Mycoprotein: production and nutritional aspects: a review
Received
21st September 2023
, Accepted 17th November 2023
First published on 17th November 2023
Abstract
To feed the increasing world population, finding sustainable sources of meat substitutes has become necessary. Mycoprotein, derived from filamentous fungi, is a good meat alternative as it provides nutrition and has some additional health benefits over conventional meat. It can act as a prebiotic, antioxidant, blood cholesterol level regulator, and blood glucose level regulator. It also plays a significant role in muscle protein development. Mycoprotein production is carried out by submerged fermentation, solid-state fermentation, or surface culture method. The yield of the mycoprotein depends on the type of microorganism or substrate used for the production. Different research studies have proved that mycoprotein produced by submerged fermentation has a higher yield and more nutritional benefits. The Food and Drug Administration gave certain fungal species the Generally Recognized as Safe (GRAS) status. Some of the species include Monascus purpureus, Aspergillus oryzae, Paradendryphiella salina, Neurospora intermedia, Rhizopus oryzae and Fusarium venenatum. This fungal product has less environmental impact than conventional meat protein. The carbon footprint of mycoprotein is 10 and 4 times less than that of beef and chicken, respectively. Although some limitations are there, i.e., less protein, nausea, vomiting, sub-chronic toxicity, and allergic reactions, mycoprotein may be widely accepted by the vegetarian population in the future as a meat replacer.
Sustainability spotlight
Mycoprotein is one of the most suitable meat protein substitutes available. This review concisely covers all the aspects of mycoprotein. This review comprises the history, various processes of production, nutritional aspects and health benefits of mycoprotein. Finally, this paper presents the environmental impact of mycoprotein over the conventional meat protein. This review will help future researchers, microbiologists and industrialists to better understand mycoprotein and assist research on the limitations and applications of mycoprotein. This new type of protein can be an affordable and convenient alternative to meat protein. This review aligns with the UN's Sustainable Development Goals [Goal 2: End hunger, achieve food security and improved nutrition and promote sustainable agriculture].
|
1. Introduction
Ensuring the world's expanding population has access to sufficient, sustainable, and nutrient-rich food is one of our biggest challenges. By 2050, given the annual growth rate of 0.8%, the world's population will be close to 9.8 billion.1 This data suggests that protein consumption will be drastically increased, which may lead to the prevalence of malnutrition across the globe. To counteract this situation, protein production must be boosted; however, the difficulty is to increase the production sustainably. Therefore, looking for suitable alternatives to meat proteins which involve lower cost and require fewer raw materials is essential.2 Emergent foods, including insects, algae, and microbes, are highly demanded as sustainable and alternative protein sources. The meat industry won't be able to meet this increase in demand by using more resources because of the ecological significance of cattle and issues with animal welfare. Demand for economical and ecologically sustainable protein in the food system is increasing significantly.3 The growing Food-Based Dietary Guidelines (FBDG) are gradually including more plant-based dietary proteins. However, other popular dietary proteins, such as those derived from fungi, appear to have gained less study. Mycoproteins derived from fungi are gaining popularity due to their excellent nutritional value, low production costs, ecological advantages, and tolerance to environmental challenges, including drought and flooding.4
Fusarium venenatum ATCC 2684 was developed in 1960 and is the prolific strain used to cultivate and harvest mycoprotein. The sale of mycoprotein as a source of food protein was authorized by the Ministry of Agriculture, Fisheries and Food (MAFF) in the United Kingdom in 1984, and it is presently available for purchase in all EU member states.5 After that, further regulatory permits were granted in the US, Norway, Australia, Switzerland, and, more recently, Canada, Thailand, Japan, and Malaysia. The Quorn™ brand of vegan and vegetarian food makes extensive use of mycoprotein. Currently, fermentation is used to produce mycoprotein on a large scale, producing a high-quality protein with little environmental impact.6 Misconceptions concerning fungal-based proteins must be clarified because there is a rising demand for nutrient-dense and sustainable new protein sources. It will be a valuable alternative for the vegetarian community and open a new gateway for food scientists, researchers, and even the industries in the meat business. This review discusses the development of mycoprotein, its manufacturing, crucial nutritional components, health aspects, ecological effects, safety issues, and upcoming difficulties.
2. History of mycoprotein
The timeline of commercialization of fungal protein is shown in Fig. 1. Practitioners and officials worldwide were concerned in the 1960s that the projected population expansion may cause future global protein shortages. Food researchers tried to create a palatable, affordable source of microbial protein. Fusarium venenatum, a fungus found in a garden in Marlow, Buckinghamshire in 1967, was eventually used to create fungal protein.7 Mycoprotein is the nucleic acid-depleted biomass that increases the mycelium (cells) of F. venenatum in a continuous fermenter like chemostat or turbidostat. Researchers have devoted much time to determining if F. venenatum-derived mycoprotein is safe for consumption. It became clear that mycoprotein could be consumed without harming human or murine models when the nucleic acid content of the cells was reduced to safe levels.8 The ATCC PTA-2684 strain was considered safe as it could not produce toxic microbial metabolites because the growth conditions (28 to 30 °C; pH – 6.0) used for manufacture were inefficient. F. venenatum A3/5 was approved for trade as food in 1984 by the Ministry of Agriculture, Fisheries and Food in the United Kingdom.8 A joint venture of two companies in the United Kingdom, namely RHM (Rank Hovis McDougall) and ICI, was solely responsible for the commercialization of mycoprotein.
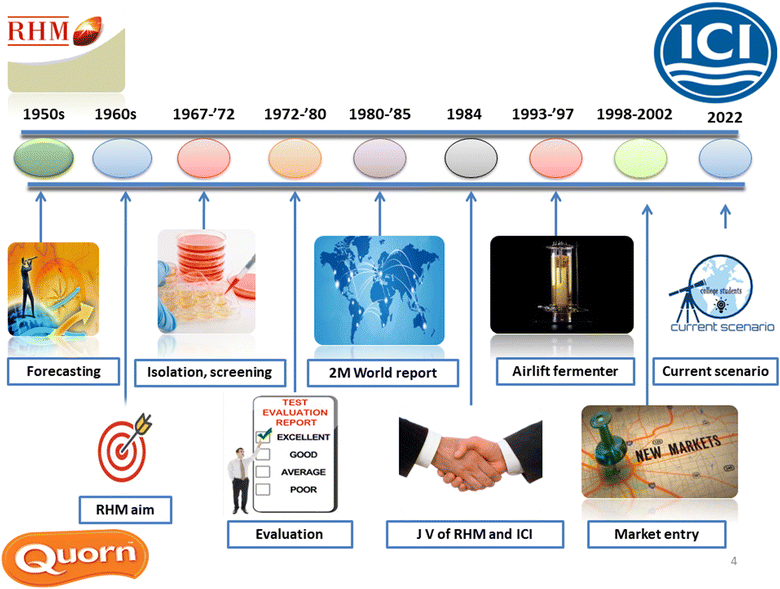 |
| Fig. 1 Historical perspective of the development and commercialization of mycoprotein. | |
3. Methods of production
Mycoprotein is produced by fermenting agro-industrial waste through microbial fermentation processes. Agro-industrial wastes like seaweed waste,9 date waste,10 soy waste,11 pineapple peel waste,12 and pea process byproducts12 can be used to produce mycoprotein biomass. There are two fermentation processes for the mass production of mycoprotein: solid-state fermentation (SSF) and submerged fermentation (SmF); another method known as the surface culture method can produce mycoprotein on a laboratory scale. Research studies aimed to develop mycoprotein employing different fermentation strategies are listed in Table 1.
Table 1 Production of fungal protein biomass by different fermentation strategies
Cultivation strategies |
Substrate |
Microorganisms |
Yield |
Composition |
References |
Submerged fermentation |
Ulva sp. |
Paradendryphiella salina
|
561.3 g kg−1 |
Protein: 48%; fibre: 3%; carbohydrate: 20% |
9
|
Submerged fermentation |
Apple; spinach; beet |
Pleurotus sp. |
250 g kg−1 |
Protein: 21%; fibre: 4%; carbohydrate: 74% |
28
|
Solid state fermentation |
Brewer spent grain |
Pleurotus albidus
|
125 g kg−1 |
Protein: 23%; fibre: 34% |
14
|
Surface culture methods |
Date juice |
F. venenatum
|
5.46 g L−1 |
— |
29
|
Submerged fermentation |
Sea weed and sea weed waste |
Paradendryphiella salina
|
564 g kg−1 |
Protein: 48%; fat: 2%; carbohydrate: 2%; fiber: 3% |
54
|
Solid state fermentation |
Stale bread and brewers' spent grains |
Neurospora intermedia and Rhizopus oryzae |
125 g kg−1 |
Protein: 46.7%; fat: 4.4%; carbohydrate: 42% |
30
|
3.1. Solid-state fermentation (SSF)
SSF is gaining popularity in the food and pharmaceutical industry because of the fermentation procedure conducted on insoluble substrates with minimal presence of liquid medium. The resulting mycoprotein has a higher PDCAAS, i.e., protein digestibility-corrected amino acid score than chicken and beef proteins, making up for the deficiency of essential amino acids in plant protein sources.13 Stoffel et al.14 developed edible mycoproteins by SSF using moisture-rich brewers' spent grains and grape waste on a potato dextrose agar (PDA) medium containing common salt solution (pH 6.5), followed by incubation (at 28 °C for 15 days). Pleurotus albidus cultivation generated more mycelial biomass (250 g/2 kg) than Agaricus blazei (212 g/2 kg) and Auricularia fuscosuccinea (56 g/2 kg) biomasses, respectively. Furthermore, as compared to the control and other strains, Pleurotus albidus biomass had greater protein content (23% w/w), total amino acids (8% w/w), and dietary fibers (34% w/w).6
The SSF method for mycoprotein synthesis consists of many successive phases, each contributing to the final protein-rich output (Fig. 2). Preparing the solid substrate requires the precise amalgamation of various raw materials.15 This concoction serves the dual function of creating an environment rich in nutrients suitable for fungal growth while preserving the substrate's structural integrity. Elements such as wheat, barley, oats, and other cereal grains are commonly used in this substrate formulation. Following that, the chosen fungus strain, usually of the Fusarium genus, is injected into the substrate. Inoculation plays a significant role in the development of fungal spores or mycelium. Subsequent incubation is carried out in a regulated environment in a bioreactor.16 Critical parameters such as temperature, humidity, and aeration are carefully managed throughout this phase to optimize fungal growth and subsequent protein synthesis. As the fungal biomass grows, it takes advantage of the available nutrients in the substrate, triggering a sequence of metabolic processes. This metabolic process for producing fungal mycelium produces mycelial biomass and mycoprotein. The synthesized mycoprotein is notable for its high concentration of critical amino acids and protein components.
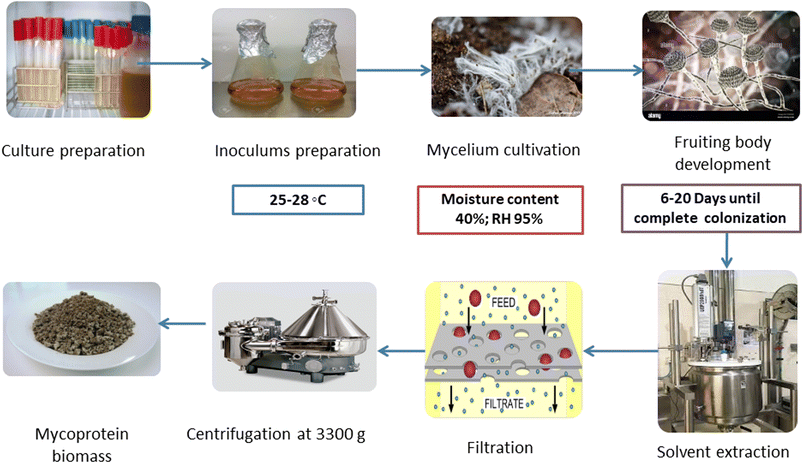 |
| Fig. 2 Preparation of mycoprotein by solid state fermentation. | |
The fungal biomass, primarily mycoprotein, is extracted from the solid substrate at an opportune juncture, specified by the end of the incubation time. This extraction method requires the careful separation of mycoprotein from both the solid substrate and any remaining fungus spores.15 For this, after subsequent cultivation of fungal mycelium, it is dissolved in an organic solvent like hexane. Then, filtration takes place, followed by subsequent centrifugation to obtain pure mycoprotein biomass. The extracted mycoprotein goes through several processing procedures to improve its sensory properties, nutritional profile, and overall quality. Texturization, flavor improvement, and shaping are the processing methods used to mimic various meat-based products. Finally, the processed mycoprotein becomes a flexible component appropriate for incorporating into various culinary items, including meat replacements.17
3.2. Submerged fermentation (SmF)
Submerged fermentation (SmF) is the process by which microorganisms grow (anaerobic/partially anaerobic) and decompose substrates (i.e., carbohydrates) in the presence of lots of free water (liquid medium).19 Despite the popularity of SmF in bioprocessing, SSF is becoming the center of attention because of higher productivity, less water consumption, and less contamination.18 Submerged microbe cultivation is growing microorganisms in a liquid medium containing carbon, nitrogen, and micronutrients. Submerged cultivation has surpassed fruiting body cultivation as a technique of producing bioactive mushroom compounds in the business due to the advantages of easy and quick management of growing conditions. Furthermore, air-lift bioreactors might be employed in submerged culture, allowing for further industrial applications.19,20
This process (Fig. 3) is initiated by cultivating a pure culture of the designated fungal strain, typically Fusarium species, in a liquid growth medium to generate an inoculum. The ensuing step involves the formulation of a nutrient-rich fermentation medium replete with carbon and nitrogen sources, vitamins, minerals, and other essential growth constituents.21 Subsequently, the prepared inoculum is introduced into the fermentation medium, serving as the source of fungal biomass and protein synthesis. The ensuing fermentation stage, executed within controlled bioreactors or fermentation vessels, demands precise regulation of environmental parameters encompassing temperature, pH, oxygen availability, and agitation.22 These conditions are meticulously modulated to optimize fungal proliferation and mycoprotein production. During the metabolic progression, the fungal cells actively metabolize the nutrients in the submerged medium, ultimately culminating in the biosynthesis of biomass enriched with mycoprotein. This proteinaceous entity is characterized by a notable content of essential amino acids, rendering it nutritionally significant.23 Notably, the submerged fermentation process is subjected to rigorous monitoring to gauge parameters such as biomass concentration, mycoprotein yield, pH stability, and oxygen sufficiency. Upon reaching the desired level of mycoprotein accumulation, the fermentation is terminated, and the fungal biomass is isolated from the liquid medium through methods such as centrifugation or filtration. The harvested biomass is subjected to additional processing steps to enhance its textural and sensory attributes.24 The resulting mycoprotein is then harnessed as a versatile ingredient in diverse food applications, effectively substituting conventional meat sources. This comprehensive process underscores the potential of submerged fermentation as a proficient route for mycoprotein production, albeit with considerations of energy demand due to the requisite liquid medium and agitation.25
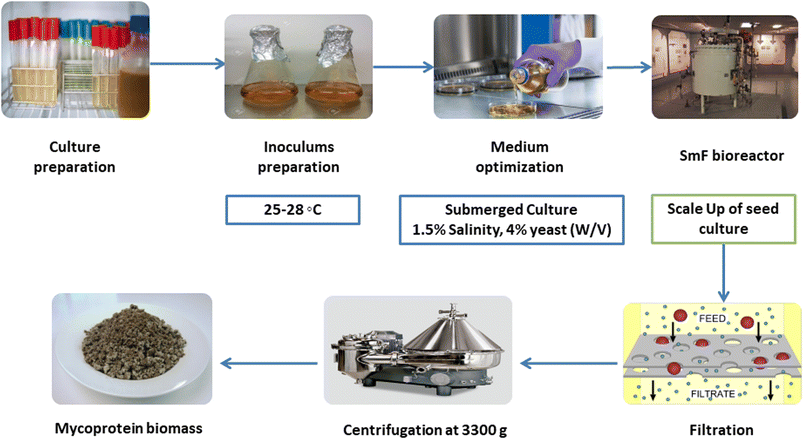 |
| Fig. 3 Preparation of mycoprotein by submerged fermentation. | |
The Quorn (submerged) fermenters, the largest continuous flow culture systems in biotechnology, use air-lift technology, distinct from conventional stirred-tank bioreactors. This design benefits mycelial production with low shear, fostering lengthy hyphal development vital for replicating meat-like textures. Air-lift fermenters require less energy than stirred tanks, efficiently mixing with a smaller energy footprint. These fermenters introduce sterile air bubbles to achieve oxygen saturation and medium mixing, contrasting mechanical impellers. Oxygen transfer primarily occurs near the riser's base due to hydrostatic pressure and pumped air turbulence, optimizing gaseous-liquid oxygen transfer. Air and culture coalesce in the riser, forming a milieu where air comprises up to 50% of the volume, gradually decreasing as the riser ascends. The upper riser's low pressure releases unused gases. Oxygen-depleted culture reoxygenates in the downcomer to prevent cell death. The cycle concludes as the reoxygenated culture re-enters the riser.19
3.3. Surface culture method (SCM)
Like the SSF and SmF, mycoprotein can also be cultivated using the SCM. However, establishing this system on a bigger scale is expensive and not economical, like SSF and SmF. In a study by Prakash et al.,26 they made Fusarium venenatum grow well, producing 4.9 g of biomass per liter in just 3 days. Optimal mycoprotein production requires 20 g L−1 date juice, 4.48 g L−1 nitrogen, and 12.97% seed size, yielding 46 to 48% protein in dried cells. Heat treatment at 65 °C for 30 min reduces single-stranded nucleic acid levels for safe food-grade use.26 This method involves cultivating fungal strains on solid substrates like pea processing byproducts (PpB).27 Controlled environmental conditions support fungal growth and mycoprotein production. In this process (Fig. 4), inoculation is carried out by the spreading method, and fungal biomass is grown on the surface of the matrix. After that, filtration takes place, followed by centrifugation to obtain mycoprotein biomass.
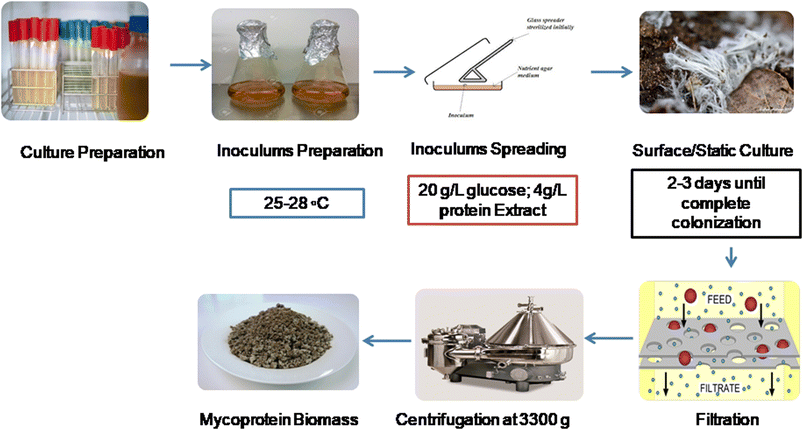 |
| Fig. 4 Preparation of mycoprotein by the surface culture technique. | |
4. Nutritional status
Due to its relatively high protein value, increased fiber and reduced saturated fatty acid content, mycoprotein is essential for a healthy diet. Mycoprotein may provide several nutritional advantages, including improving satiety and controlling blood sugar and cholesterol levels, according to experimental studies.6 Mycoprotein must be of high protein quality if it is to be used in foods that are the main course of a meal. All of the necessary amino acids are present in mycoprotein. The ideal protein bioavailability-assessed amino acid level is 0.996, indicating that the protein was produced using the best ileostomy techniques and is of high quality.31 Mycoprotein contains 6 g of fiber per 100 g, which implies that it is “rich in fiber,” according to European Commission requirements.32 The natural dietary fiber of mycoprotein comprises 12% soluble and 88% insoluble fibres, with a minor amount of chitin and predominant glucan (creating a “fibrous chitin–glucan matrix” in the small intestine region). Both linear and branched α-glucans from grains and yeast have been identified to actively participate in physiological processes related to fat metabolization and boost the immune system.32 Mycoprotein's energy makeup is about one-third fat since it contains little readily available carbohydrates. Majorly, mycoprotein contains less than 1.5 g of saturated long and short chain fatty acid per 100 g of solid. This fungal protein is rich in mono- and polyunsaturated fatty acids.33 Mycoprotein contains several water-soluble B vitamins, including pyridoxine (0.1 mg), folate (114 μg), and cobalamin (0.72 μg).34 According to nutrient claims made by the European Commission, fungal protein is a potent source of various mineral components, including zinc, phosphorus, calcium, iron, potassium, and many more.34
5. Safety assessment
As approved by the US Food and Drug Administration in 2001, mycoprotein is considered safe (GRAS) for food consumption, excluding poultry and meat products. Despite mycoprotein being a potential allergen, the company named Quorn® (largest producer), Marlow Foods, UK is selling fungi-based meat alternatives operating in 20 different countries.35 Mycoprotein foods aren't advised for children under three because of the high energy demands for quickly growing newborns, relatively low energy value, and increased fiber content.7,32
6. Health benefits of mycoprotein intake
Intake of mycoprotein increases due to its healthy nutritional profile;29 for example, consumption of mycoprotein helps to reduce the blood glucose level and modifies the secretion of gastric juices and motility of intestinal cells.36 Different studies prove that the consumption and intake of mycoprotein help regulate the blood cholesterol level. As a result, it decreases the chance of coronary and cardiovascular diseases,37 enhances the growth of good muscles, and reduces individuals' energy intake.38 All the health benefits are summarized in Fig. 5.
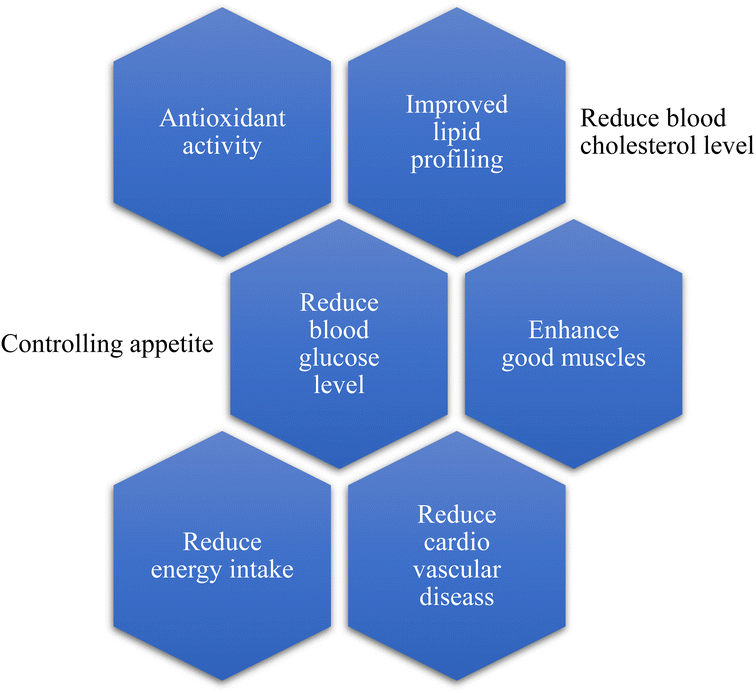 |
| Fig. 5 Health benefits of mycoprotein consumption. | |
6.1. Resistance response to glucose and insulin
The effects of mycoprotein ingestion on postprandial (after lunch or dinner period) glucose and insulin responses in healthy, lean, overweight, and obese individuals have been studied in a few clinical trials.38,39 The results of three distinct mycoprotein meal doses (44, 88, and 132 g) using matching chicken meals, blood insulin, and glucose levels were measured at various periods in overweight and obese people. Mycoprotein meals (44, 88, and 132 g) were found to have a significantly lower blood insulin incremental area under the curve (iAUC) (the area under the curve is produced from the oral glucose tolerance test, which is frequently used to diagnose impaired glucose tolerance).6 The incremental area under the curve is developed because of variations in baseline blood glucose. It essentially provides information on the glycemic index of foods, which indicates how much blood glucose is raised (after consuming any dish) at the first 60 min after consumption compared to matched-chicken meals, indicating that mycoprotein decreased the insulinogenic index (the ratio of insulin concentration at 30 min minus fasting insulin to the difference of glucose simultaneously) and increased peripheral sensitivity to insulin. However, consumption of chicken meals or mycoprotein did not cause appreciable variations in blood glucose levels at any dose. Due to the inherent properties of mycoprotein (such as protein, fiber, and hyphae composition), the process at play may result from the conversion of chitin to chitosan by alkaline deacetylation, which may cause a delay in gastric emptying. A recent double-blind, randomized, crossover trial was conducted by Coelho et al.40 to assess the participants' blood sugar levels, postprandial uric acid concentrations, and insulin sensitivity following consumption of diets containing either low nucleotide condition (L-NC) or high nucleotide condition (H-NC) mycoprotein diets (8.83%). Blood glucose and insulin concentrations increased after 30 minutes of L-NC or H-NC mycoprotein meal consumption, but not at the baseline.
6.2. Improvement in lipid profiling
It has been shown that mycoprotein-rich diets lower lipid biomarker levels in obese and overweight individuals.38,39 Animal proteins are a necessary component of controlled diets and a macronutrient-balanced diet. A diet rich in mycoproteins is for people in good health. Following the intervention, plasma levels decreased statistically significantly. Very low-density lipoprotein (VLDL) (8.36%) and intermediate-density lipoprotein (14.47%) were both lower in the mycoprotein group than in the control group. Additionally, there was a significant decrease in omega-3 fatty acids (17.26%) and docosahexaenoic acid (17.53%) compared to typical diets. The fasting blood glucose, serum interleukin-6 (anti-inflammatory), and uric acid concentrations did not significantly change throughout the trial despite the inclusion of the mycoprotein as well as fish or animal protein meals. Using the Triton X-100-induced hyperlipidemic (increased levels of lipids in the blood) rat model, Thomas et al.41 reported the anti-hyperlipidemic and anti-microbial activities of the mycoprotein. They discovered that intake of a mycoprotein diet significantly decreased the plasma levels of total cholesterol (TC) (44%), triglycerides (38%), LDL (33%), and VLDL (24%) compared with the control (Triton X-100). In a previous study, Ruxton and McMillan (2010) examined the effects of a mycoprotein diet (88 g) on total cholesterol (TC) in healthy, independent adults (aged 17 to 58). They discovered that after six weeks of dietary intervention, the level of TC was significantly lower than for the control group (from 5.28 to 3.24 mmol L−1 to 4.70 to 4.38 mmol L−1). Additionally, a decrease in TC was seen in the mycoprotein diet group (14–19%) compared to the control group (3–11%) in those who had higher baseline levels, indicating that including mycoprotein in daily diets may be helpful for the management of blood cholesterol levels in obese and overweight subjects with type 2 diabetes mellitus (T2DM) and non-alcoholic fatty liver disease (metabolic dysfunction associated fatty liver diseases).42
6.3. Synthesis of muscle proteins
Compared to plant-based protein sources, animal-based protein consumption is linked to a higher rate of muscle protein synthesis.43 The anabolic potential of soy and wheat protein sources, which are relatively low in essential amino acids like lysine, has drawn much attention.44 According to Finnigan et al.,33 mycoprotein is a distinctive fungus protein with a high PDCAAS (protein digestibility corrected amino acid score) value, a large number of EAAs (45% of total protein), and a comparatively high amount of leucine (approximately 6% of total protein). Recent research has demonstrated that mycoprotein ingestion significantly increases the postprandial bioavailability of branched-chain amino acids (BCAAs) and muscle protein synthesis in young men.39
6.4. Calorie consumption and appetite control
In many nations, people regularly take mycoprotein as part of their diets since it is a high source of dietary fiber (2/3 beta-glucan and 1/3 chitin).33,45 According to Cherta-Murillo et al.,36 consumption of mycoprotein has been shown to affect total energy expenditure. It may positively affect appetite control, particularly by regulating metabolic hormones like ghrelin (hunger hormone), glucagon-like peptide-1 (GLP-1) (potentiator of insulin secretion in the beta cell), leptin (regulation of adipose tissue mass), and peptide tyrosine (PYY) (inhibits gastric motility and increases water and electrolyte absorption in the colon). In a study conducted by Bottin et al.,38 healthy and obese adults were given low (21 g), medium (27 g), or high (32 g) isocaloric (same calorie) meals to see how they affected their energy intake. They discovered that the high mycoprotein meals significantly reduced their energy intake (616 kcal) in the 32 g group compared to the control chicken meal (676 kcal).
6.5. Antioxidant activity
Mycoprotein also has good antioxidant activity and free radical scavenging activity as mycoproteins produced by various microorganisms contain different metabolites such as flavonoids, hexadecane, and phenolic compounds.12
7. Environmental impacts of mycoprotein
The estimated global warming potential of mycoprotein is 5.55–6.15 kg CO2 eq. per kg of fungal product.27 Comparatively, the global warming potential of chicken and pork is 2–4 and 4–6 kg CO2 eq. per kg of meat, respectively. Mycoprotein performed poorly compared to other proteins regarding the environmental impact when considering calorific energy value and the amount of digested proteins. The energy, land, and water utilized in the manufacturing of meat substitutes have been reported by Smetana et al.46 When comparing the amount of land (2 m2 a kg−1 with 5–7 m2 a for chicken and 7–8 m2 a for pig) and water (500 L kg−1), mycoprotein ranks among the most efficient options. Mycoprotein was as energy-efficient as dairy substitutes (15–20 kW h kg−1), although it was less so than vegetables and insects (less than 10 kW h kg−1 and 5–15 kW h kg−1, respectively). It is more effective and environmentally friendly than animal protein, it uses less water and land resources, and the carbon footprint is 10 times and 4 times lower for products than beef and chicken, respectively. Beef and chicken have water footprints that are 20 times and 6 times larger than that of mycoproteins, respectively. Since 2012, The Carbon Trust has certified that mycoprotein's carbon footprint is lower than that of other sources of proteins.47
8. Limitations
Although the consumption of mycoprotein is of immense importance, it has some drawbacks too. Mycoprotein's protein content is lower than that of other animal sources. The entire production cost is higher than the cost of conventional meat production. It also requires advanced technologies. It can cause different adverse effects, including nausea, vomiting, diarrhea, and hives. Although it has GRAS status, it can be toxic (subacute or chronic) (as it is a microscopic fungal source) and also contains allergenic substances.
9. Future prospects
Compared to animal proteins, mycoprotein uses limited resources, including water, and emits fewer greenhouse emissions like carbon dioxide and nitrogen dioxide than commercial animal protein manufacturing.48 Foods made from plants are less hazardous to the environment. As a result, even though certain concepts are not consistently mirrored across all countries, they are becoming increasingly popular and progressively being incorporated into the FBDG.49 Vegan diets have been associated with reduced total cholesterol levels than meat, fish, and vegetarian diets, potentially due to modifications to diets.50 There are still some misconceptions, however, with some nutritionists mistaking fungal protein for “plant-based” protein, which is inaccurate as this is mainly obtained from fungal sources.51 Fungal biotechnological approaches can also transform potential organic substances into nutrient-dense food proteins, which could aid in resolving some of the most critical problems facing the world today.52 The need to further study how we may use biodiversity as a source of sustainable and healthful protein has never been greater in history, despite the fact that humans have long used biodiversity. The relevance of protein diversity in contemporary diets is highlighted by the rapid emergence of novel protein components, mainly from plants and fungi, despite the long-standing dominance of wheat and soy proteins in the protein trade.53 Given these factors, it is time to produce dietary protein from fungus, which contains mycelium mycoprotein and has been shown to affect both consumers' health and the environment positively.45
10. Conclusions
Replacing meat with meat substitutes might positively affect both societal and personal arenas. A unique alternative protein source called mycoprotein has a high level of commercial acceptance. Eating it might be great for people who don't eat meat. Due to its low fat, calories, fibres, and protein levels, it's a fantastic resource of dietary peptides and proteins. Numerous studies have shown that ingesting mycoprotein has various positive health effects, such as maintaining muscle, lowering low-density lipoprotein cholesterol, and controlling blood glucose and insulin levels. As the cost of protein source is high, it limits commercial use in developing economies, and the raw resources needed to produce it have a more significant ecological impact than other plant-based substitutes. To address these issues, innovative production techniques must be given top priority, including submerged and solid-state fermentation and the use of agricultural and food waste as a substrate for its production.
Conflicts of interest
There are no conflicts to declare.
Acknowledgements
This review received no specific grant from funding agencies in the public, commercial, or not-for-profit sectors. Authors acknowledge the Director, ICAR-National Dairy Research Institute, Karnal; Director, National Institute of Food Technology Entrepreneurship and Management, Sonipat; and Director, Indian Institute of Technology Kharagpur, for their support during the literature collection and review writing.
References
-
S. R. Nadathur, J. P. D. Wanasundara and L. Scanlin, Feeding the globe nutritious food in 2050: obligations and ethical choices, in Sustainable Protein Sources, Elsevier Inc., Academic Press, 2017, pp. 409–422, DOI:10.1016/B978-0-12-802778-3.00025-1.
- H. Aiking, Protein production: planet, profit, plus people?, Am. J. Clin. Nutr., 2014, 100(1), 483S–489S, DOI:10.3945/ajcn.113.071209.1.
- L. H. Fasolin, R. N. Pereira, A. C. Pinheiro, J. T. Martins, C. C. P. Andrade, O. L. Ramos and A. A. Vicente, Emergent food proteins–towards sustainability, health and innovation, Food Res. Int., 2019, 125, 108586, DOI:10.1016/j.foodres.2019.108586.
- C. Zhang, X. Guan, S. Yu, J. Zhou and J. Chen, Production of Meat Alternatives Using Live Cells, Cultures and Plant Proteins, Curr. Opin. Food Sci., 2023, 43, 43–52, DOI:10.1016/j.cofs.2021.11.002.
-
J. A. Whittaker, R. I. Johnson, T. J. Finnigan, S. V. Avery and P. S. Dyer, The Biotechnology of Quorn Mycoprotein: Past, Present and Future Challenges, in Grand challenges fungal biotechnology, Springer, 2020, pp. 59–79 Search PubMed.
- M. I. Ahmad, S. Farooq, Y. Alhamoud, C. Li and H. Zhang, A Review on Mycoprotein: History, Nutritional Composition, Production Methods, and Health Benefits, Trends Food Sci. Technol., 2022, 121, 14–29, DOI:10.1016/j.tifs.2022.01.027.
- A. Denny, B. Aisbitt and J. Lunn, Mycoprotein and Health, Nutr. Bull., 2008, 33(4), 298–310, DOI:10.1111/j.1467-3010.2008.00730.x.
- M. Asgar, A. Fazilah, N. Huda, R. Bhat and A. Karim, Nonmeat Protein Alternatives as Meat Extenders and Meat Analogues, Compr. Rev. Food Sci. Food Saf., 2010, 9(5), 513–529, DOI:10.1111/j.1541-4337.2010.00124.x.
- C. Landeta-Salgado, P. Cicatiello and M. E. Lienqueo, Mycoprotein and hydrophobin like protein produced from marine fungi Paradendryphiella salina in submerged fermentation with green seaweed Ulva spp, Algal Res., 2021, 56, 102314 CrossRef.
- S. F. S. Reihani and K. Khosravi-Darani, Mycoprotein production from date waste using Fusarium venenatum in a submerged culture, Appl. Food Biotechnol., 2018, 5(4), 243–352 CAS.
- J. Liang, N. Xu, A. K. Nedele, M. Rigling, L. Zhu, Y. Zhang and Y. Zhang, Upcycling of Soy Whey with Ischnoderma benzoinum toward Production of Bioflavors and Mycoprotein, J. Agric. Food Chem., 2023, 71(23), 9070–9079 CrossRef CAS PubMed.
- M. Umesh, S. Suresh, A. S. Santosh, S. Prasad, A. Chinnathambi, S. Al Obaid, G. K. Jhanani and S. Shanmugam, Valorization of pineapple peel waste for fungal pigment production using Talaromyces albobiverticillius: insights into antibacterial, antioxidant and textile dyeing properties, Environ. Res., 2023, 229, 115973 CrossRef CAS PubMed.
- S. Kim and C. H. Kim, Production of cellulase enzymes during the solid-state fermentation of empty palm fruit bunch fiber, Bioprocess Biosyst. Eng., 2012, 35, 61–67 CrossRef CAS PubMed.
- F. Stoffel, W. de Oliveira Santana, J. G. N. Gregolon, T. B. L. Kist, R. C. Fontana and M. Camassola, Production of edible mycoprotein using agroindustrial wastes: influence on nutritional, chemical and biological properties, Innovative Food Sci. Emerging Technol., 2019, 58, 102227, DOI:10.1016/j.ifset.2019.102227.
-
M. Cebrián and J. Ibarruri, Filamentous fungi processing by solid-state fermentation, in Current Developments in Biotechnology and Bioengineering, Elsevier, 2023, pp. 251–292 Search PubMed.
- U. Schweiggert-Weisz, P. Eisner, S. Bader-Mittermaier and R. Osen, Food proteins from plants and fungi, Curr. Opin. Food Sci., 2020, 32, 156–162 CrossRef.
- M. C. Okeudo-Cogan, B. S. Murray, R. Ettelaie, S. D. Connell, S. J. Radford, S. Micklethwaite and A. Sarkar, Understanding the microstructure of a functional meat analogue: demystifying interactions between fungal hyphae and egg white protein, Food Hydrocolloids, 2023, 140, 108606 CrossRef CAS.
- U. Javourez, E. A. Rosero Delgado and L. Hamelin, Upgrading agrifood co-products via solid fermentation yields environmental benefits under specific conditions only, Nat. Food, 2022, 3(11), 911–920 CrossRef CAS PubMed.
-
Grand challenges in fungal biotechnology, ed. H. Nevalainen, Springer, Cham, Switzerland, 2020 Search PubMed.
- G. Bakratsas, A. Polydera, O. Nilson, A. V. Chatzikonstantinou, C. Xiros, P. Katapodis and H. Stamatis, Mycoprotein Production by Submerged Fermentation of the Edible Mushroom Pleurotus ostreatus in a Batch Stirred Tank Bioreactor Using Agro-Industrial Hydrolysate, Foods, 2023, 12(12), 2295 CrossRef CAS PubMed.
- X. Zhao, J. Chai, F. Wang and Y. Jia, Optimization of Submerged Culture Parameters of the Aphid Pathogenic Fungus Fusarium equiseti Based on Sporulation and Mycelial Biomass, Microorganisms, 2023, 11(1), 190 CrossRef CAS PubMed.
- U. T. Dudekula, K. Doriya and S. K. Devarai, A critical review on submerged production of mushroom and their bioactive metabolites, 3 Biotech, 2020, 10, 1–12 CrossRef PubMed.
- Y. Deng, R. Wang, Y. Zhang, J. Li and R. Gooneratne, Effect of Amino Acids on Fusarium oxysporum Growth and Pathogenicity Regulated by TORC1-Tap42 Gene and Related Interaction Protein Analysis, Foods, 2023, 12(9), 1829 CrossRef CAS PubMed.
- F. Hashempour-Baltork, B. Jannat, M. Dadgarnejad, A. Mirza Alizadeh, K. Khosravi-Darani and H. Hosseini, Mycoprotein as chicken meat substitute in nugget formulation: physicochemical and sensorial characterization, Food Sci. Nutr., 2023, 11, 4289–4295 CrossRef CAS PubMed.
- S. Smetana, D. Ristic, D. Pleissner, H. L. Tuomisto, O. Parniakov and V. Heinz, Meat substitutes: resource demands and environmental footprints, Resour., Conserv. Recycl., 2023, 190, 106831 CrossRef PubMed.
- P. Prakash, S. K. R. Namasivayam and S. Swetha, Design of medium components for the enhanced production of mycoprotein by Fusarium venenatum using Plackett Burman model, Res. J. Pharm., Biol. Chem. Sci., 2015, 6(1), 1251–1255 Search PubMed , http://www.rjpbcs.com/pdf/20156(1)/[154].pdf.
- P. F. Souza Filho, R. B. Nair, D. Andersson, P. R. Lennartsson and M. J. Taherzadeh, Vegan-mycoprotein concentrate from pea-processing industry byproduct using edible filamentous fungi, Fungal Biol. Biotechnol., 2018, 5(1), 1–10 CrossRef PubMed.
- J. Ahlborn, A. Stephan, T. Meckel, G. Maheshwari, M. Rühl and H. Zorn, Upcycling of food industry side streams by basidiomycetes for production of a vegan protein source, International Journal of Recycling Organic Waste in Agriculture, 2019, 8, 447–455 CrossRef.
- S. M. Hosseini and K. Khosravi-Darani, Response surface methodology for mycoprotein production by Fusarium venenatum ATCC 20334, J. Bioprocess. Biotech., 2011, 1(1), 1–5 Search PubMed.
- R. Gmoser, R. Fristedt, K. Larsson, I. Undeland, M. J. Taherzadeh and P. R. Lennartsson, From stale bread and brewers spent grain to a new food source using edible filamentous fungi, Bioengineered, 2020, 11(1), 582–598 CrossRef CAS PubMed.
- D. Edwards and J. Cummings, The Protein Quality of Mycoprotein, Proc. Nutr. Soc., 2010, 69(OCE4), E331, DOI:10.1017/S0029665110001400.
- E. Derbyshire and K. T. Ayoob, Mycoprotein: Nutritional and Health Properties, Nutr. Today, 2019, 54(1), 7–15, DOI:10.1097/NT.0000000000000316.
-
T. Finnigan, L. Needham and C. Abbott, Mycoprotein: a healthy new protein with a low environmental impact, in Sustainable protein sources, Academic Press, 2017, pp. 305–325 Search PubMed.
- F. Saeed, M. Afzaal, A. Khalid, Y. A. Shah, H. Ateeq, F. Islam, N. Akram, A. Ejaz, G. A. Nayik and M. A. Shah, Role of mycoprotein as a non-meat protein in food security and sustainability: a review, Int. J. Food Prop., 2023, 26(1), 683–695, DOI:10.1080/10942912.2023.2178456.
- Quorn sustainability Report, 2022, http://www.quorn.co.uk.
- A. Cherta-Murillo, A. Lett, J. Frampton, E. Chambers, T. Finnigan and G. Frost, Effects of mycoprotein on glycaemic control and energy intake in humans: a systematic review, Br. J. Nutr., 2020, 123(12), 1321–1332, DOI:10.1017/S0007114520000756.
- M. Coelho, A. Monteyne, M. Dirks, T. Finnigan, F. Stephens and B. Wall, Daily mycoprotein consumption for 1 week does not affect insulin sensitivity or glycaemic control but modulates the plasma lipidome in healthy adults: a randomised controlled trial, Br. J. Nutr., 2021, 125(2), 147–160, DOI:10.1017/S0007114520002524.
- J. Bottin, J. Swann, E. Cropp, E. Chambers, H. Ford, M. Ghatei and G. Frost, Mycoprotein reduces energy intake and postprandial insulin release without altering glucagon-like peptide-1 and peptide tyrosine-tyrosine concentrations in healthy overweight and obese adults: a randomised-controlled trial, Br. J. Nutr., 2016, 116(2), 360–374, DOI:10.1017/S0007114516001872.
- M. Dunlop, S. Kilroe, J. Bowtell, T. Finnigan, D. Salmon and B. Wall, Mycoprotein represents a bioavailable and insulinotropic non-animal-derived dietary protein source: a dose–response study, Br. J. Nutr., 2017, 118(9), 673–685, DOI:10.1017/S0007114517002409.
- M. O. Coelho, A. J. Monteyne, M. V. Dunlop, H. C. Harris, D. J. Morrison, F. B. Stephens and B. T. Wall, Mycoprotein as a possible alternative source of dietary protein to support muscle and metabolic health, Nutr. Rev., 2020, 78(6), 486–497, DOI:10.1093/nutrit/nuz077.
- A. B. Thomas, T. D. Shetane and R. G. Singha, Employing Central Composite Design for Evaluation of Biomass Production by Fusarium venenatum: In Vivo Antioxidant and Antihyperlipidemic Properties, Appl. Biochem. Biotechnol., 2017, 183, 91–109, DOI:10.1007/s12010-017-2432-5.
- C. H. S. Ruxton and B. McMillan, The impact of mycoprotein on blood cholesterol levels: a pilot study, Br. Food J., 2010, 112(10), 1092–1101, DOI:10.1108/00070701011080221.
- S. Gorissen and O. Witard, Characterising the muscle anabolic potential of dairy, meat and plant-based protein sources in older adults, Proc. Nutr. Soc., 2018, 77(1), 20–31, DOI:10.1017/S002966511700194x.
- S. H. Gorissen, A. M. Horstman, R. Franssen, J. J. Crombag, H. Langer, J. Bierau, F. Respondek and L. J. Van Loon, Ingestion of Wheat Protein Increases in Vivo Muscle Protein Synthesis Rates in Healthy Older Men in a Randomized Trial, J. Nutr., 2016, 146(9), 1651–1659, DOI:10.3945/jn.116.231340.
- A. Cherta-Murillo and G. Frost, The association of mycoprotein-based food consumption with diet quality, energy intake and non-communicable diseases' risk in the UK adult population using the National Diet and Nutrition Survey (NDNS) years 2008/2009–2016/2017: a cross-sectional study, Br. J. Nutr., 2022, 127(11), 1685–1694, DOI:10.1017/S000711452100218X.
- S. Smetana, A. Mathys and A. Knoch, Meat alternatives: life cycle assessment of most known meat substitutes, Int. J. Life Cycle Assess., 2015, 20, 1254–1267, DOI:10.1007/s11367-015-0931-6.
- Quorn sustainability Report, 2017, http://www.quorn.co.uk.
- R. Warner, Analysis of the Process and Drivers for Cellular Meat Production, Animal, 2019, 13(12), 3041–3058, DOI:10.1017/S1751731119001897.
-
M. H. Stipanuk and M. A. Caudill, Biochemical, Physiological, and Molecular Aspects of Human Nutrition-E-book, Elsevier Health Sciences, 2018 Search PubMed.
- T. Y. Tong, P. N. Appleby, K. E. Bradbury, A. Perez-Cornago, R. C. Travis, R. Clarke and T. J. Key, Risks of Ischaemic Heart Disease and Stroke in Meat Eaters, Fish Eaters, and Vegetarians over 18 Years of Follow-up: Results from the Prospective EPIC-Oxford Study, BMJ, 2019, 366 DOI:10.1136/bmj.l4897.
- E. J. Van Loo, V. Caputo and J. L. Lusk, Consumer Preferences for Farm-raised Meat, Lab-grown Meat, and Plant-based Meat Alternatives: Does Information or Brand Matter?, Food Policy, 2020, 95, 101931 CrossRef.
- V. Meyer, E. Y. Basenko, J. P. Benz, G. H. Braus, M. X. Caddick, M. Csukai, R. P. De Vries, D. Endy, J. C. Frisvad and N. Gunde-Cimerman, Growing a Circular Economy with Fungal Biotechnology: A White Paper, Fungal Biol. Biotechnol., 2020, 7(1), 1–23 CrossRef PubMed.
- E. J. Derbyshire and J. Delange, Fungal Protein–What Is It and What Is the Health Evidence? A Systematic Review Focusing on Mycoprotein, Frontiers in Sustainable Food Systems, 2021, 5, 581682 CrossRef.
- C. L. Salgado, R. Muñoz, A. Blanco and M. E. Lienqueo, Valorization and upgrading of the nutritional value of seaweed and seaweed waste using the marine fungi Paradendryphiella salina to produce mycoprotein, Algal Res., 2021, 53, 102135 CrossRef.
|
This journal is © The Royal Society of Chemistry 2024 |
Click here to see how this site uses Cookies. View our privacy policy here.