Effect of storage, temperature, and pH on the preservation of the oleuropein content of olive leaf extracts
Received
6th February 2024
, Accepted 18th March 2024
First published on 3rd April 2024
Abstract
Oleuropein, upon sucessful extraction from olive leaves, is treasured as being one of the major bioactive compounds with great potential for being incorporated into numerous food formulations that are processed under specific surrounding conditions. In this regard, for oleuropein to sustainably exert bio-functional effects, it needs to remain potent in an adequate amount in every step of potential valorizations. That being the case, this study examined the stability of olive leaf extracts obtained through ohmic heating (OH) compared to the conventional heating, (Conven) in terms of the total phenolic content (TPC), oleuropein (the key dependent variable), and hydroxytyrosol. The extracts were subjected to different surrounding conditions: (i) storage temperatures of 25 °C, 4 °C, and −20 °C over different time points for eight weeks; (ii) heating at 70 °C, 90 °C, and 110 °C over different time points (min), and (iii) pH ranging from 3 to 9. The results from storage stability tests revealed that a storage temperature of −20 °C was the optimal condition for the stability of the extracts, while storage at 25 °C was the least desirable condition, particularly for oleuropein and TPC. The thermal study showed that exceeding a high temperature (particularly 110 °C) was detrimental to the oleuropein content. Also, the optimum pH value (pH 5) determined in this study narrowly resembled the pH values observed at the initial point of the extracts (4.8–5.2). Importantly, the data support the preference of ohmic heating over a conventional heating approach because substantial quantities of polyphenols remained in the OH extracts over the course of storage/heating. For oleuropein, ohmic heating at 75 °C was the most preferred approach, whereby the values continued to stay at the highest levels in the varying surrounding conditions/over different time points.
Sustainability spotlight
The concentration of polyphenols, particularly oleuropein, in olive leaf extracts, needs to remain sufficiently high to exert sustainably bio-functional effects for various high-value applications. Dealing with the stability assessments in the current study, the obtained results give grounds for the significant role of extraction technique as the leaves extracted through ohmic heating, compared to conventional heating, still contained large amounts of oleuropein and hydroxytyrosol over the course of storage and thermal trials. With respect to the United Nations Sustainable Development Goals, the data from the present study are of relevance to the following goals: Goal 2—“end hunger, achieve food security and improved nutrition and promote sustainable agriculture” and Goal 12—“ensure sustainable consumption and production patterns”.
|
Introduction
Polyphenols when extracted from olive leaves have great potential for incorporation into food and nutraceuticals as well as for medicinal applications. However, before such applications can be approached, it is essential to evaluate the stabilities of the extracts as a means to ascertain the threshold of their resistance to different surrounding environments over the course of time. This is mainly because, depending on actual conditions, the leaf extracts may be treated at high temperatures, exposed to high/low pH, or stored at different temperatures for a period of time. Therefore the extracts need to remain stable with minimum compositional changes, otherwise their value-added potential may lose its relevance. This is particularly important in view of the content of principal/desired polyphenols, including oleuropein, that distinctively contribute to the bio-functional properties of olive leaves.1–4 For oleuropein to sustainably exhibit bioactivity, it needs to be present in a considerable amount in the extract throughout its processing period. Previous research studies using different operating conditions found that the stability of oleuropein in olive leaf extracts is affected by pH conditions,5,6 storage temperature/duration,7–9 and heating temperature.10
Given the importance of the factor of preservation, the present study extended the previous research to assess the stability of olive leaf extracts obtained by ohmic heating (that showed significant effectiveness in the extraction of polyphenols)11,12 under the following environmental conditions: (i) varying storage temperatures over different time points, (ii) thermal conditions over different heating time points, and (iii) differing pH solutions. Beyond assessing the changes in the total phenolic content (TPC), special attention was placed on the variations of oleuropein under the selected conditions. The content of hydroxytyrosol was also studied throughout the stability experiments to partly analyze the assumption that the chemical depletion of oleuropein may occur concurrently with the increment in hydroxytyrosol under the same executed conditions. The leaf extracts (from ohmic heating) were compared to those processed with conventional heating (Conven) and solvent/non-heating extraction (Control) methods.
Materials and methods
Plant materials and chemicals
Olive mill leaves from the same cultivar (Picual) and growing area as those applied in our earlier experiment11 were kindly supplied by “Center for Advanced Studies in Energy and Environment”, University of Jaén, Campus of Las Lagunillas, Jaén, Spain. Upon arrival, they were cleaned, dried, and ground using the same methods as practiced previously.
All the chemicals and standard reagents (analytical and HPLC grade) were purchased from Sigma-Aldrich (Saint Louis, MO, USA).
Sample preparation
Extraction system.
The extraction processing design in this study was identical to that practiced in our previous study.11 Briefly, olive leaves were initially cleaned, washed, and dried at 37 °C for 48 h. The dried leaves were then ground and size reduced to 0.3 mm. The dry ground olive leaves (solid
:
solvent ratio of 1
:
10) were then subjected separately to each selected extraction protocol (Table 1) using the following techniques: (i) ohmic heating (OH), (ii) conventional heating (Conven), and (iii) Control (solvent agitation without heating, 25 ± 5 °C). All the extraction techniques used a magnetic stirrer, placed in the food chamber (100 mL capacity), to ensure homogeneity of the sample solution and an even distribution of the heat flow. (Stir speed: 150 rpm). Processing time (15 min) remained the same for all extraction methods.
Table 1 Summary of the mechanical processing design used for the extraction from olive leaves
Extraction system |
Specification(s) |
Ohmic heating (OH) |
Extraction solvent: aqueous ethanol (80%); temperature: 45 °C, 55 °C, 75 °C; solid : solvent ratio: 1 : 10 w/v; duration: 15 min |
Conductivity was customized (3.1–4.2 mS cm−1) using sodium chloride in advance of the extraction process; frequency (25 kHz) and electrical voltage (1–10 V cm−1) remained constant; used a K-type thermocouple (in the center of food chamber) attached to a data logger connected to software computer system |
Conventional heating (Conven) |
Extraction solvent: aqueous ethanol ( 80%); temperature: 45 °C, 55 °C, 75 °C; solid : solvent ratio: 1 : 10 w/v; duration: 15 min |
Followed the same process design as in ohmic heating with the exception of using electrodes in the chamber (i.e., electric field was not applied); used a K-type thermocouple (in the center of the food chamber) attached to a data logger connected to a software computer system |
Solvent extraction (Control) |
Extraction solvent: aqueous ethanol (80%); temperature: room temperature (20 ± 5 °C); solid : solvent ratio: 1 : 10 w/v; duration: 15 min |
Constant agitation at room temperature (without heating) using the same solvent ratio and duration as used for OH & Conven |
After the extraction process was complete, the leaf extract (hydroethanolic solution) was centrifuged at 5000 rpm for 15 min. The supernatant was then filtered through a Whatman filter paper. The filtrate was rotary evaporated (40 °C, 1 h). The obtained extract, bottled and nitrogen flushed, was then subjected to stability assessment as described below.
Stability study of the olive leaf extracts.
The stability of the polyphenols (total and target compounds) was assessed under the selected surrounding environments, as shown in Table 2.
Table 2 Surrounding conditions selected for this study to determine the stability of polar phenols of olive leaf extracts
Surrounding environment |
Description |
Storage temperature (°C) |
4 |
The leaf extracts were stored at each defined temperature over an 8 week duration. The analytical assessments were performed at weekly intervals from week 0 to the end of week 8 |
25 |
−20 |
Heating temperature (°C) |
70 |
The leaf extracts were heated at each defined temperature over a total of 60 min. The assessments were performed after 0, 20, 40, and 60 min |
90 |
110 |
pH |
3 |
The leaf extracts were adjusted to the given pH values with the prepared buffer solutions and incubated for one week prior to analysis |
5 |
7 |
9 |
Determination of the total phenolic content
The total phenolic content (TPC) was determined using the method of Singleton et al.13 with some modifications as previously noted.11 An aliquot of 100 μL extract was first diluted with 6 mL distilled water. The solution was then reacted with 500 μL of Folin–Ciocalteau reagent and vigorously mixed. After 8 min incubation at room temperature, 1.5 mL of sodium carbonate solution (20%) was added, and then diluted with distilled water to give a final volume of 10 mL solution. The mixture was incubated at room temperature in the dark for 60 min. The absorbance readings were recorded at 760 nm using a UV-vis spectrophotometer. The standard calibration curve (0.05–1.0 mg mL−1) was prepared from gallic acid stock solution.
For the calculations, a standard graph was first drawn by plotting the absorbance vs. known concentration. The concentration of total phenols (unknown concentration) was then calculated from the least-squares regression line (regression coefficient of r2 ≥ 0.99), according to eqn (1). The results were corrected for dilution and reported in mg gallic acid equivalents per gram of extract (mg GAE per g).
where,
y = absorbance,
m = slope (gradient),
x = unknown concentration,
b = intercept.
HPLC analysis of the target polyphenols
The concentrations of the oleuropein and hydroxytyrosol of the extracts were assessed in terms of their stability toward the given conditions/over different time points. These were measured through an ultrahigh-performance liquid chromatography (UHPLC) (Shimadzu Nexera X2 UHPLC) system connected to a diode array detector (Shimadzu SPD-M20A), and an integration system (Shimadzu LabSolutions software, Kyoto, Japan). The separations of the target polyphenols were performed using a reverse-phase Aquity UPLC BEH C18 column (100 mm × 2.1 mm i.d., 1.7 μm particle size, Waters Corporation) based on the gradient elution method described previously14 with slight modifications.15 The flow rate was 0.3 mL min−1. The injection volume was 5 μL. The chromatograms were recorded at 280 nm. Oleuropein and hydroxytyrosol were first identified by the retention times of the commercial standards with the UV spectra detector, and then quantified against the linear standard calibration curve. The results were reported as mg abovementioned phenol per g extract.
Statistical analysis
Significant differences (p < 0.05) between the mean values (±SD) of all the determinations were statistically assessed using SPSS software, version 27.0. Factorial repeated measures ANOVA was used to examine the magnitude of changes of each dependent variable through examining: (i) the same extraction group across the different storage times/heating times/pH values, and (ii) different extraction groups within the same storage time/heating time/pH value. Multiple comparisons means between extraction groups were assessed by running the Bonferroni post hoc test. The equality of variance was checked through using the Levene's test (when the p-value was greater than 0.05, the homogeneity of equal variance was assumed to be not violated). All the experiments were performed in triplicate and the results were presented as the mean ± standard deviation.
Effect of the storage conditions on the stability of the polyphenols of the olive leaf extracts
Storage stability of the total polyphenols.
As shown in Fig. 1, across all the extraction groups, the content of total polyphenols at 25 °C remained relatively unchanged over week 1, then significantly decreased over week 2, remained stable between week 2 and week 3, and thereafter sharply decreased over time points by the end of storage period (up to 27%). The storage at 4 °C showed significant depletions after week 1 then remained rather stable for three weeks, thereafter (after week 4) the numbers gradually dropped by the end the storage (up to 19%) . The storage at −20 °C represented the most suitable condition for TPC preservation that showed minimum depletions, in each extraction group, over time points, (up to 11% final loss), and overall the values remained more stable at this temperature compared to the other executed temperatures.
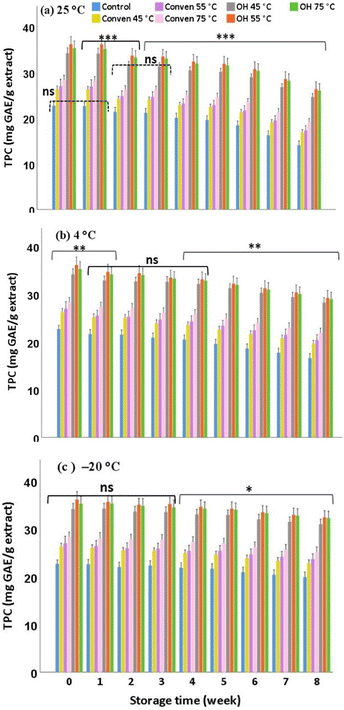 |
| Fig. 1 Changes in the total phenolic content (TPC) of olive leaf extracts (mg GAE per g extract) stored at different temperatures: [(a) 25 °C, (b) 4 °C, (c) −20 °C] over different time points (week). Extraction methods: ohmic heating (OH), conventional heating (Conven), and solvent/non-heating extraction (Control). Results are presented as mean values with standard deviation error bars. The data were analyzed by factorial repeated measures ANOVA followed by Bonferroni post hoc test. [“ns”: not significant; (*): significant differences (p < 0.05); (**) significant differences (p < 0.01); (***) significant differences (p < 0.001) between storage times for the same extraction group. | |
Comparing the extraction methods for the same storage temperature, it was found that the trends of the decrease in TPC over the different time points followed the same pattern in all groups. However, quantitatively, the OH groups contained significantly greater amounts after an eight-week storage period; among which, the OH 55 °C stored at −20 °C demonstrated the highest levels (particularly upon the storage at −20 °C, representing the final value of 32.42 ± 4.28 mg GAE per g extract). This can be explained by reason of their richness in TPC at the initial point of the extraction (week 0), in which, despite being affected proportionally throughout the storage, the numbers remained considerably high over time. Therefore, compared to the Conven and Control groups, the extraction by ohmic heating (particularly at 55 °C) conferred benefits for the conservation of the total polyphenols assessed in this study, with the freezing point (−20 °C) being the temperature of preference.
Storage stability of oleuropein.
Examining the changes in oleuropein content (Fig. 2), the trends of declines over the different time points were found to be relatively similar in all extraction groups for the same storage temperature. At 25 °C, the concentrations of oleuropein in all extracts started to decrease sharply from the first week of storage and the numbers continued to drop significantly over the course of eight weeks (for around a 45% loss). On the other hand, the storage at −20 °C followed by 4 °C caused less detrimental effects on the oleuropein content; although the numbers did decline (after week 2 at −20 °C, and after week 2 at 4 °C), the magnitude of losses (15–17%) was significantly lower than those stored at 25 °C.
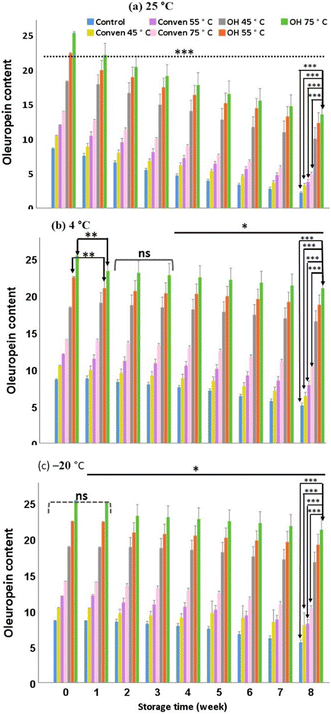 |
| Fig. 2 Changes in the oleuropein content of olive leaf extracts (mg g−1) stored at different temperatures: [(a) 25 °C, (b) 4 °C, (c) −20 °C] over different time points (week). Extraction methods: ohmic heating (OH), conventional heating (Conven), and solvent/non-heating extraction (Control). Results are presented as mean values with standard deviation error bars. The data were analyzed by factorial repeated measures ANOVA followed by Bonferroni post hoc test. [“ns”: not significant; (*): significant differences (p < 0.05); (**) significant differences (p < 0.01) between storage times for the same extraction method]; [(***) significant differences (p < 0.001) between storage times for the same extraction method, and between extraction methods within the same storage time]. | |
As mentioned earlier, the ability of polyphenols to exert optimal biological effects depends highly on their concentrations. This is particularly the case for the major active compounds, such as oleuropein, which is regarded as a leading antioxidant phenol in olive leaves.
In general, during the storage, the magnitude of falls in oleuropein is partly associated with the storage temperature and/or duration. The undesirable depletion of oleuropein may arise from oxidation induction caused by catalytic enzymes, such as polyphenol-oxidase, through their exertions to decompose oleuropein to the secondary oxidized products.16 In this regard, the efficiency of the extraction method becomes an important factor as it potentially enables high extraction yield of biophenols at the initial point (before the extracts are stored), and thus the phenolic-rich extracts, despite suffering from severe storage conditions, would be more likely to contain large numbers of phenols over the course of the storage time. This was the case here (Fig. 2), as the extracts from ohmic heating demonstrated substantial quantities of oleuropein compared to the other two extraction methods under the same storage condition. The suitability of ohmic temperature for oleuropein concentrations under storage/over time followed the order: OH 75 °C > OH 55 °C > OH 45 °C. Therefore, the extraction by ohmic heating at 75 °C is preferred particularly at freezing point (−20 °C) which enabled the highest content of oleuropein, around 25.28 mg g−1 (initial content) and 21.24 mg g−1 extract (after an eight-week storage). As a side note, in our previous study, this particular extraction method (ohmic at 75 °C) comparably favored a higher antiradical capacity of the extracts.11 This in part could be attributed to the presence and bioactivity of oleuropein, which probably acted as a pronounced radical scavenger proportion-dependently.
Storage stability of hydroxytyrosol.
As shown in Fig. 3, the content of hydroxytyrosol in all extracts increased sharply (by around 18%) at the early stage of the storage. It rose rather considerably after the first week when stored at 25 °C; after the second week when stored at 4 °C and −20 °C. The initial increments in hydroxytyrosol could be due to the concurrent reduction of oleuropein, assuming that oleuropein was not degraded via oxidation and/or de-glycosylation but rather degraded through hydrolysis from which derivatives such as hydroxytyrosol can be generated. In this case, the biosynthesis of hydroxytyrosol stems from the catalyzing actions of hydrolase enzymes such as esterase, that is reportedly responsible for the breakage of ester bonds and the subsequent formation of phenolic alcohols, including hydroxytyrosol.16–19 It is also noteworthy that, regardless of the diverse experimental methods, numerous studies suggested that the occurance of the simultaneous rise in hydroxytyrosol content anddrop in oleuropein content could be attributed to the hydrolysis reactions of oleuropein.7,16,20
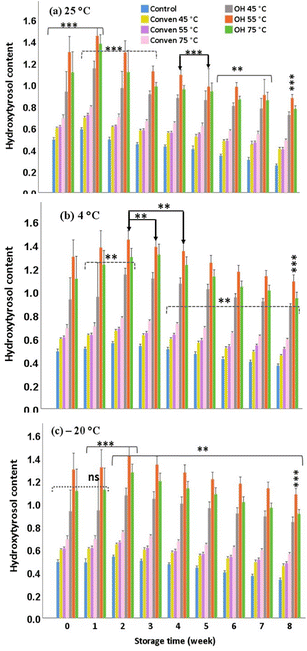 |
| Fig. 3 Changes in the hydroxytyrosol content of olive leaf extracts (mg g−1) stored at different temperatures: [(a) 25 °C, (b) 4 °C, (c) −20 °C] over different time points (week). Extraction methods: ohmic heating (OH), conventional heating (Conven), and solvent/non-heating extraction (Control). Results are presented as mean values with standard deviation error bars. All data sets were analyzed by factorial repeated measures ANOVA followed by Bonferroni post hoc test. [“ns”: not significant;(*): significant differences (p < 0.05); (**) significant differences (p < 0.01) between storage times for the same extraction group]; [(***) significant differences (p < 0.001) between storage times for the same extraction method, and between extraction groups within the same storage time]. | |
However, although the numbers increased within a short-term storage, hydroxytyrosol declined rather sharply after initial rises over time points, Indeed, compared to that oberved for oleuropein content under the same storage time points, the adverse impact of the extended periods at 4 °C and −20 °C was more significant on the depletion of hydroxytyrosol content. This may be explained by the effect of other causative factors behinde the decrease in hydroxytyrosol during the storage period, among which, it could be attributed to the adverse effects of certain enzymes such as polyphenoloxidase.16
Overall the data demonstrate that the extraction by ohmic heating is preferable over conventional heating, not only in terms of the initial recovery but also for alleviating the severity of storage by maintaining substantial levels of hydroxytyrosol over the storage period (8 weeks) when stored at 25 °C, 4 °C and −20 °C. The suitability of the temperature of ohmic heating for the extraction and preservation of hydroxytyrosol followed the order: 55 °C > 75 °C > 45 °C.
Effect of heating on the stability of the polyphenols of olive leaf extracts
Thermal stability of the total polyphenols.
The thermal stability of leaf extracts was studied at different heating temperatures (70 °C, 90 °C, and 110 °C) over different time points (20, 40, and 60 min). The obtained results show that over-exposure to high temperature may compromise the stability of the TPC in all extraction groups (Fig. 4). The values of TPC for the same extraction group remained stable at 70 °C and to a lesser extent at 90 °C.
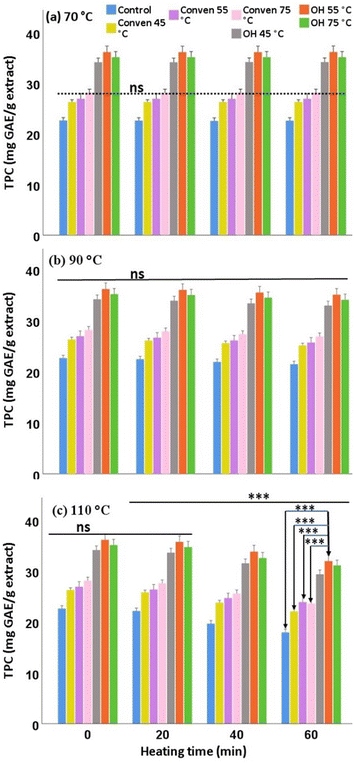 |
| Fig. 4 Changes in the total phenolic content (TPC) of olive leaf extracts (mg GAE per g) as a function of heating [(a) 70 °C, (b) 90 °C, and (c) 110 °C] over different time points (min). Extraction methods: ohmic heating (OH), conventional heating (Conven), and solvent/non-heating extraction (Control). Results are presented as mean values with standard deviation error bars. The data were analyzed by factorial repeated measures ANOVA followed by Bonferroni post hoc test. “ns”: not significant differences between heating times for the same extraction group]; [(***) significant differences (p < 0.001) between heating times for the same extraction group and between extraction groups within the same heating time]. | |
In general, the rate of falls in the polyphenols is often accelerated by the increase in temperature, as they are rather sensitive to heat and liable to be decomposed through the disruption of their covalent bonds and the formation of other secondary products, or degraded through the oxidation reactions. As seen in Fig. 4, TPC slightly dropped, in all extraction groups, when the heating at 90 °C was prolonged for 60 min (less than 5% loss). More importantly, the heating at 110 °C for 40 min and beyond caused significant drops in the total phenolic content in all extracts, with relatively sharp declines over 60 min (around15% final loss).
It should be pointed out that the magnitude of the differences between the ohmic groups and Conven/Control groups remained significantly large over time points for the same thermal trial, p < 0.001. Indeed, the ohmic heating represented a method of choice for the leaf extraction due to its role in maximizing the recovery of total phenols at the initial point (36.13 ± 4.99 mg GAE/g in OH 55 °C) which, despite being affected by the severe thermal conditions, substantial levels remained in the OH extracts upon the 60-min heating (at 110 °C the OH-55 °C yielded 31.96 ± 3.57 mg GAE per g in the end). Overall, the suitability of the extraction temperature of the ohmic technique for the thermal stability of the total phenols was determined as follows: OH 55 °C > OH 75 °C > OH 45 °C.
Thermal stability of oleuropein.
Primarily, the extracts should not be exposed to excessively high temperature for a prolonged time. This is particularly important in terms of the maintainability of certain bio-phenols, such as oleuropein, whose biological function is apparently dictated by its quantities within the extract. As seen in Fig. 5, oleuropein in all extraction groups remained relatively stable at 70 °C (for 60 min) and 90 °C (for 20 min), but showed little stability at higher temperature (110 °C) as the concentration started to decline upon 20 min and thereafter sharply dropped (up to around 22% loss) over the period of 60 min heating.
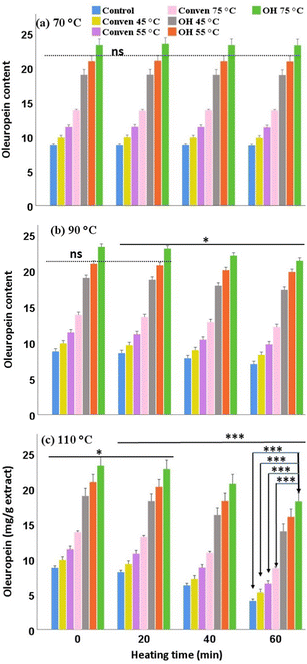 |
| Fig. 5 Changes in the oleuropein content of olive leaf extracts (mg g−1) as a function of heating [(a) 70 °C, (b) 90 °C, and (c) 110 °C] over time points (min). Extraction methods: ohmic heating (OH), conventional heating (Conven), and solvent/non-heating extraction (Control). Results are presented as mean values with standard deviation error bars. thedata were analyzed by factorial repeated measures ANOVA followed by Bonferroni post hoc test. “ns”: not significant; (*): significant differences (p < 0.05) between heating times for the same extraction group]; [(***) significant differences (p < 0.001) between heating times for the same extraction group, and between extraction groups within the same heating time]. | |
The biochemical degradation of oleuropein is typically associated with the exertion of the following catalyzing enzymes: (i) polyphenol-oxidase through an oxidation reaction, giving rise to the formation of secondary products; (ii) esterase through a hydrolytic reaction (as indicated earlier), giving rise to the formation of simple phenols, such as hydroxytyrosol; and (iii) β-glucosidase through de-glycosylation, giving rise to the formation of oleuropein aglycone and glucose.21,22 In this regard, heat is among the primary factors affecting oleuropein bioconversion which can be desirable or undesirable. Given this, the rate of temperature/duration is of importance, as seen in this study where overheating resulted in substantial drops in oleuropein content. However, the actual enzymatic mechanism (mentioned above) that could be involved in oleuropein degradation is yet to be substantiated.
Overall, regardless of the adverse effects of the heating, the results from each thermal experiment revealed that the extracts from ohmic heating contained the highest levels of oleuropein from the initial point (0 min) until the end of 60 min heating; in particular the ohmic extract at 75 °C, for which 18.2 mg g−1 remained upon extreme high temperature (110 °C) heating for 60 min. Thus, making it a preferred method of choice in regards to the initial extraction of oleuropein, as well as its stability when the leaf extracts are exposed to excessive thermal conditions over the various time points.
Thermal stability of hydroxytyrosol.
As shown in Fig. 6, in all extraction groups the hydroxytyrosol content remained stable at 70 °C (over the heating time points), and at the early stage of 90 °C. Heating at 90 °C for 40 min showed a slight increase (2–3%) in content, and this remained relatively unchanged when the heating time was extended further (60 min). At 110 °C, the concentration slightly decreased over 40 min, and then marginally increased upon 60 min heating.
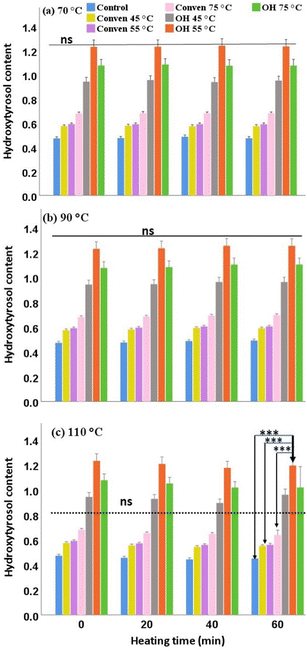 |
| Fig. 6 Changes in the hydroxytyrosol content of olive leaf extracts (mg g−1) as a function of heating [(a) 70 °C, (b) 90 °C, and (c) 110 °C] over time points (min). Extraction methods: ohmic heating (OH), conventional heating (Conven), and solvent/non-heating extraction (Control). Results are presented as mean values with standard deviation error bars. The data were analyzed by factorial repeated measures ANOVA followed by Bonferroni post hoc test. “ns”: not significant differences between heating times for the same extraction group; (***): significant differences (p < 0.001) between extraction groups within the same heating time. | |
Hydroxytyrosol, compared to oleuropein, showed more stable fluctuations during the heating trials. The rise in hydroxytyrosol content at 90 °C could be related to the concurrent degradation of oleuropein. Quantitatively, the ohmic extracts, compared to Conven and Control groups, showed significantly higher contents of hydroxytyrosol over the various heating times (1.26 ± 0.24 and 1.18 ± 0.21 remained in OH 55 °C upon heating at 90 °C and 110 °C for 60 min). Similar to that observed for storage stability of hydroxytyrosol, the suitability of this method (ohmic heating) for thermal stability of this compound follow the same order: OH 55 °C > OH 75 °C > OH 45 °C.
Effect of pH on the stability of the polyphenols of olive leaf extracts
pH stability of the total polyphenols.
As emphasized in previous reports, the pH environment of the extracts is among the major factors responsible for the fate of endogenous polyphenols.23–30
The changes in total phenols of the extracts at differing pH values are shown in Fig. 7. As can be seen, total phenolic content in each extraction group remained rather stable across pH variations as the mean differences, for the same extraction group, were not statistically significant p > 0.05. Among which, the pH of 5 can be an ideal condition for the preservation of total phenols of all extracts examined in this study.
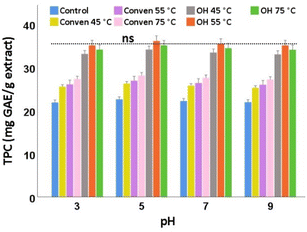 |
| Fig. 7 Changes in the total phenolic content (TPC) of olive leaf extracts (mg GAE per g) as a function of pH. Extraction methods: ohmic heating (OH), conventional heating (Conven), and solvent/non-heating extraction (Control). Results are presented as mean values with standard deviation error bars. The data were analyzed by factorial repeated measures ANOVA followed by Bonferroni post hoc test. “ns”: not significant differences between pH values for the same extraction group. | |
Indeed, the optimum pH condition evaluated here (pH 5) is within the pH range measured originally prior to the pH modifications (4.8–5.2). This partly explains that the pH of the media of the olive leaf extracts can be suitable for the protection of polyphenols, which eliminates the necessity for pH adjustment as far as the conservation of the total phenols of the extract is of concern. Moreover, similar to those observed in storage and thermal experiments, the extracts obtained by the ohmic heater (particularly at 55 °C) represented the maximum TPC values for the same pH conditon.
pH stability of oleuropein.
The stability of antioxidant polyphenols including oleuropein, is partly dictated by the extent of acidity or basicity of the extract solution.31 This may come with a challenge because the degree of susceptibility to pH is influenced differently by the molecular structure of each phenolic class within the cell; particularly, the position and numbers of their hydroxyl groups. Therefore, the pH can provide a favorable or unfavorable environment for the molecules of interest.
In the case of the extraction methods, the extracts from ohmic heating at 75 °C were evidently most desirable for the stability of oleuropein under the pH conditions examined here. The highest values belonged to the samples with pH 5 for all the extraction groups (Fig. 8). The numbers of oleuropein also responded rather favorably in an acidic medium (pH 3), which could potentially be attributed to the phenomenon of protonation of the phenol hydroxyl groups.32,33
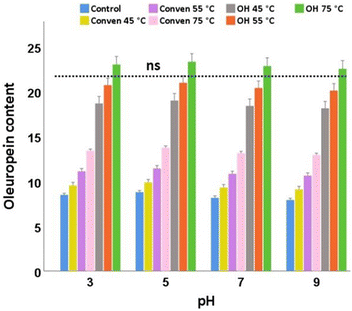 |
| Fig. 8 Changes in the oleuropein content of olive leaf extracts (mg g−1) as a function of pH. Extraction methods: ohmic heating (OH), conventional heating (Conven), and solvent/non-heating extraction (Control). Results are presented as mean values with standard deviation error bars. The data were analyzed by factorial repeated measures ANOVA followed by Bonferroni post hoc test. “ns”: not significant differences between pH values for the same extraction group. | |
Overall, there were no significant differences in oleuropein content of the same leaf extract across the differing pH conditions. Among which, the alkaline medium (pH 9) showed the least favorable pH condition as marginally the lowest content of oleuropein was found in each corresponding extraction group. This is potentially attributable to the de-protonation of the hydroxyl groups of phenols through breaking their ester bonds. Taking a cue from this, oleuropein should not be exposed to high pH solutions during various applications/functionalization.
pH stability of hydroxytyrosol.
As seen in Fig. 9, the response of each extract to pH 5 for hydroxytyrosol content rather resembled to those detected for oleuropein content. In other words, both hydroxytyrosol and oleuropein remained at maximum levels at the same pH condition. That being the case, it was likely that oleuropein (in glycosidic or aglycone form) was partially/negligibly the precursor for the liberated hydroxytyrosol under the same pH condition; with the possibility of the decomposition of other hydroxytyrosol-conjugated secoiridoids, including oleuropein derivatives, such as de-carboxymethyl oleuropein aglycone, which potentially served as one of the major substrates for the generation of hydroxytyrosol under such a condition (pH 5). With marginal differences, the acidic medium (pH 3) provided the least favorable environment for hydroxytyrosol content of each leaf extract. Overall, the suitability of pH condition for hydroxytyrosol content of each leaf extract was determined to be in the following descending order: pH 5 > pH 7 > pH 9 > pH 3.
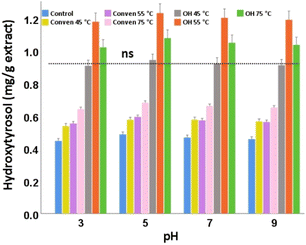 |
| Fig. 9 Changes in the hydroxytyrosol content of olive leaf extracts (mg g−1) as a function of pH. Extraction methods: ohmic heating (OH), conventional heating (Conven), and solvent/non-heating extraction (Control). Results are presented as mean values with standard deviation error bars. The data were analyzed by factorial repeated measures ANOVA followed by Bonferroni post hoc test. “ns”: not significant differences between pH values for the same extraction group. | |
Taken as a whole, for the content of total and individual polyphenols examined in this study, the pH of 5 seemed to be an ideal condition, particularly for the ohmic heating that significantly offered advantage of intensified yields of the desired bio-phenols.
Conclusions
The storage of each leaf extract at 25 °C, over time points, was found to be the least desirable keeping condition for polyphenolic content of the extract while the storage at −20 °C was evidently the most favorable condition that enabled significantly better conservation of the total and target polyphenols over the course of eight-week period.
Heating at exceeding high temperature (110 °C) for an extended time (60 min) was detrimental to the content of total phenols and oleuropein. The results confirmed that the initial pH conditions of the extracts were narrowly close to the optimum value (pH 5) determined for the protection of polyphenols, especially oleuropein.
Comparing the extraction methods, the data justify the significance of ohmic heating as a preferred mechanical extraction technique, over conventional heating, for olive leaf extraction because the ohmic extracts, after being subjected to extreme storage/thermal conditions over the extended periods, still possessed significant levels of oleuropein, total polyphenols, and hydroxytyrosol. Further research is needed to ascertain the stability of total/target polyphenols of the leaf extracts through the addition of the extracts to various food forumlations. Such investigations could provide further insights into the applicability of the extracts for potential bio-functional applications.
Author contributions
Fereshteh Safarzadeh Markhali: investigation; methodology; writing manuscript; conceptualization; data analysis; resources. José A. Teixeira: conceptualization; supervision; methodology; review and editing; resources.
Conflicts of interest
There are no conflicts to declare.
Acknowledgements
The authors wish to thank Eulogio Castro, Center for Advanced Studies in Energy and Environment, University of Jaén, Campus of Las Lagunillas, Jaén, Spain, for providing the olive leaves. This study was supported by the Portuguese Foundation for Science and Technology (FCT), under the scope of the strategic funding of UID/BIO/04469/2020 unit.
References
- G. M. Delgado-Pertíñez, A. Chesson, G. J. Provan, A. Garrido and A. Gómez-Cabrera, Ann. Zootech., 1998, 47, 141–150 CrossRef.
- N. Acar-Tek and D. Ağagündüz, Ann. Nutr. Metab., 2020, 76, 10–15 CrossRef CAS PubMed.
- S. H. Omar, Sci. Pharm., 2010, 78, 133–154 CrossRef CAS PubMed.
- F. S. Markhali, J. A. Teixeira and C. M. Rocha, Processes, 2020, 8(9), 1177 CrossRef CAS.
- K. Stamatopoulos, E. Katsoyannos and A. Chatzilazarou, Antioxidants, 2014, 3, 229–244 CrossRef PubMed.
- R. Gonzalez-Ortega, C. D. Di Mattia, P. Pittia and P. U. Natasa, J. Sci. Food Agric., 2023, 103, 2047–2056 CrossRef CAS PubMed.
- M. E. Martínez-Navarro, C. Cebrián-Tarancón, J. Oliva, M. R. Salinas and G. L. Alonso, Antioxidants, 2021, 10, 1963 CrossRef PubMed.
- M. H. Ahmad-Qasem, B. H. Ahmad-Qasem, E. Barrajon-Catalan, V. Micol, J. A. Carcel and J. V. Garcia-Perez, Ind. Crops Prod., 2016, 79, 232–239 CrossRef CAS.
- N. S. Malik, J. M. Bradford and J. Food, Agric. Environ., 2008, 6, 8–13 CAS.
- A. Mohammadi, S. M. Jafari, A. F. Esfanjani and S. Akhavan, Food Chem., 2016, 190, 513–519 CrossRef CAS PubMed.
- F. S. Markhali and J. A. Teixeira, Sustainable Food Technol., 2024, 2, 461–469 RSC.
- F. Safarzadeh Markhali, J. A. Teixeira and C. M. Rocha, Clean Technol., 2022, 4(2), 512–528 CrossRef.
- V. L. Singleton, R. Orthofer and R. M. Lamuela-Raventós, Methods Enzymol., 1999, 299, 152–178 CAS.
- J. Quero, L. F. Ballesteros, P. Ferreira-Santos, G. R. Velderrain-Rodriguez, C. M. Rocha, R. N. Pereira, J. A. Teixeira, O. Martin-Belloso, J. Osada and M. J. Rodríguez-Yoldi, Antioxidants, 2022, 11(5), 828 CrossRef CAS PubMed.
- F. S. Markhali and J. A. Teixeira, Sustainable Food Technol., 2023, 1(6), 896–905 RSC.
- A. De Leonardis, V. Macciola, F. Cuomo and F. Lopez, Food Chem., 2015, 175, 568–574 CrossRef CAS PubMed.
- E. Ramírez, M. Brenes, P. García, E. Medina and C. Romero, Food Chem., 2016, 206, 204–209 CrossRef PubMed.
- J. J. Yuan, C. Z. Wang, J. Z. Ye, R. Tao and Y. S. Zhang, Molecules, 2015, 20, 2903–2921 CrossRef PubMed.
- H. Jemai, M. Bouaziz, I. Fki, A. El Feki and S. Sayadi, Chem.-Biol. Interact., 2008, 176, 88–98 CrossRef CAS PubMed.
- S. Feng, C. Zhang, L. Liu, Z. Xu, T. Chen, L. Zhou, M. Yuan, T. Li and C. Ding, Separations, 2021, 8, 156 CrossRef CAS.
- A. V. Bounegru and C. Apetrei, Int. J. Mol. Sci., 2022, 23, 12569 CrossRef CAS PubMed.
-
L. A. Cavaca, I. M. López-Coca, G. Silvero and C. A. M. Afonson, in Studies in Natural Products Chemistry, ed. A. U. Rahman, Elsevier, 2020, vol. 64, pp. 131–180 Search PubMed.
- L. G. Roy and A. Urooj, J. Food Biochem., 2013, 37, 336–342 CrossRef CAS.
- V. R. Palvai, S. Mahalingu and A. Urooj, Int. J. Med. Chem., 2014, 2014, 1–7 Search PubMed.
-
W. Krungkri and V. Areekul, Proceedings of the 16th ASEAN Food Conference, 2019, pp. 126–l32 Search PubMed.
- Y. T. Chen, W. T. Kao and K. W. Lin, Food Chem., 2008, 107(1), 250–257 CrossRef CAS.
- M. Friedman and H. S. Jürgens, J. Agric. Food Chem., 2000, 48, 2101–2110 CrossRef CAS PubMed.
- S. Arabshahi-D, D. V. Devi and A. Urooj, Food Chem., 2007, 100, 1100–1105 CrossRef CAS.
- D. Amendola, D. M. De Faveri and G. Spigno, J. Food Eng., 2010, 97, 384–392 CrossRef CAS.
- J. Katanić, T. Boroja, N. Stanković, V. Mihailović, M. Mladenović, S. Kreft and M. M. Vrvić, Food Funct., 2015, 6, 1164–1175 RSC.
- R. Tsao, Nutrients, 2010, 2, 1231–1246 CrossRef CAS PubMed.
- M. Tagrida and S. Benjakul, J. Food Biochem., 2020, 44, e13508 CrossRef CAS PubMed.
- S. Ghosh, R. Chakraborty and U. Raychaudhuri, 3 Biotech, 2015, 5, 633–640 CrossRef PubMed.
|
This journal is © The Royal Society of Chemistry 2024 |
Click here to see how this site uses Cookies. View our privacy policy here.