DOI:
10.1039/D3FO03770C
(Paper)
Food Funct., 2024,
15, 4010-4020
Cordyceps sinensis accelerates stem cell recruitment to human skeletal muscle after exercise†
Received
6th September 2023
, Accepted 11th February 2024
First published on 18th March 2024
Abstract
Cordyceps sinensis is a parasitic fungus known to induce immune responses. The impact of Cordyceps supplementation on stem cell homing and expansion to human skeletal muscle after exercise remains unexplored. In this study, we examined how pre-exercise Cordyceps supplementation influences cell infiltration, CD34+ cell recruitment, and Pax7+ cell expansion in human skeletal muscle after high-intensity interval exercise (HIIE) on a cycloergometer. A randomized, double-blind, placebo-controlled crossover study was conducted with 14 young adults (age: 24 ± 0.8 years). A placebo (1 g cornstarch) and Cordyceps (1 g Cordyceps sinensis) were administered before exercise (at 120% maximal aerobic power). Multiple biopsies were taken from the vastus lateralis for muscle tissue analysis before and after HIIE. This exercise regimen doubled the VEGF mRNA in the muscle at 3 h post-exercise (P = 0.006). A significant necrotic cell infiltration (+284%, P = 0.05) was observed 3 h after HIIE and resolved within 24 h. This response was substantially attenuated by Cordyceps supplementation. Moreover, we observed increases in CD34+ cells at 24 h post-exercise, notably accelerated by Cordyceps supplementation to 3 h (+51%, P = 0.002). This earlier response contributed to a four-fold expansion in Pax7+ cell count, as demonstrated by immunofluorescence double staining (CD34+/Pax7+) (P = 0.01). In conclusion, our results provide the first human evidence demonstrating the accelerated resolution of exercise-induced muscle damage by Cordyceps supplementation. This effect is associated with earlier stem cell recruitment into the damaged sites for muscle regeneration.
Introduction
Cordyceps sinensis is a parasitic fungus that can survive at high altitudes and is known to induce pro-inflammatory response in vivo1 and enhanced phagocytosis.2 However, Cordyceps sinensis supplementation extends longevity in mice by ∼10%.3 Pro-inflammatory preconditioning is a classic approach to enhance muscle adaptation in humans.4Cordyceps has been widely consumed by athletes and sport enthusiasts.5,6 It remains unknown whether pre-exercise Cordyceps supplementation influences recovery from muscle damage induced by high intensity exercise.
Muscle regeneration after injury requires inflammation.7 This wound healing program requires bone marrow stem cells to develop hematopoietic immune cells and specialized progenitors. Both types of cells work in concert to clear unhealthy cells and repopulate the damaged/senescent tissues into a relatively younger cell population,8 resulting in enhanced fitness of challenged tissues. CD34+ cells are a well-characterized population of bone marrow-derived stem cells that have traditionally been used for cell regeneration in clinical practice.9,10
In response to exercise, circulating CD34+ cells transiently increase and then quickly decrease.11 A significant increase in CD34+ cells in human skeletal muscle is observed 24 h after resistance exercise, suggesting bone marrow stem cell homing and expansion in challenged tissues.12 In mice, CD34+ bone marrow stem cell transplantation increased Pax7+ satellite cells in skeletal muscle, demonstrating the hematopoietic contribution to myogenesis.13 Currently, no study has directly validated the contribution of CD34+ cells to Pax7+ cells in injured human skeletal muscle. In this study, we hypothesized that CD34+ cells contribute to Pax7+ satellite cell expansion in damaged muscle tissues following high intensity exercise.
Pax7+ satellite cells are located between the sarcolemma and basal lamina for nucleus donation to regenerating myofibers of muscle tissues.14 In this study, we examined dynamical changes of CD34+/Pax7+ cells in human skeletal muscle during a 24 h recovery period following HIIE. Furthermore, the effect of Cordyceps supplementation on this exercise response was also examined.
Materials and methods
Cordyceps supplementation
In the study, Cordyceps sinensis mycelium (Paecilomyces hepiali, TCM777, LivPhcD, TCM Biotech International Corp., Taipei, Taiwan), produced by a GMP manufacturer, was used for pre-exercise consumption. The dosage comprised a total of 3.5 mg of adenosine and 40 mg of polysaccharide. The adenosine content, a recognized active ingredient, may vary depending on the cultured environment. Therefore, the product is standardized based on the adenosine content using HPLC. Polysaccharides, specifically 1,3
:
1,6-β-glucan, present in yeast and mushrooms, are indicators for mushroom and yeast products.
Participants
We recruited sedentary young men (aged 23–30 years) with the body mass index (BMI) ranging from 18–25 kg m−2. A total of 20 candidates were initially enrolled. Six participants dropped out due to time conflict. The remaining 14 participants completed the entire counter-balanced crossover trials. Inclusion criteria were sedentary young men (exercise < 2 times a week) aged between 20 and 30 years. Exclusion criteria were smoking, inflammatory conditions, metabolic conditions, and exercise training. Participants were informed to avoid exercise or receive medical/nutraceutical supplements before HIIE. All participants provided written informed consent before study participation. The experimental protocol was conducted in compliance with the guidelines of the Declaration of Helsinki. Institutional ethical approval was obtained from the Institutional Review Board of University of Taipei with the number IRB-2020-050.
Study design
To assess the effect of pre-exercise Cordyceps sinensis supplementation on muscle recovery after HIIE, a randomized placebo-controlled counterbalanced crossover trial was performed. The participants were required to visit the laboratory on seven occasions. A familiarization to the VO2max test and the experimental protocol in the lab was performed on the first visit. The body composition was measured by dual-energy X-ray absorptiometry (DXA) (Lunar iDXA; GE Medical Systems, Madison, Wisconsin, USA) using Encore software V.13.60.033 (Encore, Madison, Wisconsin, USA) to obtain the baseline characteristics of participants (Table 1).
Table 1 Subject characteristics
N = 14 |
Mean ± SE |
Age (y) |
24 ± 0.8 |
Height (cm) |
172 ± 1.8 |
Weight (kg) |
68 ± 1.7 |
BMI (kg m−2) |
23 ± 0.6 |
VO2max (mL min−1 kg−1) |
42 ± 1.1 |
Bone (kg) |
2.7 ± 0.07 |
Bone to weight ratio (%) |
4.0 ± 0.09 |
Muscle mass (kg) |
51 ± 1.3 |
During the second visit, the maximal aerobic power (cycling power at VO2max) of all participants was assessed 2 days after the familiarization. A baseline muscle biopsy was conducted at rest two weeks after the second visit. Participants ingested either Cordyceps or placebo capsules (1 g in total), 11 h (0.5 g) and 1 h (0.5 g) before HIIE. Washout period of the crossover trial was 3 weeks. To avoid the potential nutritional effect on the assessed outcomes, a 250 kcal isocaloric standard diet (Ensure; Abbott 58 Nutrition, Taipei, Taiwan) was provided 12 h and 2 h prior to the challenge and 12 h before the last muscle biopsy (24 h post-HIIE). Participants were asked to maintain their normal diet and were allowed drinking water ad libitum for the rest of the time. The daily energy intake of participants was 1760 kcal (carbohydrate 44%, fat 39%, protein 17%) during the placebo trial and 1714 kcal (carbohydrate 45%, fat 38%, protein 17%) during the Cordyceps trial, according to a 3-day dietary recall survey by a registered dietitian. Muscle samples from vastus lateralis were collected by needle biopsy 3 h on one leg and 24 h on contra-lateral leg following HIIE. The collected biopsied muscles were used for quantitative PCR analysis (VEGF and CD34 mRNA) and immunofluorescence double staining.
Maximal aerobic power (Wmax)
An incremental exercise protocol was performed in the daytime on a cycle ergometer (Monark LC6, Stockholm, Sweden). To obtain reliable Wmax at VO2max, participants were familiarized with the cycle ergometer, the Borg scale 0–10, and the technical details of the testing. The seat height was adjusted to align with the participants’ preferred knee joint flexion when the pedals were in the bottom position. Additionally, the handlebar height was adjusted to accommodate the trunk and arms. The individual setup recorded during the familiarization phase was replicated in the experimental session to ensure consistency in positioning. The Cortex gas analyser system (Metalyzer, Leipzig, Germany) was connected to a mask to collect and analyse the participant's expired air. Gas values (O2 and CO2) were recorded each second by using the gas analyser system using MetaSoft Studio software. Heart rate signals were transferred wirelessly using Polar H10 (Polar, Kuala Lumpur, Malaysia) synchronized with the gas analyser. The gas analyser was calibrated daily during the exercise session. The maximal aerobic power (Wmax) at VO2max was established before conducting HIIE at 120% Wmax. The VO2max protocol was started with warming up for 3 min at 50 watts, followed by resting for 3 min. The incremental exercise protocol commenced at 125 W and was increased by 25 W every 2.5-minute interval until the participant reached exhaustion. Participants were instructed to maintain a pedal cadence of 60 to 70 rpm. To determine the VO2max value, participants needed to meet at least two of the following four criteria: (1) respiratory exchange ratio ≥ 1.2, (2) heart rate ≥ 95% of the age-estimated maximal heart rate for >30 seconds, (3) rated perceived exertion (RPE) on the Borg scale reaching 9–10, and (4) inability to sustain a cadence of 60 rpm for more than 10 seconds. The VO2max was determined by calculating the mean value of the final 30 seconds of oxygen uptake, while the Wmax was the maximum work rate achieved in the final stage.15
High-intensity interval exercise (HIIE)
Participants performed HIIE in the morning (around 10:30 am). The HIIE protocol utilized a cycling exercise procedure based on a previously established method.8 This involved exercising at an intensity of 120% of Wmax, maintaining a pedal cadence of 90 rpm, for 20 s, followed by a 20 s rest interval. This pattern was repeated for a total of 15 sets. The HIIE protocol was programmed using the Monark Test Software (Ver. 1.1.9.0) and conducted on the same Monark LC6 cycloergometer for VO2max determination. Prior to the HIIE session, each participant commenced with a 4 min warm-up phase at a comfortable load of 50 W, followed by a 3 min rest period. During the HIIE, participants were instructed to increase their cadence within 3–5 s to reach a target of 90 rpm at the conclusion of each rest phase. The total work achieved during the exercise from both the placebo and Cordyceps trials (placebo: 19
779 kJ; Cordyceps: 19
624 kJ) was similar across all participants.
Muscle biopsy
The muscle biopsy procedure followed the methodology outlined in a previous study.8 A qualified physician performed the muscle biopsy using an 18-G Temno disposable cutting needle (Cardinal Health, McGaw Park, Illinois, USA) under the administration of local anaesthesia (2% lidocaine). Muscle tissue samples were obtained from the vastus lateralis, located at a depth of 3 cm and 20 cm proximal to the kneecap. Three weeks following the baseline muscle biopsy, two consecutive muscle biopsies for tissue collection were performed 3 h and 24 h following HIIE from the contralateral leg at the same position during each trial. Muscle samples were immediately placed into a microtube containing 4% of paraformaldehyde (PFA) solution for immunofluorescence and hematoxylin and eosin (HE) staining.
Hematoxylin and eosin (HE) staining
Muscle necrosis and centrally nucleated myofibers of muscle cross-section were assessed by HE stains according to the previous protocol.8 A histological procedure was conducted by professional pathologists from Toson Technology Corporation (Zhubei City, Hsinchu, Taiwan). Formalin-fixed paraffin embedded muscle tissues were sliced 2–3 μm thick, deparaffinized in xylene followed by rehydrating in a graded alcohol series using ethanol (99.9%, 95%, 85% and 75%) each for 2 min, and stained with hematoxylin (for nuclei) for 3 min (BioTnA Inc., TA01HE, Kaohsiung, Taiwan). The tissue sections were stained using eosin (for the cytoplasm) for 15 s and dehydrated in ethanol after being washed in distilled water.
Immunofluorescence staining for CD34+ and Pax7+cells
Double staining was performed to localize CD34+ cells and Pax7+ cells on a muscle cross-section for each participant. Glass slides were first incubated with primary antibodies against Pax7 and CD34 protein antigens. The primary antibody against CD34 was rabbit anti-CD34 (human) (EP373Y) (ab81289, 1
:
200, Abcam, Cambridge, UK). The secondary antibody used was IgG (H + L)-488 goat anti-rabbit (TAFB01-F, Biotna, Kaohsiung, Taiwan), which reflects bright green fluorescence. The primary antibody against Pax7 was mouse anti-Pax7 (human) (clone 2F12H4) (MABD20, 1
:
200, Merck, Darmstadt, Germany). The secondary antibody was IgG (H + L)-594 goat anti-mouse (TAAB01-T, Biotna, Kaohsiung, Taiwan) which reflects red fluorescence. Nuclei were labelled with DAPI (4′,6-diamindino-2-phenylindole) (TA01DP, Biotna, Kaohsiung, Taiwan) after incubation (5–10 min) in the dark at room temperature, followed by 4 times rinsing in PBS buffer.
Imaging and quantification
To obtain unbiased results, HE staining and immunofluorescence staining of muscle tissues were analysed by two independent assessors according to the same counting criteria. Results were accepted only when values from both assessors achieved the intraclass correlation coefficient (ICC) > 0.7.
HE staining was analysed using Motic Digital Slide Assisstant System Lite 1.0 (Motic Hong Kong Limited, Hong Kong, China) at ×40. The criterion to identify the necrotic area included shrinking myofibers (disintegrated membrane) associated with cell aggregation > 7 nuclei in the wide spaces between the myofibers of muscle cross-sections. The nuclei located in the central myofiber (centronucleation) was a marker for damage-induced myofiber regeneration. For immunofluorescence, representative images were captured at ×20 magnification using an OLYMPUS OlyVIA 3.21. (OLYMPUS, Tokyo, Japan) with a scale bar ranging 200–500 μm. To avoid the effect of colouring background, the brightest slide was manually set. The captured images were analyzed using ImageJ (National Institute of Health, USA) to determine the positive area. The positive markers of CD34+ (green), Pax7+ (red), and CD34+/Pax7+ cells (yellow) were set at a minimal size of 0.8 μm2 based on the smallest diameter of detectable Pax7+ cells (1 μm).16
Real-time polymerase chain reaction (RT-PCR)
The current study used the RNeasy® Fibrous Tissue Mini Kit (QIAGEN, Hilden, North Rhine-Westphalia, Germany) to extract total RNA from the biopsied muscle. Approximately, 5 mg of muscle tissue was homogenized using 300 μl of buffer RLT with a POLYTRON® system (PT 3100 D, KINEMATICA AG, Malters, Switzerland) on ice. The buffer RLT contained guanidine thiocyanate, which inhibited the activity of endogenous ribonucleases. After incubating the lysate with RNase-free water (590 μl) and proteinase K solution (10 μl) at 55 °C for 10 min to digest proteins and release nucleic acids, RNA was isolated by binding to the silica membrane of the RNeasy Mini spin column. The addition of 450 μl of 99.5% ethanol and centrifugation facilitated RNA separation. DNase treatment at room temperature for 15 min on the silica membrane eliminated DNA contaminants. The DNase and contaminants were removed by using RPE buffer, and RNA was eluted by collecting in the tubes with 30 μl of RNase-free water.
The extracted RNA was reverse transcribed into cDNA using the iScript™ cDNA Synthesis Kit (Bio-Rad, Hercules, California, USA) following the manufacturer's instructions. The reaction mixtures for reverse transcription were incubated with an initial priming step at 25 °C for 5 min, followed by reverse transcription at 46 °C for 20 min. The PCR samples were subjected to a melting curve analysis. Inactivation was carried out at 95 °C for 1 min, followed by holding at 12 °C.
All reverse-transcribed cDNA was amplified by performing RT-PCR according to the manufacturer's instructions. All samples were measured in duplicate. The reaction mixture contained the iTaq Universal Probes Supermix (Bio-Rad, Hercules, California, USA) (1–2 μl), specific primers and probes. The primers and probes used to amplify the target were supplied from the Bio-Rad PrimePCR™ probe assay according to the unique assay ID as follows: VEGF, qHsaCEP0051468; CD34, qHsaCIP0026476; Pax7, qHsaCIP0030375; and internal standard RPP30, qHsaCEP0052683. Amplification was carried out on a CFX96 Touch Real-Time PCR Detection System (Bio-Rad, Hercules, California, USA) following the standard protocol, which involved polymerase activation and DNA denaturation at 95 °C for 30 s, followed by 40 amplification cycles at 95 °C for 5 s and 55 °C for 30 s. Gene expression, normalized to the geometric mean of a housekeeping gene (RPP30), was quantified using the 2−ΔΔCt method and expressed as fold difference relative to RPP30.
Statistical analysis
Statistical analysis was performed using SPSS 27.0 (IBM, NY, USA). A paired t-test was used to compare the difference between baseline and post-exercise values. Treatment difference between the placebo and Cordyceps trials was also compared by a paired t-test. Data are presented as mean ± standard error (SE). Type 1 error of P ≤ 0.05 was considered significant and P < 0.1 was considered moderately significant. Cohen's d indicated the effect size. The value of d was classified into small effect (<0.5), medium effect (0.5–0.7), and large effect (≥0.8).
Results
HIIE-induced VEGF expression returned to the baseline in 24 h
Effects of HIIE on VEGF mRNA in skeletal muscle under placebo- and Cordyceps-supplemented conditions are shown in Fig. 1A. HIIE doubled VEGF mRNA levels in skeletal muscle in 3 h to a similar level for the placebo (+117%, P = 0.006, d = 1.2) and Cordyceps-supplemented conditions (+108%, P = 0.02, d = 0.9). VEGF mRNA returned to the baseline in 24 h. HIIE induced CD34 mRNA (Fig. 1B) in skeletal muscle at 24 h of recovery (+78%, P = 0.05). No change in CD34 mRNA was observed in the Cordyceps-supplemented condition.
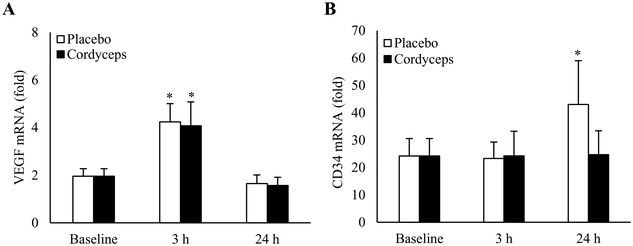 |
| Fig. 1 PCR analysis for human skeletal muscle after high-intensity interval exercise (HIIE). The increases in VEGF mRNA returned to baseline 24 h after HIIE (A). The responses for the placebo- and Cordyceps-supplemented conditions were similar. CD34 mRNA in human skeletal muscle increased 24 h after HIIE and this response was absent after Cordyceps supplementation (B). *P ≤ 0.05 compared to pre-exercise baseline. Data are presented as mean ± SE. Value expresses as fold difference relative to RPP30 (internal standard). | |
Cordyceps supplementation attenuated HIIE-induced cell infiltration
Cell infiltration in the necrotic area is indicated on the HE stains, as shown in Fig. 2. HIIE-induced necrosis (+284%) was observed at 3 h of recovery period (P = 0.04). This muscle damage response was resolved in 24 h. Under the Cordyceps-supplemented condition, this HIIE-induced damage response was minimal. Centronucleation is a conventional marker of damage-induced myofiber regeneration (Fig. 3). The pattern of this response after HIIE is similar to necrotic cell infiltration, but was only moderately significant. The myofiber density in the muscle tissues was 78 myofibers per mm2.
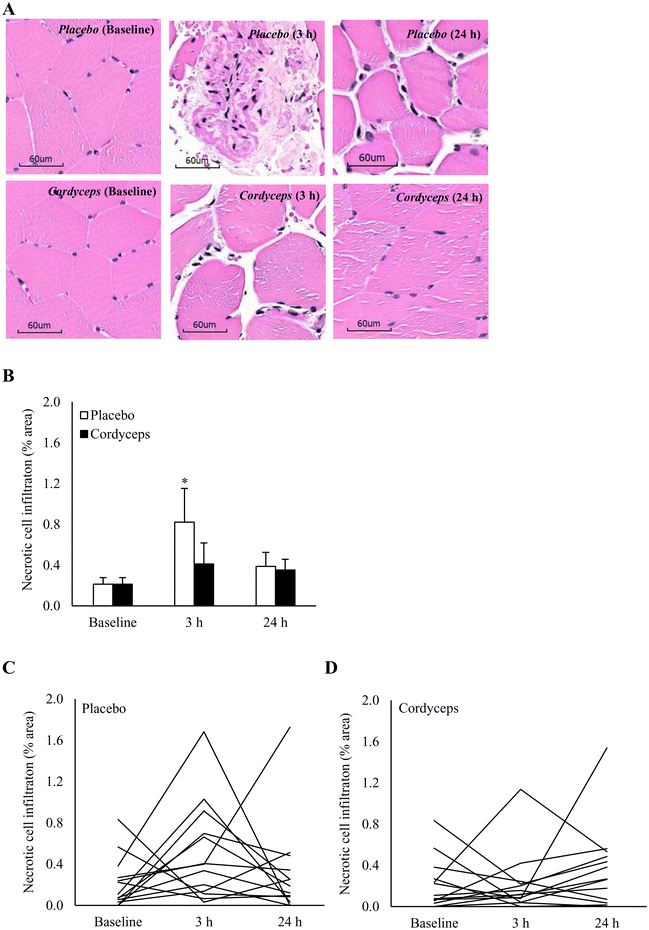 |
| Fig. 2 Effect of pre-exercise Cordyceps supplementation on muscle necrosis after high-intensity interval exercise (HIIE). Representative HE stains images of necrotic cell infiltration in human vastus lateralis (A). HIIE increased the necrotic area with increased cell infiltration, which was attenuated by Cordyceps supplementation (B). Individual response to HIIE in the placebo-supplemented (C) and Cordyceps-supplemented (D) conditions. *P ≤ 0.05 compared to pre-exercise baseline. Data are presented as mean ± SE. Scale bars = 60 μm. | |
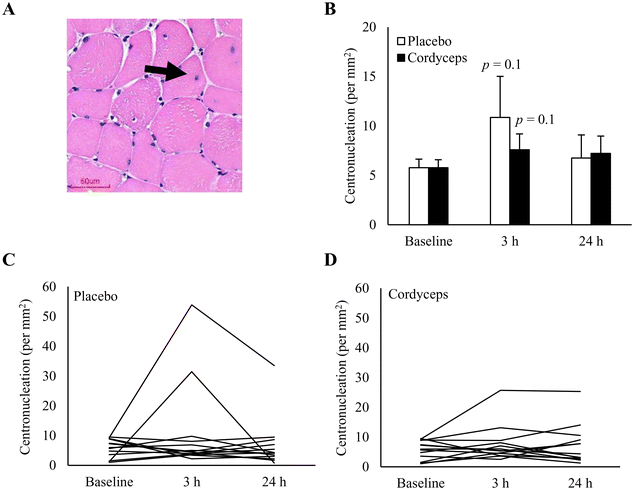 |
| Fig. 3 Effect of pre-exercise Cordyceps supplementation on centrally nucleated myofibers after high-intensity interval exercise (HIIE). A representative HE staining image indicating centrally nucleated myofibers in human vastus lateralis (A). HIIE moderately increased centronucleation at 3 h of recovery (P = 0.1) (B). Individual responses to HIIE in the placebo-supplemented (C) and Cordyceps-supplemented (D) conditions. Data are presented as mean ± SE. Scale bars = 60 μm. | |
CD34+ cell homing in the necrotic area of human skeletal muscle
To further characterize specific types of infiltrated cells in the necrotic area of muscle tissues, we conducted immunofluorescence analysis using muscle cross-sections (Fig. 4), which indicated highly concentrated CD34+ cells localized in the necrotic area and contributed to Pax7+ cells, demonstrated by double staining of CD34+/Pax7+ cells. The average sizes of CD34+ and Pax7+ cells were 13.5 (6.0–75.8) and 8.8 (6.0–266.1) μm, respectively. Representative immunofluorescence stain images of CD34+ cells (green), Pax7+ cells (red), and CD34+/Pax7+ cells (merge, yellow) in the muscle sample are shown in Fig. 4A for non-necrotic areas and Fig. 4B for necrotic areas. Immunofluorescence images indicate that CD34+ cells were highly concentrated in the necrotic areas of human skeletal muscle, regardless of exercise status.
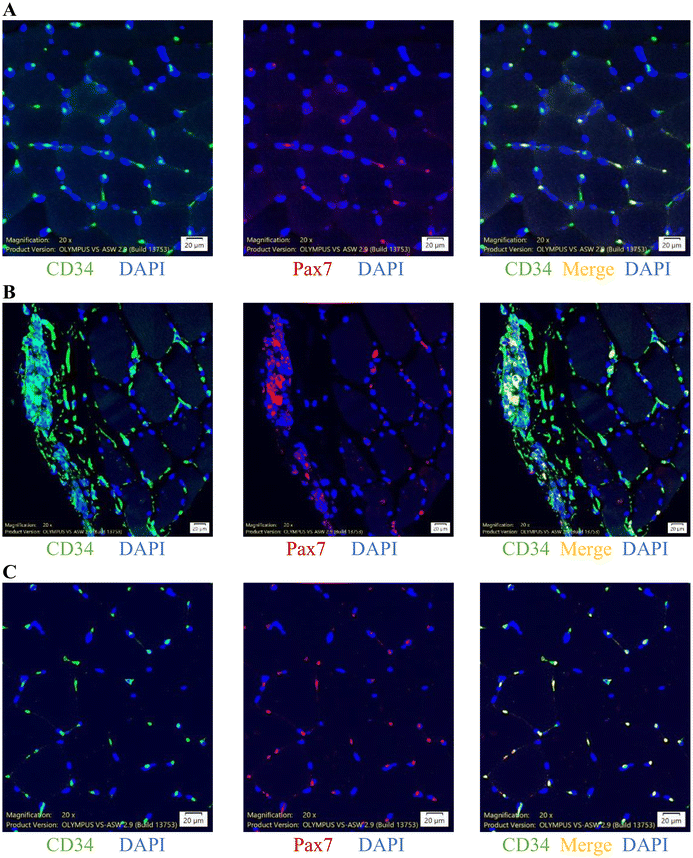 |
| Fig. 4 Immunofluorescence co-staining for stem cells (CD34+/Pax7+) in human vastus lateralis. Representative immunofluorescence stain images localize cells expressing antigens against CD34 antibodies (green), Pax7 antibodies (red), and merge (yellow) in the muscle cross-sections. Pre-exercise muscles are illustrated in (A). Following high intensity interval exercise, stem cells (CD34+, Pax7+ and CD34+/Pax7+) were increased and particularly concentrated in the necrotic area of muscle at 3 h of recovery (B). This response returned to pre-exercise baseline in 24 h (C). Scale bars = 20 μm. | |
Cordyceps accelerated recruitment of CD34+/Pax7+ cells to exercised muscle
HIIE induced a significant increase in CD34+ cells in skeletal muscle by 38% at 24 h of recovery period (Fig. 5A) (P = 0.04, d = 0.6). This effect occurred faster after pre-exercise Cordyceps supplementation, leading to an earlier increase of 51% at 3 h of recovery period (P = 0.002, d = 0.9). The HIIE-induced change was accompanied by protracted increases in Pax7+ cells from 3 h to 24 h after HIIE (P = 0.05, d = 0.7). Cordyceps supplementation resulted in a more pronounced response on Pax7+ cell expansion both at 3 h (P = 0.04, d = 0.7) and 24 h post-exercise (P = 0.03, d = 0.7), with a moderate difference between treatments (P = 0.1, d = 0.5) (Fig. 5B).
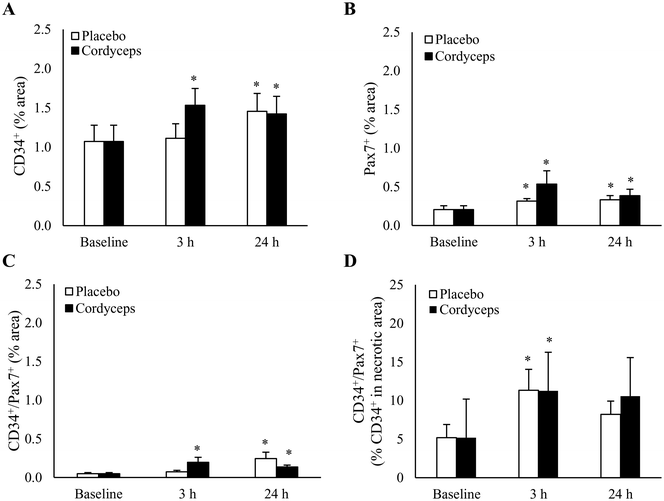 |
| Fig. 5 Semi-quantitative analysis of the pre-exercise Cordyceps supplementation effect on stem cells in human skeletal muscle after high-intensity interval exercise (HIIE). Cordyceps supplementation accelerated early CD34+ cell responses at 3 h post-exercise (A). Pax7+ cell expansion post-HIIE significantly improved with Cordyceps supplementation (B). Cordyceps supplementation advanced a fourfold increase in CD34+/Pax7+ cells at 3 h, usually observed at 24 h after HIIE (C). Immunofluorescence staining suggests a similar Cordyceps-induced response of CD34+/Pax7+ cells in the necrotic area to that of the placebo-supplemented trial regarding total CD34+ cell contribution to Pax7+ cells (D). *P ≤ 0.05 compared to pre-exercise baseline. Data are presented as mean ± SE. | |
To further determine the contribution of CD34+ cells to Pax7+ cells in exercised skeletal muscle, immunofluorescence double staining was performed (Fig. 5C). A 4-fold increase in CD34+/Pax7+ cells in the entire muscle cross-section was observed 24 h after HIIE (P = 0.02, d = 0.9), and this increase was accelerated by Cordyceps supplementation to a similar level at 3 h post-exercise (P = 0.01, d = 0.9). CD34+ cells were much more concentrated in the necrotic region of myofibers. In the necrotic region (Fig. 5D), HIIE increased the contribution of CD34+ cells to Pax7+ cells from 5% to 11% (P = 0.01, d = 0.8), and returned to the pre-exercise baseline.
Discussion
High intensity exercise causes muscle damage and induces cell infiltration in human skeletal muscle.8 In this study, we asked the question whether the infiltrated cells in the necrotic sites of exercised human skeletal muscle are associated with CD34+ cell homing. In addition, we measured the contribution of CD34+ cells to Pax7+ satellite cells after HIIE by immunofluorescence co-staining. The effect of Cordyceps supplementation on this damage response was also assessed after HIIE. The major findings of the study are: (1) CD34+ cells are highly concentrated in the necrotic area of human skeletal muscle and contributed to Pax7+ satellite cells; (2) pre-exercise Cordyceps supplementation accelerated HIIE-induced CD34+ cell infiltration in skeletal muscle; (3) CD34+/Pax7+ cells in skeletal muscle increased by ∼4-fold 24 h after HIIE and this response was accelerated to occur in 3 h post-exercise by Cordyceps supplementation; (4) pre-exercise Cordyceps supplementation accelerated resolution of HIIE-induced necrosis in human skeletal muscle.
Effects of Cordyceps supplementation in exercise-induced muscle inflammation have not previously been reported. The results of the study provide the first human evidence which demonstrates an accelerated resolution of exercise-induced muscle inflammation by pre-exercise Cordyceps supplementation. Cell infiltration in the necrotic area of human skeletal muscle indicated by HE stains was expanded by nearly 4-fold 3 h after the HIIE protocol from the pre-exercise baseline. Inflammation is known as an immune response essential for muscle regeneration after damage.7,17 The fungus Cordyceps sinensis has been reported to increase phagocytosis of human monocytes,2 the first phase of the inflammation program before entering the regenerative phase to heal injured skeletal muscle.7 Thus, the early resolution of muscle necrosis by pre-exercise Cordyceps supplementation may have been associated with potentiation of phagocytosis before and during HIIE. The results of the study provide a scientific basis to support a preconditioning strategy of using a pro-inflammatory stimulus to accelerate the resolution of muscle inflammation following high intensity exercise.
To further characterize the specific type of infiltrated cells in human skeletal muscle, we identified that CD34+ cells are highly concentrated in the necrotic area (disrupted myofibers) of human skeletal muscle. This result indicates that HIIE directs CD34+ bone marrow stem cell homing to damaged sites of human muscles. In mice, bone marrow stem cell transplantation regenerates skeletal muscle after an irradiation-induced injury.18 Increasing VEGF expression in tissues seems to require the recruitment of CD34+ bone marrow stem cells into injured tissues from circulation.19–22 In this study, we confirmed increased VEGF expression 3 h after HIIE concurrent with CD34+ cell infiltration in human skeletal muscle. Taken together, the results of the study suggest the role of CD34+ bone marrow stem cells in exercise-induced muscle regeneration in humans.
In this study, increased CD34 mRNA in human skeletal muscle was observed 24 h following HIIE. This change may be important for developing myogenic phenotype of infiltrated stem cells. This is suggested by a defective muscle regeneration after muscle injury in CD34-deficient mice.23 In this study, we do not observe significant responses in CD34 expression 3 h and 24 h after exercise in the Cordyceps-supplemented trial. This may have been associated with the faster resolution of muscle inflammation. However, due to limited time points of the muscle samples, we could not rule out the possibility of an earlier response of CD34 expression in skeletal muscle between 3 h and 24 h of recovery time under the Cordyceps-supplemented condition.
Evidence for Pax7+ satellite cells developed from CD34+ bone marrow stem cells was mostly reported in vitro and in rodents.13,24 In this study, we provided the first human evidence to validate the putative bone-to-muscle development, the increases of CD34+/Pax7+ cells in the damaged area of muscle tissues. CD34+ bone marrow stem cells contributed to ∼11% of Pax7+ satellite cells in the necrotic area of the exercised muscle. Pax7+ satellite cells are well-known as the major type of muscle stem cell to regenerate myofiber by fast nuclear donation into the cytoplasmic space. Mobilization of undifferentiated bone marrow stem cells is required to support muscle regeneration, since the amount of myogenic precursor exceeds resident satellite cells.25 Exercise can transiently deplete Pax7+ satellite cells and rapidly replenish them within 3 h after exercise, a process unlikely to rely on cell proliferation within such a short period.26 The result of the study further explains the requirement of CD34+ bone marrow cell homing to replenish Pax7+ cell pool in skeletal muscle. Pax7+ satellite cells were doubled after HIIE over the course of a 24 h recovery period, suggesting a further satellite cell expansion after the early CD34+ cell homing during recovery.27
Pre-exercise Cordyceps supplementation accelerated recruitment of CD34+ bone marrow stem cells to the exercised human skeletal muscle 3 h post-exercise. This increase was followed by a sustained Pax7+ cell expansion in the same muscle during 3–24 h post-exercise. This result strengthens the previous report indicating the stimulatory effect of Cordyceps on both stem cell proliferation and differentiation.28 This effect seems to associate with the pro-inflammatory properties of Cordyceps. CD34+ bone marrow stem cell homing to injured tissues is known to involve with pro-inflammatory cytokine release during the early phagocytic phase of inflammation.29 Taken together, CD34+ cell recruitment to injured sites plays a crucial role in regenerating human skeletal muscle following HIIE-induced muscle inflammation.30 This tissue remodelling can be enhanced by pre-conditioning with Cordyceps supplementation to explain the early resolution of HIIE-induced muscle inflammation.
Limitations
It is worthy to note that CD34+ cells contributed to ∼11% of Pax7+ cells in the necrotic area of human skeletal muscle. CD34+ bone marrow stem cells are multipotent which can develop into other progenitors, such as vascular endothelial cells, neural stem cells, adipocytes, smooth muscle, and fibroblasts.31,32 Future studies are needed to elucidate relative contributions of CD34+ cells to develop specific cell types in human muscle tissues and the mediators involved in this cell-type specific regulation.33 Furthermore, the results of the study on young participants present a limitation for knowledge generalization to older population. Higher age is generally associated with greater levels of body weight (high cell population) and basal inflammation. Growth in weight is associated with progressive decreases in bone-to-body mass ratio, which may contribute to relative inadequacy of bone marrow cell production to regenerate cell in tissues.34 Elevated basal inflammation typically witnessed in overweight individuals can increase background demand for CD34+ cells to renew peripheral tissues of individuals with higher cell population. Therefore, it is hard to predict whether pre-exercise Cordyceps supplementation can also accelerate resolution of muscle inflammation for elderly, particularly those with heavier weight.
The Cordyceps used in this study contain adenosine and polysaccharides. It is possible that the observed acceleration in CD34+ cell homing and Pax7+ cell expansion is due to a synergistic effect of both components. Adenosine, known for its potent impact on the innate immune response,35 is expected to modify the time required to resolve inflammation post-exercise. We cannot eliminate the potential influence of polysaccharides on the observed exercise response in human skeletal muscle. Previous reports indicate an increase in polysaccharide concentration in the blood due to gut leakage after high-intensity exercise,36 potentially bolstering the immune response in skeletal muscle. However, further studies are necessary to confirm this hypothesis. Furthermore, the effect of adenosine on the protein kinases/phosphatase involving muscle adaptation to exercise requires more investigations from animal and cell studies to provide deeper insight into the observed effect related to muscle stem cell homing and expansion.37
Conclusions
The results of the study demonstrate the contributions of CD34+ bone marrow stem cells to Pax7+ satellite cells in damaged human skeletal muscle after high intensity exercise. In this study, we have further shown a substantially shortened period of muscle inflammation after exercise concurrent with accelerated increases in CD34+/Pax7+ cells in muscle tissues after Cordyceps supplementation. These findings suggest that the pro-inflammatory fungal-based supplement may potentiate immune response to protect muscle against damage induced by exercise.
Abbreviations
BMI | Body mass index |
CD34 | Cluster of differentiation 34 |
DAPI | 4′,6-Diamidino-2-phenylindole |
HIIE | High intensity intermittent exercise |
ICC | Intraclass correlation coefficient |
Pax7 | Paired box 7 |
PFA | Paraformaldehyde |
RPE | Rated perceived exertion |
rpm | Revolution per minute |
RT-PCR | Real-time reverse transcription–polymerase chain reaction |
SE | Standard error |
VEGF | Vascular endothelial growth factor |
W
max
| Maximum work rate |
Author contributions
CHK, CYH, and LKC contributed to the conception and design of this study; LD, YCL, and WHJ contributed to the main part of the experiment; LD, YCL, and LFL participated in the in vivo experiment; LD, YCL, LFL, and AN performed the tissue analysis; CHK, LD, YCL, LFL, and AN analysed the data; LD, CYH, CHK, CYH, and LKC contributed to drafting the paper and revising it strictly for vital intellectual content. All data were generated in-house, and no paper mill was used. All authors agree to be accountable for all aspects of work ensuring integrity and accuracy.
Data availability
The dataset used and/or analysed is available in the supplementary file.
Conflicts of interest
The Cordyceps used in the study is a fermented material supplied by TCM Biotech Taiwan and WynHealth Consultants, USA.
Acknowledgements
This study was funded by the National Science and Technology Council of Taiwan (Grant Number 109-2622-H-845-005) jointly with TCM Biotech Taiwan and WynHealth Consultants, USA.
References
- S. Ka Wai Lee, C. Kwok Wong, S. Kai Kong, K. Nam Leung and C. Wai Kei Lam, Immunomodulatory activities of HERBSnSENSES Cordyceps – in vitro and in vivo studies, Immunopharmacol. Immunotoxicol., 2006, 28, 341–360 CrossRef PubMed.
- C. F. Kuo, C. C. Chen, C. F. Lin, M. S. Jan, R. Y. Huang, Y. H. Luo, W. J. Chuang, C. C. Sheu and Y. S. Lin, Abrogation of streptococcal pyrogenic exotoxin B-mediated suppression of phagocytosis in U937 cells by Cordyceps sinensis mycelium via production of cytokines, Food Chem. Toxicol., 2007, 45, 278–285 CrossRef CAS PubMed.
- N. Z. Tan, J. L. Barger, Y. Zhang, S. B. Ferguson, Z.-M. Wu, T. A. Prolla, M. Bartlett and J.-S. Zhu, The lifespan-extending effect of Cordyceps sinensis Cs-4 in normal mice and its molecular mechanisms, FASEB J., 2011, 25, 599 Search PubMed.
- A. Franz, M. Behringer, J. F. Harmsen, C. Mayer, R. Krauspe, C. Zilkens and M. Schumann, Ischemic preconditioning blunts muscle damage responses induced by eccentric exercise, Med. Sci. Sports Exercise, 2018, 50, 109–115 CrossRef PubMed.
- C. Gupta, D. Prakash and S. Gupta, Nutraceuticals for athletes, Adv. Food Technol. Nutr. Sci. Open J., 2016, 2, 73–82 CrossRef CAS.
-
T. Smith, F. Majid, V. Eckl and C. M. Reynolds, Herbal supplement sales in US increase by record-breaking 17.3% in 2020, 2021.
- J. G. Tidball, Regulation of muscle growth and regeneration by the immune system, Nat. Rev. Immunol., 2017, 17, 165–178 CrossRef CAS PubMed.
- W. H. Jean, Y. W. Hsieh, L. F. Lai, L. Dewi, Y. C. Liao, M. Ye, S. H. Yu, C. L. Kao, C. Y. Huang and C. H. Kuo, Senolytic effect of high intensity interval exercise on human skeletal muscle, Aging, 2023, 15, 765–776 CrossRef CAS PubMed.
- V. Schächinger, B. Assmus, M. B. Britten, J. Honold, R. Lehmann, C. Teupe, N. D. Abolmaali, T. J. Vogl, W. K. Hofmann, H. Martin, S. Dimmeler and A. M. Zeiher, Transplantation of progenitor cells and regeneration enhancement in acute myocardial infarction: final one-year results of the TOPCARE-AMI Trial, J. Am. Coll. Cardiol., 2004, 44, 1690–1699 CrossRef PubMed.
- B. E. Strauer, M. Brehm, T. Zeus, M. Köstering, A. Hernandez, R. V. Sorg, G. Kögler and P. Wernet, Repair of infarcted myocardium by autologous intracoronary mononuclear bone marrow cell transplantation in humans, Circulation, 2002, 106, 1913–1918 CrossRef PubMed.
- L. Dewi, Y.-C. Lin, A. Nicholls, G. Condello, C.-Y. Huang and C.-H. Kuo, Pax7 + satellite cells in human skeletal muscle after exercise: a systematic review and meta-analysis, Sports Med., 2023, 53, 457–480 CrossRef PubMed.
- T. X. Y. Lee, J. Wu, W. H. Jean, G. Condello, A. Alkhatib, C. C. Hsieh, Y. W. Hsieh, C. Y. Huang and C. H. Kuo, Reduced stem cell aging in exercised human skeletal muscle is enhanced by ginsenoside Rg1, Aging, 2021, 13, 16567–16576 CrossRef CAS PubMed.
- P. A. Dreyfus, F. Chretien, B. Chazaud, Y. Kirova, P. Caramelle, L. Garcia, G. Butler-Browne and R. K. Gherardi, Adult bone marrow-derived stem cells in muscle connective tissue and satellite cell niches, Am. J. Pathol., 2004, 164, 773–779 CrossRef PubMed.
- A. Mauro, Satellite cell of skeletal muscle fibers, J. Biophys. Biochem. Cytol., 1961, 9, 493–495 CrossRef CAS PubMed.
- J. D. Trinity, J. F. Lee, M. D. Pahnke, K. C. Beck and E. F. Coyle, Attenuated relationship between cardiac output and oxygen uptake during high-intensity exercise, Acta Physiol., 2012, 204, 362–370 CrossRef CAS PubMed.
- C. L. Chen, H. Zhang, Q. Ye, W. Y. Hsieh, T. K. Hitchens, H. H. Shen, L. Liu, Y. J. Wu, L. M. Foley, S. J. Wang and C. Ho, A new nano-sized iron oxide particle with high sensitivity for cellular magnetic resonance imaging, Mol. Imaging Biol., 2011, 13, 825–839 CrossRef PubMed.
- J. G. Tidball, Inflammatory processes in muscle injury and repair, Am. J. Physiol.: Regul., Integr. Comp. Physiol., 2005, 288, R345–R353 CrossRef CAS PubMed.
- S. Y. Corbel, A. Lee, L. Yi, J. Duenas, T. R. Brazelton, H. M. Blau and F. M. Rossi, Contribution of hematopoietic stem cells to skeletal muscle, Nat. Med., 2003, 9, 1528–1532 CrossRef CAS PubMed.
- M. A. S. Moore, K. Hattori, B. Heissig, J.-H. Shieh, S. Dias, R. G. Crystal and S. Rafii, Mobilization of endothelial and hematopoietic stem and progenitor cells by adenovector-mediated elevation of serum levels of SDF-1, VEGF, and angiopoietin-1, Ann. N. Y. Acad. Sci., 2001, 938, 36–47 CrossRef CAS PubMed.
- C. Kalka, H. Masuda, T. Takahashi, R. Gordon, O. Tepper, E. Gravereaux, A. Pieczek, H. Iwaguro, S. I. Hayashi, J. M. Isner and T. Asahara, Vascular endothelial growth factor 165 gene transfer augments circulating endothelial progenitor cells in human subjects, Circ. Res., 2000, 86, 1198–1202 CrossRef CAS PubMed.
- D. Zisa, A. Shabbir, M. Mastri, G. Suzuki and T. Lee, Intramuscular VEGF repairs the failing heart: role of host-derived growth factors and mobilization of progenitor cells, Am. J. Physiol.: Regul., Integr. Comp. Physiol., 2009, 297, R1503–R1515 CrossRef CAS PubMed.
- J. P. Skora, M. Antkiewicz, D. Kupczynska, K. Kulikowska, B. Strzelec, D. Janczak and P. Barc, Local intramuscular administration of ANG1 and VEGF genes using plasmid vectors mobilizes CD34+ cells to peripheral tissues and promotes angiogenesis in an animal model, Biomed. Pharmacother., 2021, 143, 112186 CrossRef CAS PubMed.
- L. A. Alfaro, S. A. Dick, A. L. Siegel, A. S. Anonuevo, K. M. McNagny, L. A. Megeney, D. D. Cornelison and F. M. Rossi, CD34 promotes satellite cell motility and entry into proliferation to facilitate efficient skeletal muscle regeneration, Stem Cells, 2011, 29, 2030–2041 CrossRef CAS PubMed.
- B. Zheng, B. Cao, M. Crisan, B. Sun, G. Li, A. Logar, S. Yap, J. B. Pollett, L. Drowley, T. Cassino, B. Gharaibeh, B. M. Deasy, J. Huard and B. Péault, Prospective identification of myogenic endothelial cells in human skeletal muscle, Nat. Biotechnol., 2007, 25, 1025–1034 CrossRef CAS PubMed.
- G. Ferrari, G. Cusella, D. Angelis, M. Coletta, E. Paolucci, A. Stornaiuolo, G. Cossu and F. Mavilio, Muscle regeneration by bone marrow-derived myogenic progenitors, Science, 1998, 279, 1528–1530 CrossRef CAS PubMed.
- J. Wu, S. Saovieng, I. S. Cheng, J. Jensen, W.-H. Jean, A. Alkhatib, C.-L. Kao, C.-Y. Huang and C.-H. Kuo, Satellite cells depletion in exercising human skeletal muscle is restored by ginseng component Rg1 supplementation, J. Funct. Foods, 2019, 58, 27–33 CrossRef CAS.
- J. P. Nederveen, S. Joanisse, C. M. Séguin, K. E. Bell, S. K. Baker, S. M. Phillips and G. Parise, The effect of exercise mode on the acute response of satellite cells in old men, Acta Physiol., 2015, 215, 177–190 CrossRef CAS PubMed.
- K. Jędrejko, K. Kała, K. Sułkowska-Ziaja, J. Pytko-Polończyk and B. Muszyńska, Effect of Cordyceps spp. and cordycepin on functions of bones and teeth and related processes: a review, Molecules, 2022, 27, 8170 CrossRef PubMed.
- A. Ludin, S. Gur-Cohen, K. Golan, K. B. Kaufmann, T. Itkin, C. Medaglia, X. J. Lu, G. Ledergor, O. Kollet and T. Lapidot, Reactive oxygen species regulate hematopoietic stem cell self-renewal, migration and development, as well as their bone marrow microenvironment, Antioxid. Redox Signal., 2014, 21, 1605–1619 CrossRef CAS PubMed.
- T. T. Peternelj and J. S. Coombes, Antioxidant supplementation during exercise training: beneficial or detrimental?, Sports Med., 2011, 41, 1043–1069 CrossRef PubMed.
- S. Abe, M. Suzuki, K. H. Cho, G. Murakami, B. H. Cho and Y. Ide, CD34-positive developing vessels and other structures in human fetuses: an immunohistochemical study, Surg. Radiol. Anat., 2011, 33, 919–927 CrossRef PubMed.
- S. Lecourt, J. P. Marolleau, O. Fromigué, K. Vauchez, R. Andriamanalijaona, B. Ternaux, M. N. Lacassagne, I. Robert, K. Boumédiene, F. Chéreau, P. Marie, J. Larghéro, M. Fiszman and J. T. Vilquin, Characterization of distinct mesenchymal-like cell populations from human skeletal muscle in situ and in vitro, Exp. Cell Res., 2010, 316, 2513–2526 CrossRef CAS PubMed.
- C. Christov, F. Chretien, R. Abou-Khalil, G. Bassez, G. Vallet, F. J. Authier, Y. Bassaglia, V. Shinin, S. Tajbakhsh, B. Chazaud and R. K. Gherardi, Muscle satellite cells and endothelial cells: close neighbors and privileged partners, Mol. Biol. Cell, 2007, 18, 1397–1409 CrossRef CAS PubMed.
- Y. H. Lin, S. C. Tsai, S. J. Chuang, M. B. Harris, K. Masodsai, P. N. Chen, C. C. Hsieh, T. Killian, C. Y. Huang and C. H. Kuo, Whole-life body composition trajectory and longevity: role of insulin, Aging, 2021, 13, 9719–9731 CrossRef CAS PubMed.
- G. Haskó and B. Cronstein, Regulation of inflammation by adenosine, Front. Immunol., 2013, 4, 85 Search PubMed.
- A. T. Bosenberg, J. G. Brock-Utne, S. L. Gaffin, M. T. Wells and G. T. Blake, Strenuous exercise causes systemic endotoxemia, J. Appl. Physiol., 1988, 65, 106–108 CrossRef CAS PubMed.
- Y. Yan, M. Li, J. Lin, Y. Ji, K. Wang, D. Yan, Y. Shen, W. Wang, Z. Huang, H. Jiang, H. Sun and L. Qi, Adenosine monophosphate activated protein kinase contributes to skeletal muscle health through the control of mitochondrial function, Front. Pharmacol., 2022, 13, 947387 CrossRef CAS PubMed.
|
This journal is © The Royal Society of Chemistry 2024 |
Click here to see how this site uses Cookies. View our privacy policy here.