Bovine colostrum as a promising nutraceutical: a systematic review
Received
31st December 2023
, Accepted 27th February 2024
First published on 14th March 2024
Abstract
Nowadays, it is essential to identify the benefits of sustainable living with nutritional food for the world's expanding population. This systematic review explores the unique composition of bovine colostrum, the initial milk produced by mammals post-parturition, compared to mature milk. We delve into extensive studies to examine its potential applications in human nutrition and health. Meticulous characterisation of its constituent fractions reveals a diverse array of potentially bioactive molecules, demonstrating their influence on neonatal development and their relevance in this era. Bovine colostrum can be a suitable alternative to mature milk as it is a rich source of numerous components such as bioactive proteins, fats, oligosaccharides, minerals and vitamins. Advance technologies were also used to preserve and commercialise it. So, a comprehensive discussion of its potential benefits for human health and exploring various preservation techniques for bovine colostrum presents a thorough overview of its multifaceted applications in promoting health and well-being.
Sustainability spotlight
Bovine colostrum is the first milk that a bovine produces after giving birth and it contains numerous natural micro- and macro-nutrients, immunoglobulin, peptides, growth factors, etc. This systematic review clearly addresses every possible aspect of bovine colostrum. This systematic review included the chemical composition, technological advancements for preservation, and potential health advantages of bovine colostrum. Lastly, the environmental impact of bovine colostrum compared to traditional mature milk is presented in this research. Future scientists, food technologists, dairy technologists, and industrialists will find this review useful in their understanding of bovine colostrum and in their investigation of its uses and limitations. This form of milk offers several components which have shown numerous health benefits. So it may be used to formulate milk and milk products. The UN's sustainable development goals [goal 2: zero hunger, achieve food security and improved nutrition and promote sustainable agriculture] are in line with this review.
|
1. Introduction
The first milk produced by a bovine after giving birth is termed colostrum, and it contains a wealth of natural macro- and micro-nutrients, immunoglobulins, peptides with anti-microbial activity and growth factors. Bovine colostrum differs from regular milk by containing lower lactose levels and higher protein levels, making it a potentially suitable option for individuals with lactose intolerance. It exhibits a slimy and viscous texture, a slight reddish-yellow color, a strong odour, and a bitter taste. Although, it is crucial for the growth, development, and immunological and nutritional support of the newborn calf. It is processed by the dairy industry for conservation and offered for sale as it can boost the immune system and improve overall human health.
Additionally, there is substantial evidence that bovine colostrum may be beneficial for treating a range of illnesses in both adults and children1,2 and as a supplement for athletes to aid exercise performance and recovery.3,4 Bovine colostrum has gained attention because of its therapeutic and medicinal properties, particularly during the COVID-19 pandemic. Research publications on colostrum and its health benefits have reportedly increased. Bovine colostrum is known for its immune support, nutritional richness, and potential antimicrobial properties. Fig. 1 shows an increased global trend of research related to colostrum and its health benefits; it would be helpful to analyse the details in Fig. 1 for a more comprehensive understanding.
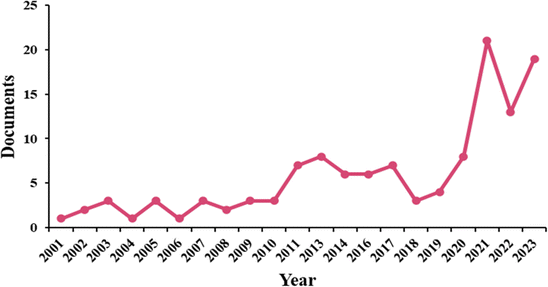 |
| Fig. 1 Global trend of research related to colostrum and its health benefits. | |
Currently, colostrum-developed cheeses and other traditional products showing popularity in European civilizations and those from Scandinavia, India, and other places.5 Furthermore, it has been dried to preserve nutritional contents which possess numerous health benefits.6 Colostrum preparations are sold in the US and EU with the promise of improving GI and immunity, among other health benefits. Although the idea of using dried colostrum, which is usually in the form of pills or tablets, seems appealing, there are drawbacks to this use because of the small quantity of colostrum taken in comparison to clinically established consumption rates. However, recent studies indicated that colostrum has important natural anti-microbial components that help to develop immunity in calves.7 The intake of colostrum enhances the function of GI8–12 and it showed effects in metabolic and endocrine systems.9,11 Colostrum contains bioactive protein, which shows numerous health benefits, such as the potentiality to repair skeletal muscle and improve immunity.13 Meanwhile, many research studies reported the development of neonatal immunity in newborn calves due to feeding of colostrum that contains components such as maternal immune cells, growth factors, cytokines, antimicrobial compounds and immunoglobulins.14–16
Moreover, colostrum from bovine origin showed anti-viral and anti-bacterial effects and can be considered as a nutraceutical.17 Its application is not limited to people; there is evidence that it is useful in animal husbandry and for the health and welfare of domestic pets and large animals.18–22 Currently, several studies also advocate its effect on gastrointestinal disease23 and immune function.24 Although this practice is linked to these cultural or regional beliefs, the wealth of well-characterized bioactive constituents and specific prebiotic substances in this diet may provide more scientific evidence in favour of this traditional knowledge. Traditionally, people used to consume liquid fresh colostrum, but pasteurised colostrum is now sold as a stand-alone beverage in certain stores; however, production is still very low.25 This systematic review provides a brief knowledge about bovine colostrum by discussing its composition, processing techniques for its preservation and its potential applications towards health benefits.
2. Methods
This systematic review aims to present a comprehensive exploration of colostrum as a nutraceutical, focusing on its medicinal properties. On December 25, 2023, an exhaustive search was conducted on Scopus, covering documents from 2000 to 2023. To ensure the inclusion of relevant information without language bias, papers in all languages were considered within the inclusion criteria. The Scopus advanced search employed the following query string:
TITLE-ABS-KEY ((“bovine colostrum*”) AND (“processing*”) OR (“health” OR medicinal* OR therapeutic))
The search results were exported in the .csv format, capturing essential details such as (i) citation, (ii) bibliography, (iii) abstract and keywords, (iv) funding details, and (v) other information. Exclusion criteria were rigorously applied to eliminate results lacking complete information. A meticulous review of all abstracts was carried out, excluding papers not directly relevant to the application of colostrum in human health. The selected papers were then obtained in full text and systematically organized using the Mendeley reference manager.
3. Results and discussion
The systematic review, initiated with a Scopus advanced search, unveiled 230 primary documents. Focusing on the technological advancements in bovine colostrum and their health benefits over the last 23 years (2000–2023), the database was strategically filtered to encompass articles specifically within the domain of “Agricultural and Biological Sciences, Veterinary and Medicine.” In the initial screening phase, 192 documents were carefully evaluated based on their titles and abstracts. Subsequently, a thorough review and categorization of each article using its title and abstract resulted in 179 papers deemed suitable for full-text evaluation. Following stringent criteria, including originality, relevance to the specific subject, and traceability, 160 articles were ultimately included. An illustrative overview of the comprehensive process is illustrated in Fig. 2.
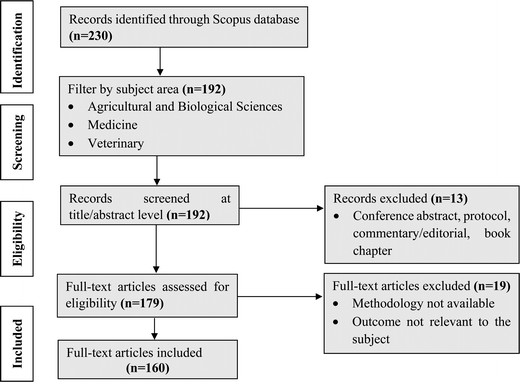 |
| Fig. 2 Study selection procedure flow. | |
4. Chemical composition of bovine colostrum
The composition of milk undergoes significant changes throughout lactation, and the content and nutritional value of bovine colostrum differ from those of mature milk.26Fig. 3 illustrates the nutritional and bioactive components present in bovine colostrum. Colostrum has a much lower lactose concentration and comparatively higher protein and fat content than mature milk as per Table 1.
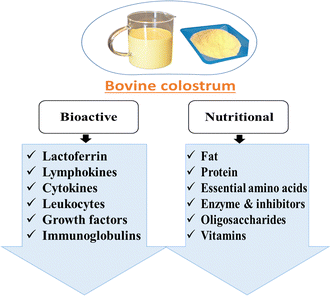 |
| Fig. 3 Nutritional and bioactive components of bovine colostrum. | |
Table 1 Compositional differences between bovine colostrum and mature milk
Constituents |
Bovine colostrum |
Mature milk |
References |
Fat (mg mL−1) |
64 |
39 |
27 and 26 |
67 |
— |
28
|
Lactose (mg mL−1) |
27 |
49 |
27 and 26 |
24 |
|
28
|
Protein (mg mL−1) |
140 |
36 |
27 and 26 |
149 |
|
28
|
Casein (mg mL−1) |
43 |
25 |
29
|
Whey (mg mL−1) |
120 |
5.10 |
29
|
Total solids (mg mL−1) |
276.40 |
125 |
28
|
Ash (mg mL−1) |
0.50 |
7 |
28
|
Immunoglobulins (mg mL−1) |
IgG |
55 |
0.26 |
27 and 30 |
IgA |
1.66 |
0.04–0.06 |
28, 31 and 32 |
IgM |
4.32 |
0.03–0.06 |
28, 31 and 33 |
Oligosaccharides (mg mL−1) |
— |
0.3–0.5 |
34
|
Lactoferrin (mg mL−1) |
0.82 |
0.10–0.30 |
35
|
Lactoperoxidase (mg mL−1) |
— |
13–30 |
36
|
Calcium (mg kg−1) |
4716 |
1220 |
28 and 37 |
1518 |
— |
38
|
Phosphorus (mg kg−1) |
4452 |
1520 |
28 and 37 |
1586 |
— |
38
|
Magnesium (mg kg−1) |
733 |
120 |
28 and 37 |
219.70 |
— |
38
|
Sodium (mg kg−1) |
1059 |
580 |
28 and 37 |
516.70 |
— |
38
|
Potassium (mg kg−1) |
2845 |
1520 |
28 and 37 |
1297 |
— |
38
|
Zinc (mg kg−1) |
38.10 |
5.30 |
28 and 37 |
151 |
— |
38
|
Iron (mg kg−1) |
5.33 |
0.80 |
28
|
34.66 |
— |
38
|
Manganese (mg kg−1) |
0.10 |
0.20 |
28 and 37 |
2.62 |
— |
38
|
Vitamin a (mg kg−1) |
4.90 |
460 |
28 and 37 |
Vitamin E (mg kg−1 of fat) |
77.17 |
2.10 |
28 and 37 |
Vitamin B12 (μg ml−1) |
0.60 |
4.50 |
28 and 37 |
This is in line with the requirements of the growing calf, for whom the health benefits of immunoglobulin transfer via passive means.39 Additionally, the mineral concentration of milk decreases in conjunction with the amount of milk produced throughout lactation (Table 1). So, colostrum can be an alternative to mature milk as it contains lower lactose but higher protein than mature milk. Moreover, protein is termed a complete food as its constituents, such as whey protein and amino acids, possess high protein digestibility,40 despite the fact that colostrum has higher quantities of less digestible immunoglobulins (Table 1).
While the current dietary guidelines for protein consumption are 0.8 g per kg per day for a normal adult who engages in little physical activity,41,42 an expanding amount of data points to a possible higher optimum intake of 1.2–1.6 g per kg per day,43–45 and to support the synthesis of skeletal muscle protein, this consumption has to be balanced with other food.46 The ideal protein intake to prevent the skeletal muscle loss that comes with age is frequently not reached, especially in older populations. This is made worse by the decreased proteolytic activity that comes with ageing.47 Colostrum may therefore provide a full, palatable, and easily digested protein supply that may be included in a diet that is calorie-appropriate. Bovine colostrum contains a variety of protein-derived bioactive peptides, vitamins, minerals, and other nutrients in addition to macronutrients that may enhance its macronutrient profile.
4.1 Fats
Compared to mature milk, colostrum has a larger amount of fat,64 and the content of these fats is also different. It has a larger concentration of palmitic, palmitoleic, and myristic acids than mature milk, according to research on the composition of colostrum and the changes that occur throughout the transition to mature milk.65 Despite the fact that these fat profiles are ideal for growing calves,66 these fat profiles as well as the higher concentration of saturated fat have been linked to long-term adverse health effects. However, the literature is divided on the degree to which dairy fats contribute to cardiovascular disease.67 There is evidence that these fatty acids function as signaling molecules and that they regulate the liver's process of lipogenesis as dietary fats.68
Additionally, removing these lipids lowers the content of certain fat-soluble vitamins contained in milk, such as vitamins D and A, in colostrum. The ability to efficiently separate fats from the aqueous fraction of milk—the portion that contains proteins, carbohydrates, minerals, and some vitamins—is relevant to consumers because it allows for the reduction or removal of these fats from colostrum prior to downstream processing, potentially opening the door to the production of low-fat or fat-free colostrum products. It is theorised that there might not always be a net advantage from the trade-off between dairy fats and the elimination of bioactive components that are present in the fat portion of dairy foods.67 Carefully monitored clinical trials examining the connection between dietary lipids in colostrum and health are required to address this discrepancy in the literature.
4.2 Vitamins or minerals present in colostrum
High concentrations of water- and fat-soluble vitamins, which are essential to human health, are also present in cow colostrum.69 Remarkably, retinoic acid, retinol, retinyl esters, retinal, and provitamin A carotenoids have all been observed to have substantial quantities of vitamin A in colostrum.70–72 Vitamin E, in the form of tocopherols and tocotrienols (∼77.17 mg kg−1), is found in low-density lipoproteins in colostrum.28,69
Phylloquinone and menaquinones, two forms of vitamin K, are also present in higher concentrations in colostrum than in mature milk.70 Colostrum contains higher amounts of vitamin D than mature milk.73 In addition to supporting the absorption of calcium and phosphorus in the small intestine, vitamin D plays significant functions in immunological function.74 It exists in two forms: cholecalciferol (vitamin D3) and ergocalciferol (vitamin D2). During the first five days after giving birth, their concentrations drop from 1.2 to 0.36 IU g−1.75 In addition, the water-soluble component of colostrum has higher concentrations of vitamin B and vitamin C than mature milk,76 which offers a natural source of these vital vitamins that are crucial for human health. Mature milk and colostrum from cows are considered to be excellent sources of many nutrients, particularly phosphorus and calcium.74 According to recent research, adult cow milk contains much lower mean quantities of several essential elements than colostrum. Calf development and the maintenance of their strong bones and teeth depend on calcium. Additionally, phosphorus is essential for the metabolic rate and physiological processes such as skeletal tissue growth, energy metabolism, protein synthesis, and fatty acid transport.77 Bovine colostrum contains zinc, selenium, and magnesium in quite substantial amounts.74
4.3 Bioactive proteins
4.3.1 Immunoglobulins (Igs).
A substantial portion of the total protein in cow colostrum is composed of complex proteins called immunoglobulins (Igs), also referred to as antibodies. Bovine colostrum primarily contains three distinct isotypes of immunoglobulins: IgG (IgG1 and IgG2), IgA, and IgM. In bovine colostrum, immunoglobulin G accounts for 85–90% of the total immunoglobulin content, making it the predominant immunoglobulin. In cow colostrum, IgG1 makes up 80–90% of the total IgG concentration, followed by IgM, IgA, and IgG2.17,78,79 These immunoglobulins destroy intestinal pathogens like bacteria, microorganisms, and viruses, and are crucial to the survival of calves. For many years, researchers have focused on the crucial topic of using cow colostrum as a source of antibody preparations to promote both human and cow health.80 Colostrum has a higher quantity of IgG than mature milk; in the first few days following birth, it can reach 50–100 mg mL−1. This is one of the key differentiators between colostrum and mature milk.31,81,82 Before parturition, the concentration of IgG1 and IgG2 in cow serum drops; these antibodies are transported from the blood into the colostrum. Almost all of the IgG found in colostrum actually comes from cow serum and is transported into the colostrum and milk.83,84
Since cows cannot transmit IgG through the placenta, calves' survival heavily depends on the transfer of IgG from cow colostrum to them in order to offer passive immunity. A large concentration of IgG is therefore required for calves to survive.85 In fact, calves not fed with colostrum showed higher infections and experienced greater rates of morbidity and mortality.29,86,87
4.3.2 Lactoferrin.
Lactoferrin (LF), a versatile glycoprotein with approximately 80 kDa molecular mass and 700 amino acids, exhibits two alpha-helix-connected lobes (N and C) capable of binding various metal ions (Cu2+, Zn2+, Mn3+, and ferric ion).88,89 Bovine colostrum contains around 0.80 mg mL−1 of lactoferrin, a cationic glycoprotein that binds iron.35 It is one of the main proteins in the milk serum of all mammals, which has a variety of uses, including antibacterial, antifungal, antiviral, antiparasitic, anticancer, and immunomodulatory (anti-inflammatory) properties.17,33,90–92 Lactoferrin obtained from bovine colostrum possess antibacterial activity by preventing the growth of bacteria, viruses, yeasts, and protozoa that cause disease. It also helps to preserve intestinal permeability and stability by preventing pathogens from adhering to epithelial cells.82,93,94
Furthermore, some research indicates that lactoferrin from bovine colostrum can boost the proliferation of cells called osteoblasts, which are involved in the production of bones, as well as the release of certain growth factors from these cells.95,96 Moreover, it has been established that it contributes to the intestinal absorption of iron and the stimulation of phagocytes and immunological responses. Intestinal tissue, monocytes, macrophages, neutrophils, lymphocytes, platelets, and some bacteria all express lactoferrin receptors.97 Supplements containing lactoferrin from cows are hypothesised to boost immunity and affect immune cell activity through several antioxidative properties.98 Colostrum contains the highest proportion of this protein, four times more than mature milk.99
In addition to this, Parkinson's disease originates from the death of dopaminergic cells, often induced by oxidative stress and glial dysfunction. Lactoferrin, a versatile glycoprotein present in brain regions, demonstrates a protective effect by reducing oxidative damage and initiating receptor-mediated transcytosis across the blood–brain barrier. This dual action suggests the potential use of colostrum-derived LF in transporting therapeutic agents for Parkinson's treatment.100
Furthermore, some researchers have reported the stability of LF. In simulated oral, gastric, and intestinal digestion, iron-saturated (holo)-, native-, and iron-depleted (apo)-bovine lactoferrin (LF) were hydrolyzed to <10 kDa peptides. Holo-LF released 93% iron within 2 hours of gastric digestion, with antioxidant activities 3–8 times higher during gastric digestion. Apo-LF exhibited higher antioxidant activity than holo-LF after gastric and intestinal digestion, with minor differences observed among the LF forms in surface charge and iron binding/release characteristics.101 This stability information can be valuable in the utilization of colostrum-derived LF.
4.3.3 Lactoperoxidase.
Bovine colostrum contains a significant amount of lactoperoxidase, a basic glycoprotein that catalyses the oxidation of thiocyanate to produce intermediate molecules having antimicrobial properties.102 In mature cow milk, lactoperoxidase concentrations range from 13 to 30 mg L−1, while in colostrum, it is between 11 and 45 mg L−1.36 Its initial concentration in cow colostrum is minimal, but three to five days after parturition, it reaches its maximum level. Additionally, compared to mature milk, the activity of lactoperoxidase catalase is higher in cow colostrum.103,104 Furthermore, toxic oxidation products are generated from lactoperoxidase activity and suppress bacterial metabolism via oxidation of the essential sulfhydryl group of proteins. It affected the Gram positive and Gram negative bacteria such as Pseudomonas aeruginosa, Salmonella typhimurium, Listeria monocytogenes, Streptococcus mutans, and Staphylococcus aureus.105 Poliovirus, HIV and vaccinia virus are also inhibited by the lactoperoxidase system.94,106,107
4.3.4 Oligosaccharides.
Oligosaccharides are found in a major quantity in bovine colostrum. Colostrum contains 0.7–1.2 mg mL−1 of oligosaccharides, most of which are acidic oligosaccharides, which are less prevalent in adult cow's milk.34,108 Thus far, forty different oligosaccharide compositions have been identified in cow colostrum.109–111 Cows differ from one another in terms of the total colostrum oligosaccharides due to genetic variability.112 Predominant oligosaccharides in bovine colostrum are 6′ siayllactosamine (6′SLN), 6′ sialyllactose (6′SL), 3′ sialyllactose (3′SL), and disialyllactose (DSL). 3′SL is 70% of total oligosaccharide content in bovine colostrum.108,110,113,114 The colostrum levels of 3′SL, 6′SL, and 6′SLN peaked after parturition and declined 48 hours after delivery, although levels of neutral oligosaccharides rose.104 Breed-specific variations in oligosaccharide content have also been noted. Colostrum from Jersey cows had concentrations of 3′SL, 6′SL, 6′SLN, and DSL of 867, 136, 220, and 283 μg mL−1, respectively; following parturition, and these values were 681, 243, 239, and 201 μg mL−1, respectively, in Holstein colostrum.115 The majority of the prebiotic elements in bovine colostrum are composed of both complex, conjugated N-glycans and free oligosaccharides, often known as bovine milk oligosaccharides, or BMOs.116 The majority of the prebiotic elements in bovine colostrum are composed of both complex, conjugated N-glycans and free oligosaccharides, often known as bovine milk oligosaccharides, or BMOs.116 BMOs are mostly sialylated, or acidic, oligosaccharides with a limited propensity for fucosylation, in contrast to human milk oligosaccharides or HMOs.109 Recent developments in enzymatic glycosylation have made it possible to improve the structural properties of BMOs. So, they more closely resemble HMOs.117 Complex milk processing has hindered BMO separation from high lactose concentrations,118 although methods are emerging,119 limiting its use in human nutrition and health. Although pilot trials with pure BMOs in adults have not shown generalizable alterations in GI microbial populations,120 future research in newborns may be more promising due to recent in vitro experiments.121,122
Bovine mature milk and colostrum include complex and hybrid N-glycans, which can be used as prebiotics in a manner similar to HMOs/BMOs.123 Additionally, conjugating N-glycans to milk proteins allows for various recovery techniques. Protein separation from lactose and treatment to separate N-glycans from their conjugates may be a promising method for purifying these glycans.124N-glycans from bovine colostrum, high in N-glycosylated proteins,125 may be a promising source of bioactive prebiotic substrates. Research reveals that complex N-glycans from bovine milk proteins in colostrum are highly selective for specific bacteria in the adult GI microbiome. Bifidobacterium species with access to these glycans are more limited than those with access to bigger repeating polymers of less complicated oligosaccharides (e.g., Bifidobacterium longum subsp. infantis). Several strains of these species have been linked to reduced intestinal inflammation and enhanced GI barrier function in humans.126,127 Recent research suggests that oligosaccharides from bovine colostrum may have anti-infective properties against a particularly invasive strain of C. jejuni.128
4.4 Factors influencing colostrum composition
Numerous factors influence the composition and physical characteristics of colostrum, including individuality, parity, breed, nutrition, pre-partum, time post-partum and duration of the dry period in cows. In general, colostrum exhibits higher levels of nucleotides, cytokines, growth factors, hormones, minerals and vitamins, ash, non-protein nitrogen, peptides, protein and fat, while containing lower levels of lactose compared to mature milk. The concentration of these components undergoes a rapid decline within the initial 3 days of lactation, with the exception of lactose content.48–50
4.4.1 Individual differences in animals.
The quality of cow colostrum varies based on individual and genetic backgrounds.29 For instance, different cattle exhibit varying levels of immunoglobulin G (IgG) in their colostrum, as well as different volumes during their first milking.51,52 Among the breeds examined, Friesian-Holstein cows produce the lowest quantities of IgG (41.2 g L−1), while Jersey cows yield the highest (66.5 g L−1).53 In terms of cow parity, first-calf heifers generate less colostrum and have lower IgG concentrations compared to cows in their second or subsequent lactations. Following the second calving, the quality of bovine colostrum improves with parity, with older cows often providing the highest-quality colostrum.52
Another unique factor influencing the quality of cow colostrum is illness. For instance, low-quality colostrum is a detrimental outcome of mastitis, an inflammation of the cow's mammary gland. Cows with infected mammary glands exhibit reduced volumes and concentrations of bovine colostrum IgG compared to those without infection.54 Additionally, the age of the cows also impacts the quality of colostrum. The findings of several studies generally support the notion that older cows produce higher-quality colostrum than younger cows.51,52,55 It is hypothesized that increased pathogen exposure, enhanced immunity, and body condition score contribute to the correlation between older age and high-quality colostrum.29
4.4.2 Environmental factors.
The amount of IgG in the colostrum is greatly influenced by the time of colostrum milking after calf delivery. The IgG content in colostrum will be considerably improved by early or instant colostrum milking. Colostrum obtained 6, 10, and 14 hours after delivery is said to have a lower IgG content than colostrum collected 2 hours after delivery.56 Additionally, a different study revealed that the quality of bovine colostrum in North American herds peaks right after parturition and falls off if milking is postponed.51,56 The season of calving has an impact on the quality of bovine colostrum as well.
In comparison to cows calving in the autumn, summer-calving cows produce colostrum of inferior quality.51 The calving season has an impact on the proportion of fat in cow colostrum at 24 and 48 hours after delivery. The fat percentage of colostrum is higher in winter than the spring and summer. Seasonal variations in water, nutrition, and metabolism might be one of the causes.57,58
The approximately six to eight-week dry period is a critical time for cows as this time is necessary for fetal development, regeneration of milk secretion tissue, and lactation preparation.59–61 During the final 15–20 days of the dry period, colostrum begins to be released, and its composition continues to change until parturition.60,62 It is even reported that the length of the dry period and the concentration of IgG have a positive correlation.63
5. Technologies used to preserve bovine colostrum
Newborn calves are born agammaglobulinemia, relying on the timely consumption of maternal colostrum for immunity through passive transfer. However, various factors, including multiple births, acute mastitis, and maladapted maternal behaviour, can impede access to colostrum resulting in shortages. Deliberate discarding of colostrum from cows infected with pathogens further exacerbates these challenges. In the UK, colostrum is often harvested and stored, but storage conditions, such as leaving it uncovered at room temperature, can lead to bacterial contamination. Bacterial contamination in colostrum samples is widespread, exceeding threshold limits in individual and pooled samples.129 Coliform species, in particular, have been identified as detrimental to IgG absorption through various mechanisms.130 To mitigate bacterial contamination, producers are advised to employ rigorous hygiene practices, including teat cleaning, equipment scrubbing, and the use of sterile bags. The preservation of bovine colostrum is a critical aspect in ensuring the retention of its essential bioactive components. Preserving high-quality, microbe-free colostrum is crucial for producing colostrum-based products for human consumption.131,132 Thermal treatments, such as pasteurization and sterilization, are effective in eliminating pathogens and ensuring high-quality colostrum production for human consumption.131,133 Techniques such as lyophilization, spray-drying, pasteurization, and emerging technologies like high-pressure processing are being used to preserve bovine colostrum. The positive and negative aspects of the processing technologies for colostrum preservation are shown in Table 2.
Table 2 Merits and demerits of the preservation techniques for colostrum preservation
Preservation technique |
Merits |
Demerits |
Thermal treatments |
HTST pasteurization |
Reduces bacterial counts in colostrum and inactivate Mycobacterium avium subsp. paratuberculosis, Salmonella, and Mycoplasma species135,136,138–140,144 |
Strict method of preservation137 |
Enhances immunity138–140 |
Heating colostrum beyond 60 °C can damage IgG molecules141,142 |
Weight gain in dairy calves138–140 |
High capital costs associated with its plant implementation144 |
LTLT pasteurization |
Reduces bacterial contaminants133 |
Allows some bacteria survival133 |
|
Decrease in IgG concentration (12.3–58.5%)135,147 |
UHT |
Ensures aseptic handling and prolonged stability148 |
Reduction (15–35%) in IgG concentration148 |
Spray drying |
Cost-effective139 |
— |
Preservation of immunoglobulin function139 |
— |
Non-thermal treatment |
Microfiltration |
Efficient in removing bacteria and preserving bioactive components in colostrum145 |
— |
|
Recovers ∼80% of IgG and other whey proteins146 |
— |
Lyophilization |
Retains immunoglobulin concentration145 |
30% lower absorption of IgG by the calf143 |
High pressure processing |
Retains 20% more bovine IgG than soy milk during heat treatment (75–78 °C)150 |
Application of >400 MPa altered viscosity and denatured IgG152 |
|
Effective in suppression of bacterial growth152 |
— |
5.1 Thermal and non-thermal treatments
Pasteurization, a heat-treatment process, aims to eliminate pathogenic microorganisms in beverages and foods, reducing their viability rather than destroying all microorganisms.133,134 High-temperature short-time (HTST) pasteurization, involving rapid heating to 72 °C for 15 seconds, is a common method for colostrum. It is effective against pathogens like Mycobacterium paratuberculosis.135,136 Furthermore, pasteurization is not considered as a strict method of preservation; it serves as a valuable tool for the storage and management of colostrum shelf life. It is reported that a higher bacterial concentration in the calf's gut could negatively impact the passive transfer of IgG.137 Numerous studies have confirmed that heat treatment, resulting in reduced bacterial counts in colostrum, contributes to enhanced immunity and weight gain in dairy calves.138–140 However, heating colostrum beyond 60 °C can damage IgG molecules. Immunoglobulins, which are mono- or polymeric proteins (formed by two light and two heavy polypeptide chains connected by disulphide bonds into a Y-shaped structure), can undergo initially reversible unfolding under excessive heat treatment.141 This unfolding results in the loss of a globular configuration and may progress to irreversible denaturation and aggregation through hydrophobic and disulphide interactions.142
The effects of colostrum stored under various conditions and fed to Irish spring-born calves were investigated. It was found that pasteurized colostrum led to serum IgG concentrations twice as high as that in colostrum stored under warm conditions (∼22 °C).143 Furthermore, pasteurization has proven effective in destroying Mycobacterium avium subsp. paratuberculosis, Salmonella, and Mycoplasma species in milk deliberately spiked with these organisms.144 Despite these advantages, the adoption of the pasteurization process in UK dairy farms is limited due to the substantial capital costs associated with its plant implementation.144
Microfiltration, especially with Tetra Pak's Bactocatch system, offers an alternative to extend shelf-life, efficiently removing bacteria and preserving bioactive components in colostrum.145 The membrane filtration process in studies has shown a recovery of at least 80% of IgG and other whey proteins.146
Low-temperature long-time (LTLT) pasteurization, also known as holder pasteurization, involves heating a batch of colostrum to 63 °C for a minimum of 30 minutes, reducing bacterial contaminants but allowing some bacteria survival.133 Studies indicate varying effects on IgG concentration; while Meylan et al. (1996) observed a 12.3% decrease,147 Godden et al. (2003) reported a 58.5% decline.135 Extended pasteurization times, such as 60 minutes at 60 °C, can reduce bacteria concentration and improve serum IgG levels, but with potential viscosity changes.135
Sterilization, achieved through methods like heating, ionizing radiation, or high hydrostatic pressure processing, involves the irreversible inactivation or destruction of all viable organisms, including bacterial spores. The ultra-high temperature (UHT) process, a common sterilization method, heats dairy products to 135 °C for a brief period (2–5 s), extending shelf-life at room temperature. While UHT treatment ensures aseptic handling and prolonged stability, it results in a significant reduction (15–35%) in IgG concentration compared to pasteurization, with nearly negligible functional IgG concentrations.148
5.2 Lyophilisation, spray drying or freeze drying
Lyophilization or freeze drying is a drying process which generally involves drying at lower temperatures and under vacuum conditions. It stands out as the most preferred dehydration method for heat-sensitive biological materials but has an adverse effect on the fat that leads to rapid spoilage. Additionally, the absorption of IgG by the calf from lyophilized colostrum is reported to be 30% lower than that from fresh colostrum.143 Research studies have also indicated that freeze-drying does not alter the concentration of immunoglobulins in colostrum.144 It is reported that around 34% and 25% of total immunoglobulins were lost during freeze-drying of colostrum.145 Data from first milking of postpartum colostrum samples (18 Egyptian buffaloes and 36 Holstein cows) showed that freeze-dried colostrum (at 7 °C for 3 months) had significantly reduced IgG concentrations compared with frozen colostrum.138 In contrast, spray-drying has been demonstrated to produce dried colostrum wherein both the quantity and function of immunoglobulins are preserved. This method proves to be the most cost-effective in maintaining the therapeutic potential of colostrum for human consumption.139
Furthermore, it is reported that a combination of ultrasonication, fermentation, and dehydration could potentially be employed to reduce microbial contamination of bovine colostrum. However, further investigations are necessary to assess the impact of these treatment methods on sensitive biologically active compounds in bovine colostrum.149
5.3 High pressure processing
High-pressure processing has emerged as a promising preservation method for colostrum immunoglobulins.143 It retained 20% more bovine IgG as compared to soy milk during heat treatment (75–78 °C).150 However, IgA molecules in human breast milk were destroyed during high-pressure processing.151 The effective suppression of bacterial growth was observed for 9 days at 4 °C after treating the colostrum at 300 and 400 MPa for 10 minutes. IgG remained intact till 300 MPa, but applying 400 MPa resulted in altered viscosity of the colostrum and denaturation of IgG.152 It was found that IgG was stable till 400 MPa, if the duration of treatment was limited to 30 minutes. However, increasing pressure (500 or 600 MPa) or extending the duration resulted in increased denaturation and aggregation.138,153
Advanced technologies such as nanotechnology and liposomal technology are employed to enhance the preparation of bovine colostrum for human consumption, offering numerous benefits. Nanotechnology, operating on an ultra-small scale, modifies the physicochemical properties of materials, providing opportunities for novel product development in various fields, including the dairy industry.154 Nanoencapsulation within nanometer-sized vesicles safeguards fragile bioactive proteins in the dairy industry, preventing denaturation or degradation.155 Liposomal technology, another advanced method, is utilized to preserve bovine colostrum and enhance its bioavailability for human health.98,156 Liposomal encapsulation demonstrates controlled release, increased bioavailability, and protection against environmental factors.157
Bovine colostrum, processed using these advanced technologies, is consumed by humans in various forms, such as pills/tablets, powder, cheese and liquid. In dairy products, liquid bovine colostrum is incorporated into items like cheese and yoghurt, enhancing sensory acceptance and nutritional content.158 In dietary supplements, bovine colostrum is available in different forms, including powder, liquid, tablets, or pills, with specialized drying processes like low heat spray drying or freeze drying to prevent protein denaturation.159,160 These supplements are popular for improving health, particularly among athletes seeking benefits such as reduced exercise-induced muscle damage and inflammation.4,161 Additionally, bovine colostrum is utilized for preventing diarrhea, treating AIDS-related gastrointestinal diseases, promoting overall gut health and enhancing immune system function,162,163 with evidence suggesting tissue repair, maintenance of gastrointestinal mucosal integrity and immune modulation.
6. Therapeutic applications of colostrum
Bovine colostrum exhibits a diverse array of potential health benefits (Fig. 4), which are discussed below in a summarized manner.
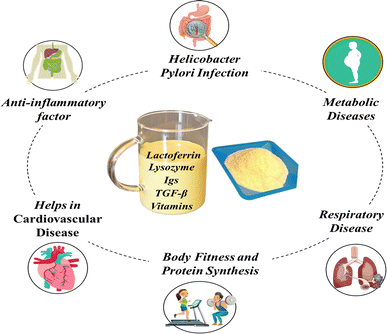 |
| Fig. 4 Therapeutic properties of bovine colostrum. | |
6.1 Effect in cardiovascular disease
Bovine colostrum possesses advantageous properties that have the potential to reduce the levels of triglycerides (TGs), total cholesterol, ketones, and blood glucose, making it a promising supplement for promoting heart health.164 Additionally, colostrum contains leptin, a hormone-like substance that can contribute to the functioning and repair of heart muscle.165,166 Heart muscle cells act as feature receptors for both the growth hormone and IGF-1, with scientific evidence suggesting that the growth hormone may directly influence the heart. At the same time, the effects of IGF-1 could be indirect, operating through separate hormonal pathways. The presence of these substances in bovine colostrum underscores its potential as a valuable supplement for supporting heart health. Consequently, bovine colostrum may emerge as a promising natural alternative for individuals seeking to bolster their cardiovascular well-being.167
6.2 Effect on metabolic diseases
Both type I and type II diabetes have a genetic component, leading to lower IGF-1 levels in individuals with diabetes.166,168 Bovine colostrum is a rich source of IGF-1, which can be a beneficial supplement, restoring hormone levels and improving glucose utilization. This is crucial for type I diabetics reliant on insulin and can also benefit type II diabetics by reducing blood and urine glucose levels.169 Additionally, lactoferrin, a component of colostrum, plays a protective role against prion protein (PrP)-induced neurotoxicity. This protective mechanism involves ensuring the stability of hypoxia-inducible factor-1 alpha (HIF-1α) and increasing the expression of cellular prion protein (PrPc) in neuronal cells. Moreover, lactoferrin facilitates the stabilization of HIF-1α by regulating the expression and enzymatic activity of prolyl-hydroxylases 2 (PHD2).170
6.3 Effect on respiratory disease
The immune system is less effective in combating respiratory disorders. This decline is attributed to the shrinking of the thymus gland, responsible for producing T-lymphocytes that play a crucial role in regulating the immune system. However, research indicates that colostrum harbor growth factors capable of restoring the thymus gland to its normal function.171,172 T-lymphocytes, integral immune cells for combating infections, particularly killer T-lymphocytes, generate cell-mediated responses. Helper or suppressor T-lymphocytes further regulate and strengthen the immune response. The consumption of colostrum, rich in growth factors supporting the thymus gland, may enable individuals to enhance their immune system and more effectively combat respiratory disorders.173,174
6.4 Preventive effect against Helicobacter pylori infection
Helicobacter pylori (H. pylori) is a bacterium known to induce stomach-related issues. One mechanism through which H. pylori infects the stomach involves adhering to the lining using fat.175 Nevertheless, research has demonstrated that colostrum can effectively inhibit the adherence of H. pylori to the stomach lining, thereby preventing issues such as stomach ulcers.176 Colostrum contains components that can bind to the same sites in the stomach as H. pylori, impeding the bacteria's ability to attach. Utilizing colostrum may serve as a valuable means of protection against H. pylori infection, particularly for individuals unable to breastfeed.
6.5 Effective in body fitness
As individuals age, the tendency to experience muscle loss and challenges in fat reduction becomes apparent. However, colostrum, enriched with IGF-1, can stimulate the growth of lean muscle tissue and prompt the body to utilize fat for energy. In the presence of IGF-1, the body undergoes a shift in the primary fuel source for exercise from carbohydrates or glucose to fat, thereby increasing fat burning.167 This property holds potential benefits for individuals aiming to lose weight or enhance their body composition. Furthermore, the nutritional quality of colostrum consumed by calves plays a pivotal role in influencing their immune system and intestinal health.
6.6 Combat against COVID-19
The highly contagious SARS-CoV-2 virus caused COVID-19, which primarily affects the respiratory system, manifesting symptoms like cough, fever, and difficulty breathing.177 In severe cases, it can lead to pneumonia, acute respiratory distress syndrome (ARDS), and fatalities. While vaccines are being administered globally, the quest for effective treatments for infected individuals persists. Bovine colostrum, containing bioactive molecules, presents potential benefits for Covid-19 patients.178 A protein in bovine colostrum, lactoferrin, exhibits antiviral, antibacterial, and anti-inflammatory properties. It has demonstrated the ability to inhibit SARS-CoV-2 replication in vitro and may assist in mitigating inflammation in the lungs and other organs. High levels of immunoglobulins in bovine colostrum act as antibodies, aiding in the fight against infections.143 These antibodies can potentially neutralize the SARS-CoV-2 virus, reducing the severity and duration of COVID-19.179–181 Growth factors, including insulin-like growth factor-1 (IGF-1) and transforming growth factor-beta (TGF-β), support the growth and repair of tissues damaged by Covid-19.182,183 Prebiotic compounds promote the growth of beneficial gut bacteria. This can enhance gut health, crucial in Covid-19, which may induce gastrointestinal symptoms, thereby supporting overall immune function.
7. Conclusion
Bovine colostrum emerges as a valuable reservoir of nutritional and bioactive components, showcasing potential benefits across diverse dimensions of human health. Its role in reinforcing the immune system, averting infections, supporting metabolic health, optimizing body composition, and promoting cardiovascular well-being is underscored. Notably, the presence of immunoglobulins and lactoferrin positions bovine colostrum as a natural alternative for individuals seeking health fortification, particularly amid the ongoing COVID-19 pandemic.
This systematic review highlights the combined benefits of lysozyme, lactoperoxidase, proline-rich polypeptides, growth factors, metabolic factors, and minor nutrients in bovine colostrum. The integration of bovine colostrum into a balanced diet emerges as a promising strategy for sustaining and enhancing overall health in comparison with mature milk. This information provides a foundation for researchers, food technologists, dairy technologists, and industrialists to explore new possibilities for bovine colostrum as an emerging nutraceutical.
Conflicts of interest
There is no conflict of interest.
Acknowledgements
The authors would like to acknowledge the Director, ICAR-National Dairy Research Institute, Karnal for the providing support during the collection of literature and drafting this review.
References
- Y. Panahi, G. Falahi, M. Falahpour, Y. Moharamzad, M. R. Khorasgani, F. Beiraghdar and M. M. Naghizadeh, Bovine colostrum in the management of nonorganic failure to thrive: a randomized clinical trial, J. Pediatr. Gastroenterol. Nutr., 2010, 50, 551–554 CrossRef CAS PubMed.
- R. J. Playford, C. E. Macdonald, D. P. Calnan, D. N. Floyd, T. Podas, W. Johnson, A. C. Wicks, O. Bashir and T. Marchbank, Co-administration of the health food supplement, bovine colostrum, reduces the acute non-steroidal anti-inflammatory drug-induced increase in intestinal permeability, Clin. Sci., 2001, 100, 627–633 CrossRef CAS.
- J. D. Buckley, G. D. Brinkworth and M. J. Abbott, Effect of bovine colostrum on anaerobic exercise performance and plasma insulin-like growth factor, I, J. Sports Sci., 2003, 21, 577–588 CrossRef PubMed.
- Y. Kotsis, A. Mikellidi, C. Aresti, E. Persia, A. Sotiropoulos, D. B. Panagiotakos, S. Antonopoulou and T. Nomikos, A low-dose, 6-week bovine colostrum supplementation maintains performance and attenuates inflammatory indices following a loughborough intermittent shuttle test in soccer players, Eur. J. Nutr., 2018, 57, 1181–1195 CrossRef CAS PubMed.
-
J. M. Rocha, Scientific and Medical Research Related to Bovine Colostrum its Relationship and Use in the Treatment of Disease in Humans Selected, 2016 Search PubMed.
- E. G. Silva, A. H. Rangel, L. Mürmam, M. F. Bezerra and J. P. Oliveir, Bovine colostrum: benefits of its use in human food, Food Sci. Technol., 2019, 39, 355–362, DOI:10.1590/fst.14619.
- A. C. M. Van Hooijdonk, K. D. Kussendrager and J. M. Steijns,
In vivo antimicrobial and antiviral activity of components in bovine milk and colostrum involved in non-specific defence, Br. J. Nutr., 2000, 84, 127–134, DOI:10.1017/S000711450000235X.
- J. W. Blum and H. M. Hammon, Bovine colostrum – more than just an immunoglobulin supplier, Schweiz. Arch. Tierheilkd., 2000, 142, 221–228 CAS.
- J. Blum and H. Hammon, Colostrum effects on the gastrointestinal tract, and on nutritional, endocrine and metabolic parameters in neonatal calves, Livest. Prod. Sci., 2000, 66, 151–159, DOI:10.1016/S0301-6226(00)00222-0.
- U. Hadorn, H. Hammon, R. M. Bruckmaier and J. W. Blum, Delaying colostrum intake by one day has important effects on metabolic traits and on gastrointestinal and metabolic hormones in neonatal calves, J. Nutr., 1997, 127, 2011–2023, DOI:10.1093/jn/127.10.2011.
- P. Guilloteau, I. L. Huërou-Luron, J. A. Chayvialle, R. Toullec, R. Zabielski and J. W. Blum, Gut regulatory peptides in young cattle and sheep, J. Vet. Med. Ser. A, 1997, 44, 1–23, DOI:10.1111/j.1439-0442.1997.tb01082.x.
- C. Bühler, H. Hammon, G. L. Rossi and J. W. Blum, Small intestinal morphology in eight-day-old calves fed colostrum for different durations or only milk replacer and treated with long-R3-insulin-like growth factor i and growth hormone, J. Anim. Sci., 1998, 76, 758–765, DOI:10.2527/1998.763758x.
- H. J. Korhonen, Production and properties of health-promoting proteins and peptides from bovine colostrum and milk, Cell. Mol. Biol., 2013, 59, 12–24, DOI:10.1170/T943.
- M. Goto, M. Maruyama, K. Kitadate, R. Kirisawa, Y. Obata and M. Koiwa,
et al., Detection of interleukin-1. BETA. In sera and colostrum of dairy cattle and in sera of neonates, J. Vet. Med. Sci., 1997, 59, 437–441, DOI:10.1292/jvms.59.437.
- A. J. Reber, A. Lockwood, A. R. Hippen and D. J. Hurley, Colostrum induced phenotypic and trafficking changes in maternal mononuclear cells in a peripheral blood leukocyte model for study of leukocyte transfer to the neonatal calf, Vet. Immunol. Immunopathol., 2006, 109, 139–150, DOI:10.1016/j.vetimm.2005.08.014.
- H. Yamanaka, K. Hagiwara, R. Kirisawa and H. Iwai, Proinflammatory cytokines in bovine colostrum potentiate the mitogenic response of peripheral blood mononuclear cells from newborn calves through IL-2 and CD25 expression, Microbiol. Immunol., 2003, 47, 461–468, DOI:10.1111/j.1348-0421.2003.tb03371.x.
- S. Bagwe, L. J. P. Tharappel, G. Kaur and H. S. Buttar, Bovine colostrum: an emerging nutraceutical, J. Complementary Integr. Med., 2015, 12, 175–185, DOI:10.1515/jcim-2014-0039.
- C. K. Fenger, T. Tobin, P. J. Casey, E. A. Roualdes, J. L. Langemeier and D. M. Haines, Bovine colostrum supplementation optimises earnings, performance and recovery in racing thoroughbreds, Comp. Exerc. Physiol., 2014, 10, 233–238 Search PubMed.
- C. K. Fenger, T. Tobin, P. J. Casey, E. A. Roualdes, J. L. Langemeier, R. Cowles and D. M. Haines, Enhanced bovine colostrum supplementation shortens the duration of respiratory disease in thoroughbred yearlings, J. Equine Vet. Sci., 2016, 42, 77–81 CrossRef.
- E. Satyaraj, A. Reynolds, R. Pelker, J. Labuda, P. Zhang and P. Sun, Supplementation of diets with bovine colostrum influences immune function in dogs, Br. J. Nutr., 2013, 110, 2216–2221 CrossRef CAS PubMed.
- U. Hadorn, H. Hammon, R. M. Bruckmaier and J. W. Blum, Delaying colostrum intake by one day has important effects on metabolic traits and on gastrointestinal and metabolic hormones in neonatal calves, J. Nutr., 1997, 127, 2011–2023 CrossRef CAS PubMed.
- M. Yvon, D. Levieux, M. C. Valluy, J. P. Pelissier and P. P. Mirand, Colostrum protein digestion in newborn lambs, J. Nutr., 1993, 123, 586–596 CrossRef CAS PubMed.
- K. Chandwe and P. Kelly, Colostrum therapy for human gastrointestinal health and disease, Nutrients, 2021, 13, 1956, DOI:10.3390/nu13061956.
- S. Ghosh and M. Iacucci, Diverse immune effects of bovine colostrum and benefits in human health and disease, Nutrients, 2021, 13, 3798, DOI:10.3390/nu13113798.
- A. W. Scammell, Production and uses of colostrum, Aust. J. Dairy Technol., 2001, 56, 74–82 CAS.
- J. A. Foley and D. E. Otterby, Availability, storage, treatment, composition, and feeding value of surplus colostrum: a review, J. Dairy Sci., 1978, 61, 1033–1060, DOI:10.3168/jds.S0022-0302(78)83686-8.
- A. Dunn, A. Ashfield, B. Earley, M. Welsh, A. Gordon and S. J. Morrison, Evaluation of factors associated with immunoglobulin G, fat, protein, and lactose concentrations in bovine colostrum and colostrum management practices in grassland-based dairy systems in Northern Ireland, J. Dairy Sci., 2017, 100, 2068–2079, DOI:10.3168/jds.2016-11724.
- S. I. Kehoe, B. M. Jayarao and A. J. Heinrichs, A survey of bovine colostrum composition and colostrum management practices on pennsylvania dairy farms, 2007 farms, J. Dairy Sci., 2007, 90, 4108–4116, DOI:10.3168/jds.2007-0040.
- S. Godden, Colostrum management for dairy calves, Vet. Clin. N. Am. Food Anim. Pract., 2008, 24, 19–39, DOI:10.1016/j.cvfa.2007.10.00.
- C. Conesa, M. Lavilla, L. Sánchez, M. D. Pérez, L. Mata and P. Razquín,
et al., Determination of IgG levels in bovine bulk milk samples from different regions of Spain, 2005 different regions of Spain, Eur. Food Res. Technol., 2005, 220, 222–225, DOI:10.1007/s00217-004-1016-0.
- K. Stelwagen, E. Carpenter, B. Haigh, A. Hodgkinson and T. T. Wheeler, Immune components of bovine colostrum and milk, J. Anim. Sci., 2009, 87, 3–9, DOI:10.2527/jas.2008-1377.
- R. Pakkanen and J. Aalto, Growth factors and antimicrobial factors of bovine colostrum, Int. Dairy J., 1997, 7, 285–297, DOI:10.1016/S0958-6946(97)00022-8.
- A. Mero, H. Miikkulainen, J. Riski, R. Pakkanen, J. Aalto and T. Takala, Effects of bovine colostrum supplementation on serum IGF-I, IgG, hormone, and saliva IgA during training, J. Appl. Physiol., 1997, 83, 1144–1151, DOI:10.1152/jappl.1997.83.4.1144.
- S. J. T. Bruggencate, I. M. Bovee-Oudenhoven, A. L. Feitsma, E. Van Hoffen and M. H. Schoterman, Functional role and mechanisms of sialyllactose and other sialylated milk oligosaccharides, Nutr. Rev., 2014, 72, 377–389, DOI:10.1111/nure.12106.
-
H. S. Buttar, S. M. Bagwe, S. K. Bhullar and G. Kaur, Health benefits of bovine colostrum in children and adults, in Dairy in Human Health and Disease across the Lifespan, ed. R. R. Watson, R. J. Collier and V. R. Preedy, Elsevier, 2017, pp. 3–20 Search PubMed.
- R. Hahn, P. Schulz, C. Schaupp and A. Jungbauer, Bovine whey fractionation based on cation-exchange chromatography, J. Chromatogr. A, 1998, 795, 277–287, DOI:10.1016/S0021-9673(97)01030-3.
- I. T. Khan, M. Nadeem, M. Imran, R. Ullah, M. Ajmal and M. H. Jaspal, Antioxidant properties of milk and dairy products: a comprehensive review of the current knowledge, Lipids Health Dis., 2019, 18, 41, DOI:10.1186/s12944-019-0969-8.
- N. D. Dande and J. Nande, Nutritional composition of bovine colostrum: palatability evaluation of food products prepared using bovine colostrum, Int. J. Nutr. Pharmacol. Neurol. Dis., 2020, 10, 8–13, DOI:10.4103/ijnpnd.ijnpnd_77_19.
- D. M. Weaver, J. W. Tyler, D. C. VanMetre, D. E. Hostetler and G. M. Barrington, Passive transfer of colostral immunoglobulins in calves, J. Vet. Intern. Med., 2000, 14, 569–577, DOI:10.1111/j.1939-1676.2000.tb02278.x.
-
K. Stelwagen, Mammary gland, milk biosynthesis and secretion milk protein, in Encyclopedia of Dairy Sciences, ed. J. W. Fuquay, Elsevier, 2011, pp. 359–366 Search PubMed.
- M. Richter, K. Baerlocher, J. M. Bauer, I. Elmadfa, H. Heseker and E. Leschik-Bonnet,
et al., Revised reference values for the intake of protein, Ann. Nutr. Metab., 2019, 74, 242–250, DOI:10.1159/000499374.
- J. Y. Dong, Z. L. Zhang, P. Y. Wang and L. Q. Qin, Effects of high-protein diets on body weight, glycaemic control, blood lipids and blood pressure in type 2 diabetes: meta-analysis of randomised controlled trials, Br. J. Nutr., 2013, 110, 781–789, DOI:10.1017/S0007114513002055.
- J. Y. Dong, Z. L. Zhang, P. Y. Wang and L. Q. Qin, Effects of high-protein diets on body weight, glycaemic control, blood lipids and blood pressure in type 2 diabetes: meta-analysis of randomised controlled trials, Br. J. Nutr., 2013, 110, 781–789, DOI:10.1017/S0007114513002055.
- N. Santesso, E. A. Akl, M. Bianchi, A. Mente, R. Mustafa and D. Heels-Ansdell,
et al., Effects of higher-versus lower-protein diets on health outcomes: a systematic review and meta-analysis, Eur. J. Clin. Nutr., 2012, 66, 780–788, DOI:10.1038/ejcn.2012.37.
- T. P. Wycherley, J. D. Buckley, M. Noakes, P. M. Clifton and G. D. Brinkworth, Comparison of the effects of weight loss from a high-protein versus standard-protein energy-restricted diet on strength and aerobic capacity in overweight and obese men, Eur. J. Nutr., 2013, 52, 317–325, DOI:10.1007/s00394-012-0338-0.
- L. E. Norton and G. J. Wilson, Optimal protein intake to maximize muscle protein synthesis examinations of optimal meal protein intake and frequency for athletes, 2009 athletes, Agro Food Ind. Hi-Tech., 2009, 20, 54–57 CAS.
- R. R. Deer and E. Volpi, Protein intake and muscle function in older adults, 2015 adults, Curr. Opin. Clin. Nutr. Metab Care, 2015, 18, 248–253, DOI:10.1097/MCO.0000000000000162.
- R. Moreno-Rojas, M. A. Amaro-Lopez and G. Zurera-Cosano, Mkronutrients in natural cow, ewe and goat milk, Int. J. Food Sci. Nutr., 1993, 44, 37–46, DOI:10.3109/09637489309017421.
- W. Nowak, R. Mikuła, A. Zachwieja, K. Paczynska, E. Pecka and K. Drzazga,
et al., The impact of cow nutrition in the dry period on colostrum quality and immune status of calves, Pol. J. Vet. Sci., 2012, 15, 77–82, DOI:10.2478/v10181-011-0117-5.
- I. Hyrslova and G. Krausova, Goat and bovine colostrum as a basis for new probiotic functional foods and dietary supplements, J. Microb. Biochem. Technol., 2016, 08, 56–59, DOI:10.4172/1948-5948.1000262.
- D. E. Morin, P. D. Constable, F. P. Maunsell and G. C. McCoy, Factors associated with colostral specific gravity in dairy cows, J. Dairy Sci., 2001, 84, 937–943, DOI:10.3168/jds.S0022-0302(01)74551-1.
- K. M. Morrill, E. Conrad, A. Lago, J. Campbell, J. Quigley and H. Tyler, Nationwide evaluation of quality and composition of colostrum on dairy farms in the United States, 2012 United States, J. Dairy Sci., 2012, 95, 3997–4005, DOI:10.3168/jds.2011-5174.
- L. D. Muller and D. K. Ellinger, Colostral immunoglobulin concentrations among breeds of dairy cattle, J. Dairy Sci., 1981, 64, 1727–1730, DOI:10.3168/jds.S0022-0302(81)82754-3.
- F. P. Maunsell, D. E. Morin, P. D. Constable, W. L. Hurley, G. C. McCoy and I. Kakoma,
et al., Effects of mastitis on the volume and composition of colostrum produced by holstein cows, J. Dairy Sci., 1998, 81, 1291–1299, DOI:10.3168/jds.S0022-0302(98)75691-7.
- S. M. Gulliksen, K. I. Lie, L. Sølverød and O. Østerås, Risk factors associated with colostrum quality in norwegian dairy cows, J. Dairy Sci., 2008, 91, 704–712, DOI:10.3168/jds.2007-0450.
- M. Moore, J. W. Tyler, M. Chigerwe, M. E. Dawes and J. R. Middleton, Effect of delayed colostrum collection on colostral IgG concentration in dairy cows, J. Am. Vet. Med. Assoc., 2005, 226, 1375–1377, DOI:10.2460/javma.2005.226.1375.
- H. Erdem and I. C. Okuyucu, Non-genetic factors affecting some colostrum quality traits in holstein cattle, Pak. J. Zool., 2020, 52, 557–564, DOI:10.17582/journal.pjz/20190219100236.
- J. W. West, Effects of heat-stress on production in dairy cattle, J. Dairy Sci., 2003, 86, 2131–2144, DOI:10.3168/jds.S0022-0302(03)73803-X.
- E. L. Annen, R. J. Collier, M. A. McGuire and J. L. Vicini, Effects of dry period length on milk yield and mammary epithelial cells, J. Dairy Sci., 2004, 87, E66–E76, DOI:10.3168/jds.S0022-0302(04)70062-4.
- R. J. Collier, E. L. Annen-Dawson and A. Pezeshki, Effects of continuous lactation and short dry periods on mammary function and animal health, Animal, 2012, 6, 403–414, DOI:10.1017/S1751731111002461.
- A. Kok, R. J. van Hoeij, B. J. Tolkamp, M. J. Haskell, A. T. M. van Knegsel and I. J. M. de Boer,
et al., Behavioural adaptation to a short or no dry period with associated management in dairy cows, Appl. Anim. Behav. Sci., 2017, 186, 7–15, DOI:10.1016/j.applanim.2016.10.017.
- G. M. Barrington, T. B. McFadden, M. T. Huyler and T. E. Besser, Regulation of colostrogenesis in cattle, Livest. Prod. Sci., 2001, 70, 95–104, DOI:10.1016/S0301-6226(01)00201-9.
- Y. Le Cozler, R. Guatteo, E. Le Dréan, H. Turban, F. Leboeuf and K. Pecceu,
et al., IgG1 variations in the colostrum of holstein dairy cows, Animal, 2016, 10, 230–237, DOI:10.1017/S1751731115001962.
- M. Czerniewicz, K. Kielczewska and A. Kruk, Comparison of some physicochemical properties of milk from holstein-friesian and jersey cows, 2006 cows, Polish J. Food Nutr. Sci., 2006, 15, 61 CAS.
- T. F. O'Callaghan, M. O'Donovan, J. P. Murphy, K. Sugrue, D. Mannion and W. P. McCarthy,
et al., Evolution of the bovine milk fatty acid profile – from colostrum to milk five days post parturition, Int. Dairy J., 2020, 104, 104655, DOI:10.1016/j.idairyj.2020.104655.
- J. D. Quigley and J. J. Drewry, Nutrient and immunity transfer from cow to calf pre- and postcalving, J. Dairy Sci., 1998, 81, 2779–2790, DOI:10.3168/jds.S0022-0302(98)75836-9.
- J. B. German, R. A. Gibson, R. M. Krauss, P. Nestel, B. Lamarche and W. A. van Staveren,
et al., A reappraisal of the impact of dairy foods and milk fat on cardiovascular disease risk, Eur. J. Nutr., 2009, 48, 191–203, DOI:10.1007/s00394-009-0002-5.
-
J. B. German, N. Argov-Argaman and B. J. Boyd, Milk lipids: a complex nutrient delivery system, in Nestle Nutrition Institute Workshop Series, ed. S. M. Donovan, J. B. German, B. Lönnerdal and A. Lucas, 2019, pp. 217–225 Search PubMed.
- B. A. McGrath, P. F. Fox, P. L. H. McSweeney and A. L. Kelly, Composition and properties of bovine colostrum: a review, Dairy Sci. Technol., 2016, 96, 133–158, DOI:10.1007/s13594-015-0258-x.
-
P. A. Morrissey and T. R. Hill, Fat-soluble vitamins and vitamin c in milk and milk products, in Advanced Dairy Chemistry, ed. P. McSweeney and P. F. Fox, Springer, New York, 2009, pp. 527–589 Search PubMed.
- S. K. Jensen, A. K. B. Johannsen and J. E. Hermansen, Quantitative secretion and maximal secretion capacity of retinol, β-carotene and α-tocopherol into cows' milk, J. Dairy Res., 1999, 66, 511–522, DOI:10.1017/S0022029999.003805.
- C. Debier and Y. Larondelle, Vitamins A and E: metabolism, roles and transfer to offspring, Br. J. Nutr., 2005, 93, 153–174, DOI:10.1079/BJN20041308.
- H. E. Indyk and D. C. Woollard, The endogenous vitamin K1 content of bovine milk: temporal influence of season and lactation, Food Chem., 1995, 54, 403–407, DOI:10.1016/0308-8146(95)00091-V.
- P. C. Pereira, Milk nutritional composition and its role in human health, Nutrition, 2014, 30, 619–627, DOI:10.1016/j.nut.2013.10.011.
- K. M. Henry and S. K. Kon, A note on the vitamin D content of cow's colostrum, Biochem. J., 1937, 31, 2199–2201, DOI:10.1042/bj0312199.
-
P. Marnila and H. Korhonen, Colostrum, in Encyclopedia of Dairy Sciences, ed. H. Roginski, Elsevier, 2002, pp. 473–478 Search PubMed.
- A. Haug, A. T. Høstmark and O. M. Harstad, Bovine milk in human nutrition – a review, Lipids Health Dis., 2007, 6, 25, DOI:10.1186/1476-511X-6-25.
- S. Ahmad, F. M. Anjum, N. Huma, A. Sameen and T. Zahoor, Composition and physico-chemical characteristics of buffalo milk with particular emphasis on lipids, proteins, minerals, enzymes and vitamins, J. Anim Plant Sci., 2013, 23, 62–74 Search PubMed.
- G. M. Barrington, T. E. Besser, W. C. Davis, C. C. Gay, J. J. Reeves and T. B. McFadden, Expression of immunoglobulin G1 receptors by bovine mammary epithelial cells and mammary leukocytes, J. Dairy Sci., 1997, 80, 86–93, DOI:10.3168/jds.S0022-0302(97)75915-0.
- H. Korhonen and A. Pihlanto, Technological options for the production of health-promoting proteins and peptides derived from milk and colostrum, Curr. Pharm. Des., 2007, 13, 829–843, DOI:10.2174/138161207780363112.
- M. Kramski, G. F. Lichtfuss, M. Navis, G. Isitman, L. Wren and G. Rawlin,
et al., Anti-HIV-1 antibody-dependent cellular cytotoxicity mediated by hyperimmune bovine colostrum IgG, Eur. J. Immunol., 2012, 42, 2771–2781, DOI:10.1002/eji.201242469.
- H. Korhonen, P. Marnila and H. S. Gill, Milk immunoglobulins and complement factors, Br. J. Nutr., 2000, 84, 75–80, DOI:10.1017/S0007114500002282.
- C. R. Baumrucker and R. M. Bruckmaier, Colostrogenesis: IgG1 transcytosis mechanisms, J. Mammary Gland Biol. Neoplasia, 2014, 19, 103–117, DOI:10.1007/s10911-013-9313-5.
- M. Sasaki, C. L. Davis and B. L. Larson, Production and turnover of IgG1 and IgG2 immunoglobulins in the bovine around parturition, J. Dairy Sci., 1976, 59, 2046–2055, DOI:10.3168/jds.S0022-0302(76)84486-4.
- A. M. Virtala, Y. Gröhn, G. Mechor and H. Erb, The effect of maternally derived immunoglobulin G on the risk of respiratory disease in heifers during the first 3 months of life, Prev. Vet. Med., 1999, 39, 25–37, DOI:10.1016/S0167-5877(98)00140-8.
- A. L. Beam, J. E. Lombard, C. A. Kopral, L. P. Garber, A. L. Winter and J. A. Hicks,
et al., Prevalence of failure of passive transfer of immunity in newborn heifer calves and associated management practices on US dairy operations, J. Dairy Sci., 2009, 92(8), 3973–3980, DOI:10.3168/jds.2009-2225.
- S. M. McGuirk and M. Collins, Managing the production, storage, and delivery of colostrum, Vet. Clin. N. Am. Food Anim. Pract., 2004, 20, 593–603, DOI:10.1016/j.cvfa.2004.06.005.
- B. F. Iglesias-Figueroa, E. A. Espinoza-Sánchez, T. S. Siqueiros-Cendón and Q. Rascón-Cruz, Lactoferrin as a nutraceutical protein from milk, an overview, Int. Dairy J., 2019, 89, 37–41 CrossRef CAS.
- I. A. García-Montoya, T. S. Cendón, S. Arévalo-Gallegos and Q. Rascón-Cruz, Lactoferrin a multiple bioactive protein: an overview, Biochim. Biophys. Acta Gen. Subj., 2012, 1820(3), 226–236 CrossRef PubMed.
- H. Wakabayashi, H. Oda, K. Yamauchi and F. Abe, Lactoferrin for prevention of common viral infections, J. Infect. Chemother., 2014, 20, 666–671, DOI:10.1016/j.jiac.2014.08.003.
- D. E. W. Chatterton, D. N. Nguyen, S. B. Bering and P. T. Sangild, Anti-inflammatory mechanisms of bioactive milk proteins in the intestine of newborns, Int. J. Biochem. Cell Biol., 2013, 45, 1730–1747, DOI:10.1016/j.biocel.2013.04.028.
- P. Manzoni, Clinical benefits of lactoferrin for infants and children, J. Pediatr., 2016, 173, S43–S52, DOI:10.1016/j.jpeds.2016.02.075.
- E. J. Dial, L. R. Hall, H. Serna, J. J. Romero, J. G. Fox and L. M. Lichtenberger, Antibiotic properties of bovine lactoferrin on helicobacter pylori, Dig. Dis. Sci., 1998, 43, 2750–2756, DOI:10.1023/A:1026675916421.
- K. Yamauchi, M. Tomita, T. J. Giehl and R. T. Ellison, Antibacterial activity of lactoferrin and a pepsin-derived lactoferrin peptide fragment, Infect. Immun., 1993, 61, 719–728, DOI:10.1128/IAI.61.2.719-728.1993.
- J. Lee, S. H. Kwon, H. M. Kim, S. N. Fahey, D. R. Knighton and A. Sansom, Effect of a growth protein-colostrum fraction* on bone development in juvenile rats, Biosci. Biotechnol. Biochem., 2008, 72, 1–6, DOI:10.1271/bbb.60695.
- K. Nakajima, Y. Kanno, M. Nakamura, X. D. Gao, A. Kawamura and F. Itoh,
et al., Bovine milk lactoferrin induces synthesis of the angiogenic factors VEGF and FGF2 in osteoblasts via the p44/p42 MAP kinase pathway, BioMetals, 2011, 24, 847–856, DOI:10.1007/s10534-011-9439-0.
- T. Siqueiros-Cendón, S. Arévalo-Gallegos, B. F. Iglesias-Figueroa, I. A. GarcíaMontoya, J. Salazar-Martínez and Q. Rascón-Cruz, Immunomodulatory effects of lactoferrin, Acta Pharmacol. Sin., 2014, 35, 557–566, DOI:10.1038/aps.2013.200.
- F. Superti, Lactoferrin from bovine milk: a protective companion for life, Nutrients, 2020, 12, 2562, DOI:10.3390/nu12092562.
- L. Sánchez, P. Aranda, M. D. Pérez and M. Calvo, Concentration of lactoferrin and transferrin throughout lactation in cow's colostrum and milk, Biol. Chem. Hoppe Seyler., 1988, 369, 1005–1008, DOI:10.1515/bchm3.1988.369.2.1005.
- M. Kaplan, A. Arslan, H. Duman, M. Karyelioğlu, B. Baydemir, B. B. Günar and S. Karav, Production of bovine colostrum for human consumption to improve health, Front. Pharmacol, 2022, 12, 796824 CrossRef PubMed.
- B. Wang, Y. P. Timilsena, E. Blanch and B. Adhikari, Mild thermal treatment and in-vitro digestion of three forms of bovine lactoferrin: effects on functional properties, Int. Dairy J., 2017, 64, 22–30 CrossRef CAS.
- P. F. Fox and A. L. Kelly, Indigenous enzymes in milk: overview and historical aspects—Part 1, Int. Dairy J., 2006, 16, 500–516, DOI:10.1016/j.idairyj.2005.09.013.
-
N. Y. Farkye and N. Bansal, Enzymes indigenous to milk other enzymes, in Encyclopedia of Dairy Sciences, ed. J.
W. Fuquay, P. J. Fox and P. L. H. McSweeney, Elsevier, Amsterdam, 2011, pp. 327–334 Search PubMed.
-
Shakeel-ur-Rehman and N. Y. Farkye, Enzymes indigenous to milk lactoperoxidase, in Encyclopedia of Dairy Sciences, ed. H. Roginski, Elsevier, 2002, pp. 938–941 Search PubMed.
- L. M. Wolfson and S. S. SUMNER, Antibacterial activity of the lactoperoxidase system: a review, J. Food Prot., 1993, 56, 887–892, DOI:10.4315/0362-028X-56.10.887.
- M. E. Belding, S. J. Klebanoff and C. G. Ray, Peroxidase-mediated virucidal systems, Science, 1970, 167, 195–196, DOI:10.1126/science.167.3915.195.
-
T. Tanaka, X. Xuan, K. Fujisaki and K. Shimazaki, Expression and characterization of bovine milk antimicrobial proteins lactoperoxidase and lactoferrin by vaccinia virus, in Insight and Control of Infectious Disease in Global Scenario, ed. P. K. Roy, IntechOpen, 2012, pp. 127–133 Search PubMed.
- T. Nakamura, H. Kawase, K. Kimura, Y. Watanabe, M. Ohtani and I. Arai,
et al., Concentrations of sialyloligosaccharides in bovine colostrum and milk during the prepartum and early lactation, J. Dairy Sci., 2003, 86, 1315–1320, DOI:10.3168/jds.S0022-0302(03)73715-1.
- N. Tao, E. J. DePeters, S. Freeman, J. B. German, R. Grimm and C. B. Lebrilla, Bovine milk glycome, J. Dairy Sci., 2008, 91, 3768–3778, DOI:10.3168/jds.2008-1305.
- N. Tao, E. J. DePeters, J. B. German, R. Grimm and C. B. Lebrilla, Variations in bovine milk oligosaccharides during early and middle lactation stages analyzed by high-performance liquid chromatography-chip/mass spectrometry, J. Dairy Sci., 2009, 92, 2991–3001, DOI:10.3168/jds.2008-1642.
- D. Barile, M. Marotta, C. Chu, R. Mehra, R. Grimm and C. B. Lebrilla,
et al., Neutral and acidic oligosaccharides in Holstein-Friesian colostrum during the first 3 days of lactation measured by high performance liquid chromatography on a microfluidic chip and time-of-flight mass spectrometry, J. Dairy Sci., 2010, 93, 3940–3949, DOI:10.3168/jds.2010-3156.
- M. R. Ninonuevo, Y. Park, H. Yin, J. Zhang, R. E. Ward and B. H. Clowers,
et al., A strategy for annotating the human milk glycome, J. Agric. Food Chem., 2006, 54, 7471–7480, DOI:10.1021/jf0615810.
- S. Martín-Sosa, M. J. Martín, L. A. García-Pardo and P. Hueso, Sialyloligosaccharides in human and bovine milk and in infant formulas: variations with the progression of lactation, J. Dairy Sci., 2003, 86, 52–59, DOI:10.3168/jds.S0022-0302(03)73583-8.
-
T. Urashima, M. Kitaoka, S. Asakuma and M. Messer, Milk oligosaccharides, in Advanced Dairy Chemistry, ed. P. McSweeney and P. F. Fox, Springer, New York, 2009, pp. 295–349 Search PubMed.
- P. McJarrow and J. van Amelsfort-Schoonbeek, Bovine sialyl oligosaccharides: seasonal variations in their concentrations in milk, and a comparison of the colostrums of jersey and friesian cows, Int. Dairy J., 2004, 14, 571–579, DOI:10.1016/j.idairyj.2003.11.006.
- S. Karav, J. Bell, A. Le Parc, Y. Liu, D. A. Mills and D. E. Block,
et al., Characterizing the release of bioactive N-glycans from dairy products by a novel endo-β-N-acetylglucosaminidase, Biotechnol. Prog., 2015, 31, 1331–1339, DOI:10.1002/btpr.2135.
- V. Weinborn, Y. Li, I. M. Shah, H. Yu, D. C. Dallas and J. B. German,
et al., Production of functional mimics of human milk oligosaccharides by enzymatic glycosylation of bovine milk oligosaccharides, Int. Dairy J., 2020, 102, 104583, DOI:10.1016/j.idairyj.2019.104583.
- A. M. Zivkovic and D. Barile, Bovine milk as a source of functional oligosaccharides for improving human health, Adv. Nutr., 2011, 2, 284–289, DOI:10.3945/an.111.000455.
- R. C. Robinson, E. Colet, T. Tian, N. A. Poulsen and D. Barile, An improved method for the purification of milk oligosaccharides
by graphitised carbon-solid phase extraction, Int. Dairy J., 2018, 80, 62–68, DOI:10.1016/j.idairyj.2017.12.009.
- S. T. Westreich, J. Salcedo, B. Durbin-Johnson, J. T. Smilowitz, I. Korf and D. A. Mills,
et al., Fecal metatranscriptomics and glycomics suggest that bovine milk oligosaccharides are fully utilized by healthy adults, J. Nutr. Biochem., 2020, 79, 108340, DOI:10.1016/j.jnutbio.2020.108340.
- L. M. A. Jakobsen, U. K. Sundekilde, H. J. Andersen, D. S. Nielsen and H. C. Bertram, Lactose and bovine milk oligosaccharides synergistically stimulate B, Longum subsp. Longum growth in a simplified model of the infant gut microbiome, J. Proteome Res., 2019, 18, 3086–3098, DOI:10.1021/acs.jproteome.9b00211.
- L. M. A. Jakobsen, M. X. Maldonado-Gómez, U. K. Sundekilde, H. J. Andersen, D. S. Nielsen and H. C. Bertram, Metabolic effects of bovine milk oligosaccharides on selected commensals of the infant microbiome-commensalism and postbiotic effects, Metabolites, 2020, 10, 167, DOI:10.3390/metabo10040167.
- S. Karav, A. Le Parc, J. M. Leite Nobrega de Moura Bell, S. A. Frese, N. Kirmiz and D. E. Block,
et al., Oligosaccharides released from milk glycoproteins are selective growth substrates for infant-associated bifidobacterial, Appl. Environ. Microbiol., 2016, 82, 3622–3630, DOI:10.1128/AEM.00547-16.
- A. Bunyatratchata, Y. P. Huang, G. Ozturk, J. L. Cohen, M. Bhattacharya and J. Mln De Moura Bell,
et al., Effects of industrial thermal treatments on the release of bovine colostrum glycoprotein n-glycans by endo-β-N-acetylglucosaminidase, J. Agric. Food Chem., 2020, 68, 15208–15215, DOI:10.1021/acs.jafc.0c05986.
- X. Cao, M. Yang, N. Yang, X. Liang, D. Tao and B. Liu,
et al., Characterization and comparison of whey N-glycoproteomes from human and bovine colostrum and mature milk, Food Chem., 2019, 276, 266–273, DOI:10.1016/j.foodchem.2018.09.174.
- B. M. Henrick, S. Chew, G. Casaburi, H. K. Brown, S. A. Frese and Y. Zhou,
et al., Colonization by B. Infantis EVC001 modulates enteric inflammation in exclusively breastfed infants, Pediatr. Res., 2019, 86, 749–757, DOI:10.1038/s41390-019-0533-2.
- R. M. Duar, G. Casaburi, R. D. Mitchell, L. N. C. Scofield, C. A. O. Ramirez and D. Barile,
et al., Comparative genome analysis of bifidobacterium among commercial probiotics, Nutrients, 2020, 12, 3247, DOI:10.3390/nu12113247.
- J. A. Lane, K. Mariño, J. Naughton, D. Kavanaugh, M. Clyne and S. D. Carrington,
et al., Anti-infective bovine colostrum oligosaccharides: campylobacter jejuni as a case study, Int. J. Food Microbiol., 2012, 157, 182–188, DOI:10.1016/j.ijfoodmicro.2012.04.027.
- L. Robbers, R. Jorritsma, M. Nielen and A. Koets, A scoping review of on-farm colostrum management practices for optimal transfer of immunity in dairy calves, Front. Vet. Sci., 2021, 8, 668639 CrossRef PubMed.
- J. M. Pickard, M. Y. Zeng, R. Caruso and G. Núñez, Gut microbiota: role in pathogen colonization, immune responses, and inflammatory disease, Immunol. Rev., 2017, 279(1), 70–89 CrossRef CAS PubMed.
- A. J. Phipps, D. S. Beggs, A. J. Murray, P. D. Mansell, M. A. Stevenson and M. F. Pyman, Survey of bovine colostrum quality and hygiene on northern Victorian dairy farms, J. Dairy Sci., 2016, 99(11), 8981–8990 CrossRef CAS PubMed.
- S. Sotudeh, M. Rabbani Khorasgani, Z. Etemadifar and S. H. Zarkesh-Esfahani, Effects of spray-drying, freeze-drying and pasteurization on microbiological quality and IgG level of bovine colostrum, J. Food Qual Hazards Control, 2018, 5(2), 54–60 CrossRef CAS.
- J. M. Jay and J. M. Jay, High-temperature food preservation and characteristics of thermophilic microorganisms, Modern Food Microbiol., 1995, 347–369 Search PubMed.
- R. E. James, C. E. Polan and K. A. Cummins, Influence of administered indigenous microorganisms on uptake of [iodine-125] gamma-globulin in vivo by intestinal segments of neonatal calves, J. Dairy Sci., 1981, 64, 52–61 CrossRef CAS PubMed.
- S. M. Godden, S. Smith, J. M. Feirtag, L. R. Green, S. J. Wells and J. P. Fetrow, Effect of on-farm commercial batch pasteurization of colostrum on colostrum and serum immunoglobulin concentrations in dairy calves, J. Dairy Sci., 2003, 86(4), 1503–1512 CrossRef CAS PubMed.
- J. R. Stabel, S. Hurd, L. Calvente and R. F. Rosenbusch, Destruction of Mycobacterium paratuberculosis, Salmonella spp., and Mycoplasma spp. in raw milk by a commercial on-farm high-temperature, short-time pasteurizer, J. Dairy Sci., 2004, 87(7), 2177–2183 CrossRef CAS PubMed.
- J. L. Johnson, S. M. Godden, T. Molitor, T. Ames and D. Hagman, Effects of feeding heat-treated colostrum on passive transfer of immune and nutri- tional parameters in neonatal dairy calves, J. Dairy Sci., 2007, 90, 5189–5198 CrossRef CAS PubMed.
- J. A. Elizondo-Salazar and A. J. Heinrichs, Feeding heat-treated colostrum or unheated colostrum with two different bacterial concentrations to neo- natal dairy calves, J. Dairy Sci., 2009, 92, 4565–4571 CrossRef CAS PubMed.
- S. L. Gelsinger, C. M. Jones and A. J. Heinrichs, Effect of colostrum heat treatment and bacterial population on immunoglobulin G absorption and health of neonatal calves, J. Dairy Sci., 2015, 98, 4640–4645 CrossRef CAS PubMed.
- K. Puppel, M. Golebiewski, G. Grodkowski, J. Slosarz, M. Kunowska-Slosarz, P. Solarczyk, M. Lukasiewicz, M. Balcerak and T. Przysucha, Composition and factors affecting quality of bovine colostrum: a review, Animals, 2019, 9, 1–14 CrossRef PubMed.
- H. E. Indyk, J. W. Williams and H. A. Patel, Analysis of denaturation of bovine IgG by heat and high pressure using an optical biosensor, Int. Dairy J., 2008, 18, 359–366 CrossRef CAS.
- C. Cummins, D. P. Berry, J. P. Murphy, I. Lorenz and E. Kennedy, The effect of colostrum storage conditions on dairy heifer calf serum immuno- globulin G concentration and preweaning health and growth rate, J. Dairy Sci., 2017, 100, 525–535 CrossRef CAS PubMed.
- J. R. Stabel, S. Hurd, L. Calvente and R. F. Rosenbusch, Destruction of Mycobacterium paratuberculosis, Salmonella spp., and Mycoplasma spp. in raw milk by a commercial on-farm high-temperature, short-time pasteur-izer, J. Dairy Sci., 2004, 87, 2177–2183 CrossRef CAS PubMed.
- S. G. Borad and A. K. Singh, Colostrum immunoglobulins: processing, preservation and application aspects, Int. Dairy J., 2018, 85, 201–210 CrossRef CAS.
- S. F. Hansen, S. A. Hogan, J. Tobin, J. T. Rasmussen, L. B. Larsen and L. Wiking, Microfiltration of raw milk for production of high-purity milk fat globule membrane material, J. Food Eng., 2020, 276, 109887 CrossRef CAS.
- M. Piot, J. Fauquant, M. N. Madec and J. L. Maubois, Preparation of serocolostrum by membrane microfiltration, Le Lait, 2004, 84(4), 333–341 CrossRef CAS.
- M. Meylan, D. M. Rings, W. P. Shulaw, J. J. Kowalski, S. Bech-Nielsen and G. F. Hoffsis, Survival of Mycobacterium paratuberculosis and preservation of immunoglobulin G in bovine colostrum under experimental conditions simulating pasteurization, Am. J. Vet. Res., 1996, 57(11), 1580–1585 CAS.
- E. Li-Chan, A. Kummer, J. N. Losso, D. D. Kitts and S. Nakai, Stability of bovine immunoglobulins to thermal treatment and processing, Food Res. Int., 1995, 28(1), 9–16 CrossRef CAS.
- F. Klobasa, M. C. Goel and E. Werhahn, Comparison of freezing and lyophilizing for preservation of colostrum as a source of immunoglobulins for calves, J. Anim. Sci., 1998, 76, 923–926 CrossRef CAS PubMed.
- L. Elfstrand, H. Lindmark-Mansson, M. Paulson, L. Nyberg and B. Akesson, Immunoglobulins, growth factors and growth hormone in bovine colostrum and the effects of processing, Int. Dairy J., 2002, 12, 879–887 CrossRef CAS.
- A. M. Abd El-Fattah, F. H. R. Abd Rabo, S. M. El-Dieb and H. A. Satar El-Kashef, Preservation methods of buffalo and bovine colostrum as a source of bioactive components, Int. Dairy J., 2014, 39, 24–27 CrossRef CAS.
- B. J. Chelack, P. S. Morley and D. M. Haines, Evaluation of methods for dehydration of bovine colostrum for total replacement of normal colostrum in calves, Can. Vet. J., 1993, 34, 407–412 CAS.
- E. Bartkiene, V. Lele, V. Sakiene, P. Zavistanaviciute, M. Ruzauskas, A. Stankevicius, J. Grigas, A. Pautienius, J. Bernatoniene, V. Jakstas, D. Zadeike, P. Viskelis and G. Juodeikiene, Fermented, ultrasoni- cated, and dehydrated bovine colostrum: changes in antimicrobial properties and immunoglobulin content, J. Dairy Sci., 2020, 103, 1315–1323 CrossRef CAS PubMed.
- C. Chellaram, G. Murugaboopathi, A. A. John, R. Sivakumar, S. Ganesan, S. Krithika and G. Priya, Significance of nanotechnology in food industry, APCBEE Proc., 2014, 8, 109–113 CrossRef CAS.
- P. R. Srinivas, M. Philbert, T. Q. Vu, Q. Huang, J. L. Kokini, E. Saos and S. A. Ross, Nanotechnology research: applications in nutritional sciences, J. Nutr., 2010, 140(1), 119–124 CrossRef CAS PubMed.
- P. Subramanian, Lipid-based nanocarrier system for the effective delivery of nutraceuticals, Molecules, 2021, 26(18), 5510 CrossRef CAS PubMed.
- M. Reza Mozafari, C. Johnson, S. Hatziantoniou and C. Demetzos, Nanoliposomes and their applications in food nanotechnology, J. Liposome Res., 2008, 18(4), 309–327 CrossRef CAS PubMed.
- A. Ayar, H. Sıçramaz and I. Çetin, The effect of bovine colostrum on the lactic flora of yogurt and kefir, JSM Biotechnol. Bioeng., 2016, 3, 1062–1067 Search PubMed.
- S. Stewart, S. Godden, R. Bey, P. Rapnicki, J. Fetrow, R. Farnsworth and C. Ferrouillet, Preventing bacterial contamination and proliferation during the harvest, storage, and feeding of fresh bovine colostrum, J. Dairy Sci., 2005, 88(7), 2571–2578 CrossRef CAS PubMed.
- Y. Kotsis, A. Mikellidi, C. Aresti, E. Persia, A. Sotiropoulos, D. B. Panagiotakos and T. Nomikos, A low-dose, 6-week bovine colostrum supplementation maintains performance and attenuates inflammatory indices following a loughborough intermittent shuttle test in soccer players, Eur. J. Nutr., 2018, 57, 1181–1195 CrossRef CAS PubMed.
- A. W. Jones, D. S. March, R. Thatcher, B. Diment, N. P. Walsh and G. Davison, The effects of bovine colostrum supplementation on in vivo immunity following prolonged exercise: a randomised controlled trial, Eur. J. Nutr., 2019, 58, 335–344 CrossRef CAS PubMed.
- C. H. Florén, S. Chinenye, L. Elfstrand, C. Hagman and I. Ihse, ColoPlus, a new product based on bovine colostrum, alleviates HIV-associated diarrhoea, Scand. J. Gastroenterol., 2006, 41(6), 682–686 CrossRef PubMed.
- K. Patel and R. Rana, Pedimune in recurrent respiratory infection and diarrhoea—the Indian experience—the pride study, Ind. J. Pediatr., 2006, 73, 585–591 CrossRef PubMed.
- S. Q. Li, H. Q. hang, V. M. Balasubramaniam, Y. Z. Lee, J. A. Bomser, S. J. Schwartz and C. P. Dunne, Comparison of effects of high-pressure processing and heat treatment on immunoactivity of bovine milk immunoglobulin G in enriched soymilk under equivalent microbial inactivation levels, J. Agric. Food Chem., 2006, 54, 739–746 CrossRef CAS PubMed.
- M. Permanyer, C. Castellote, C. Ramírez-Santana, C. Audí, F. J. Pérez-Cano, M. Castell, M. C. López-Sabater and A. Franch, Maintenance of breast milk immunoglobulin A after high-pressure processing, J. Dairy Sci., 2010, 93, 877–883 CrossRef CAS PubMed.
- T. Masuda, H. Y. Rehinarudo, K. Suzuki, T. Sakai and T. Morichi, The effect of high hydrostatic pressure treatment on the preservability and the immunological activity of bovine colostrum, Asian-Australas. J. Anim. Sci., 2000, 13, 1323–1328 CrossRef.
- D. M. Foster, K. P. Poulsen, H. J. Sylvester, M. E. Jacob, K. E. Casulli and B. E. Farkas, Effect of high-pressure processing of bovine colostrum on immuno- globulin G concentration, pathogens, viscosity, and transfer of passive immunity to calves, J. Dairy Sci., 2016, 99, 8575–8588 CrossRef CAS PubMed.
- A. S. Cross, H. J. Karreman, L. Zhang, Z. Rosenberg, S. M. Opal and A. Lees, Immunization of cows with novel core glycolipid vaccine induces anti-endotoxin antibodies in bovine colostrum, Vaccine, 2014, 32(46), 6107–6114 CrossRef CAS PubMed.
- E. Eilers, T. Ziska, T. Harder, A. Plagemann, M. Obladen and A. Loui, Leptin determination in colostrum and early human milk from mothers of preterm and term infants, Early Hum. Dev., 2011, 87(6), 415–419 CrossRef CAS PubMed.
- H. Kumar, S. Kumari, N. Kumar and S. Ranvir, Colostrum as a nutraceutical, Energy, 2021, 58, 130 Search PubMed.
- R. Granata, F. Broglio, D. Migliorino, S. Cutrupi, G. Baldanzi, M. Sireno and A. Pucci, Neonatal and adult human heart tissues from normal subjects and patients with ischemic, dilated or hypertrophic cardiomyopathy express insulin-like growth factor binding protein-3 (IGFBP-3), J. Endocrinol. Investig., 2000, 23, 724–726 CrossRef CAS PubMed.
- H. Kuipers, E. Van Breda, G. Verlaan and R. Smeets, Effects of oral bovine colostrum supplementation on serum insulin-like growth factor-I levels, Nutrition, 2002, 18(7–8), 566–567 CrossRef CAS PubMed.
- M. Musumeci, J. Simporè, A. D'Agata, L. Malaguarnera, C. Carrozza, C. Zuppi and S. Musumeci, Biologic substances present in human colostrums demonstrate the evolution of this essential nutrient for growth and development: insulin-like growth factor-I and prolactin, Nutr. Res., 2005, 25(2), 133–142 CrossRef CAS.
- F. Palm, C. Nagel, R. M. Bruckmaier, J. E. Aurich and C. Aurich, Clinical parameters, intestinal function, and IGF1 concentrations in colostrum-deprived and colostrum-fed newborn pony foals, Theriogenology, 2013, 80(9), 1045–1051 CrossRef CAS PubMed.
- C. M. Shing, J. M. Peake, K. Suzuki, D. G. Jenkins and J. S. Coombes, Bovine colostrum modulates cytokine production in human peripheral blood mononuclear cells stimulated with lipopolysaccharide and phytohemagglutinin, J. Interferon Cytokine Res., 2009, 29(1), 37–44 CrossRef CAS PubMed.
- A. W. Jones, S. J. Cameron, R. Thatcher, M. S. Beecroft, L. A. Mur and G. Davison, Effects of bovine colostrum supplementation on upper respiratory illness in active males, Brain Behav. Immun., 2014, 39, 194–203 CrossRef CAS PubMed.
- F. He, E. Tuomola, H. Arvilommi and S. Salminen, Modulation of human humoral immune response through orally administered bovine colostrum, FEMS Immunol. Med. Microbiol., 2001, 31(2), 93–96 CrossRef CAS PubMed.
- M. X. Maldonado-Gómez, I. Martínez and F. Bottacini,
et al., Stable engraftment of Bifidobacterium longum AH1206 in the human gut depends on individualized features of the resident microbiome, Cell Host Microbe, 2016, 20(4), 515–526 CrossRef PubMed.
- N. R. Pedersen, ERRATUM: pedersen NR et al.: immunomodulatory capacity of bovine colostrum, Pteridines, 2011, 22(1), 60 CrossRef.
- O. P. Mehta, P. Bhandari, A. Raut, S. E. O. Kacimi and N. T. Huy, Coronavirus disease (COVID-19): comprehensive review of clinical presentation, Front. Public Health, 2021, 8, 582932 CrossRef PubMed.
- H. Duman and S. Karav, Bovine colostrum and its potential contributions for treatment and prevention of COVID-19, Front. Immunol., 2023, 14, 1–17 Search PubMed.
- K. Kangro, M. Kurašin, K. Gildemann, E. Sankovski, E. Žusinaite, L. S. Lello and M. Ustav, Bovine colostrum-derived antibodies against SARS-CoV-2 show great potential to serve as prophylactic agents, PLoS One, 2022, 17(6), e0268806 CrossRef CAS PubMed.
- A. Batista da Silva Galdino, A. H. do Nascimento Rangel, H. S. Buttar, M. Sales Lima Nascimento, E. Cristina Gavioli, R. D. P. Oliveira and K. Anaya, Bovine colostrum: benefits for the human respiratory system and potential contributions for clinical management of COVID-19, Food Agric. Immunol., 2021, 32(1), 143–162 CrossRef CAS.
|
This journal is © The Royal Society of Chemistry 2024 |