Effects of brining and standardized drying on the composition, shelf stability, microbial safety and antioxidant status of four wild edible vegetables†
Received
8th March 2024
, Accepted 29th May 2024
First published on 30th May 2024
Abstract
Wild edible vegetables (WEVs) are integral for sustained nutrition and livelihood of forest-dwelling tribal communities. Shelf-life enhancement of WEVs is crucial to overcome their perishability and ensure availability. Chemical preservatives are detrimental to their commercial ‘organic’ status, and modern processing technologies are often challenging to be implemented by the marginalized farmers. In this study, four nutritionally important WEVs popularly cultivated, consumed and marketed in the hilly, forest-covered state of Mizoram, India, namely, Solanum aethiopicum (ST), Solanum torvum (TP), Solanum anguivi (TT) and Leucaena leucocephala (JZ), were subjected to brining and drying to enhance their shelf-lives. The drying method was standardized using response surface methodology at 80 °C and 540 minutes, obtaining the lowest moisture content with the highest total phenolic content (TPC) and DPPH radical scavenging activity (DRSA). A strong positive correlation was obtained between TPC and DRSA values of ST (R = 0.738), TP (R = 0.760), TT (R = 0.977) and JZ (R = 0.935). Both brined bottled and dried vacuum-packaged samples were studied over 35 days of storage. Packaged dried samples were stored at room temperature (RT) and under refrigeration (RF). Brined WEVs showed a decrease in moisture and carbohydrate contents. Microbial conversion of sugars to lactic acid was evidenced by lowering pH to below 3.0. Bacterial activity also markedly enhanced TPC, flavonoids and DRSA values by causing free phenolics release and their structural transformation. However, overall microbial population in terms of aerobic coliform bacteria and fungal species were efficiently retarded up to more than 65% by the two treatments. Vacuum-packaged RF samples exhibited lowered microbial metabolism. Pigment degeneration and tissue structure changes were indicated by changed color and hardness. Dried tissue brittleness enhanced the extractability of bioactive compounds. Vacuum-packaging improved antioxidant retention in the processed WEVs.
Sustainability spotlight
Fresh organic wild edible produces grow abundantly in forest-clad, rain-fed hills. Such produces are the major means of nutritional and financial security of the marginalized forest dwellers. Difficult terrains, insufficient resources and market demands for ‘chemical-free’ status make it further grim to process these fruits and vegetables using popular chemical techniques. Emphasis on sustainable processing solutions aligns with the global sustainability goals of combatting poverty, ensuring nutrition and food safety and promoting good health and well-being. Besides, they show potential to boost the livelihoods of the forest-dwelling tribal populations, fostering holistic sustainable communities. This study uses the cost-effective natural processing techniques of brining and drying to minimally process four widely consumed wild edible vegetables from Mizoram, India, to enhance their storage stability and use.
|
1. Introduction
Food security ensures safe and nutritious food. Progressive agricultural practices, soil, climate, irrigation, and constant goods supply are primary requisites for food security. Geographically secluded and economically marginalized groups have been substantially predisposed to food insecurity, often leading to hunger and undernutrition.1 Increasing population, physical inaccessibility, transportation limitations, sporadic food supply chain and landscape vulnerability are secondarily associated prominent factors. Sustainable solutions in food processing align with the global sustainability goals. Sustainable processing of naturally growing organic produces would address food availability and mitigate scarcity issues by increasing the shelf lives of these seasonal produces. This aligns with the broader goal of achieving food availability and improving access to nutrition during challenging times.
Mizoram is a predominantly hilly state located at the southernmost extremity of North-East India, sharing international boundaries with Bangladesh and Myanmar. The state population grew by about 20% during 2011 to 2023. With about 85.41% forest cover, the rainfed landscape sees a frail road connectivity.2,3 Historically, the tribal population of the region has been largely dependent on various wild edible vegetables (WEVs). A few prominent species are Fagopyrum tataricum, Solanum indicum, Marsdenia maculata, Rhynchotechum ellipticum, Ensete superbum, Aganope thyrsiflora, Caryota mitis, Aralia dasyphylla, Centella asiatica, Trevesia palmata, Parkia timoriana, Amaranthus viridis, Gnetum gnemon, Oroxylum indicum, Amorphophallus nepalensis, Amomum dealbatum, Solanum aethiopicum, Solanum torvum, Solanum incanum and Leucaena leucocephala.3,4 The WEVs are ethnically claimed to have nutritional and therapeutic benefits due to their antioxidative, antimicrobial potential and lack of use of pesticides and insecticides.4 Several WEVs and their extracts have ethnomedicinal uses against anemia, diabetes, toxicity and inflammatory diseases, as established by in vivo and in vitro studies.5–7 In recent times, the local farmers have created semi-natural ecosystems for organized cultivation of the WEVs. Of these, S. aethiopicum, S. torvum, S. anguivi and Leucaena leucocephala, locally known as Samtawk, Tawkpui, Tawkte, and Japan Zawngtah, respectively have been successful in attaining abundant local commercialization and gaining popularity owing to their higher yield and relatively prolonged availability over others. However, being semi-perishable, a great proportion of each WEV produce is annually wasted.8 No work on technologically preserving the major WEVs of Mizoram, encompassing process control for shelf-life enhancement, nutrient and bioactive retention and microbial safety, has been reported till date.
Globally, most research studies on WEVs have been reported from countries and regions having traditions of using WEVs as food and remedy.5,9–12 Considering the meagre economic conditions of the marginalized tribal farmers and the organic (synthetic-chemical free) status of these produces, cost-effective minimal processing methods could be identified as the primary sustainable preservation approach. Essentially, most studies on processing of WEVs have been on blanching, drying, boiling, traditional fermentation and their effects on nutritional, antinutritional and bioactive properties.9,13–16
Drying is the most conventional method of food preservation. With drying causing a decrease in moisture content and water activity, food surfaces become inhospitable to microorganisms causing spoilage. Apart from classical drying methods such as sun-, air- and oven-drying, most modern drying methods such as vacuum-, freeze- and microwave-drying, have been developed and extensively used in food industries.17 Vegetables are variably affected by drying conditions. Reports of increased nutritional and bioactive potential of dried vegetables as well as those contradicting it have been published.18,19 The standardization of drying conditions is therefore crucial. Brining is another conventional preservation method that uses salt solution (2 to 15%). The salt concentration in brine creates a hypertonic environment around microbial cells. As moisture moves from lower concentration to higher, most cells dehydrate and their metabolic functions disrupt, inhibiting their growth and spoilage activities. Brining also helps in the preservation of nutrients and antioxidant compounds.20,21 Such basic processing techniques ensure the ‘organic status’ of WEVs, promoting good health and well-being of the consumers. Besides, they show potential to boost the economic livelihoods of the forest-dwelling tribal populations, fostering holistic sustainable communities.
This study encompasses brining and drying of Solanum aethiopicum, Solanum torvum, Solanum anguivi and Leucaena leucocephala followed by storage in appropriate conventional packaging systems. Nutritional, phytochemical, physicochemical and microbiological changes over the storage periods were determined. Based on the results of these characteristics, the process and storage conditions for each WEV were standardized.
2. Materials and methods
The WEV samples (Fig. 1) were collected from the natural wild habitats of Aizawl, Mizoram, and coded considering their local names as SM (Samtawk), TP (Tawkpui), TT (Tawkte) and JZ (Japan Zawngtah). The collected samples were stored in a shed for 5 h and destemmed before processing. Chemicals of analytical grade were procured from Himedia, SRL Chemicals and Merck (India).
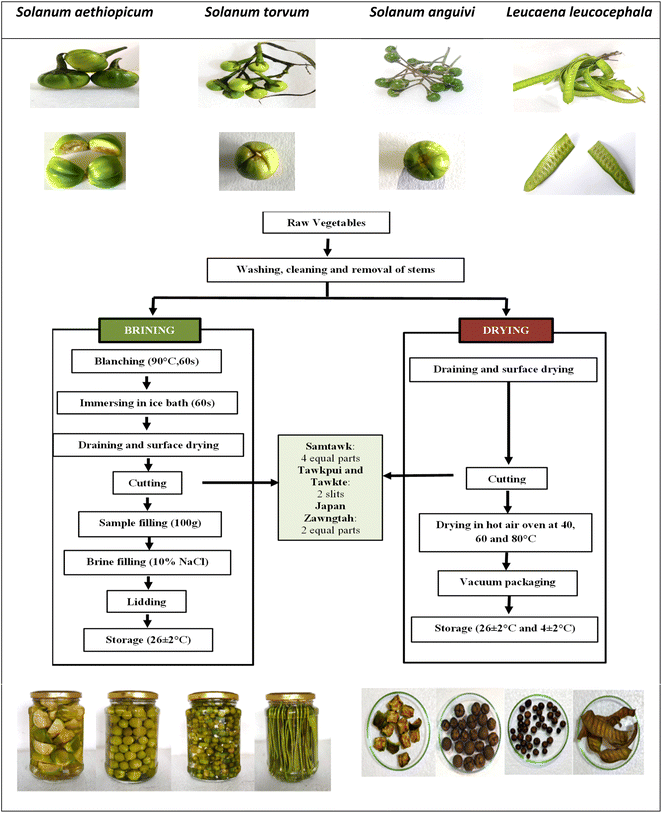 |
| Fig. 1 Raw and cut vegetables (top), methods of brining and drying (middle), brined and dried samples (bottom). | |
2.1. Brining of vegetables
For brining, the WEVs were thoroughly washed, followed by blanching in water at 90 °C for 60 s. The blanched vegetables were immediately immersed in chilled water (1–4 °C) for 60 s, drained and surface-dried under constant airflow at room temperature (RT, 26 ± 2 °C). The WEVs were then cut as follows. SM: 4 equal parts, TP and TT: 2 slits each, and JZ: 2 equal parts (Fig. 1). After that, 100 g each of the cut samples were then transferred to steam-sterilized glass jars. The glass jars were then filled with 10% sodium chloride brine solution, followed by air-tight lidding and storage at RT (Fig. 1). The stored vegetables were analyzed for biochemical changes and microbial activities on every 5th day till the 35th day of storage.
2.2. Drying of vegetables
Vegetable samples (250 g each) were cut, spread as a single layer and dried in a hot air oven at 40 °C, 60 °C and 80 °C for variable durations. Response surface methodology (RSM) was used to optimize the best drying temperature and time combinations for each vegetable using the Design Expert 12.0 software. The dried vegetables were vacuum-packaged (Model D05, Enem) in 100 g polypropylene packages and stored at RT and under refrigeration (RF, 4 ± 2 °C) (Fig. 1). Biochemical and microbial analyses were performed after every 5 days till the 35th day of storage.
2.2.1. Drying process optimization.
Central composite face-centered (CCFC) model of RSM was used for process optimization. Drying temperature and drying time were taken as the two independent variables at 5 levels. The CCFC model consisted of 13 experiments including 5 centre, 4 axial and 4 factorial points. The dependent variables or responses were moisture content, total phenolic content (TPC) and DPPH (2,2-diphenyl-1-picrylhydrazyl) radical scavenging antioxidant activity (DRSA). The experimental data obtained were fit into a second-order polynomial model using the following equation: | Y = a0 + a1X1 + a2X2 + a11X12 + a22X22 + a12X1X2 | (1) |
where Y is the predicted response for the dependent variables, a0 is the fitted response at the centre point, a1 and a2 are the linear points of predicted responses, a12 is the interaction effect, and a11 and a22 are the squared effects. X1 and X2 are the independent variables. The data were statistically analyzed by ANOVA at two levels and 95% confidence level (p ≤ 0.05) for significance.
2.3. Proximate composition
Changes in the moisture, protein, fat and ash contents of the brined and dried WEVs (at RT and RF) over 35 days of storage were assessed using standard AOAC (2019) protocols.22 Readings were taken on every 5th day of storage. The total carbohydrate content was calculated by difference. The results are expressed in percentage on wet basis for moisture content and dry basis for fat, protein, ash and carbohydrate.
2.4. Phytochemicals and antioxidant activities
2.4.1. Extraction of samples.
Raw, brined and dried samples were subjected to lyophilization in a freeze dryer (Harvest Right, Cole-Parmer, India). The samples were then powdered and sieved (particle size = 100–212 μm). One gram of each sample was extracted in 10 ml ethanol at 40 °C for 4 h and centrifuged at 1000 rpm for 60 min. The obtained supernatant was stored at −20 °C until further analyses.
2.4.2. Total phenolic content.
The Folin–Ciocâlteu Reagent (FCR) method was used to estimate the TPC of the raw and preserved WEV samples.23 For this, 500 μl of the ethanolic extract was mixed with 2.5 ml of 10% (w/v) FCR (Merck, India). Within 8 min, 2 ml of 7.5% sodium carbonate was added and vortexed before incubation in the darkness at 30 °C for 30 min. The absorbance was measured at 765 nm and compared with a calibration curve prepared for gallic acid. The TPC is expressed as gallic acid equivalents per gram (mgGAE per g) of sample.
2.4.3. Total flavonoid content.
The total flavonoid content (TFC) was measured by a method described by Alam et al. (2020) with slight modifications.24 Briefly, 0.5 ml ethanolic extract was mixed with 1.5 ml of 95% ethanol, 0.1 ml of 10% aluminium chloride, 0.1 ml of 1 M potassium acetate and 2.8 ml of distilled water. After vortexing, the mixture was allowed to stand for 5 min and 1 ml of 1 M sodium hydroxide was added. The absorbance was measured at 510 nm and plotted against a standard curve prepared using a standard quercetin solution. The TFC values are expressed as quercetin equivalents per hundred grams (mg QE per 100 g) of sample.
2.4.4. DPPH radical scavenging activity.
A blank solution was prepared by mixing 100 μl ethanol with 1 ml of 0.1 mM DPPH. Then, 100 μl ethanolic sample extract was mixed with 1 ml of the DPPH solution (0.1 mM) and incubated in the darkness for 30 min. The absorbance was measured at 517 nm, and the results are expressed as percentage DRSA using the following equation:24 | 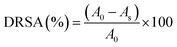 | (2) |
where A0 is the absorbance of the blank and As is the absorbance of the sample extract.
2.4.5. Ferric reducing antioxidant power.
The ferric reducing antioxidant power (FRAP) was determined using the method reported by Aryal et al. (2019).23 An FRAP solution was freshly prepared by mixing 5 ml of a 10 mM 2,4,6-TPTZ [2,4,6-tri(2-pyridyl)-1,3,5-triazine] solution in 40 mM hydrochloric acid with 5 ml of 20 mM ferric chloride and 50 ml of 0.3 M acetate buffer (pH 3.6). Briefly, 100 μl aliquot of extract was thoroughly mixed with 3 ml of the FRAP solution and incubated at 37 °C for 4 min. The absorbance was determined at 593 nm taking distilled water as blank. A calibration curve was constructed using a concentration range of 0.1 to 1 mM aqueous solutions of ferrous sulphate. The FRAP values are expressed as millimoles of ferrous equivalent Fe(II) per gram (mmol g−1) of sample.
2.5. Color values
The color values L* (lightness or darkness), a* [greenness (−) to redness (+)] and b* [yellowness (−) to blueness (+)] of the samples were determined using a Hunter Lab Color Quest spectrophotometer (Ultrascan Vis, USA). The brined samples were carefully surface-wiped using tissue paper before examination. The total color difference (ΔE*) was calculated using the following equation: |  | (3) |
2.6. Texture
The hardness of the samples was measured using a texture analyzer (TA.XT. Plus, Stable Microsystems, UK) fitted with a 50 kg load cell. Each sample was carefully placed on the platform using forceps and compressed using a 35 mm–diameter probe along the thickness at a speed of 5 mm s−1. Considering the difference in sample thickness, the compression distance was set at 2 mm for SM and TP, whereas 1 mm for TT and JZ.
2.7. pH
The pH of the brined fruit samples was analyzed using a pH meter (Labtronics LT-23, India).
2.8. Microbial count
As described by Managa et al. (2021), 1.0 g of sample was blended with 10 ml sterile peptone water (0.1%).25 The aliquot was serially diluted up to 10-fold and plated by a pour plate method. The enumeration of total aerobic bacteria was done by culturing on plate count agar followed by incubation at 37 °C for 48 h. For lactic acid bacteria (LAB) and coliform bacteria, plating was done on MRS agar and Hi-Crome E. coli agar respectively and incubated similarly. The yeast and mold count of the plant samples was performed on M-BCG Yeast and Mold agar after incubating at 30 °C for 48 h. The microbial count is expressed as log CFU ml−1.
2.9. Statistical analysis
Data are presented as arithmetic means of at least three replicates for each experimented sample. Design Expert 12.0 was used for the analysis of the optimization data. The differences in the experimental values were analyzed using Duncan's multiple range test (p ≤ 0.05) and ANOVA, and correlation was measured through bivariate analysis by Pearson's coefficient (two tailed test) using the SPSS (version 21) statistical software.
3. Results and discussions
3.1. Drying process optimization
The CCFC-derived experimental results of the variables and their responses for each WEV are provided in the ESI (ESI, Tables S1, S3, S5 and S7).† Quadratic interaction and lack of fit of the responses were identified. The R2 values for the three dependent variables (responses) were above 0.80, which was indicative of adequate fit. The lack of fit for all the fitted models was found to be not significant (p ≤ 0.05). Hence, it was established that the selected model could be used to design and optimize variables for drying of the WEVs.
3.1.1. Effect of drying temperature and drying time on the responses.
The optimization protocol was adopted to obtain dried vegetables with high TPC and DRSA. In relation to the independent variables, changes in responses and their regression equations are provided in ESI ((E1) to (E12)).† The regression coefficient, significance and lack of fit obtained by statistical analysis of the responses are also provided (ESI, Tables S2, S4, S6 and S8†). Significant influence of the independent variables on the responses could be identified for each WEV. Three-dimensional surface plots were generated for the responses and assessed to obtain the optimal conditions of drying treatments (ESI, Fig. S1†). As drying temperature and time were reduced, responses in the form of TPC and DRSA showed lower values with higher values of moisture content. Inversely, an increase in temperature and time generated products with lower moisture, higher TPC and higher DRSA.26
Polynomial equations of second order were formulated to ascertain the efficacy of the drying process. Based on the obtained F values, it was established that the models representing moisture content, TPC and DRSA could reliably predict the effects of drying temperature and time on the antioxidant properties of the respective WEVs. Through ANOVA analysis, the coefficient values (R2) were found to be significant and without pure or residual errors. Notably, temperature exerted a more pronounced influence on the responses than time. This was confirmed by Pearson's correlation coefficients obtained through bivariate analysis between the independent variables and responses (ESI, Table S9†). Significant correlation between moisture content and drying temperature was observed for all the WEVs (R > 0.80). Non-significant correlation was observed with drying time (R < 0.55). TPC also exhibited a similar pattern. Drying temperature and TPC were significantly correlated (R > 0.65). Drying time gave relatively lower R values (R = 0.569, 0.473, 0.653, and 0.502) for ST, TP, TT and JZ, respectively. However, significantly strong correlation (R > 0.60) was observed between both drying temperature and drying time with DRSA. Consistently, the highest TPC and DRSA were observed at a temperature of 80 °C for all WEVs.
Under higher drying temperature and longer drying time, the extractability of phenolics and antioxidant components got enhanced due to the higher heat exposure, causing brittleness in the cell wall and interstitial materials. This could enhance tissue breakdown upon milling, releasing more bound phenolics. The hydrogen donating capacity of phenolic compounds relates to their antioxidant behavior. Hence, a strong positive correlation was obtained between the increased TPC and the DRSA values of ST (R = 0.738), TP (R = 0.760), TT (R = 0.977) and JZ (R = 0.935). High-temperature exposure can prevent the degradation of phenolic components by inactivating oxidative enzymes. Moreover, high-temperature dry heat can reportedly break down high-molecular weight phenolic compounds to lower molecular weight phenolic acids, enhancing their extractability and quantification.27
3.1.2. Verification of the model.
Optimization was centered on minimizing the moisture content while concurrently maximizing the TPC and DRSA. For drying each WEV, the suitability of the model was established under the optimal values 80 °C and 540 min of time and temperature, respectively (ESI, Table S10†). The experimental values were in agreement with the predicted values (ESI, Table S11†).
3.2. Proximate composition
The proximate compositions of the raw WEVs and their processed and stored samples on 0th and 35th day of storage are listed in Table 1. The detailed trends in changes are illustrated in ESI (Fig. S2 and S3).† Along with apparent and obvious moisture reduction due to high salt concentration creating osmotic stress on tissues, the carbohydrate content in the brined samples also reduced over storage. This could be indicative of the microbial activity.15 During the brining process, microorganisms convert carbohydrates into simple sugars for metabolic uses. The sugars are further converted into acids and alcohols amongst other compounds, resulting in an overall decline in the carbohydrate content. Osmotic stress – induced cell plasmolysis could have aided in the release of minerals and proteins from the cells, thereby enhancing their quantifiable values over storage. Dried RF samples showed a slightly lower reduction in moisture content as compared to RT samples. Razak et al. (2018) reported that the free moisture content of stored vegetables increases at a higher temperature of storage, irrespective of the method of packaging and is attributed to the higher activity of microorganisms at elevated storage temperatures.28,29 Enhanced dry matter content per unit mass of the dried samples resulted in increased values of carbohydrate contents calculated on dry basis.
Table 1 Proximate compositions of fresh, brined and dried vegetables over storagea
Parameters |
Processing type |
ST |
TP |
TT |
JZ |
Fresh |
0th day |
35th day |
Fresh |
0th day |
35th day |
Fresh |
0th day |
35th day |
Fresh |
0th day |
35th day |
The values are reported as mean ± standard deviation of triplicate determinations. ST: Samtawk, TP: Tawkpui, TT: Tawkte, JZ: Japan Zawngtah. Values of each parameter marked with same superscripted alphabet do not vary significantly from one another.
|
Moisture (% w.b) |
Brined |
86.24 ± 2.72a |
86.24 ± a1.92 |
76.55 ± 1.86c |
83.23 ± 3.07a |
83.23 ± 3.07a |
75.87 ± 2.45c |
54.44 ± 2.29a |
54.44 ± 2.29a |
47.62 ± 2.16c |
20.02 ± 2.24a |
20.02 ± 1.24a |
11.78 ± 1.65c |
Dried (RT) |
1.94 ± 0.05b |
2.97 ± 002d |
1.74 ± 0.04b |
2.82 ± 0.04d |
1.02 ± 0.07b |
2.34 ± 0.05d |
1.04 ± 0.04b |
2.45 ± 0.04d |
Dried (RF) |
2.35 ± 0.08bd |
2.19 ± 0.23bd |
2.03 ± 0.06d |
2.10 ± 0.02d |
Fat (%) |
Brined |
4.32 ± 0.02a |
4.32 ± 0.02a |
4.39 ± 0.07a |
20.90 ± 0.04a |
20.90 ± 0.04a |
20.93 ± 0.67a |
20.46 ± 0.04a |
20.46 ± 0.04a |
20.50 ± 0.76a |
15.20 ± 0.07a |
15.20a ± 0.07 |
15.27 ± 0.09a |
Dried (RT) |
4.32 ± 0.07a |
4.19 ± 0.04a |
20.54 ± 1.23a |
20.35 ± 1.04a |
20.29 ± 0.87a |
20.06 ± 0.78a |
15.10a ± 011 |
15.02 ± 0.12a |
Dried (RF) |
4.21 ± 0.08a |
20.46 ± 2.22a |
20.12 ± 0.98a |
15.09 ± 0.18a |
Protein (%) |
Brined |
10.10 ± 0.24a |
10.10 ± 0.24a |
14.71 ± 0.98b |
25.11 ± 0.28a |
25.11 ± 0.28a |
28.26 ± 1.21b |
16.66 ± 0.35a |
16.66 ± 0.35a |
18.84 ± 0.67b |
20.40 ± 1.31a |
20.40 ± 1.31a |
22.25 ± 1.22b |
Dried (RT) |
9.98 ± 0.32a |
9.90 ± 0.27a |
24.69 ± 1.45a |
24.45 ± 1.67a |
16.55 ± 0.55a |
16.30 ± 0.45a |
20.26 ± 0.76a |
19.99 ± 0.88a |
Dried (RF) |
9.88 ± 0.22a |
24.59 ± 1.02a |
16.40 ± 0.12a |
20.08 ± 0.76a |
Ash (%) |
Brined |
8.87 ± 0.27a |
8.87 ± 0.27a |
13.30 ± 0.21b |
12.29 ± 0.33a |
12.29 ± 0.33a |
16.62 ± 0.93b |
7.06 ± 0.12a |
7.06 ± 0.12a |
9.87 ± 0.03b |
7.67 ± 0.09a |
7.67 ± 0.09a |
10.13 ± 0.11b |
Dried (RT) |
8.68 ± 0.29a |
8.61 ± 0.18a |
12.08 ± 0.67a |
12.02 ± 0.78a |
7.05 ± 0.08a |
7.02 ± 0.02a |
7.65 ± 0.04a |
7.59 ± 0.06a |
Dried (RF) |
8.66 ± 0.21a |
12.05 ± 0.62a |
7.03 ± 0.78a |
7.53 ± 0.07a |
Carbohydrate (%) |
Brined |
76.69 ± 1.72a |
76.69 ± 1.72a |
68.31 ± 1.34 |
41.68 ± 1.30a |
41.68 ± 1.30a |
34.02 ± 0.88b |
55.81 ± 1.04a |
55.81 ± 1.04a |
50.24 ± 1.54b |
56.73 ± 2.44a |
56.73 ± 2.44a |
51.79 ± 2.11b |
Dried (RT) |
74.25 ± 1.45b |
74.49 ± 1.89b |
40.98 ± 1.34a |
40.56 ± 1.19c |
55.29 ± 1.18a |
55.72 ± 1.23a |
56.19 ± 1.78a |
55.39 ± 2.12c |
Dried (RF) |
74.95 ± 1.54b |
40.82 ± 1.22ac |
54.85 ± 1.33a |
55.62 ± 2.02c |
3.3. pH
The initial pH of 6.65, 7.40, 7.18, and 6.33 recorded for freshly brined ST, TP, TT, and JZ dropped to 3.06, 3.14, 3.18, and 3.20 respectively during the 35 days of storage (Fig. 2). This confirmed the growth of LAB population producing organic acids (predominantly lactic acid) through the Embden–Meyerhof, phosphoketolase, tagatose-6-phosphate and Leloir pathways of carbohydrate metabolism.30 Lactic acid, amongst other effects in brined products, also adds to preservation advantages. LAB consumed the sugars present in the vegetables and converted them to lactic acid. Due to acid accumulation, the pH of the brines dropped progressively over the storage time. This drop in pH was accompanied by the decrease in the carbohydrate content in the vegetable samples (Table 1) due to the metabolic conversion of carbohydrates into sugars and further to lactic acid.
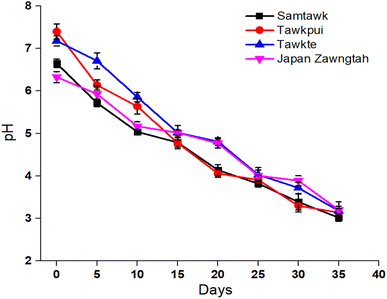 |
| Fig. 2 Change in the pH of brined vegetables over time. | |
3.4. Microbial count
Brining was able to retard total bacterial and fungal count in each stored WEV (Table 2). Salt and anaerobic conditions in the brining jars impeded bacterial and fungal growth. Overall microbial death was concurrently accelerated with the declining pH of the brining medium during the storage period. Very few to no visible bacterial colony was detected on and after the 15th day of storage. Till 15th day, anaerobic conditions encouraged the growth of LAB, which was followed by a decline with the lowering of pH to below 4.0, suggesting acid over-exposure by the 20th day.31,32 The high concentration of salt in brine is also responsible for retarding the establishment of numerous spoilage microbial species, however it also aids in the establishment of LAB.32 Popular fermented vegetable products such as kimchi or sauerkraut require the addition of salt to initiate the necessary lactic acid fermentation of the vegetables.31 However, pH control is crucial for prolonged sustenance of the fermenting culture.
Table 2 Total plate count, lactic acid bacteria count (LAB), coliform count and yeast and mold count of the brined vegetables over storagea
Days |
Total plate count |
LAB count |
Coliform count |
Yeast and mold count |
ST |
TP |
TT |
JZ |
ST |
TP |
TT |
JZ |
ST |
TP |
TT |
JZ |
ST |
TP |
TT |
JZ |
The values are reported as mean ± standard deviation of triplicate determinations. ST: Samtawk, TP: Tawkpui, TT: Tawkte, JZ: Japan Zawngtah, ND: not detected. Values of each parameter marked with same superscripted alphabet do not vary significantly from one another.
|
0 |
5.2 ± 0.01d |
6.3 ± 0.03e |
5.7 ± 0.01e |
4.9 ± 0.05d |
4.7 ± 0.02b |
4.9 ± 0.02bc |
5.3 ± 0.01c |
4.2 ± 0.02c |
3.1 ± 0.01c |
2.5 ± 0.01c |
2.3 ± 0.01c |
1.9 ± 0.01b |
4.1 ± 0.01e |
4.6 ± 0.01f |
3.8 ± 0.02e |
4.4 ± 0.02e |
5 |
4.7 ± 0.05d |
6.1 ± 0.01e |
5.3 ± 0.02de |
4.4 ± 0.03 cd |
5.1 ± 0.02b |
5.0 ± 0.01bc |
5.7 ± 0.04c |
4.8 ± 0.04c |
2.9 ± 0.01c |
2.6 ± 0.02c |
2.1 ± 0.02bc |
1.5 ± 0.01 ab |
4.0 ± 0.05e |
4.3 ± 0.02f |
3.9 ± 0.03e |
4.9 ± 0.01d |
10 |
4.1 ± 0.01cd |
5.4 ± 0.01de |
4.7 ± 0.02d |
4.1 ± 0.01cd |
6.7 ± 0.01c |
6.4 ± 0.06cd |
6.8 ± 0.03d |
6.6 ± 0.01d |
1.7 ± 0.01b |
1.4 ± 0.01b |
1.3 ± 0.1ab |
0.6 ± 0.01a |
3.5 ± 0.02de |
3.7 ± 0.01ef |
3.1 ± 0.03de |
3.4 ± 0.02c |
15 |
3.7 ± 0.01c |
4.6 ± 0.02cd |
4.1 ± 0.01cd |
3.8 ± 0.01c |
7.3 ± 0.04c |
6.9 ± 0.01d |
7.3 ± 0.01d |
6.9 ± 0.3d |
0.5 ± 0.00a |
0.7 ± 0.0ab |
0.4 ± 0.01a |
0.1 ± 0.00a |
2.6 ± 0.01cd |
2.8 ± 0.2de |
2.2 ± 0.04cd |
2.1 ± 0.06b |
20 |
3.3 ± 0.02bc |
4.1 ± 0.01c |
3.6 ± 0.03c |
3.0 ± 0.02bc |
5.4 ± 0.01b |
5.8 ± 0.03c |
7.1 ± 0.06d |
5.1 ± 0.01c |
ND |
0.1 ± 0.0a |
ND |
ND |
1.7 ± 0.04bc |
2.1 ± 0.01c |
1.8 ± 0.01bc |
1.8 ± 0.01b |
25 |
2.9 ± 0.02b |
3.1 ± 0.02b |
3.0 ± 0.01bc |
2.8 ± 0.03b |
5.1 ± 0.03b |
5.6 ± 0.02c |
5.1 ± 0.02c |
4.2 ± 0.06c |
ND |
ND |
ND |
ND |
1.3 ± 0.02ab |
1.4 ± 0.03bc |
1.1 ± 0.02ab |
1.5 ± 0.2ab |
30 |
2.1 ± 0.03ab |
2.4 ± 0.01ab |
2.5 ± 0.02ab |
2.1 ± 0.01b |
3.6 ± 0.01a |
4.3 ± 0.03b |
3.8 ± 0.01b |
3.2 ± 0.02b |
ND |
ND |
ND |
ND |
0.7 ± 0.01a |
1.1 ± 0.06ab |
0.8 ± 0.01a |
1.2 ± 0.01a |
35 |
1.3 ± 0.01a |
1.9 ± 0.06a |
2.0 ± 0.01a |
1.1 ± 0.01a |
3.1 ± 0.02a |
2.9 ± 0.05a |
2.5 ± 0.02a |
1.9 ± 0.1a |
ND |
ND |
ND |
ND |
0.5 ± 0.01a |
0.4 ± 0.01a |
0.2 ± 0.01a |
0.7 ± 0.1a |
Drying marginally reduced the population of microbiota (Tables 3 and 4). The high temperature maintained during drying in combination with low water activity generated could inactivate microbial cells present on the WEV surface. However, populations of microbes present beneath the surface could have potentially survived the heat and dehydration stress due to the presence of sucrose, polyols, polypeptides and amino acids providing growth environment within the tissues. Low moisture conditions can reportedly enhance thermal resistance of certain microbial species.33 This was made apparent by the significant number of colonies observed on plating. Additionally, fruits and vegetables having low pH are lesser prone to microbial infestations.33 Vacuum-packed, RT stored vegetables showed steady microbial population till 25th day followed by a slight increase thereafter (Table 4). However, RF storage significantly reduced the microbial populations in the vacuum-packaged WEVs (Table 4).
Table 3 Total plate count, lactic acid bacteria count (LAB), coliform count and yeast and mold count of the dried vegetables over room temperature (RT) storagea
Days |
Total plate count |
LAB count |
Coliform count |
Yeast and mold count |
ST |
TP |
TT |
JZ |
ST |
TP |
TT |
JZ |
ST |
TP |
TT |
JZ |
ST |
TP |
TT |
JZ |
The values are reported as mean ± standard deviation of triplicate determinations. ST: Samtawk, TP: Tawkpui, TT: Tawkte, JZ: Japan Zawngtah, ND: not detected. Values of each parameter marked with same superscripted alphabet do not vary significantly from one another.
|
Fresh |
5.7 ± 0.06abc |
6.5 ± 0.02b |
5.7 ± 0.05bc |
5.2 ± 0.01a |
4.3 ± 0.02 ab |
4.6 ± 0.01b |
5.1 ± 0.01 ab |
4.2 ± 0.05bc |
2.9 ± 0.01c |
2.6 ± 0.01c |
2.1 ± 0.01b |
2.1 ± 0.03b |
3.7 ± 0.03a |
4.1 ± 0.05b |
4.2 ± 0.01 ab |
4.7 ± 0.02b |
0 |
5.1 ± 0.05ab |
5.8 ± 0.01 ab |
5.4 ± 0.1abc |
4.7 ± 0.03a |
3.7 ± 0.01a |
3.5 ± 0.02a |
4.7 ± 0.02a |
3.2 ± 0.01a |
1.8 ± 0.00b |
1.2 ± 0.02b |
1.5 ± 0.02b |
1.1 ± 0.01a |
3.1 ± 0.01a |
3.4 ± 0.01 ab |
3.6 ± 0.04a |
3.9 ± 0.03 ab |
5 |
4.9 ± 0.01a |
5.4 ± 0.02a |
4.7 ± 0.02a |
4.5 ± 0.02a |
3.9 ± 0.03a |
3.5 ± 0.01a |
4.5 ± 0.03a |
3.6 ± 0.04 ab |
0.7 ± 0.01a |
0.3 ± 0.00 ab |
0.4 ± 0.00a |
0.2 ± 0.00a |
3.3 ± 0.02a |
3.1 ± 0.01a |
3.9 ± 0.03 ab |
3.4 ± 0.01a |
10 |
5.0 ± 0.04ab |
5.5 ± 0.01a |
4.9 ± 0.02ab |
4.9 ± 0.06a |
4.5 ± 0.01 ab |
4.1 ± 0.02 ab |
4.9 ± 0.01a |
3.5 ± 0.01 ab |
ND |
0.1 ± 0.00a |
ND |
ND |
3.7 ± 0.05a |
3.3 ± 0.05 ab |
4.1 ± 0.04 ab |
3.7 ± 0.01a |
15 |
5.4 ± 0.01abc |
5.9 ± 0.05 ab |
5.3 ± 0.01abc |
5.1 ± 0.01a |
4.9 ± 0.05bc |
4.7 ± 0.01b |
5.1 ± 0.02 ab |
3.9 ± 0.03a |
ND |
ND |
ND |
ND |
3.6 ± 0.01a |
2.9 ± 0.02a |
4.6 ± 0.05b |
3.5 ± 0.06a |
20 |
5.4 ± 0.05abc |
6.1 ± 0.03 ab |
5.1 ± 0.03abc |
5.1 ± 0.04a |
5.6 ± 0.06c |
5.3 ± 0.03bc |
5.9 ± 0.01bc |
4.7 ± 0.02 cd |
ND |
ND |
ND |
ND |
3.9 ± 0.04a |
2.7 ± 0.03a |
4.2 ± 0.06 ab |
3.1 ± 0.01a |
25 |
5.9 ± 0.02bc |
6.3 ± 0.01 ab |
5.7 ± 0.01bc |
5.0 ± 0.02a |
5.8 ± 0.02 cd |
5.9 ± 0.02 cd |
6.3 ± 0.06c |
5.2 ± 0.03d |
ND |
ND |
ND |
ND |
3.4 ± 0.01a |
3.1 ± 0.02a |
4.3 ± 0.01 ab |
3.8 ± 0.02 ab |
30 |
5.7 ± 0.01abc |
6.0 ± 0.05 ab |
5.9 ± 0.05c |
5.3 ± 0.05a |
6.6 ± 0.01d |
6.3 ± 0.02de |
6.1 ± 0.01c |
5.6 ± 0.01d |
ND |
ND |
ND |
ND |
3.3 ± 0.06a |
3.0 ± 0.02a |
4.6 ± 0.00b |
3.5 ± 0.02a |
35 |
6.1 ± 0.02bc |
6.6 ± 0.01b |
5.7 ± 0.04bc |
5.5 ± 0.06a |
6.5 ± 0.05d |
6.9 ± 0.01e |
6.1 ± 0.03c |
5.4 ± 0.05d |
ND |
ND |
ND |
ND |
3.4 ± 0.01a |
3.1 ± 0.03a |
4.4 ± 0.02 ab |
3.1 ± 0.01a |
Table 4 Total plate count, lactic acid bacteria count (LAB), coliform count and yeast and mold count of the dried vegetables over refrigerated (RF) storagea
Days |
Total plate count |
LAB count |
Coliform count |
Yeast and mold count |
ST |
TP |
TT |
JZ |
ST |
TP |
TT |
JZ |
ST |
TP |
TT |
JZ |
ST |
TP |
TT |
JZ |
The values are reported as mean ± standard deviation of triplicate determinations. ST: Samtawk, TP: Tawkpui, TT: Tawkte, JZ: Japan Zawngtah, ND: not detected. Values of each parameter marked with same superscripted alphabet do not vary significantly from one another.
|
Fresh |
5.7 ± 0.06d |
6.5 ± 0.02e |
5.7 ± 0.05f |
5.2 ± 0.01f |
4.3 ± 0.02a |
4.6 ± 0.01a |
5.1 ± 0.01a |
4.2 ± 0.05a |
2.9 ± 0.01c |
2.6 ± 0.01c |
2.1 ± 0.01b |
2.1 ± 0.03b |
3.7 ± 0.03e |
4.1 ± 0.05e |
4.2 ± 0.01d |
4.7 ± 0.02f |
0 |
5.1 ± 0.05d |
5.8 ± 0.01de |
5.4 ± 0.01f |
4.7 ± 0.03f |
3.7 ± 0.01a |
3.5 ± 0.02a |
4.7 ± 0.02a |
3.2 ± 0.01a |
1.8 ± 0.00b |
1.2 ± 0.02b |
1.5 ± 0.02 ab |
1.1 ± 0.01a |
3.1 ± 0.01de |
3.4 ± 0.01cde |
3.6 ± 0.04d |
3.9 ± 0.03ef |
5 |
4.9 ± 0.01d |
5.8 ± 0.07de |
5.1 ± 0.05ef |
4.5 ± 0.01ef |
3.9 ± 0.08a |
3.5 ± 0.03a |
4.5 ± 0.04a |
3.6 ± 0.02a |
0.3 ± 0.01a |
0.1 ± 0.00a |
0.7 ± 0.00a |
0.4 ± 0.01a |
3.5 ± 0.03de |
3.6 ± 0.07de |
3.7 ± 0.01d |
3.4 ± 0.01de |
10 |
3.1 ± 0.01c |
5.1 ± 0.02d |
4.4 ± 0.03de |
3.7 ± 0.07de |
4.1 ± 0.01a |
3.7 ± 0.01a |
4.7 ± 0.01a |
3.5 ± 0.02a |
ND |
ND |
ND |
ND |
3.3 ± 0.5de |
3.4 ± 0.01cde |
3.6 ± 0.01d |
3.1 ± 0.02de |
15 |
2.8 ± 0.02c |
3.6 ± 0.01c |
3.9 ± 0.03d |
3.1 ± 0.04d |
3.9 ± 0.02a |
4.0 ± 0.02a |
4.5 ± 0.03a |
3.9 ± 0.06a |
ND |
ND |
ND |
ND |
2.7 ± 0.01 cd |
3.3 ± 0.06cde |
2.4 ± 0.03c |
2.7 ± 0.01 cd |
20 |
2.4 ± 0.02bc |
2.7 ± 0.01bc |
2.2 ± 0.07c |
2.1 ± 0.02c |
4.0 ± 0.03a |
3.9 ± 0.01a |
4.6 ± 0.01a |
3.7 ± 0.01a |
ND |
ND |
ND |
ND |
1.9 ± 0.01bc |
3.0 ± 0.02bcd |
2.1 ± 0.03bc |
2.0 ± 0.02bc |
25 |
1.7 ± 0.01b |
1.9 ± 0.02b |
1.7 ± 0.01bc |
1.8 ± 0.01bc |
4.3 ± 0.01a |
3.6 ± 0.02a |
4.9 ± 0.04a |
4.0 ± 0.02a |
ND |
ND |
ND |
ND |
1.3 ± 0.01 ab |
2.5 ± 0.01bc |
1.4 ± 0.05 ab |
1.4 ± 0.04 ab |
30 |
0.5 ± 0.01a |
0.8 ± 0.02a |
1.1 ± 0.02 ab |
0.9 ± 0.01 ab |
4.1 ± 0.01a |
3.7 ± 0.03a |
4.7 ± 0.05a |
4.0 ± 0.03a |
ND |
ND |
ND |
ND |
0.7 ± 0.02a |
2.1 ± 0.03 ab |
0.8 ± 0.00a |
1.1 ± 0.01 ab |
35 |
0.3 ± 0.01a |
0.6 ± 0.01a |
0.5 ± 0.02a |
0.3 ± 0.01a |
4.1 ± 0.02a |
3.9 ± 0.01a |
4.5 ± 0.03a |
3.9 ± 0.03a |
ND |
ND |
ND |
ND |
0.5 ± 0.02a |
1.4 ± 0.04a |
0.5 ± 0.01a |
0.7 ± 0.01a |
This indicated that vacuum packaging individually did not pose a significant hurdle for the microbial population. A similar observation was made by Beltrán et al. (2005) where potato strips sanitized with ozonated water had higher shelf life than the strips packaged directly in vacuum-sealed bags.34 Tian et al. (2013) reported that the survival of pathogenic E. coli O157:H7 in minimally processed vegetables was influenced by their storage temperature.35 Lower temperatures were associated with slower metabolic rates and reduced cellular activity in microbial species, in particular mesophilic bacteria and fungus that thrive within the temperature range of 25 °C to 40 °C. Vacuum would have certainly reduced the chances of aerobic bacterial and fungal species to establish in the WEVs. Populations of anaerobic LAB remained stable at RF storage, whereas their populations were observed to increase over storage time at RT. Refrigeration temperature reduces the cellular metabolism of LAB.36
3.5. Texture
The hardness of the brined vegetables exhibited a decreasing trend over storage time (Fig. 3). This decline was sharpest for JZ, which showed the maximum hardness (1402.37 g) on the initial day followed by a steep decline (to 132.45 g) over the 35 days of storage. ST, TP and TT showed a decrease from 168.54 g to 46.02 g, 1125.92 g to 76.54 g and 230.06 g to 68.20 g, respectively. Pectinolytic enzymes predominantly cause tissue degradation and softening over brined storage. Moreover, given their classification as high-moisture foods, the moisture content is integral to maintaining the inherent firmness of vegetable produces. Hence, a decrease in the moisture content values post brining is also associated with the reduction in the firmness of the vegetable tissues, as indicated by their lower hardness values. Mocanu et al. (2022) and McMurtrie and Johanningsmeier (2018) reported a decrease in the firmness of green pickled tomatoes and brine fermented cucumbers, respectively.37,38 The concentration of sodium in brine or pickling medium also plays a critical role in firmness retention.38 Additionally, the use of sodium salts, compared to magnesium or potassium salts exerts an increased antimicrobial effect, inhibiting the development of spoilage microbes and promoting the production of exopolygalacturonase, thereby retaining tissue firmness.37
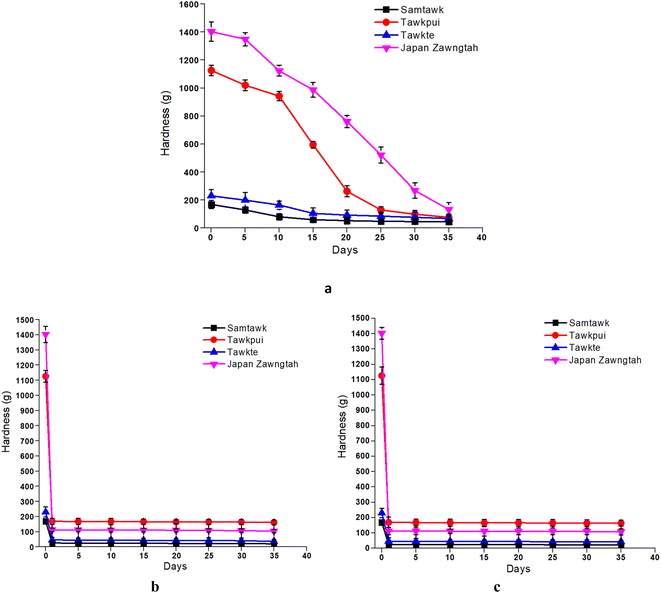 |
| Fig. 3 Hardness of the brined vegetables (a) and dried vegetables over RT (b) and RF (c) storage. | |
Upon drying (Fig. 4), ST, TP, TT, and JZ demonstrated a decrease in hardness from 168.54 g, 1125.92 g, 230.06 g and 1402.37 g to 25.42 g, 168.73 g, 45.98 g and 112.18 g, respectively (Fig. 3). Dried vegetables showed lower hardness values requiring a lower deformation force, which can also be termed as developed ‘softness’ of the vegetable pulp, as reported by Guiné and Barroca (2012).26 Both RF and RT storage led to a further decrease in the hardness of the dried samples. Comparatively, RF samples retained hardness more than RT samples. A similar trend of softness development at higher temperatures due to gradual shrinkage of the dried tissues has also been reported in dried pumpkin and bell peppers.26,39
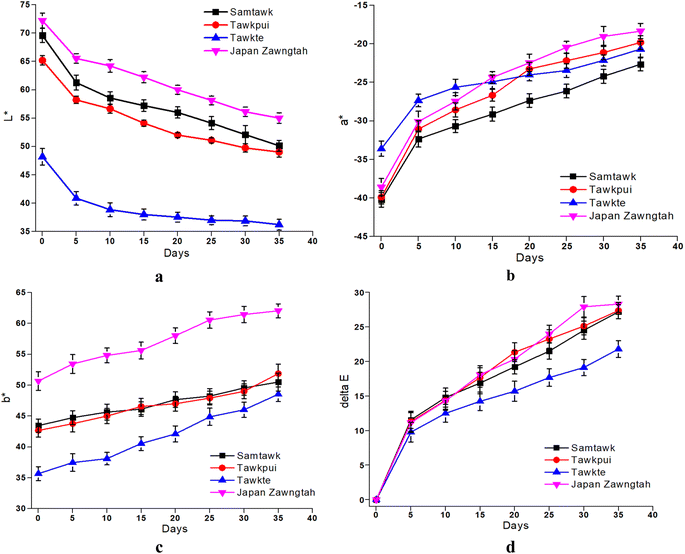 |
| Fig. 4 Color values L* (a), a* (b), b* (c) and ΔE (d) of brined vegetables over storage. | |
3.6. Color
The changes in L*, a*, b* and ΔE values of the brined samples are depicted in Fig. 4 and those of dried samples in Fig. 5. Gradually reducing L* values suggested loss of surface luster over brined storage. The general visual observation of the samples turning reddish brown was supported by increased a*, b* and ΔE. The acidic pH generated in the brine induced chemical oxidation of the phytochemical compounds, leading to production of brown o-quinones, which took over the green color of the fruits. A low-pH environment also produces magnesium-free chlorophyll derivative compounds, contributing to the loss of green pigments in the fruits.15 TP showed the most prominent decrease in L* for both brined and dried samples. Drying significantly decreased the L* values from 69.58, 65.20, 48.21, and 72.20 in raw ST, TP, TT, and JZ to 50.24, 48.23, 35.23 and 56.54, respectively. High-temperature drying resulted in simultaneous degradation of natural pigments such as chlorophyll, partial oxidation of phenolics and formation of Maillard reaction compounds such as melanoidins.40 These compounds generated are known to possess abundant antioxidant activity. Studies suggest a correlation between the generation of melanoidins, often associated with the Maillard reaction, and the development of compounds exhibiting antioxidant properties. Consequently, the increase in brown coloration (depicted by the a* value) has a positive influence in the antioxidant activity, which can be confirmed with the increase in DRSA values after processing (Fig. 7 and Section 3.8). Shrinkage also resulted in the concentration of pigments per unit mass of the dried samples. Increased a*, increased b* and ΔE values more than 1 were indicative of decomposed chlorophyll and increased browning due to drying. Vacuum-packaging prevented further pigment oxidation as suggested by insignificant color changes (ΔE) during 35 days of RF and RT storage.
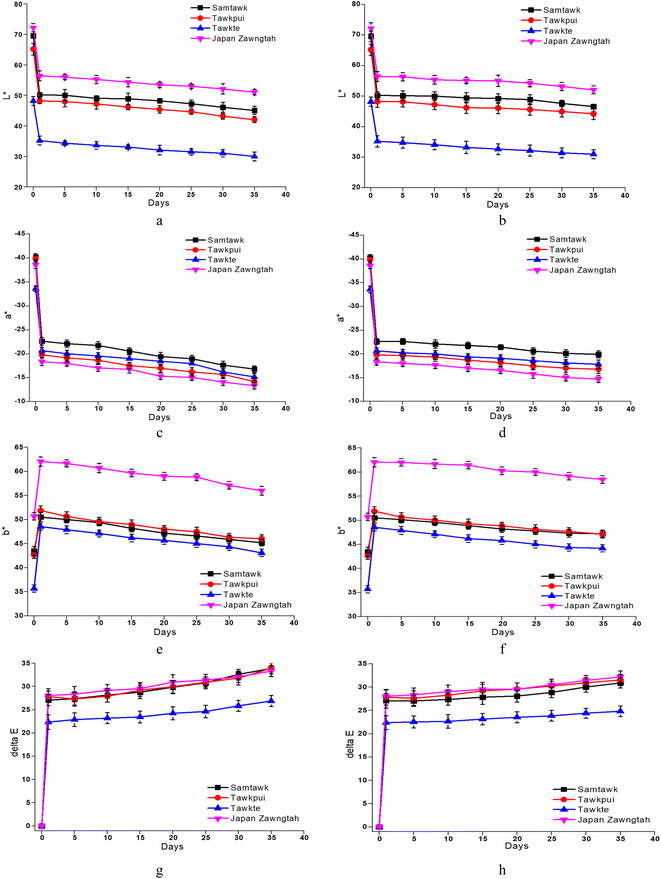 |
| Fig. 5 Color values L*, a*, b* and ΔE of dried vegetables over RT (a, c, e and g) and RF (b, d, f and h) storage. | |
3.7. Total phenolics and flavonoid contents
Both the TPC and the TFC increased in the brined WEVs (Fig. 6). The increase in TPC was 65% in both ST and TP, 30% in TT and 15% in JZ. The TFC increase was 55% in ST, 95% in TP, 70% in TT and 25% in JZ as compared to their respective raw samples. Although peculiar, such increase in the concentrations of bioactive compounds have also been reported in carrots,41 daikon radish and red cabbage ferment mix,42 dried fig fruit juice fermented using LAB culture,43 in individually fermented red, white and Chinese cabbage with 3% salt at 18 °C for 30 days,21 and in market pickled commercial chayote.25 Moreover, Irakoze et al. (2023) reported such increase in fermented African Black Nightshade and African Spider Plant.16 These are attributed to the enzyme catalyzed changes caused by lacto fermentation. Gradual accumulation of lactic acid in the stored brine was indicative of the increase in LAB. LABs produce enzymes such as tannase, amylase, esterase, β-glucosidase, phenolic acid decarboxylase (PAD), reductase, and benzyl alcohol dehydrogenase, which can biologically transform phenolic compounds and also increase their concentration.44 The enzymes help in the breakdown of the ester linkages between the phenolic acid polymers, which, in turn, releases free phenolic acids. Polyphenolic compounds such as hydroxycinnamic acids are present in glycosylated or esterified forms in plants. Esterified polyphenolic compounds such as hydroxycinnamoyl esters are hydrolyzed by cinnamoyl ester hydrolases (also known as cinnamoyl esterase), resulting in the release of free phenolic acids.45 In a study on fermentation of avocado leaves,44 the profile of the phenolic compounds, namely, kaempferol, rutin and dehydro-p-coumaric acid was modified due to bacterial metabolism by Pediococcus pentosaceus CECT 4695T, Levilactobacillus brevis CECT 5354, Pediococcus acidilactici CECT 5765T and Lactiplantibacillus plantarum CECT 9567. This significantly increased TPC by 71, 62, 55 and 21%, respectively. Additionally, the low pH stabilizes the native and formed phenolics and renders them quantifiable.15,43 Multiple studies have confirmed the association of the stability of phenolic compounds with low pH.14,16,41
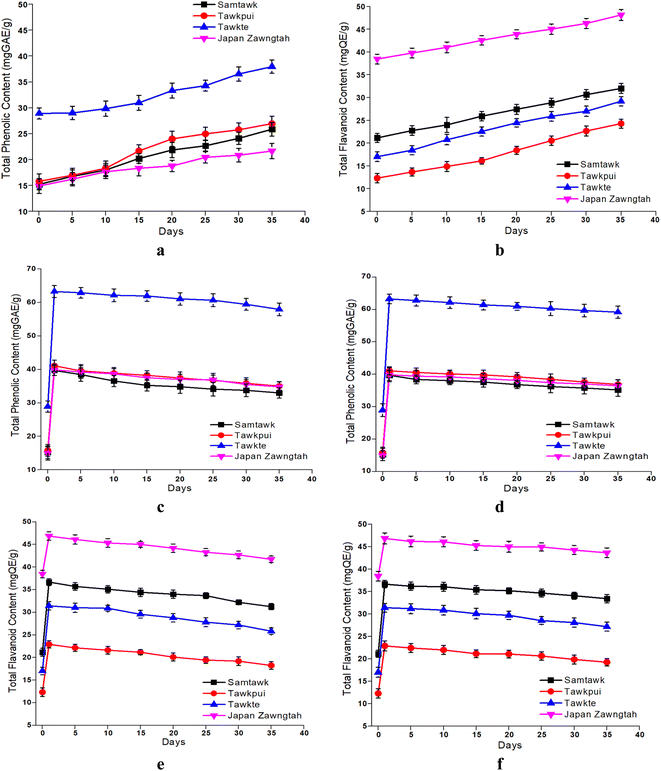 |
| Fig. 6 TPC and TFC of brined (a and b) and dried vegetables over RT (c and e) and RF (d and f) storage. | |
Lactobacillus species, with the help of predominant enzyme systems and the lactic acid formed, induce transformation of phenolic compounds, improving the bioavailability and extractability of these inherent phenolic compounds. During gastrointestinal digestion, phenolic compounds are subject to significant metabolic transformations by xenobiotic enzymes prevalent in the liver and enterocytes. This metabolic process results in their breakdown and subsequent excretion through bile, feces, and urine, leading to a reduction in their bioavailability. Some phenolics such as caffeic acid and chlorogenic acid exhibit remarkable absorption properties. Studies have demonstrated that following enzymatic hydrolysis, approximately 95% of caffeic acid and 33% of chlorogenic acid are absorbed in the stomach and small intestine.46 Chlorogenic acid, specifically, possesses the ability to traverse the gastrointestinal tract and stimulate the proliferation of Bifidobacterium species, thereby contributing to gut health.47 Bioavailability of phenolic acids, namely, gallic, vanillic, 2,5-dihydroxybenzoic, coumaric, ferulic and ellagic acids and flavonoids, namely, catechin, quercetin and luteolin were found to be improved after the fermentation of nightshade leaves with L. plantarum75.14 A decline in phenolic compounds was observed in post gastrointestinal digestion of Solanum nigrum.48 However, even with this reduction, their bioactivity against oxidative stress and prevention of DNA oxidative damage remained potent.
Enhanced tissue brittleness in dried vegetables caused higher cellular breakdown during grinding, aiding in the release of the sequestrated phenolics and their higher extractability.27 Thermal depolymerization of cell wall lignin results in easier release of these low-molecular weight monomeric phenolic acid derivatives such as phenol, guaiacol, vanillin, syringol, apocynin, guaiacyl acetone, vanillyl alcohol, syringaldehyde and desasppidinol.49,50 This resulted in increased TPC and TFC values (Fig. 6). Thermal conversion of single high-molecular weight phenolic compounds into more low-molecular weight phenolic compounds could be another reason.51 This conversion is explained by the esterification of phenolic compounds and inherent acids in the vegetables under heat, promoting the formation of phenolic acid esters. Studies also suggest that thermal treatment helps in the degradation of complex compounds like hydrolysable tannins and phenol–protein complex, leading to an increase the content of phenolics such as gallic, caffeic, ferulic, p-coumaric, sinapic, cinnamic and chlorogenic acids.52 Such transformation of phenolic compounds from unextractable to their extractable forms could have further enhanced their quantifiability. Rodríguez et al. (2014) reported lower loss of TPC at higher temperatures in apples.53 Highest loss in TPC was observed at 30 °C (38.7 ± 1.6%) followed by 50 °C (26.5 ± 1.9%), while minimal loss in TPC was observed at 70 °C (19.7 ± 1.6%). The shelf life of vegetables stored under vacuum demonstrates a higher phytochemical retention due to the absence of oxygen in the package's environment.29 Low temperature is associated with the retention of plant phytochemicals, principally due to the lower volatility of bioactive molecules.54 Therefore, dried samples stored at RF showed marginally higher retention of TPC and TFC than RT stored samples.
3.8. Antioxidant activity
Both DRSA and FRAP studies indicated increased antioxidant potential of the vegetables over brining and storage which was in accordance with the enhanced TPC and TFC (Fig. 7). Another possible explanation for the increased antioxidant activity in stored brined samples could be the free radical scavenging capacity of LAB through their inherent enzymatic and non-enzymatic mechanisms.55 Significantly higher DRSA than raw WEVs were hence observed, amounting 87.86% to 94.23% in ST, 69.86% to 79.54% in TP, 89.72% to 95.23% in TT and 77.32% to 86.37% in JZ. Likewise, almost 99% increase was observed in FRAP values in all the brined samples over raw samples. Sayin and Alkan (2015) observed similar changes upon pickling 10 vegetables over a period of 60 days.56 As carried out in this study, brief blanching treatments prior to brining can improve the antioxidative potentials of vegetables. In a study by Managa et al. (2020), the concentrations of chlorogenic, neochlorogenic, cryptochlorogenic and caffeoylmalic acids were elevated upon blanching of Chinese cabbage (Brassica rapa L. subsp. Chinensis) and African nightshade (Solanum retroflexum Dun.) leaves at 95 °C for 5 min.57 Similarly, the concentration of chlorogenic acid was increased after blanching in the leaves of Solanum retroflexum Dun. attributed to the hydrolysis of dicaffeoylquinic acid and formation of different caffeoylquinic acid isomers.58
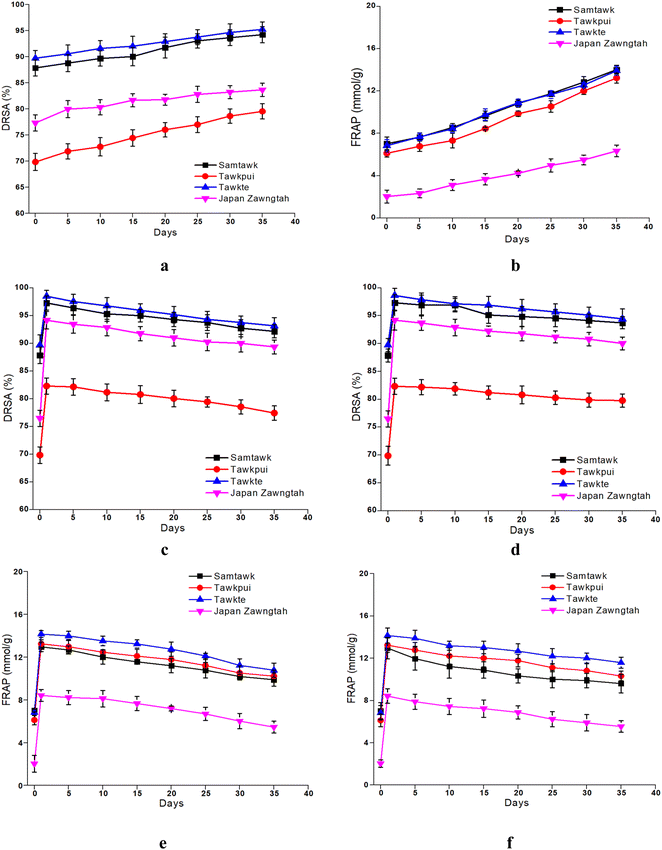 |
| Fig. 7 DRSA and FRAP of brined (a and b) and dried vegetables over RT (c and e) and RF (d and f) storage. | |
Drying increased DRSA of ST by 10%, TP by 28%, TT by 10% and JZ by 19%. Similarly, FRAP showed a significant increase up to 95% in the vegetables (Fig. 7), which is due to the enhancement of TPC and TFC. This could be associated with the thermal inhibition of peroxidase (POD), polyphenol oxidase (PPO), pectin methyl esterase (PME) and ascorbate oxidase (AO) enzymes at high drying temperatures.59 Higher antioxidant activities of dried vegetables could also be a result of partially oxidized polyphenols and formation of Maillard reaction products (MRPs) upon heat treatment followed by storage.60 These generated partially oxidized polyphenols are known to possess increased antioxidant activity compared to the non-oxidized polyphenolic compounds. Lutz and Hernández Carolina (2015) reported higher content of TPC upon drying of apples at temperatures ranging from 40 °C to 130 °C compared to the fresh ones, which was claimed to be due to the cellular breakdown and release of phenolic compounds from the matrix as well as formation of MRPs.61 The MRP synthesis processes produce more electron and hydrogen donors such as melanoidins. Due to their anionic nature, these melanoidins show sequestrating behaviors and can scavenge free radicals to terminate oxidation, thereby enhancing the antioxidative potential.62 The increase in the a* values is depictive of the increase in the redness of the WEV samples caused by the formation of these MRPs. Concurrent reasons were also depicted in studies on Prunus domestica,63Malus domestica,53 Zingiber officinale Roscoe,64Myrtus communis L. leaves52 and Solanum paniculatum65 amongst others.
Vacuum-packaging resulted in the retention of antioxidant components in the dried vegetables due to lack of oxygen, thereby preventing them from oxidation.66 However, DRSA and FRAP decreased progressively upon storage at RT and RF (Fig. 7). These reductions in antioxidant activity could be attributed to the enzymatic decomposition of phenolic compounds, oxidation of constitutive phenolic compounds, moisture loss and change in the total soluble solids. Additionally, higher retention of phenolic compounds and the increase in the antioxidant activity during refrigerated storage could be due to the biosynthesis of phenolics by enzymes in the cold environment.67
Many studies have emphasized the advantageous health impacts of consuming antioxidant-rich foods. These benefits extend to lowering the risk of non-communicable diseases and mitigating the effects of premature aging. As discussed earlier, the ability of chlorogenic acid to surpass the gastrointestinal tract and its high absorptive properties, its increased intake and thereby absorption has been suggested to reduce the risk of type 2 diabetes mellitus.68 The mechanism through which chlorogenic acid exerts its influence is by inhibiting α-glucosidase, an enzyme involved in carbohydrate digestion, thereby helping to regulate blood sugar levels. This occurs through the activation of adenosine monophosphate-activated protein kinase (AMPK), a key regulator of cellular energy metabolism. By activating AMPK, chlorogenic acid helps in modulating glucose and lipid metabolism and stimulating glucose uptake in skeletal muscle, which aids in regulating blood sugar.68 Therefore, this component helps in the prevention of diabetes by suppressing postprandial hyperglycemia. Solanum species have been reported to possess anticancer, antioxidant, antidepressant, antihypertensive, anti-inflammatory, hepatoprotective, anti-obesogenic, hypoglycemic, hypolipidemic and antidiabetic properties, due to the presence of phenolic compounds myricetin, quercetin-3-O-robinoside, 3-caffeoylquinic acid, 3,4-dicaffeoylquinic acid and rutin and alkaloids, saponins, terpenes, flavonoids, coumarins, and carotenoids.47 However, proper investigation into the mechanisms underlying the above-mentioned effects is crucial.
In vitro studies on dried leaf extracts of Solanum scabrum have confirmed chemo-protective properties against aflatoxin, the prominent carcinogen present in the liver by showing anti-genotoxicity against it.69 The change in the composition of the inherent phenolic compounds after processing might have been attributed to the increase in antioxidant activity, thereby confirming their chemoprotective effects.
4. Conclusions
The two minimal preservation techniques, namely, brining and drying and subsequent storage could successfully improve the shelf lives of the four lesser known WEVs by suppressing the growth of spoilage microorganisms. Interestingly, enhanced phenolic content and their imparted antioxidant activities were prominent for each preserved vegetable. The preservation methods can be up-scaled and adopted by the tribal growers for storage of the WEVs, which, in turn, would help in the reduction of food wastage and profit generation through sale of the preserved products. Modern preservation techniques such as non-thermal processes, modified atmospheric packaging, canning and improved sterilization processes can be adopted. However, considering the economic feasibility, practicality, and implementation in the forest-rich region where these crops have been ethnically cultivated, shelf-life enhancement through natural preservation techniques remains the most feasible possibility. Hence, in developing countries like Africa, Tunisia, Namibia, India, and Myanmar, where wild edible produces are plentiful, traditional preservation methods such as brining, pickling, and drying remain the most viable options. Thus, research in a similar line is evidenced and therefore provided in this study. Advocating these processing techniques to meet the global sustainability goals of combatting poverty, ensuring nutrition and safety is crucial. Studies related to the development of functional powders from dried WEVs to combat non-communicable and metabolic diseases affirm their applicability in the nutraceutical and pharmaceutical industries. Encapsulation and drug delivery systems and mechanisms to ensure maximum bioavailability are vital in this regard and should be taken up. Implementing the sustainable processing techniques and directing the wild edible produce to the food, nutraceutical and pharmaceutical industries can play a pivotal role in the economic growth and development of native communities.
Author contributions
Gunjana Deka; investigation, data curation, formal analysis, writing – original draft. Sayantan Chakraborty; investigation, data curation, writing – original draft. Madhu Kumari; resources, data curation. HC Lalbiakdiki; resources, data curation. Tridip Kumar Hazarika; methodology. Himjyoti Dutta; funding acquisition, review and editing, visualization, methodology, conceptualization, supervision.
Conflicts of interest
The authors declare no conflicts of interest relevant to this article.
References
-
Food and Agriculture Organization, The state of food security and nutrition in the world 2023: Urbanization, agrifood system transformation and healthy diets across the rural-urban continuum (Food & Agriculture Organization), in Food & Agriculture Organization of the United Nations, FAO, 2023 Search PubMed.
- V. P. Sati and L. Vangchhia, Food security status in rural areas of Mizoram, Northeast India, J. Mt. Sci., 2017, 14(4), 795–805, DOI:10.1007/s11629-016-4092-2.
- R. Lalmuanpuii, B. Zodinpuii and J. Lalbiaknunga, Zothanpuia. Traditional food preparation of wild edible vegetables among the ethnic groups of Mizoram, Northeast India, J. Ethn. Foods, 2021, 8, 1–11, DOI:10.1186/s42779-021-00104-5.
- L. Ralte, U. Bhardwaj and Y. T. Singh, Traditionally used edible Solanaceae plants of Mizoram, India have high antioxidant and antimicrobial potential for effective phytopharmaceutical and nutraceutical formulations, Heliyon, 2021, 7(9), e07907, DOI:10.1016/j.heliyon.2021.e07907.
- R. A. Abdulkadir, N. Mat, M. M. Hasan and S. M. Jahan, In vitro antioxidant activity of the ethanolic extract from fruit, stem, and leaf of Solanum torvum, ScienceAsia, 2016, 42(3), 184, DOI:10.2306/scienceasia1513-1874.2016.42.184.
- C. N. Ekweogu, V. C. Ude, P. Nwankpa, O. Emmanuel and E. A. Ugbogu, Ameliorative effect of aqueous leaf extract of Solanum aethiopicum on phenylhydrazine-induced anaemia and toxicity in rats, Toxicol. Res., 2020, 36(3), 227–238, DOI:10.1007/s43188-019-00021-5.
- N. Satyanarayana, S. V. Chinni, R. Gobinath, P. Sunitha, A. Uma Sankar and B. S. Muthuvenkatachalam, Antidiabetic activity of Solanum torvum fruit extract in streptozotocin-induced diabetic rats, Front. Nutr., 2022, 9, 987552, DOI:10.3389/fnut.2022.987552.
-
B. K. Singh, K. A. Pathak, and Y. Ramakrishna, Underutilized Vegetable Crops and Spices of Mizoram: Needs Exploration and Utilization. Developing the Potential of Underutilized Horticultural Crops of Hill Regions, Today & Tomorrow's Printers and Publishers, 2013 Search PubMed.
- N. N. Mbondo, W. O. Owino, J. Ambuko and D. N. Sila, Effect of drying methods on the retention of bioactive compounds in African eggplant, Food Sci. Nutr., 2018, 6(4), 814–823, DOI:10.1002/fsn3.623.
- M. G. Mokganya and M. P. Tshisikhawe, Medicinal uses of selected wild edible vegetables consumed by Vhavenda of the Vhembe District Municipality, South Africa, S. Afr. J. Bot., 2019, 122, 184–188, DOI:10.1016/j.sajb.2018.09.029.
- L. Sergio, F. Boari, M. Pieralice, V. Linsalata, V. Cantore and D. Di Venere, Bioactive phenolics and antioxidant capacity of some wild edible greens as affected by different cooking treatments, Foods, 2020, 9(9) DOI:10.3390/foods9091320.
- W. Batool, W. Hussain, R. Hassan, T. Tasleem, S. Ullah and A. Ali, A study of wild vegetables: proximate and mineral analysis of selected wild edible vegetables of Parachinar, district Kurram KP, Pakistan, Pak. J. Bot., 2021, 55(1), 291–298, DOI:10.30848/pjb2023-1(21).
-
B. Agyei-Poku, The Effect of Pre-Treatment and Oven Drying Temperatures on the Nutritional, Anti-nutritional Values and Colour Properties of the Fruits of Solanum Torvum, 2017, https://ir.knust.edu.gh/handle/123456789/11444 Search PubMed.
- A. Degrain, V. Manhivi, F. Remize, C. Garcia and D. Sivakumar, Effect of lactic acid fermentation on color, phenolic compounds and antioxidant activity in African nightshade, Microorganisms, 2020, 8(9), 1324, DOI:10.3390/microorganisms8091324.
- N. Helilusiatiningsih, Sumarji and R. A. Shobirin, The influence of blanching and drying process towards extract of bioactive compounds in Solanum torvum and its antioxidant activities, Formosa Journal of Multidisciplinary Research, 2023, 2(4), 795–808, DOI:10.55927/fjmr.v2i4.4005.
- M. L. Irakoze, E. N. Wafula and E. E. Owaga, Effect of lactic acid fermentation on phytochemical content, antioxidant capacity, sensory acceptability and microbial safety of African black nightshade and African spider plant vegetables, Bacteria, 2023, 2(1), 48–59, DOI:10.3390/bacteria2010004.
- G.-D. Mocanu, O.-V. Nistor, O. E. Constantin, D. G. Andronoiu, V. V. Barbu and E. Botez, The effect of sodium total substitution on the quality characteristics of green pickled tomatoes (Solanum lycopersicum L.), Molecules, 2022, 27(5), 1609, DOI:10.3390/molecules27051609.
- M. McSweeney and K. Seetharaman, State of polyphenols in the drying process of fruits and vegetables, Crit. Rev. Food Sci. Nutr., 2015, 55(5), 660–669, DOI:10.1080/10408398.2012.670673.
- S. Kamiloglu, G. Toydemir, D. Boyacioglu, J. Beekwilder, R. D. Hall and E. Capanoglu, A review on the effect of drying on antioxidant potential of fruits and vegetables, Crit. Rev. Food Sci. Nutr., 2016, 56(sup1), S110–S129, DOI:10.1080/10408398.2015.1045969.
- Z. Shang, M. Li, W. Zhang, S. Cai, X. Hu and J. Yi, Analysis of phenolic compounds in pickled chayote and their effects on antioxidant activities and cell protection, Food Res. Int., 2022, 157(111325), 111325, DOI:10.1016/j.foodres.2022.111325.
- R. B. Parada, E. Marguet, C. A. Campos and M. Vallejo, Improving the nutritional properties of Brassica L. vegetables by spontaneous fermentation, Foods Raw Mater., 2022, 97–105, DOI:10.21603/2308-4057-2022-1-97-105.
-
AOAC International, Official Methods of Analysis of AOAC INTERNATIONAL: 3-Volume Set, ed. Latimer Jr G. W., Oxford University Press, 2023 Search PubMed.
- S. Aryal, M. K. Baniya, K. Danekhu, P. Kunwar, R. Gurung and N. Koirala, Total phenolic content, flavonoid content and antioxidant potential of wild vegetables from western Nepal, Plants, 2019, 8(4), 96, DOI:10.3390/plants8040096.
- M. K. Alam, Z. H. Rana, S. N. Islam and M. Akhtaruzzaman, Comparative assessment of nutritional composition, polyphenol profile, antidiabetic and antioxidative properties of selected edible wild plant species of Bangladesh, Food Chem., 2020, 320(126646), 126646, DOI:10.1016/j.foodchem.2020.126646.
- M. G. Managa, S. A. Akinola, F. Remize, C. Garcia and D. Sivakumar, Physicochemical Parameters and Bioaccessibility of Lactic Acid Bacteria Fermented Chayote Leaf (Sechium edule) and Pineapple (Ananas comosus) Smoothies, Front. Nutr., 2021, 8, 1–14, DOI:10.3389/fnut.2021.649189.
- R. P. F. Guiné and M. J. Barroca, Effect of drying treatments on texture and color of vegetables (pumpkin and green pepper), Food Bioprod. Process., 2012, 90(1), 58–63, DOI:10.1016/j.fbp.2011.01.003.
- S. A. Al Maiman, N. A. Albadr, I. A. Almusallam, M. J. Al-Saád, S. Alsuliam and M. A. Osman,
et al., The potential of exploiting economical solar dryer in food preservation: Storability, physicochemical properties, and antioxidant capacity of solar-dried tomato (Solanum lycopersicum) fruits, Foods, 2021, 10(4), 734, DOI:10.3390/foods10040734.
- N. A. Razak, N. A. Hamid and A. R. Shaari, Effect of storage temperature on moisture content of encapsulated Orthosiphon stamineus spray-dried powder, AIP Conf. Proc., 2018, 2030, 020194, DOI:10.1063/1.5066835.
- N. A. Razak, N. A. Hamid and A. R. Shaari, Effect of vacuum and non-vacuum packaging on total phenolic content of encapsulated Orthosiphon stamineus spray-dried powder during storage, Solid State Phenom., 2018, 280, 330–334, DOI:10.4028/www.scientific.net/ssp.280.330.
- S. Akoth, A. Nuwagira, S. Byakika and I. M. Mukisa, Pickling as a preservation technique for Solanum aethiopicum; An edible green leafy vegetable, J. Adv. Food Sci. Technol., 2023, 66–73, DOI:10.56557/jafsat/2023/v10i38347.
- H. Liang, Z. He, X. Wang, G. Song, H. Chen and X. Lin,
et al., Effects of salt concentration on microbial diversity and volatile compounds during suancai fermentation, Food Microbiol., 2020, 91(103537), 103537, DOI:10.1016/j.fm.2020.103537.
- Y. Tang, G. Chen, D. Wang, R. Hu, H. Li and S. Liu,
et al., Effects of dry-salting and brine-pickling processes on the physicochemical properties, nonvolatile flavour profiles and bacterial community during the fermentation of Chinese salted radishes, LWT, 2022, 157(113084), 113084, DOI:10.1016/j.lwt.2022.113084.
- S. Bourdoux, D. Li, A. Rajkovic, F. Devlieghere and M. Uyttendaele, Performance of drying technologies to ensure microbial safety of dried fruits and vegetables, Compr. Rev. Food Sci. Food Saf., 2016, 15(6), 1056–1066, DOI:10.1111/1541-4337.12224.
- D. Beltrán, M. V. Selma, J. A. Tudela and M. I. Gil, Effect of different sanitizers on microbial and sensory quality of fresh-cut potato strips stored under modified atmosphere or vacuum packaging, Postharvest Biol. Technol., 2005, 37(1), 37–46, DOI:10.1016/j.postharvbio.2005.02.010.
- J.-Q. Tian, Y.-M. Bae and S.-Y. Lee, Survival of foodborne pathogens at different relative humidities and temperatures and the effect of sanitizers on apples with different surface conditions, Food Microbiol., 2013, 35(1), 21–26, DOI:10.1016/j.fm.2013.02.004.
- O. Alegbeleye, O. A. Odeyemi, M. Strateva and D. Stratev, Microbial spoilage of vegetables, fruits and cereals, Appl. Food Res., 2022, 2(1), 100122, DOI:10.1016/j.afres.2022.100122.
- G.-D. Mocanu, O.-V. Nistor, O. E. Constantin, D. G. Andronoiu, V. V. Barbu and E. Botez, The effect of sodium total substitution on the quality characteristics of green pickled tomatoes (Solanum lycopersicum L.), Molecules, 2022, 27(5), 1609, DOI:10.3390/molecules27051609.
- E. K. McMurtrie and S. D. Johanningsmeier, Quality of cucumbers commercially fermented in calcium chloride brine without sodium salts, J. Food Qual., 2018, 2018, 1–13, DOI:10.1155/2018/8051435.
- R. P. F. Guiné and M. J. Barroca, Effect of drying on the textural attributes of bell pepper and pumpkin, Drying Technol., 2011, 29(16), 1911–1919, DOI:10.1080/07373937.2011.596297.
- S. M. Oliveira, T. R. S. Brandão and C. L. M. Silva, Influence of drying processes and pretreatments on nutritional and bioactive characteristics of dried vegetables: A review, Food Eng. Rev., 2016, 8(2), 134–163, DOI:10.1007/s12393-015-9124-0.
- J. P. Tamang, D.-H. Shin, S.-J. Jung and S.-W. Chae, Functional properties of microorganisms in fermented foods, Front. Microbiol., 2016, 7, 1–13, DOI:10.3389/fmicb.2016.00578.
- S. Vatansever, A. Vegi, J. Garden-Robinson and C. A. Hall III, The effect of fermentation on the physicochemical characteristics of dry-salted vegetables, J. Food Res., 2017, 6(5), 32, DOI:10.5539/jfr.v6n5p32.
-
E. D. Wijayanti, N. C. E. Setiawan, and J. P. Christi, Effect of lactic acid fermentation on total phenolic content and antioxidant activity of fig fruit juice (ficus carica), in Proceedings of the Health Science International Conference (HSIC 2017), Paris, France, Atlantis Press, 2017 Search PubMed.
- S. De Montijo-Prieto, M. d. C. Razola-Díaz, F. Barbieri, G. Tabanelli, F. Gardini and M. Jiménez-Valera,
et al., Impact of lactic acid bacteria fermentation on phenolic compounds and antioxidant activity of avocado leaf extracts, Antioxidants, 2023, 12(2), 298, DOI:10.3390/antiox12020298.
-
R. Muñoz, B. de las Rivas, F. López de Felipe, I. Reverón, L. Santamaría, M. Esteban-Torres, et al., Biotransformation of Phenolics by Lactobacillus plantarum in Fermented Foods, in Fermented Foods in Health and Disease Prevention, Elsevier, 2017, pp. 63–83 Search PubMed.
- F. Tomas-Barberan, R. García-Villalba, A. Quartieri, S. Raimondi, A. Amaretti and A. Leonardi,
et al., In vitro transformation of chlorogenic acid by human gut microbiota, Mol. Nutr. Food Res., 2014, 58(5), 1122–1131, DOI:10.1002/mnfr.201300441.
- C. A. Elizalde-Romero, L. A. Montoya-Inzunza, L. A. Contreras-Angulo, J. B. Heredia and E. P. Gutiérrez-Grijalva, Solanum fruits: Phytochemicals, bioaccessibility and bioavailability, and their relationship with their health-promoting effects, Front. Nutr., 2021, 8, 1–9, DOI:10.3389/fnut.2021.790582.
- S. M. Moyo, J. C. Serem, M. J. Bester, V. Mavumengwana and E. Kayitesi, The impact of boiling and in vitro human digestion of Solanum nigrum complex (Black nightshade) on phenolic compounds bioactivity and bioaccessibility, Food Res. Int., 2020, 137(109720), 109720, DOI:10.1016/j.foodres.2020.109720.
- S. Liang and C. Wan, Biorefinery lignin to renewable chemicals via sequential fractionation and depolymerization, Waste Biomass Valorization, 2017, 8(2), 393–400, DOI:10.1007/s12649-016-9600-7.
- J. Rodríguez-Ramírez, L. L. Méndez-Lagunas, A. López-Ortiz, S. Muñiz-Becerá and K. Nair, Solar drying of strawberry using polycarbonate with UV protection and polyethylene covers: Influence on anthocyanin and total phenolic content, Sol. Energy, 2021, 221, 120–130, DOI:10.1016/j.solener.2021.04.025.
- M. Y. Kim, N. Yoon, Y. J. Lee, K. S. Woo, H. Y. Kim and J. Lee,
et al., Influence of thermal processing on free and bound forms of phenolics and antioxidant capacity of rice hull (Oryza sativa L.), Prev. Nutr. Food Sci., 2020, 25(3), 310–318, DOI:10.3746/pnf.2020.25.3.310.
- A. Snoussi, I. Essaidi, H. Ben Haj Koubaier, H. Zrelli, I. Alsafari and T. Živoslav,
et al., Drying methodology effect on the phenolic content, antioxidant activity of Myrtus communis L. leaves ethanol extracts and soybean oil oxidative stability, BMC Chem., 2021, 15(31), 1–11, DOI:10.1186/s13065-021-00753-2.
- Ó. Rodríguez, J. V. Santacatalina, S. Simal, J. V. Garcia-Perez, A. Femenia and C. Rosselló, Influence of power ultrasound application on drying kinetics of apple and its antioxidant and microstructural properties, J. Food Eng., 2014, 129, 21–29, DOI:10.1016/j.jfoodeng.2014.01.001.
- T. Ahad, A. Gull, J. Nissar, L. Masoodi and A. H. Rather, Effect of storage temperatures, packaging materials and storage periods on antioxidant activity and non-enzymatic browning of antioxidant treated walnut kernels, J. Food Sci. Technol., 2020, 57(10), 3556–3563, DOI:10.1007/s13197-020-04387-5.
- J.-Y. Lee, C.-J. Kim and B. Kunz, Identification of lactic acid bacteria isolated from kimchi and studies on their suitability for application as starter culture in the production of fermented sausages, Meat Sci., 2006, 72(3), 437–445, DOI:10.1016/j.meatsci.2005.08.013.
- F. K. Sayin and B. Alkan, The effect of pickling on total phenolic contents and antioxidant activity of 10 vegetables, J. Food Health Sci., 2015, 1(3), 135–141, DOI:10.3153/jfhs15013.
- M. G. Managa, Y. Sultanbawa and D. Sivakumar, Effects of Different Drying Methods on Untargeted Phenolic Metabolites, and Antioxidant Activity in Chinese Cabbage (Brassica rapa L. subsp. chinensis) and Nightshade (Solanum retroflexum Dun.), Molecules, 2020, 25(6), 1326, DOI:10.3390/molecules25061326.
- M. G. Managa, J. Shai, A. D. Thi Phan, Y. Sultanbawa and D. Sivakumar, Impact of household cooking techniques on African nightshade and Chinese cabbage on phenolic compounds, antinutrients, in vitro antioxidant, and β-glucosidase activity, Front. Nutr., 2020, 7, 1–13, DOI:10.3389/fnut.2020.580550.
- Y. Y. Lim and J. Murtijaya, Antioxidant properties of Phyllanthus amarus extracts as affected by different drying methods, LWT, 2007, 40(9), 1664–1669, DOI:10.1016/j.lwt.2006.12.013.
- S. Kamiloglu and E. Capanoglu, Polyphenol content in figs (ficus caricaL.): Effect of sun-drying, Int. J. Food Prop., 2015, 18(3), 521–535, DOI:10.1080/10942912.2013.833522.
- M. Lutz and J. Hernández Carolina, Phenolic content and antioxidant capacity in fresh and dry fruits and vegetables grown in Chile, CyTA, CyTA–J. Food, 2015, 13(4), 541–547, DOI:10.1080/19476337.2015.1012743.
- M. Nooshkam, M. Varidi and M. Bashash, The Maillard reaction products as food-born antioxidant and antibrowning agents in model and real food systems, Food Chem., 2019, 275, 644–660, DOI:10.1016/j.foodchem.2018.09.083.
- A. Piga, A. Del Caro and G. Corda, From plums to prunes: influence of drying parameters on polyphenols and antioxidant activity, J. Agric. Food Chem., 2003, 51(12), 3675–3681, DOI:10.1021/jf021207+.
- K. An, D. Zhao, Z. Wang, J. Wu, Y. Xu and G. Xiao, Comparison of different drying methods on Chinese ginger (Zingiber officinale
Roscoe): Changes in volatiles, chemical profile, antioxidant properties, and microstructure, Food Chem., 2016, 197, 1292–1300, DOI:10.1016/j.foodchem.2015.11.033.
- B. H. F. Saqueti, E. S. Alves, M. C. Castro, I. B. Ponhozi, J. M. Silva and J. V. Visentainer,
et al., Influence of drying and roasting on chemical composition, lipid profile and antioxidant activity of jurubeba (Solanum paniculatum L.), J. Food Meas. Char., 2022, 16(4), 2749–2759, DOI:10.1007/s11694-022-01370-w.
- O. Norkaew, P. Boontakham, K. Dumri, A. N. L. Noenplab, P. Sookwong and S. Mahatheeranont, Effect of post-harvest treatment on bioactive phytochemicals of Thai black rice, Food Chem., 2017, 217, 98–105, DOI:10.1016/j.foodchem.2016.08.084.
- R. Niazmand and S. Yeganehzad, Capability of oxygen-scavenger sachets and modified atmosphere packaging to extend fresh barberry shelf life, Chem. Biol. Technol. Agric., 2020, 7, 1–14, DOI:10.1186/s40538-020-00195-3.
- L.-T. Zhang, C.-Q. Chang, Y. Liu and Z.-M. Chen, Effect of chlorogenic acid on disordered glucose and lipid metabolism in db/db mice and its mechanism, Zhongguo Yixue Kexueyuan Xuebao, 2011, 33(3), 281–286, DOI:10.3881/j.issn.1000-503X.2011.03.015.
- G. Odongo, N. Schlotz, S. Baldermann, S. Neugart, S. Huyskens-Keil and B. Ngwene,
et al., African nightshade (Solanum scabrum Mill.): Impact of cultivation and plant processing on its health promoting potential as determined in a human liver cell model, Nutrients, 2018, 10(10), 1532, DOI:10.3390/nu10101532.
|
This journal is © The Royal Society of Chemistry 2024 |
Click here to see how this site uses Cookies. View our privacy policy here.