification, see Table 2. | |
MS and MS2 data of the compounds identified in the propolis sample are shown in Table 2. Overall, the HPLC-ESI-MS2 analysis allowed for the identification of 50 phenolic compounds, encompassing phenolic acids and flavonoids. As readily evident from Fig. 1, the same qualitative composition was found in all three investigated extracts. These data are in agreement with previous work.41,67–73
Table 2 HPLC-ESI-MSn data obtained for the analysis of propolis constituents in the negative ion mode. Fragmentation data were compared with those available in the literature
Peak # |
Compound name |
t
R (min) |
[M − H]− |
MS2 product ions |
t
R = retention time (min). |
1 |
Pinobanksin-methyl-ether |
10.6 |
285 |
267 (100), 253 (30), 242 (18), 239 (74), 224 (35) |
2 |
Pinobanksin-5-methyl-ether |
11.9 |
285 |
267 (100), 253 (18), 239 (16), 223 (19) |
3 |
Quercetin-3-methyl ether |
12.9 |
315 |
301 (18), 300 (100) |
4 |
Pinobanksin |
14.3 |
271 |
253 (100), 215 (22), 209 (26), 151 (31), 107 (26) |
5 |
Apigenin |
14.6 |
269 |
225 (68), 149 (68) |
6 |
Kaempferol |
15.3 |
285 |
257 (100), 169 (64) |
7 |
Isorhamnetin |
16.0 |
315 |
301 (9), 300 (100) |
8 |
Luteolin-methyl-ether |
16.7 |
299 |
284 (100) |
9 |
Quercetin-dimethyl-ether |
17.6 |
329 |
314 (100), 299 (5) |
10 |
Galangin-5-methyl-ether |
19.2 |
283 |
268 (60), 239 (100), 240 (59), 211 (57) |
11 |
Pinobanksin-5-methyl-ether-3-O-acetate |
19.4 |
327 |
285 (100), 267 (13), 239 (9) |
12 |
Quercetin-7-methyl ether |
21.1 |
315 |
301 (3), 297 (12), 193 (54), 165 (100), 121 (18) |
13 |
Quercetin-dimethyl-ether |
23.5 |
329 |
314 (100), 299 (6) |
14 |
Kaempferol dimethyl ether derivative |
25.3 |
343 |
328 (100), 313 (42), 285 (5) |
15 |
Caffeic acid prenyl ester |
25.6 |
247 |
179 (100), 161 (4), 135 (19) |
16 |
Chrysin |
26.3 |
253 |
209 (100), 143 (36) |
17 |
Caffeic acid prenyl ester isomer |
26.9 |
247 |
179 (100), 135 (47) |
18 |
Caffeic acid prenyl ether |
27.0 |
247 |
203 (33), 179 (100), 134 (46), 135 (41) |
19 |
Caffeic acid benzyl ester |
27.3 |
269 |
178 (49), 134 (100) |
20 |
Pinocembrin |
27.8 |
255 |
213 (100), 169 (29), 151 (65), 107 (21) |
21 |
Pinobanksin-acetate |
28.0 |
313 |
271 (100), 253 (38) |
22 |
Pinobanksin derivative |
29.1 |
371 |
271 (4), 253 (100) |
23 |
Galangin |
29.4 |
269 |
227 (100), 197 (65) |
24 |
Pinobanksin-3-O-acetate |
31.0 |
313 |
271 (13), 253 (100) |
25 |
Caffeic acid phenylethyl ester (CAPE) |
31.8 |
283 |
179 (100), 135 (44) |
26 |
Methoxy-chrysin |
32.4 |
283 |
268 (100), 239 (11) |
27 |
p-Coumaric acid prenyl ester |
36.1 |
231 |
163 (100), 119 (32) |
28 |
Pinobanksin-3-O-propionate |
37.8 |
327 |
253 (100), 271 (83) |
29 |
Caffeic acid cinnamyl ether |
40.2 |
295 |
251 (21), 178 (84), 161 (10), 134 (100) |
30 |
Chrysin derivative |
42.1 |
327 |
253 (100) |
31 |
p-Coumaric acid derivative |
43.0 |
267 |
163 (100), 119 (36) |
32 |
Pinobanksin-5-methyl-ether-3-O-pentanoate |
44.9 |
369 |
267 (100), 285 (78), 239 (49) |
33 |
Pinobanksin-butyrate or isobutyrate |
46.1 |
341 |
271 (24), 253 (100), 151 (10) |
34 |
Pinobanksin-butyrate or isobutyrate |
46.4 |
341 |
271 (100), 253 (37) |
35 |
p-Coumaric acid cinnamyl ether |
47.8 |
279 |
235 (32), 162 (100), 118 (33) |
36 |
Pinobanksin-butyrate or isobutyrate |
48.4 |
341 |
271 (4), 253 (100) |
37 |
Chrysin derivative |
48.9 |
353 |
253 (100) |
38 |
Pinobanksin-pentenoate |
49.2 |
353 |
271 (100), 253 (34) |
39 |
Caffeic acid derivative |
49.8 |
399 |
355 (84), 179 (100), 135 (67) |
40 |
Caffeic acid derivative |
50.1 |
399 |
355 (8), 179 (100), 135 (20) |
41 |
Pinobanksin derivative |
50.7 |
389 |
271 (100), 253 (18) |
42 |
Pinobanksin pentanoate |
51.6 |
355 |
271 (4), 253 (100) |
43 |
Pinobanksin hexenoate |
52.2 |
367 |
271 (100), 253 (33) |
44 |
Fatty acid |
52.4 |
297 |
279 (94), 237 (100), 209 (34), 171 (30), 141 (15) |
45 |
Pinobanksin derivative |
52.8 |
403 |
271 (16), 253 (100) |
46 |
Pinobanksin hexanoate |
53.7 |
369 |
271 (5), 253 (100) |
47 |
Fatty acid |
54.0 |
293 |
197 (43), 185 (100), 125 (12) |
48 |
Pinobanksin hexanoate |
54.0 |
369 |
271 (5), 253 (100) |
49 |
Fatty acid |
54.6 |
293 |
275 (22), 197 (31), 185 (100), 125 (18) |
50 |
Pinobanksin derivative |
54.9 |
429 |
271 (100), 253 (20) |
3.3. Cytotoxicity testing
For cytotoxicity testing, the cells were exposed for 4 h to the BPP extract S1 over a range of five scalar concentrations, from 25 to 500 μg mL−1. The highest concentration chosen was based on previous solubility tests. The viability of cells was evaluated by the double staining procedure with AO/DAPI fluorochromes. Results were expressed as percentage variation of cell viability with respect to the negative control (untreated cells), taken as 100%.
The results showed that the cell viability of HepG2 and HepaRG cells decreased in a concentration-dependent manner (Fig. 2), with a more marked cytotoxic effect of the BPP extract S1 in HepG2 tumour cells. After 4 h exposure, the HepG2 cell viability significantly decreased following treatment with the highest test concentrations of the BPP extract (i.e., 250 and 500 μg mL−1), as compared with the negative control.
 |
| Fig. 2 Effects of BPP extract S1 on cell viability in HepG2 and HepaRG cells after 4 or 24 h exposure. Cytotoxic effects were assessed by AO/DAPI double staining. Results are summarized as mean ± SEM [0 μg mL−1 = vehicle control: 98 : 2 (v/v) DMSO : EtOH]. | |
The results of the AO/DAPI viability test determined the choice of concentrations to be evaluated afterwards. The next steps were then conducted using BPP extract S1 concentrations which did not show cytotoxic effects. In this approach, we considered as cytotoxic those concentrations that led to cell viability lower than 55 ± 5%, in accordance with OECD guidelines.74
3.4. Genotoxicity/antigenotoxicity testing
Genotoxicity testing was performed using the three highest concentrations that did not show cytotoxic effects in the AO/DAPI viability test (i.e., 25, 50, and 100 μg mL−1). After a 4 h-exposure, none of the three tested concentrations of the BPP extract S1 induced any statistically significant increase in the extent of DNA strand breakage as measured by the standard alkaline comet assay, as compared with negative controls. On the other hand, the positive control (i.e., 1 μM 4NQO) yielded a clear genotoxic response (p < 0.05), thus indicating the sensitivity and validity of the test system (data not shown).
3.4.1. Co-exposure treatment.
Fig. 3 summarizes the extent of primary DNA damage in HepG2 and HepaRG cell cultures concomitantly exposed to BPP extract S1 and 4NQO. HepG2 cells showed a typical J-shaped concentration-response curve of residual DNA damage, with BPP extract S1 showing a statistically significant antigenotoxic effect at concentrations of 25 and 50 μg mL−1. Observed genotoxic inhibition rate (GIR%) values were 89.4 and 85.4% for the 25 and 50 μg mL−1 concentrations, respectively, the 25 μg mL−1 concentration being the most effective. In contrast, no antigenotoxic effects were observed for concentrations higher or lower than 25 and 50 μg mL−1.
 |
| Fig. 3 Antigenotoxic effects of the BPP extract S1 on 4NQO-induced DNA damage in HepG2 and HepaRG cells: co-exposure protocol. Each result is expressed as the mean ± standard error of the mean (SEM) of three independent experiments. *p < 0.05 vs. 1 μM 4NQO, one-way ANOVA. | |
The same, less marked trend was observed for HepaRG cells. In this case, a statistically significant antigenotoxic effect was observed at concentrations of 12.5 and 25 μg mL−1. Observed genotoxic inhibition rate (GIR%) values were 48.7 and 41.45% for 12.5 and 25 μg mL−1 concentrations.
3.4.2. Pre- and post-exposure treatment.
Fig. 4 and 5 summarize the observed antigenotoxic effects obtained in HepG2 and HepaRG cells subjected to pre- or post-treatment with the BPP extract S1 toward the extent of 4NQO-induced DNA damage. Statistically significant effects were never observed, even if for HepaRG cells an effect trend is visible in the post-treatment protocol.
 |
| Fig. 4 Antigenotoxic effects of the BPP extract S1 on 4NQO-induced DNA damage in HepG2 and HepaRG cells: pre-exposure protocol. Each result is expressed as the mean ± standard error of the mean (SEM) of three independent experiments. | |
 |
| Fig. 5 Antigenotoxic effects of the BPP extract S1 on 4NQO-induced DNA damage in HepG2 and HepaRG cells: post-exposure protocol. Each result is expressed as the mean ± standard error of the mean (SEM) of three independent experiments. | |
3.5. Anti-inflammatory activity: cyclooxygenase activity (HepG2 cells)
COX activity assay was performed using the three highest concentrations which did not show cytotoxic effects in HepG2 cells in the AO/DAPI assay. The BPP extract S1 showed marked COX-inhibiting activity in HepG2 cells as well as a clear, linear concentration-effect relationship with the highest effect observed at 100 μg mL−1 (Fig. 6).
 |
| Fig. 6 Anti-inflammatory potentialities of the BPP extract S1 in HepG2 cells assessed by the COX activity assay; results of each experimental set are summarised as the mean ± standard error of the mean (SEM) of three independent experiments. r, Pearson's correlation coefficient. | |
3.6. Anti-inflammatory activity: cytokine determination (PBMCs)
To evaluate the anti-inflammatory activity of the BPP extract S1 on human PBMCs, the cytotoxicity of the extract on healthy donor cells was evaluated. The viability of PBMCs treated with different concentrations of BPP extract S1 has been determined by an ATP assay. A CC50 of 118.05 μg mL−1 has been observed after 24 h incubation. Based on this result, it was decided to test the BPP extract S1 at the non-toxic concentration of 50 μg mL−1. The anti-inflammatory activity was determined by stimulating PBMCs with the BPP extract S1 before, after, or simultaneously with the LPS inflammatory stimulus.
Pre-treatment with 50 μg mL−1 BPP extract S1 induced a significant decrease in TNF-α production in LPS-treated cells (Fig. 7A), suggesting a possible inflammatory prevention effect. A similar downregulation was observed with the LPS + BPP extract S1 co-treatment (Fig. 7B). No effect was observed when the cells were treated with the BPP extract S1 after 4 h stimulation with LPS (Fig. 7C). The pro-inflammatory cytokine IL1-β production was significantly reduced both in the pre-treatment (Fig. 8A) and when the extract was added to the cells in combination with LPS (Fig. 8B) with the BPP extract S1 but also in the post-treatment (Fig. 8C).
 |
| Fig. 7 Effect of co- (A), pre- (B), or post-(C) treatment with BPP extract S1 (50 μg mL−1) on TNF-α production by PBMCs stimulated with LPS. TNF-α was determined by using an ELISA assay. *p < 0.05 and **p < 0.01 (treated cells vs. untreated cells); #p < 0.05 and ##p < 0.01 (BPP extract S1 + LPS treated cells vs. LPS treated cells). | |
 |
| Fig. 8 Effect of co- (A), pre- (B), or post-(C) treatment with BPP extract S1 (50 μg mL−1) on IL-1β production by PBMCs stimulated with LPS. IL-1β was determined by using an ELISA assay. *p < 0.05 and **p < 0.01 (treated cells vs. untreated cells); #p < 0.05 and ##p < 0.01 (BPP extract S1 + LPS treated cells vs. LPS treated cells). | |
3.7. ROS production
The antioxidant effect of BPP extract S1 at 5 and 50 μg mL−1 on ROS production by human neutrophils activated by PMA was evaluated by a chemiluminescence assay. The results shown in Fig. 9 demonstrated that BPP extract S1, at both doses studied, was able to reduce ROS production by neutrophils activated with PMA.
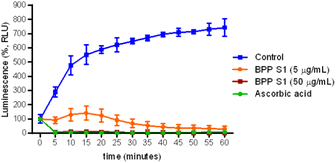 |
| Fig. 9 Effect of BPP extract S1 (5 and 50 μg mL−1) on ROS production by human PMN stimulated with 10−7 PMA. The chemiluminescence produced by the cells was monitored for 60 min in a luminometer. Ascorbic acid was used as positive control. Results are expressed as Relative Luminescence Unit (RLU). Data represent the mean of three independent experiments performed in triplicate. | |
4. Discussion
Many studies have unquestionably demonstrated that the TPC of a plant-derived extract (but this can be extended also to non-plant-derived matrices) is directly related to its antioxidant properties, which is intimately connected to TAC.75 The latter property is strictly dependent upon the synergistic interaction effects of all antioxidants in a given matrix (especially of dietary interest).76 TAC is therefore regarded as a global measure of non-enzymatic antioxidant efficiency, and considers the cumulative and synergistic action of all the antioxidants present in a given matrix, thereby providing an integrated parameter rather than the simple sum of measurable antioxidants.77
Both for the TPC and the TAC evaluation, it is a common practice that researchers adapt, case-by-case, slightly different procedures, which rather complicates to make accurate comparisons among literature data, especially when obtained in different laboratories. However, being aware of this actual limitation, we tried to make some comparative analyses of our results with those available in the scientific literature. As previously anticipated (section 3.1), the TPC of BPP extract S1 was found to be among the highest measured so far for similar extracts.64–66
As far as the TAC is concerned, three different spectrophotometric methods were applied. Numerous studies have clearly highlighted a strict dependence of TAC on the type of assay selected for its evaluation.39,40 Indeed, since TAC value is strictly dependent on the chemical characteristics of the phenols present in a mixture (such as in the present case), a single method to univocally determine this value in vitro does not exist. As a result, the application of different assays for a realistic TAC evaluation is strongly recommended.39,40 Those methods must be sensitive enough to compounds activating either single electron transfer (ET) or hydrogen atom transfer (HAT) mechanisms, or both. Accordingly, in the present study, TAC values were determined through the FRAP, DPPH and ABTS methods, with the first being a well-known ET reaction-based assay, while the other two scavenging models were used to explore the HAT ability.39,40 According to the results shown in section 3.1, the TAC values obtained for BPP extract S1 were among the highest found in the literature for similar extracts.64,65
As far as the HPLC-ESI-MSn analysis of BPP extract S1 is concerned, due to the high number of components, the way allowing their identification will be discussed according to the chemical class. Regarding phenolic acids, caffeic acid and p-coumaric acid derivatives (compounds 15, 17–19, 25, 27, 29, 31, 35, 39 and 40) were detected in the sample. They were all characterized by a fragment corresponding to the loss of the carboxyl group (−44 Da) in their MS2 spectra.67 Compounds 15, 17, 18 and 27 showed a product ion corresponding to the loss of a prenyl group (−68 Da), and, therefore, they were identified as prenyl derivatives. In particular, prenyl esters of caffeic and p-coumaric acids (compounds 15, 17 and 27) showed the [M − prenyl]− and [M − prenyl − CO2]− fragments.67–73 Compound 18, in addition to the previously mentioned fragments, showed an additional product ion at m/z 203, corresponding to [M − CO2]−, leaving the hypothesis of the presence of a free carboxyl group; therefore, it was tentatively identified as a caffeic acid prenyl ether. The same rationale was followed for compounds 29 and 35, which showed the presence of the [M − CO2]− product ion, in addition to the [M − cinnamyl]− and [M − cinnamyl − CO2]− ones; therefore, they were tentatively identified as cinnamyl ethers of caffeic and p-coumaric acid, respectively. A benzyl derivative (compound 19) was identified, according to the presence of a product ion attributable to the loss of 91 Da.67–73 Compound 25 was identified as caffeic acid phenylethyl ester (CAPE), as the fragmentation pattern was in accordance to what has been previously described in the literature for the same compound.67 Finally, compounds 31, 39 and 40 were identified as caffeic and p-coumaric acid derivatives, given the presence of product ions at m/z values corresponding to [M − H]− and [M − CO2]−, respectively.
As to flavonoids, the analysis of the sample allowed for the detection of dihydroflavonols (compounds 1, 2, 4, 11, 21, 22, 24, 28, 32–34, 36, 38, 41–43, 45, 46, 48 and 50), flavones (compounds 5, 8, 16, 26, 30 and 37), flavanones (compound 20), and flavonols (3, 6, 7, 9, 10 and 12–14), either as free form or their methylated or esterified forms. In particular, it was possible to identify the aglycones forms of pinobanksin (compound 4, [M − H]− = 271) apigenin (compound 5, [M − H]− = 269), kaempferol (compound 6, [M − H]− = 285), isorhamnetin (compound 7, [M − H]− = 315), chrysin (compound 16, [M − H]− = 253), pinocembrin (compound 20, [M − H]− = 255) and galangin (compound 23, [M − H]− = 269). The MS2 spectrum of compounds 4 and 20 revealed a product ion at m/z 151, which is the generated from a retro Diels–Alder mechanism.67 Methylated flavonoids (1–3, 8–14, 26 and 32) showed also a significant [M − H − CH3]− product ion (−15 Da).67 In addition, fragments commonly described to be generated from these compounds are related to the loss CO (−28 Da), CO2 (−44 Da) and C2H2O (−42 Da), as well as the consecutive losses of these small molecules.67
The MS2 spectrum of the methyl ethers of pinobanksin (compounds 1, 2, and 11), quercetin (compounds 3, 9 and 13), kaempferol (compound 14), luteolin (compound 8) and galangin (compound 10) showed the presence of product ions attributable to the loss of a single (for mono-methylated derivative) or multiple (for di-methylated derivatives) methyl groups (−15 Da). Regarding these propolis constituents, in most cases their fragmentation patterns suggested that the methyl substituents are linked to the γ-benzopyrone moiety, but the exact position could not be discriminated by the MS2 analysis, and the identification was attributed on the basis of the comparison of the experimental data with those available in the literature.67–73
The esterified derivatives of the above-cited aglycons (compounds 21, 24, 28, 33, 34, 36, 38, 42, 43, 46 and 48) with different groups (acetate, propionate, butyrate or isobutyrate, pentanoate, pentenoate, hexanoate and hexenoate) were also identified, given the presence of products ions in their MS2 spectra attributable to the loss of the respective ester groups.67–73 The exact position of the ester bond, when possible, was assigned by comparing both the chromatographic data and the fragmentation pattern with data available in the literature for the same compounds in propolis samples.67–73 Compound 38 was tentatively identified as pinobanksin pentenoate, as its fragmentation pattern resembled that of other pinobanksin esters, but it showed a neutral loss of 82 Da, which might correspond to a pentenoate group, since it differed from only 2 Da from the neutral loss observed for compound 42. The same rationale was followed for the tentative identification of compound 43, where the neutral loss of 96 Da might be attributable to a hexenoate group. As for the position of the double bonds in these molecules, further NMR analysis must be performed on pure compounds to confirm their structures.
Additional compounds with a fragmentation pattern attributable to fatty acids were also identified, though their structure was not identified (compounds 44, 47 and 49).73
Many relevant potential health properties have been demonstrated for many of the compounds identified in the three BPP extracts, some of which are briefly reported below.
Dihydroflavonols such as pinobanksin and its derivatives have been demonstrated to produce a remarkable anti-proliferative effect, induced through apoptosis in a B-cell lymphoma cancer cell line.78
Chrysin was shown to act against murine B16F10, human colon carcinoma HCT-15 and human hepatoma Hep-3B cells.79
This compound plays an important role in the prevention of several pathophysiological conditions and disorders, including cancer, oxidative stress, inflammatory disorders, diabetes mellitus, cardiovascular diseases, obesity, and allergic events.80
Independent studies in numerous animal models have also revealed its neuroprotective and hepatoprotective functions along with its contribution in improving reproductive health.81
The inclusion of chrysin-containing foods in the diet has been also demonstrated to mitigate the occurrence of several diseases. Accordingly, it has been suggested to rely upon chrysin-containing foods as a prophylactic strategy to reduce the risk of several diseases.80
As far as pinocembrin is concerned, several in vitro and preclinical studies have put into light its very favourable antioxidant, anti-inflammatory, antimicrobial, neuroprotective, cardioprotective and anticancer activities.82,83
Relevant health-beneficial properties have been also demonstrated for galangin. These compounds are indeed able to exert anti-oxidative and radical scavenging activities,84–86 besides being able to inhibit mast cell-derived allergic inflammation.87 Interestingly, galangin has also shown anti-inflammatory effects on collagen-induced arthritis mice without toxicity.88
Anti-inflammatory properties have been also demonstrated for the caffeic acid phenethyl ester (CAPE). This compound has been found to be an influential inhibitor in T-cell receptor-mediated T-cell activation.89
The relevant synergistic action of the above compounds has been also well demonstrated.90
Undoubtedly, to better deepen our BPP extract health effects, further investigations (such as in vivo ones certainly) are necessary since many of the in vitro studies on polyphenols do not consider the physiological concentrations after their intake likewise the degree of their bioavailability and absorption when circulating in blood vessels or even the metabolic transformation which normally occurs and the contemporary presence of more than one metabolite.
Nevertheless, several studies in recent decades have found out that metabolites originating in vivo from ingested polyphenols may carry out various positive roles in the human body's biological pathways,91 coherently to those highlighted in the present study.
The investigation of potential health-promoting properties resulted in interesting features, which corroborate the results obtained in chemical analysis and tests. BPP extract S1 did not lead to a significant loss of viability until the concentration of 100 μg mL−1 in both hepatic cell lines (i.e., HepG2 and HepaRG).
As regards antigenotoxicity testing, the BPP extract S1 showed a typical, J-shaped hormetic response (in the co-exposure protocol), with hormesis that can be defined as an adaptive, non-monotonic, biphasic dose-response relationship, characterized by small quantities having opposite effects than large quantities.92 Biphasic dose-dependent effects have been largely reported in the literature, in particular for polyphenolic compounds that are known to induce hormetic responses in a wide range of biological models, affecting numerous endpoints of biomedical and therapeutic significance.93 Additionally, hormesis has been identified as an adaptive mechanism by which mild stressors can enhance the protective capacity of the host, while those at very high levels are harmful or lethal; obviously, at very low levels no effects are observed. In other words, there is an optimum dose (or narrow range of doses) for a compound or a phytochemical to exert beneficial functions, and an overdose will likely lead to side effects or toxicity.94
Moreover, the BPP extract S1 showed interesting anti-inflammatory potentialities, both in HepG2 cells (a clear, linear concentration-effect inhibition of COX activity) and in PBMCs. Our results support previous studies82,95 that indicate poplar buds as a potential natural anti-inflammatory agent by regulating the production of immune mediators. The extract (50 μg mL−1) suppressed the production of key pro-inflammatory cytokines (IL-1β and TNF-α). Interestingly, the same concentration was also able to exert a remarkable antigenotoxic activity in HepG2 cells. The anti-inflammatory activity of bud extracts could be somewhat associated with the presence of flavonoids, in particular with the synergy of caffeic acid and flavonoids present in poplar.96
In addition, the BPP extract S1 was also able to modulate ROS production, in line with previous studies in stimulated mammalian PMN.97,98 These data support information obtained from the evaluation of antioxidant and antigenotoxic activity, and attest to the high quality of this product in its potential health benefits. Given the link between inflammatory status and oxidative stress with noncommunicable diseases (NCDs),99,100 these results unquestionably merit further investigation. If these properties will be confirmed in vivo and prospective studies, this product may be included—in different forms—in healthy diets, along with other antioxidant and anti-inflammatory products101–103 and be helpful in the prevention of a broad spectrum of NCDs.
5. Conclusions
The results showed an interesting chemical profile and promising antioxidant, antigenotoxic, anti-inflammatory and ROS-modulating activities of this specific BPP extract, suggesting that this product deserves deeper investigations, paving the way for future research. In vivo studies will be a possible line to take, which may help corroborate the hypothesis of the potential health benefits of this product.
Author contributions
Conceptualization: RS, MM, and MV. Data curation: MA, RS, MM, and MV. Formal analysis: MA, FP, RS, MM, and MV. Funding acquisition: MM. Investigation: MA, IV, VB, ECC, TR, CF, CR, DP, FP, and DB. Methodology: DP, FP, RS, and MM. Project administration: MM. Resources: DP, FP, RS, DB, MM, and MV. Software: MA, RS, and MM. Supervision: DP, RS, and MM. Validation: DP, FP, RS, and MM. Visualization: MA, RS, and MM. Writing – original draft: MA, RS, and MM. Writing – review and editing: MA, IV, VB, ECC, TR, CF, CR, DP, FP, DB, RS, MM, and MV.
Conflicts of interest
The authors have no competing interests to declare that are relevant to the content of this article.
Acknowledgements
This study was supported by the University of Perugia (Fondo di Finanziamento della Ricerca di Base del Dipartimento di Scienze Farmaceutiche 2019 – Prof. Massimo Moretti).
References
- J. Kocot, M. Kiełczykowska, D. Luchowska-Kocot, J. Kurzepa and I. Musik, Antioxidant Potential of Propolis, Bee Pollen, and Royal Jelly: Possible Medical Application, Oxid. Med. Cell. Longevity, 2018, 2018, 7074209, DOI:10.1155/2018/7074209.
- C. Sun, Z. Wu, Z. Wang and H. Zhang, Effect of Ethanol/Water Solvents on Phenolic Profiles and Antioxidant Properties of Beijing Propolis Extracts, J. Evidence-Based Complementary Altern. Med., 2015, 2015, 595393, DOI:10.1155/2015/595393.
- V. R. Pasupuleti, L. Sammugam, N. Ramesh and S. H. Gan, Honey, Propolis, and Royal Jelly: A Comprehensive Review of Their Biological Actions and Health Benefits, Oxid. Med. Cell. Longevity, 2017, 2017, 1259510, DOI:10.1155/2017/1259510.
- T. Bonamigo, J. F. Campos, A. S. Oliveira, H. F. V. Torquato, J. B. P. Balestieri, C. A. L. Cardoso, E. J. Paredes-Gamero, K. de Picoli Souza and E. L. Dos Santos, Antioxidant and Cytotoxic Activity of Propolis of Plebeia Droryana and Apis Mellifera (Hymenoptera, Apidae) from the Brazilian Cerrado Biome, PLoS One, 2017, 12(9), e0183983, DOI:10.1371/journal.pone.0183983.
- S. El-Guendouz, B. Lyoussi and M. G. Miguel, Insight on Propolis from Mediterranean Countries: Chemical Composition, Biological Activities and Application Fields, Chem. Biodivers., 2019, 16(7), e1900094, DOI:10.1002/cbdv.201900094.
- T. Bonamigo, J. F. Campos, T. M. Alfredo, J. B. P. Balestieri, C. A. L. Cardoso, E. J. Paredes-Gamero, K. de Picoli Souza and E. L. Dos Santos, Antioxidant, Cytotoxic, and Toxic Activities of Propolis from Two Native Bees in Brazil: Scaptotrigona Depilis and Melipona Quadrifasciata Anthidioides, Oxid. Med. Cell. Longevity, 2017, 2017, 1038153, DOI:10.1155/2017/1038153.
- S. Boisard, A.-M. Le Ray, J. Gatto, M.-C. Aumond, P. Blanchard, S. Derbré, C. Flurin and P. Richomme, Chemical Composition, Antioxidant and Anti-AGEs Activities of a French Poplar Type Propolis, J. Agric. Food Chem., 2014, 62(6), 1344–1351, DOI:10.1021/jf4053397.
- J. M. Sforcin, A. Fernandes, C. A. Lopes, V. Bankova and S. R. Funari, Seasonal Effect on Brazilian Propolis Antibacterial Activity, J. Ethnopharmacol., 2000, 73(1–2), 243–249, DOI:10.1016/s0378-8741(00)00320-2.
- S. Silici and S. Kutluca, Chemical Composition and Antibacterial Activity of Propolis Collected by Three Different Races of Honeybees in the Same Region, J. Ethnopharmacol., 2005, 99(1), 69–73, DOI:10.1016/j.jep.2005.01.046.
- K. Graikou, M. Popova, O. Gortzi, V. Bankova and I. Chinou, Characterization and Biological Evaluation of Selected Mediterranean Propolis Samples. Is It a New Type?, LWT – Food Sci. Technol., 2016, 65, 261–267, DOI:10.1016/j.lwt.2015.08.025.
- V. Bankova, Chemical Diversity of Propolis and the Problem of Standardization, J. Ethnopharmacol., 2005, 100(1–2), 114–117, DOI:10.1016/j.jep.2005.05.004.
- A. Salatino and M. L. F. Salatino, Brazilian Red Propolis: Legitimate Name of the Plant Resin Source, MOJ Food Process. Technol., 2018, 6(1), 21–22, DOI:10.15406/mojfpt.2018.06.00139.
- M. L. F. Bittencourt, P. R. Ribeiro, R. L. P. Franco, H. W. M. Hilhorst, R. D. Castro and L. G. Fernandez, Metabolite Profiling, Antioxidant and Antibacterial Activities of Brazilian Propolis: Use of Correlation and Multivariate Analyses to Identify Potential Bioactive Compounds, Food Res. Int., 2015, 76(Pt 3), 449–457, DOI:10.1016/j.foodres.2015.07.008.
- A. Daugsch, C. S. Moraes, P. Fort and Y. K. Park, Brazilian Red Propolis–Chemical Composition and Botanical Origin, J. Evidence-Based Complementary Altern. Med., 2008, 5(4), 435–441, DOI:10.1093/ecam/nem057.
- P. Ristivojević, J. Trifković, F. Andrić and D. Milojković-Opsenica, Poplar-Type Propolis: Chemical Composition, Botanical Origin and Biological Activity, Nat. Prod. Commun., 2015, 10(11), 1869–1876 CrossRef.
- M. Shahinozzaman, D. N. Obanda and S. Tawata, Chemical Composition and Pharmacological Properties of Macaranga-Type Pacific Propolis: A Review, Phytother. Res., 2021, 35(1), 207–222, DOI:10.1002/ptr.6819.
- S. Bogdanov, Propolis: Composition, Health, Medicine: A Review, Bee Prod. Sci., 2014, 1–40 Search PubMed.
- J. M. Sforcin, Biological Properties and Therapeutic Applications of Propolis, Phytother. Res., 2016, 30(6), 894–905, DOI:10.1002/ptr.5605.
- S. Martinotti and E. Ranzato, Propolis: A New Frontier for Wound Healing?, Burns Trauma, 2015, 3, 9, DOI:10.1186/s41038-015-0010-z.
- N. Zabaiou, A. Fouache, A. Trousson, S. Baron, A. Zellagui, M. Lahouel and J.-M. A. Lobaccaro, Biological Properties of Propolis Extracts: Something New from an Ancient Product, Chem. Phys. Lipids, 2017, 207(Pt B), 214–222, DOI:10.1016/j.chemphyslip.2017.04.005.
- A. K. Kuropatnicki, E. Szliszka and W. Krol, Historical Aspects of Propolis Research in Modern Times, J. Evidence-Based Complementary Altern. Med., 2013, 2013, 964149, DOI:10.1155/2013/964149.
- S. Castaldo and F. Capasso, Propolis, an Old Remedy Used in Modern Medicine, Fitoterapia, 2002, 73(Suppl 1), S1–S6, DOI:10.1016/s0367-326x(02)00185-5.
-
E. Crane, The Past and Present Importance of Bee Products to Man, in Bee Products: Properties, Applications, and Apitherapy, ed. A. Mizrahi and Y. Lensky, Springer US, Boston, MA, 1997; pp. 1–13. DOI:10.1007/978-1-4757-9371-0_1.
- E. Rojczyk, A. Klama-Baryła, W. Łabuś, K. Wilemska-Kucharzewska and M. Kucharzewski, Historical and Modern Research on Propolis and Its Application in Wound Healing and Other Fields of Medicine and Contributions by Polish Studies, J. Ethnopharmacol., 2020, 262, 113159, DOI:10.1016/j.jep.2020.113159.
- R. Silva-Carvalho, F. Baltazar and C. Almeida-Aguiar, Propolis: A Complex Natural Product with a Plethora of Biological Activities That Can Be Explored for Drug Development, J. Evidence-Based Complementary Altern. Med., 2015, 2015, 206439, DOI:10.1155/2015/206439.
- I. Przybyłek and T. M. Karpiński, Antibacterial Properties of Propolis, Molecules, 2019, 24(11), 2047, DOI:10.3390/molecules24112047.
- M. S. Almuhayawi, Propolis as a Novel Antibacterial Agent, Saudi J. Biol. Sci., 2020, 27(11), 3079–3086, DOI:10.1016/j.sjbs.2020.09.016.
- A. Magnavacca, E. Sangiovanni, G. Racagni and M. Dell'Agli, The Antiviral and Immunomodulatory Activities of Propolis: An Update and Future Perspectives for Respiratory Diseases, Med. Res. Rev., 2022, 42(2), 897–945, DOI:10.1002/med.21866.
- P. Cerqueira, A. Cunha and C. Almeida-Aguiar, Potential of Propolis Antifungal Activity for Clinical Applications, J. Appl. Microbiol., 2022, 133(3), 1207–1228, DOI:10.1111/jam.15628.
- L. A. de L Paula, A. C. B. B. Cândido, M. F. C. Santos, C. R. Caffrey, J. K. Bastos, S. R. Ambrósio and L. G. Magalhães, Antiparasitic Properties of Propolis Extracts and Their Compounds, Chem. Biodivers., 2021, 18(9), e2100310, DOI:10.1002/cbdv.202100310.
- H. Nazari-Bonab, P. Jamilian, N. Radkhah, M. Zarezadeh and M. Ebrahimi-Mameghani, The Effect of Propolis Supplementation in Improving Antioxidant Status: A Systematic Review and Meta-Analysis of Controlled Clinical Trials, Phytother. Res., 2023, 37(9), 3712–3723, DOI:10.1002/ptr.7899.
- F. F. Dos Santos, R. P. Morais-Urano, W. R. Cunha, S. G. de Almeida, P. S. D. S. R. Cavallari, H. A. Manuquian, H. d. A. Pereira, R. Furtado, M. F. C. Santos and M. L. Amdrade E Silva, A Review on the Anti-Inflammatory Activities of Brazilian Green, Brown and Red Propolis, J. Food Biochem., 2022, 46(10), e14350, DOI:10.1111/jfbc.14350.
- F. Zulhendri, R. Lesmana, S. Tandean, A. Christoper, K. Chandrasekaran, I. Irsyam, A. A. Suwantika, R. Abdulah and N. Wathoni, Recent Update on the Anti-Inflammatory Activities of Propolis, Molecules, 2022, 27(23), 8473, DOI:10.3390/molecules27238473.
- S. Altabbal, K. Athamnah, A. Rahma, A. F. Wali, A. H. Eid, R. Iratni and Y. Al Dhaheri, Propolis, A Detailed Insight of Its Anticancer Molecular Mechanisms, Pharmaceuticals, 2023, 16(3), 450, DOI:10.3390/ph16030450.
- E. Forma and M. Bryś, Anticancer Activity of Propolis and Its Compounds, Nutrients, 2021, 13(8), 2594, DOI:10.3390/nu13082594.
- K. D. Helfenberg, The Analysis of Beeswax and Propolis, J. Prakt. Chem./Chem.-Ztg., 1908, 31, 987–998 CAS.
-
NICEATM/ICCVAM, Test Method Protocol for Solubility Determination, In Vitro Cytotoxicity Validation Study Phase III, National Institute of Environmental Health Sciences (NIEHS), Research Triangle Park, NC (USA), 2006 Search PubMed.
- M. Popova, E. Giannopoulou, K. Skalicka-Woźniak, K. Graikou, J. Widelski, V. Bankova, H. Kalofonos, G. Sivolapenko, K. Gaweł-Bęben, B. Antosiewicz and I. Chinou, Characterization and Biological Evaluation of Propolis from Poland, Molecules, 2017, 22(7), E1159, DOI:10.3390/molecules22071159.
- L. Pucciarini, F. Ianni, V. Petesse, F. Pellati, V. Brighenti, C. Volpi, M. Gargaro, B. Natalini, C. Clementi and R. Sardella, Onion (Allium Cepa L.) Skin: A Rich Resource of Biomolecules for the Sustainable Production of Colored Biofunctional Textiles, Molecules, 2019, 24(3), 634, DOI:10.3390/molecules24030634.
- C. Puri, L. Pucciarini, M. Tiecco, V. Brighenti, C. Volpi, M. Gargaro, R. Germani, F. Pellati, R. Sardella and C. Clementi, Use of a Zwitterionic Surfactant to Improve the Biofunctional Properties of Wool Dyed with an Onion (Allium Cepa L.) Skin Extract, Antioxidants, 2020, 9(11), 1055, DOI:10.3390/antiox9111055.
- F. Pellati, F. P. Prencipe, D. Bertelli and S. Benvenuti, An Efficient Chemical Analysis of Phenolic Acids and Flavonoids in Raw Propolis by Microwave-Assisted Extraction Combined with High-Performance Liquid Chromatography Using the Fused-Core Technology, J. Pharm. Biomed. Anal., 2013, 81–82, 126–132, DOI:10.1016/j.jpba.2013.04.003.
- M. Acito, D. Bartolini, M. R. Ceccarini, C. Russo, S. Vannini, L. Dominici, M. Codini, M. Villarini, F. Galli, T. Beccari and M. Moretti, Imbalance in the Antioxidant Defence System and Pro-Genotoxic Status Induced by High Glucose Concentrations: In Vitro Testing in Human Liver Cells, Toxicol. in Vitro, 2020, 69, 105001, DOI:10.1016/j.tiv.2020.105001.
- R. di Vito, S. Levorato, C. Fatigoni, M. Acito, L. Sancineto, G. Traina, M. Villarini, C. Santi and M. Moretti, In Vitro Toxicological Assessment of PhSeZnCl in Human Liver Cells, Toxicol. Res., 2022, 39(1), 105–114, DOI:10.1007/s43188-022-00148-y.
- D. P. Aden, A. Fogel, S. Plotkin, I. Damjanov and B. B. Knowles, Controlled Synthesis of HBsAg in a Differentiated Human Liver Carcinoma-Derived Cell Line, Nature, 1979, 282(5739), 615–616, DOI:10.1038/282615a0.
- B. B. Knowles, C. C. Howe and D. P. Aden, Human Hepatocellular Carcinoma Cell Lines Secrete the Major Plasma Proteins and Hepatitis B Surface Antigen, Science, 1980, 209(4455), 497–499, DOI:10.1126/science.6248960.
- P. Gripon, S. Rumin, S. Urban, J. Le Seyec, D. Glaise, I. Cannie, C. Guyomard, J. Lucas, C. Trepo and C. Guguen-Guillouzo, Infection of a Human Hepatoma Cell Line by Hepatitis B Virus, Proc. Natl. Acad. Sci. U. S. A., 2002, 99(24), 15655–15660, DOI:10.1073/pnas.232137699.
-
EFSA Scientific Committee, Scientific Opinion on Genotoxicity Testing Strategies Applicable to Food and Feed Safety Assessment, EFS2, 2011, 9(9). DOI:10.2903/j.efsa.2011.2379.
- M. Villarini, R. Pagiotti, L. Dominici, C. Fatigoni, S. Vannini, S. Levorato and M. Moretti, Investigation of the Cytotoxic, Genotoxic, and Apoptosis-Inducing Effects of Estragole Isolated from Fennel (Foeniculum Vulgare), J. Nat. Prod., 2014, 77(4), 773–778, DOI:10.1021/np400653p.
- D. Shah, M. Naciri, P. Clee and M. Al-Rubeai, NucleoCounter—An Efficient Technique for the Determination of Cell Number and Viability in Animal Cell Culture Processes, Cytotechnology, 2006, 51(1), 39–44, DOI:10.1007/s10616-006-9012-9.
- M. Di Nunzio, V. Valli, L. Tomás-Cobos, T. Tomás-Chisbert, L. Murgui-Bosch, F. Danesi and A. Bordoni, Is Cytotoxicity a Determinant of the Different in Vitro and in Vivo Effects of Bioactives?, BMC Complementary Altern. Med., 2017, 17(1), 453, DOI:10.1186/s12906-017-1962-2.
- C. C. Munari, J. M. Alves, J. K. Bastos and D. C. Tavares, Evaluation of the Genotoxic and Antigenotoxic Potential of Baccharis Dracunculifolia Extract on V79 Cells by the Comet Assay, J. Appl. Toxicol., 2010, 30(1), 22–28, DOI:10.1002/jat.1467.
- G. Lombardi, S. Vannini, F. Blasi, M. C. Marcotullio, L. Dominici, M. Villarini, L. Cossignani and M. Moretti, In Vitro Safety/Protection Assessment of Resveratrol and Pterostilbene in a Human Hepatoma Cell Line (HepG2), Nat. Prod. Commun., 2015, 10(8), 1403–1408 CrossRef PubMed.
- R. R. Tice, E. Agurell, D. Anderson, B. Burlinson, A. Hartmann, H. Kobayashi, Y. Miyamae, E. Rojas, J. C. Ryu and Y. F. Sasaki, Single Cell Gel/Comet Assay: Guidelines for in Vitro and in Vivo Genetic Toxicology Testing, Environ. Mol. Mutagen., 2000, 35(3), 206–221, DOI:10.1002/(sici)1098-2280(2000)35:3<206::aid-em8>3.0.co;2-j.
- A. R. Collins, The Comet Assay for DNA Damage and Repair: Principles, Applications, and Limitations, Mol. Biotechnol., 2004, 26(3), 249–261, DOI:10.1385/MB:26:3:249.
- N.-B. Liu, T. Peng, C. Pan, Y.-Y. Yao, B. Shen and J. Leng, Overexpression of Cyclooxygenase-2 in Human HepG2, Bel-7402 and SMMC-7721 Hepatoma Cell Lines and Mechanism of Cyclooxygenase-2 Selective Inhibitor Celecoxib-Induced Cell Growth Inhibition and Apoptosis, World J. Gastroenterol., 2005, 11(40), 6281–6287, DOI:10.3748/wjg.v11.i40.6281.
- H. Koga, S. Sakisaka, M. Ohishi, T. Kawaguchi, E. Taniguchi, K. Sasatomi, M. Harada, T. Kusaba, M. Tanaka, R. Kimura, Y. Nakashima, O. Nakashima, M. Kojiro, T. Kurohiji and M. Sata, Expression of Cyclooxygenase-2 in Human Hepatocellular Carcinoma: Relevance to Tumor Dedifferentiation, Hepatology, 1999, 29(3), 688–696, DOI:10.1002/hep.510290355.
- H. Chen, W. Cai, E. S. H. Chu, J. Tang, C.-C. Wong, S. H. Wong, W. Sun, Q. Liang, J. Fang, Z. Sun and J. Yu, Hepatic Cyclooxygenase-2 Overexpression Induced Spontaneous Hepatocellular Carcinoma Formation in Mice, Oncogene, 2017, 36(31), 4415–4426, DOI:10.1038/onc.2017.73.
- M. Cervello and G. Montalto, Cyclooxygenases in Hepatocellular Carcinoma, World J. Gastroenterol., 2006, 12(32), 5113–5121, DOI:10.3748/wjg.v12.i32.5113.
- G. Aparicio Gallego, S. Díaz Prado, P. Jiménez Fonseca, R. García Campelo, J. Cassinello Espinosa and L. M. Antón Aparicio, Cyclooxygenase-2 (COX-2): A Molecular Target in Prostate Cancer, Clin. Transl. Oncol., 2007, 9(11), 694–702, DOI:10.1007/s12094-007-0126-0.
- M. Zhang, Z.-G. Xu, Z. Shi, D. Shao, O. Li, W. Li, Z.-J. Li, K.-Z. Wang and L. Chen, Inhibitory Effect of Celecoxib in Lung Carcinoma by Regulation of Cyclooxygenase-2/Cytosolic Phospholipase A2 and Peroxisome Proliferator-Activated Receptor Gamma, Mol. Cell. Biochem., 2011, 355(1–2), 233–240, DOI:10.1007/s11010-011-0859-5.
- L. Wang, L.-F. Zhang, J. Wu, S.-J. Xu, Y.-Y. Xu, D. Li, J.-T. Lou and M.-F. Liu, IL-1β-Mediated Repression of microRNA-101 Is Crucial for Inflammation-Promoted Lung Tumorigenesis, Cancer Res., 2014, 74(17), 4720–4730, DOI:10.1158/0008-5472.CAN-14-0960.
- M. Acito, M. Palomba, C. Fatigoni, M. Villarini, L. Sancineto, C. Santi and M. Moretti, Fagiolina Del Trasimeno, an Italian Cowpea Landrace: Effect of Different Cooking Techniques and Domestic Storage on Chemical and Biological Features, Int. J. Food Sci. Technol., 2022, 57, 6557–6571, DOI:10.1111/ijfs.15998.
- Y. M. Lucisano-Valim, L. M. Kabeya, A. Kanashiro, E. M. S. Russo-Carbolante, A. C. M. Polizello, A. E. C. S. Azzolini, S. C. Silva, J. L. C. Lopes, C. A. de Oliveira and B. A. Mantovani, Simple Method to Study the Activity of Natural Compounds on the Chemiluminescence of Neutrophils upon Stimulation by Immune Complexes, J. Pharmacol. Toxicol. Methods, 2002, 47(1), 53–58, DOI:10.1016/s1056-8719(02)00206-x.
- M. Miłek, E. Ciszkowicz, M. Tomczyk, E. Sidor, G. Zaguła, K. Lecka-Szlachta, A. Pasternakiewicz and M. Dżugan, The Study of Chemical Profile and Antioxidant Properties of Poplar-Type Polish Propolis Considering Local Flora Diversity in Relation to Antibacterial and Anticancer Activities in Human Breast Cancer Cells, Molecules, 2022, 27(3), 725, DOI:10.3390/molecules27030725.
- A. Kurek-Górecka, Ş. Keskin, O. Bobis, R. Felitti, M. Górecki, M. Otręba, J. Stojko, P. Olczyk, S. Kolayli and A. Rzepecka-Stojko, Comparison of the Antioxidant Activity of Propolis Samples from Different Geographical Regions, Plants, 2022, 11(9), 1203, DOI:10.3390/plants11091203.
- F. Gatea, A. Matei, E. D. Teodor and G. Radu, Antioxidant Properties and Polyphenols Composition of Some Romanian Propolis Samples, Rev. Roum. Chim., 2015, 60, 65–74 Search PubMed.
- F. Pellati, G. Orlandini, D. Pinetti and S. Benvenuti, HPLC-DAD and HPLC-ESI-MS/MS Methods for Metabolite Profiling of Propolis Extracts, J. Pharm. Biomed. Anal., 2011, 55(5), 934–948, DOI:10.1016/j.jpba.2011.03.024.
- R. Pavlovic, G. Borgonovo, V. Leoni, L. Giupponi, G. Ceciliani, S. Sala, A. Bassoli and A. Giorgi, Effectiveness of Different Analytical Methods for the Characterization of Propolis: A Case of Study in Northern Italy, Molecules, 2020, 25(3), 504, DOI:10.3390/molecules25030504.
- L. Saftić, Ž Peršurić, E. Fornal, T. Pavlešić and S. Kraljević Pavelić, Targeted and, Untargeted LC-MS Polyphenolic Profiling and Chemometric Analysis of Propolis from Different Regions of Croatia, J. Pharm. Biomed. Anal., 2019, 165, 162–172, DOI:10.1016/j.jpba.2018.11.061.
- P. Ristivojević, J. Trifković, U. Gašić, F. Andrić, N. Nedić, Ž Tešić and D. Milojković-Opsenica, Ultrahigh-Performance Liquid Chromatography and Mass Spectrometry (UHPLC-LTQ/Orbitrap/MS/MS) Study of Phenolic Profile of Serbian Poplar Type Propolis, Phytochem. Anal., 2015, 26(2), 127–136, DOI:10.1002/pca.2544.
- S. I. Falcão, N. Vale, P. Gomes, M. R. M. Domingues, C. Freire, S. M. Cardoso and M. Vilas-Boas, Phenolic Profiling of Portuguese Propolis by LC-MS Spectrometry: Uncommon Propolis Rich in Flavonoid Glycosides, Phytochem. Anal., 2013, 24(4), 309–318, DOI:10.1002/pca.2412.
- A. A. Righi, G. Negri and A. Salatino, Comparative Chemistry of Propolis from Eight Brazilian Localities, J. Evidence-Based Complementary Altern. Med., 2013, 2013, 267878, DOI:10.1155/2013/267878.
- S. I. Falcão, M. Vilas-Boas, L. M. Estevinho, C. Barros, M. R. M. Domingues and S. M. Cardoso, Phenolic Characterization of Northeast Portuguese Propolis: Usual and Unusual Compounds, Anal. Bioanal. Chem., 2010, 396(2), 887–897, DOI:10.1007/s00216-009-3232-8.
-
OECD, Guidance Document on Revisions to OECD Genetic Toxicology Test Guidelines, Organisation for Economic Co-operation and Development, Paris, France, 2015 Search PubMed.
- D. A. Jacobo-Velázquez and L. Cisneros-Zevallos, Correlations of Antioxidant Activity against Phenolic Content Revisited: A New Approach in Data Analysis for Food and Medicinal Plants, J. Food Sci., 2009, 74(9), R107–R113, DOI:10.1111/j.1750-3841.2009.01352.x.
- K. Wang and S. Hu, The Synergistic Effects of Polyphenols and Intestinal Microbiota on Osteoporosis, Front. Immunol., 2023, 14, 1285621, DOI:10.3389/fimmu.2023.1285621.
- M. A. Nascimento-Souza, P. G. Paiva, H. S. D. Martino and A. Q. Ribeiro, Dietary Total Antioxidant Capacity as a Tool in Health Outcomes in Middle-Aged and Older Adults: A Systematic Review, Crit. Rev. Food Sci. Nutr., 2018, 58(6), 905–912, DOI:10.1080/10408398.2016.1230089.
- E. Alday, D. Valencia, A. L. Carreño, P. Picerno, A. L. Piccinelli, L. Rastrelli, R. Robles-Zepeda, J. Hernandez and C. Velazquez, Apoptotic Induction by Pinobanksin and Some of Its Ester Derivatives from Sonoran Propolis in a B-Cell Lymphoma Cell Line, Chem.-Biol. Interact., 2015, 242, 35–44, DOI:10.1016/j.cbi.2015.09.013.
- S. Patel, Emerging Adjuvant Therapy for Cancer: Propolis and Its Constituents, J. Diet. Suppl., 2016, 13(3), 245–268, DOI:10.3109/19390211.2015.1008614.
- S. Naz, M. Imran, A. Rauf, I. E. Orhan, M. A. Shariati, n. Iahtisham-Ul-Haq, n. IqraYasmin, M. Shahbaz, T. B. Qaisrani, Z. A. Shah, S. Plygun and M. Heydari, Chrysin: Pharmacological and Therapeutic Properties, Life Sci., 2019, 235, 116797, DOI:10.1016/j.lfs.2019.116797.
- M. F. Tolba, S. S. Azab, A. E. Khalifa, S. Z. Abdel-Rahman and A. B. Abdel-Naim, Caffeic Acid Phenethyl Ester, a Promising Component of Propolis with a Plethora of Biological Activities: A Review on Its Anti-Inflammatory, Neuroprotective, Hepatoprotective, and Cardioprotective Effects, IUBMB Life, 2013, 65(8), 699–709, DOI:10.1002/iub.1189.
- S. De Marco, M. Piccioni, R. Pagiotti and D. Pietrella, Antibiofilm and Antioxidant Activity of Propolis and Bud Poplar Resins versus Pseudomonas Aeruginosa, J. Evidence-Based Complementary Altern. Med., 2017, 2017, 5163575, DOI:10.1155/2017/5163575.
- M. H. Elbatreek, I. Mahdi, W. Ouchari, M. F. Mahmoud and M. Sobeh, Current Advances on the Therapeutic Potential of Pinocembrin: An Updated Review, Biomed. Pharmacother., 2023, 157, 114032, DOI:10.1016/j.biopha.2022.114032.
- T. P. T. Cushnie and A. J. Lamb, Assessment of the Antibacterial Activity of Galangin against 4-Quinolone Resistant Strains of Staphylococcus Aureus, Phytomedicine, 2006, 13(3), 187–191, DOI:10.1016/j.phymed.2004.07.003.
- J. Gwak, J. Oh, M. Cho, S. K. Bae, I.-S. Song, K.-H. Liu, Y. Jeong, D.-E. Kim, Y.-H. Chung and S. Oh, Galangin Suppresses the Proliferation of β-Catenin Response Transcription-Positive Cancer Cells by Promoting Adenomatous Polyposis Coli/Axin/Glycogen Synthase Kinase-3β-Independent β-Catenin Degradation, Mol. Pharmacol., 2011, 79(6), 1014–1022, DOI:10.1124/mol.110.069591.
- M. Y. Heo, S. J. Sohn and W. W. Au, Anti-Genotoxicity of Galangin as a Cancer Chemopreventive Agent Candidate, Mutat. Res., 2001, 488(2), 135–150, DOI:10.1016/s1383-5742(01)00054-0.
- H.-H. Kim, Y. Bae and S.-H. Kim, Galangin Attenuates Mast Cell-Mediated Allergic Inflammation, Food Chem. Toxicol., 2013, 57, 209–216, DOI:10.1016/j.fct.2013.03.015.
- J.-E. Huh, I.-T. Jung, J. Choi, Y.-H. Baek, J.-D. Lee, D.-S. Park and D.-Y. Choi, The Natural Flavonoid Galangin Inhibits Osteoclastic Bone Destruction and Osteoclastogenesis by Suppressing NF-κB in Collagen-Induced Arthritis and Bone Marrow-Derived Macrophages, Eur. J. Pharmacol., 2013, 698(1–3), 57–66, DOI:10.1016/j.ejphar.2012.08.013.
- N. Márquez, R. Sancho, A. Macho, M. A. Calzado, B. L. Fiebich and E. Muñoz, Caffeic Acid Phenethyl Ester Inhibits T-Cell Activation by Targeting Both Nuclear Factor of Activated T-Cells and NF-kappaB Transcription Factors, J. Pharmacol. Exp. Ther., 2004, 308(3), 993–1001, DOI:10.1124/jpet.103.060673.
- J. M. Sforcin and V. Bankova, Propolis: Is There a Potential for the Development of New Drugs?, J. Ethnopharmacol., 2011, 133(2), 253–260, DOI:10.1016/j.jep.2010.10.032.
- G. Serreli and M. Deiana, In Vivo Formed Metabolites of Polyphenols and Their Biological Efficacy, Food Funct., 2019, 10(11), 6999–7021, 10.1039/c9fo01733j.
- D. P. Hayes, Adverse Effects of Nutritional Inadequacy and Excess: A Hormetic Model, Am. J. Clin. Nutr., 2008, 88(2), 578S–581S, DOI:10.1093/ajcn/88.2.578S.
- A. Murakami, Novel Mechanisms Underlying Bioactivities of Polyphenols via Hormesis, Curr. Opin. Toxicol., 2022, 30, 100337, DOI:10.1016/j.cotox.2022.02.010.
- E. J. Calabrese, Hormesis: Path and Progression to Significance, Int. J. Mol. Sci., 2018, 19(10), 2871, DOI:10.3390/ijms19102871.
- E. Pannucci, D. D'Eliseo, F. Ieri, A. Romani, L. Santi, R. Bernini, M. Sabatti and F. Velotti, Perspectives on Populus Spp. (Salicaceae) Bud Extracts as Antioxidant and Anti-Inflammatory Agents, Nat. Prod. Res., 2022, 36(6), 1648–1652, DOI:10.1080/14786419.2021.1896512.
- L. Pobłocka-Olech, I. Inkielewicz-Stepniak and M. Krauze-Baranowska, Anti-Inflammatory and Antioxidative Effects of the Buds from Different Species of Populus in Human Gingival Fibroblast Cells: Role of Bioflavanones, Phytomedicine, 2019, 56, 1–9, DOI:10.1016/j.phymed.2018.08.015.
- L. M. C. Simões, L. E. Gregório, A. A. Da Silva Filho, M. L. de Souza, A. E. C. S. Azzolini, J. K. Bastos and Y. M. Lucisano-Valim, Effect of Brazilian Green Propolis on the Production of Reactive Oxygen Species by Stimulated Neutrophils, J. Ethnopharmacol., 2004, 94(1), 59–65, DOI:10.1016/j.jep.2004.04.026.
- C. Russo, M. Piccioni, M. L. Lorenzini, C. Catalano, V. Ambrogi, R. Pagiotti and D. Pietrella, Bud-Poplar-Extract-Embedded Chitosan Films as Multifunctional Wound Healing Dressing, Molecules, 2022, 27(22), 7757, DOI:10.3390/molecules27227757.
- R. Akhigbe and A. Ajayi, The Impact of Reactive Oxygen Species in the Development of Cardiometabolic Disorders: A Review, Lipids Health Dis., 2021, 20(1), 23, DOI:10.1186/s12944-021-01435-7.
- D. Furman, J. Campisi, E. Verdin, P. Carrera-Bastos, S. Targ, C. Franceschi, L. Ferrucci, D. W. Gilroy, A. Fasano, G. W. Miller, A. H. Miller, A. Mantovani, C. M. Weyand, N. Barzilai, J. J. Goronzy, T. A. Rando, R. B. Effros, A. Lucia, N. Kleinstreuer and G. M. Slavich, Chronic Inflammation in the Etiology of Disease across the Life Span, Nat. Med., 2019, 25(12), 1822–1832, DOI:10.1038/s41591-019-0675-0.
- W. Kalt, A. Cassidy, L. R. Howard, R. Krikorian, A. J. Stull, F. Tremblay and R. Zamora-Ros, Recent Research on the Health Benefits of Blueberries and Their Anthocyanins, Adv. Nutr., 2020, 11(2), 224–236, DOI:10.1093/advances/nmz065.
- M. Acito, C. Fatigoni, M. Villarini and M. Moretti, Effect of Cooking and Domestic Storage on the Antioxidant Activity of Lenticchia Di Castelluccio Di Norcia, an Italian PGI Lentil Landrace, Int. J. Environ. Res. Public Health, 2023, 20(3), 2585, DOI:10.3390/ijerph20032585.
- W. J. Craig, A. R. Mangels, U. Fresán, K. Marsh, F. L. Miles, A. V. Saunders, E. H. Haddad, C. E. Heskey, P. Johnston, E. Larson-Meyer and M. Orlich, The Safe and Effective Use of Plant-Based Diets with Guidelines for Health Professionals, Nutrients, 2021, 13(11), 4144, DOI:10.3390/nu13114144.
Footnotes |
† Electronic supplementary information (ESI) available. See DOI: https://doi.org/10.1039/d3fo05059a |
‡ These authors share the first authorship. |
§ These authors share senior and last authorship. |
|
This journal is © The Royal Society of Chemistry 2024 |
Click here to see how this site uses Cookies. View our privacy policy here.