A comprehensive review of food waste valorization for the sustainable management of global food waste
Received
6th September 2023
, Accepted 13th November 2023
First published on 4th December 2023
Abstract
Food waste (FW) is a global problem and a hidden opportunity for the value-added product conversion. Thus, food waste valorization is a growing science toward the waste-to-wealth conversion. FW can be generated either on a domestic or industrial scale, even if a zero-waste policy is practiced, owing to the unavoidable portion of food waste generated during the processing, cooking, and transportation of food materials. Previous studies have reported that FW is a potential sink for valuable bioactive molecules and bioenergy. In addition, earlier reports noted that the application of contemporary and advanced valorization processes for food waste management was limited to developed countries, and a significant portion of FW remained untouched owing to the lack of research in the rest of the global arena. As a result, this comprehensive review scrutinized several vital and advanced options for the sustainable valorization of global food waste, focusing on its prospects and challenges. In particular, this study deciphers the potential of unexplored valorization approaches and integrated biorefinery strategies for the holistic management of global food waste. Owing to the unavoidable waste generation during food processing, handling, and transportation, the sustainable valorization of FW is a phenomenal option for meeting the sustainable development goals (SDGs) of the United Nations (UN). Finally, this review paves the way for adopting sustainable technologies to convert waste into wealth through integrated valorization and biorefinery approaches toward the efficient recycling of global food waste.
Sustainability spotlight
In the modern society, food waste derived from households (kitchens) and industries is considered a critical environmental concern because of its underutilization and the lack of sustainable recycling. Moreover, food waste and industrial steams are sources of hidden bioactive molecules and value-added products. Despite numerous possibilities for food waste management, the waste-to-wealth conversion through sustainable approaches is limited. Consequently, the potential of food waste remains unexplored because of the lack of sufficient research and policymaking. Thus, this comprehensive review summarizes the existing approaches towards food waste valorization, including cutting-edge sustainable technologies for the proper utilization and recycling of unutilized but potential waste streams in light of SDG 2 (zero hunger), SDG 7 (affordable and clean energy), and SDG 12 (responsible consumption and reproduction).
|
1. Introduction
In the global context, one of the most common types of biowaste generated today is food waste (FW). The manufacturing, handling, storage, processing, distribution, and consumption of food largely contributes to the quantity of global FW generation.1,2 One of the ways towards food-waste management is to segregate the FW into edible and non-edible waste during its disposal.2 Over the last decade, food processing has become one of the fastest-growing industries in the world and a considerable amount of waste is produced during the processing of food. According to statistics, the production, distribution, and retail of food and beverages account for approximately 20% of the total 14 MMT of the waste generated annually.3 The fruit and vegetable (F & V) industry generates more waste compared to other food processing sectors, accounting for 25–30% of the total waste, which might be anything from peels and rinds to seeds, cores, rags, stones, pods, vines, skins, and pomaces.4 The processing of fruits and vegetables generates a massive amount of trash, and the proper disposal of this waste increases overall production costs. Therefore, in most situations, fruit and vegetable wastes are discarded into the environment to reduce the production costs.5 As fruit and vegetable waste is biodegradable, it decomposes rapidly, emitting unpleasant odors. If not managed promptly, this can lead to significant environmental and health issues. Fruits and vegetable waste that is improperly disposed of can produce germs and attract disease-carrying insects, such as flies and mosquitoes. It can also contaminate the surface and groundwater, leading to phytotoxicity6 and significant health-related issues.6,7
A daily diet that includes at least five servings of fruits and vegetables has been shown to significantly lower the risk of several chronic illnesses and the onset of age-related issues.8 Fruits and vegetables have special health advantages because of their abundance of bioactive substances; these include phenolic compounds, terpenes, terpenoids, alkaloids, flavonoids, carotenes, and other bioactives.9,10 Bioactive compounds, including primary metabolites, such as proteins, carbohydrates (cellulose, hemicellulose, lignin, and pectin), and lipids, are often found in fruits and vegetables. These bioactive compounds are typically present at very low concentrations in these foods. Plants produce these metabolites to thrive and enhance their ability to withstand environmental stress, diseases, and sunlight.10 These biologically active molecules have antioxidant, antibacterial, and anti-inflammatory properties that help combat chronic diseases, such as oxidative stress. The efficacy of these compounds depends on their bioactivity, chemical structure, and dosage used.11
Governments and the food industry have made it one of the top priorities to eliminate or significantly reduce food waste. Waste valorization in a circular economy involves the production of valuable products, such as fuels, materials, and chemicals. In addition to producing value-added goods, such as organic fertilizers, animal feed, biofuels, and electricity from food waste, it contributes to economic, social, and environmental improvements.12,13 Over the last decade, numerous studies have focused on the transformation of value-added products from FW, including edible ingredients, functional foods, nutraceuticals, medicines, and cosmetics.14–17
However, additional factors must be considered to ensure the suitability of industrial processes for various matrices. Ensuring adaptability to a variety of matrices, integrating all biorefinery plant activities, and maintaining environmental and economic sustainability are the essential aspects to consider. Therefore, choosing a particular extraction technique for one or more classes of bioactive compounds from waste depends on the compromise between different factors. The recovery and reuse of waste and by-products were performed according to a circular economy model that includes the concepts of biorefinery and industrial symbiosis. The goals of the relevant technologies and techniques are increased sustainability, no or reduced organic solvent usage, and minimal environmental effects. Furthermore, new procedures have recently been developed to chemically characterize vegetable and fruit waste and by-products, including sample preparation. This method has replaced conventional methods of solvent extraction using organic solvents, which can pose environmental and health concerns.18–21
In particular, circular economy practices are implemented for sustainable recycling and utilization of neglected agri-food wastes,22,23 while developing countries are still suffering from food waste due to the lack of economic funds, public awareness, and political will, among others.24,25 Developing countries such as China, Serbia, and India have started to implement such principles,26–28 although FW valorization will benefit through the circular economy regardless of countries' status across the world.29–31
Novel, easier, sustainable, safe, greener, and more cost-effective approaches for extracting value-added products that can be utilized as clean labels in food systems have been the subject of ongoing research. This review explores innovative eco-friendly methods for extracting bioactive compounds from fruit and vegetable wastes, particularly for food applications. This article compares these techniques to traditional processes, highlighting the advantages of biorefinery processes, including reducing waste generation, creating new revenue streams, combining multiple technologies, sharing resources, and possibly attaining energy self-sufficiency by producing biogas or using inert fiber materials as fuel.32 Research on the integrated exploitation of plant-derived waste in the production of diverse goods has evolved over the last decade.33 Therefore, this review aims to provide an updated summary of the most recent integrated processing techniques applied to different agriculture-derived waste or by-products toward sustainable recycling. Finally, sustainable recycling and the biorefinery processing of food wastes are critically discussed for sustainable waste management.
2. Review motivation and literature arrangement
In recent decades, food waste recycling and valorization have been considered a growing sustainable research theme worldwide. Thus, the literature search and arrangement were squeezed, scrutinized, and confined within the specified keywords during data archive collection for this comprehensive review to meet the specific objectives of this review. According to previous investigations, advanced food waste recycling and valorization approaches were practiced by specific countries but were limited in the rest of the world. Consequently, food waste containing hidden resources remains untapped, particularly on an industrial scale. According to the United Nations (UN) Sustainable Development Goals (SDGs), food waste management is highly recommended for the attainment of SDG 2 (zero hunger), SDG 7 (affordable and clean energy), and SDG 12 (responsible consumption and production). Therefore, the prime goal of this comprehensive review was to explore food waste as a global concern and the existing valorization approaches to strengthen the sustainable development goals of the United Nations. We carefully collected and thoroughly assessed previously published papers, including original research and review articles. We aimed to identify the main challenges in managing global food waste sustainably and to understand the primary issues faced during implementations. For this purpose, we utilized specific keywords such as “food waste recycling,” “food waste generation,” “valorization of food waste,” “food waste circular economy,” “food waste stream,” “sources of food waste,” “food waste conversion,” “challenges of food waste valorization,” and “solution for food waste management.” We conducted searches using renowned scholarly search engines, such as Web of Science, Scopus, Google Scholar, and reputable publishers' websites, to gather relevant data for this review.
The selected data archives were skimmed and narrowed down to meet the specific goals of this comprehensive review. The basic steps were the selection of raw data, exclusion/inclusion, revising the data archive, and finalizing literature for this review paper but we did not follow any structured review protocol. Although this review does not adhere to a specific methodology, its central theme revolves around sustainable global food waste management. It emphasizes the contemporary technologies for food waste recycling and their role in achieving the Sustainable Development Goals (SDGs) set forth by the United Nations. Initially, the collected papers (approximately 400, including original research and review papers) were critically analyzed, and the most relevant papers were finally chosen for establishing the review theme. The core ideas and highlights of this review, following critical evaluation, are summarized in Fig. 1.
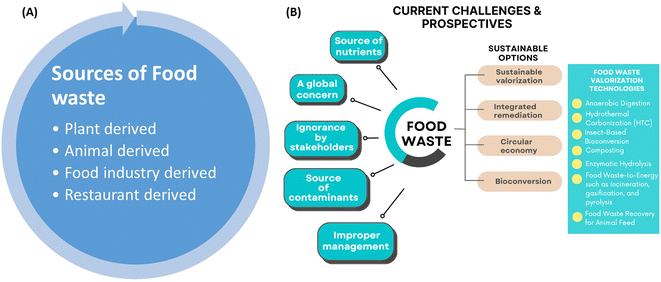 |
| Fig. 1 A schematic showing the global food waste originating from various sources (A) and current challenges and perspectives (B). The figure data were extracted from the critical summary of previous studies and is displayed to pinpoint the key findings of the review theme. | |
3. Food waste and environmental concern: a global macro problem
Food waste, food loss, and food surplus are the key terminologies of global food waste generation.34 In general, food waste can be defined as a specific part of edible food that becomes inedible as a result of anthropogenic and pest damage, intentional waste, pre- and post-harvest losses, and immature consumption during the food supply chain.3,35,36 However, food waste is considered an emerging concern throughout the world. Substantial nutritional, environmental, and economic losses are evident from global food waste due to detrimental consequences and management complexities.37–39 The nutritional and economic losses caused by food waste have been studied globally. Approximately 35% of the total loss of food was documented through different stages of the food supply chain, from production (pre-harvesting) to consumption (post-harvesting) pathways.1 Additionally, a combined and pioneer appraisal was reported to focus on the compound nutritional and environmental consequences of food waste in a global context.38 Previous studies have noticed a positive correlation between greenhouse gas (GHS) footprint and water footprint with the reduction of global food waste.38,40 Intensive agriculture with hybrid and exhaustive crop species may require a large amount of major macronutrients, such as nitrogen and phosphorus. A countable amount of global nitrogen was lost through food waste at various food supply chain processes on a European scale.41
Several global and regional studies have explained the global challenges of food in terms of nutritional, environmental, and economic aspects.1,38,39,42 Food waste is also called Food Loss and Waste (FLW). The generation of FLW has been facilitated through various household and kitchen wastes in several countries around the world without proper recycling.1,43 The recent global pandemic (COVID-19) and the resulting lockdown in many affected countries may seriously threaten the current food waste management policies. Thus, food waste has emerged as a global threat due to poor management in such an adverse global pandemic.44 Consequently, global food waste and food loss have been reported as priority research themes for highly industrial regions of the world.45 Therefore, global environment and food entities such as the United Nations Environment Programme (UNEP), Food and Agriculture Organization (FAO), and International Food Policy Research Institute (IFPRI) have revised several rules and act with respect to food waste minimization to sustain food security and food safety, and reduce global hunger.34,35,37 Thus, waste-to-wealth technology and sustainable recycle, reduce, reuse, and recovery (4R) waste management concepts have been attempted to manage food waste in a sustainable environment policy.45–47
3.1. Agri-food waste and environmental pollutants
FW derived from agricultural goods has been reported as a potential bio-toolkit for soil improvement due to the inclusion of numerous nutrients.48,49 Pesticides, trace metals, volatile organic compounds (VOCs), and other emerging pollutants may limit the use of FW as a soil amendment in the long run.49,50 Chemical substances that have been present in the environment for a long time but have recently gained prominence owing to human activities are known as emerging contaminants (ECs).51,52 Antibiotics, pesticides, pharmaceuticals, nanoparticles, medicinal equipment, hormones, industrial materials, personal care items, veterinary medicines, contaminated and spoiled food, fertilizers, and hazardous metabolites have all been identified in agricultural areas as potential ECs.53,54
Soil contamination by ECs is a worldwide problem, and its effects unreasonably impact the global pollution index because of their increased industrial and developmental activity.55–58 Numerous studies have shown that pesticides are used indiscriminately over acceptable levels in many populous nations, including India and Bangladesh.53,59 In underdeveloped nations, the World Health Organization (WHO) reports approximately 3 million cases of pesticide poisoning and 22
000 deaths per year.60 Additionally, the widespread use of pesticides for the past five to six decades has resulted in increased health risks for all living things, including humans, due to the environmental degradation caused by the explosive growth of the pesticide industry.53,61
The illustration (Fig. 2) shows that surface runoff, agricultural irrigation, and precipitation can transport emerging contaminants through the food chain. These pollutants are now detected in several fresh and processed foodstuffs, such as fruits, vegetables, livestock, and fish, as well as in soil and water sources.53,62,63 In addition, plastic pollutants, including microplastics and nanoplastics, can accumulate and translocate through food waste into the food web chain, posing a great threat to aquatic and terrestrial food systems.64,65 The rising release of pollutants into water and soil poses a serious concern, as it endangers local plants and wildlife, harms soil fertility, and causes toxicity to soil organisms. Additionally, it contributes to antibiotic resistance in soil bacteria, hinders plant growth, and diminishes the survival and reproductive capabilities of soil invertebrates.66 The unpredictability of environmental pollutants such as heavy metals in FW might be viewed as a major impediment to protracted recycling and valorization of FW for agricultural purposes and value-added products.63,67 Although some microbiological and enzymatic degradation of pesticides and heavy metals has been studied, including MSW,52,68 further extensive and meticulous studies for sustainable recycling of FW are needed.
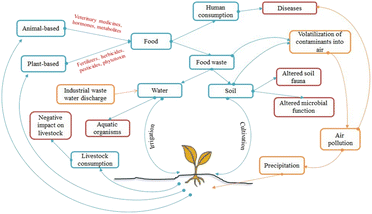 |
| Fig. 2 A simplified diagram showing the interaction of legacy chemicals and the chemicals of emerging concern, while food waste acts as the building block. | |
4. Food waste stream: a hidden treasure of bioactive molecules
Most organic waste and food industries leave huge byproducts as food waste streams. Food waste streams derived from the food industry are considered sources of diverse nutrients and bioactive compounds.46,47,69 Various food industries, such as fruit juice, cheese making, and brewery, are sources of essential minerals and nutrients. Carbohydrates, cellulose, hemicellulose, proteins, lignin, pectin, lipids, and antioxidants are the major detected nutrients and valuable compounds in the food waste streams of major food industries, including potato, cereal, fish, and fruit processing.70 Vegetable waste streams are a neglected portion of the food industry worldwide. However, the vegetable waste stream has been reported as a rich source of extracted pectin (a heteropolysaccharide derived from cell walls and lamellae of vegetables). Early observations documented the potential of rejected vegetable waste streams (carrot, green bean, and leek) from the food industry to extract commercial pectin.69 A recent study explored the conversion of wet waste streams in the USA into valuable biofuels, bioenergy, and bioproducts using waste-to-energy (WtE) approaches.71 Based on the study findings, four different wet wastes, namely, food waste, animal manure, sewage sludge, and oil, fat, and greases, were used in the WtE approach. All wet wastes, excluding oil, fat, and greases, were converted through conventional waste management strategies, including anaerobic digestion and incineration for bioconversion into valuable energy and biofuels.
Food loss is considered a critical global threat due to the negligence of nutrient and energy-rich food waste streams derived from major food industries. The Food and Agriculture Organization (FAO) of the United Nations has observed that the food loss in industries occurs because of the improper disposal of food waste streams by these respective industries.70 This leads to a considerable loss of potential nutrients and energy-rich food due to the improper management of food waste streams. Therefore, food waste valorization is receiving the researchers' attention to convert discarded food waste into high-value products and energy. The unscrupulous disposal of food waste due to regional ignorance of waste management rules causes environmental damage and economic challenges for the global circular economy. An encouraging review explained the conversion potential of several food waste streams into valuable biopolymers such as cellulose, chitin, collagen, lignin, hemicellulose, xanthan gum, organic acids, cooking oil, etc.72 The valorization from food waste streams is a sustainable approach concerning the circular economy, green environment, and recovery of valuable neglected food nutrients through conversion into precious bioproducts.70 The resource recovery (RR) strategy is linked to the water–energy–food cycle and is used to predict both sustainable economic turnover and environmentally friendly performance.73 The resource recovery technique is beneficial in the economic and socio-technical realms concerning the sustainable management of food waste streams. Thus, the sustainable and profitable conversion of food waste streams into valuable products was suggested for a win–win circular economy by properly managing industrial waste streams.47,72–74 The food waste stream management through various sustainable approaches is tremendously important for sociotechnical acceptance, in addition to environmental aspects.74,75 A list of commonly used food wastes associated with bioactive compounds is given in Table 1.
Table 1 Bioactive compounds from food waste streams and by-products
Categories |
Primary constituents |
Type of waste |
Methods of extraction |
Ref. |
Carbohydrates |
Pectin, chitin and chitosan, cellulose, xanthan gum, polyhydroxyalkanoate, polyhydroxybutyrate |
Citrus fruits, apple pomace, sweet potato pulp and peel, carrot, crustacean shells |
Freeze drying, acid-assisted solvent extraction, centrifugation, ethanol extraction, ultrasonic degradation, supercritical CO2 extraction |
6 and 76–84 |
Phenolic compound |
Chlorogenic acid, caffeic acid, ferulic acid, p-coumaric acid, sinapic acid, p-coumaroyl-quinic acid, hydroxybenzoic acid, gallic acid, ferulic acid, hydroxy-cinnamic acid derivatives, chlorogenic acids, geraniin, corilagin, gallic acids, ellagic acid, ellagitannins, chlorogenic acid, vanillic acid |
Olive mill wastewater, white grape pomace, apple pomace, peel and pulp of orange, lemon, plum pomace, mango kernel seed, banana peel, sweet cucumber, rambutan, dragon fruit, pineapple, guava, potato peel, beetroot pomace, broccoli |
Concentration, acid-assisted solvent extraction, ethanol precipitation, concentration, dilution, micro-filtration, ultra-filtration, supercritical fluid extraction, microwave-assisted extraction, ultrasound-assisted extraction, pressurized liquid extraction, enzyme-assisted extraction |
85–95
|
Flavonoids |
Isorhamnetin, kaempferol, quercetin, rhamnetin glycoconjugates, procyanidin b2, (−)-epicatechin, eriocitrin, hesperidin, narirutin, quercetin, isoquercitrin, fisetin, rutin, quercetin, kaempferol, myricitin, laricitrin, catechin epicatechin |
Apple pomace, citrus peel, mango kernel, banana peel, beetroot, broccoli |
Solvent extraction, supercritical fluid extraction, microwave-assisted extraction, ultrasound-assisted extraction, pressurized liquid extraction, enzyme-assisted extraction |
85–90, 93 and 94 |
Phytochemicals |
Anthocyanins, carotenoids, betalains, lycopene |
Apple pomace, plum pomace, mango peel, carrot, banana peel, berries, beetroot, tomato pomace and peel |
Enzymatic hydrolysis, solvent extraction, subcritical water extraction, microwave-assisted extraction, ultrasound-assisted extraction, pressurized liquid extraction, enzyme-assisted extraction |
88,94 and 96–99 |
Organic acids |
Acetic acid, fumaric acid, citric acid, lactic acid, succinic acid, propionic acid, gluconic acid |
Waste cheese whey, apple industry waste biomass, apple pomace ultrafiltration sludge, banana peel, citrus peel waste, fruit and vegetable waste, fruit and vegetable waste, apple pomace, sugarcane molasses |
Fermentation |
100–108
|
Lipid |
Grape seed oil, triglycerides, free fatty acids (linoleic, palmitic, and oleic acids) |
Brewers' spent grain, grape seeds |
Soxhlet extraction, pressurized carbon dioxide extraction with compressed carbon dioxide as solvent and ethanol as co-solvent |
109 and 110 |
Bioactive peptides |
Angiotensin-converting enzyme (ACE) inhibitory peptides, antioxidant peptides, anti-inflammatory peptides, antidiabetic peptides, antihypertensive peptides, lactokinins, lactoferricin, immunoglobulin-derived peptides |
Fish processing waste (head, bones, and skin), whey protein by-products, soybean residues, white grape pomace |
Water extraction, ultrasonic-assisted extraction, enzymatic-assisted extraction, alkaline solubilization, and acid precipitation, precipitation (isoelectric precipitation), high voltage electrical discharge, skimming, micro-filtration, ultra-filtration, filtration, freeze drying, enzymatic hydrolysis, fermentation |
81 and 110–114 |
Lignans |
Syringaresinol and secoisolariciresinol |
Flaxseed waste and sesame seed hulls |
Enzymatic-assisted extraction |
109 and 110 |
Essential oils |
Orange oil, lemon oil, grapefruit oil, oregano oil, thyme oil, rosemary oil, cinnamon oil, grape seed oil, vanilla oil, pumpkin seed oil, and almond oil |
Citrus peels, herb and spice residues, grape pomace, vanilla pods, seed and nuts waste, plum seed |
Cold pressing, steam distillation, solvent extraction, supercritical fluid extraction, microwave-assisted hydro-distillation |
115 and 116 |
5. Contemporary valorization approach for food waste conversion
Food waste valorization is an emerging strategy for converting various types of food waste into value-added products. To boost the circular economy approach, different innovative and sustainable anaerobic digestions have been optimized for efficient food waste management.44 Application of immobilized enzymes has also been reported to meet sustainable economic returns through bioconversion of food waste into valuable resources.43 Previous research has reviewed conventional (orthodox) and innovative (emerging) valorization approaches for vegetable and fruit wastes.117 However, conventional practices of food waste management may include animal feeding of surplus food materials, orthodox landfilling, composting for organic manure, and incineration as thermal treatments. The aforementioned individual techniques face challenges because of their limited efficiency, high energy consumption, high moisture content, low calorific value, variable composition, and potential contamination. These traditional methods can indirectly increase economic profits, but they may lead to the loss of valuable biomaterials extracted from food waste streams. Critical resource depletion, including nutrients and bioactive compounds, and adverse environmental impacts were predicted due to conventional and unplanned food waste management.118 Therefore, integrated and emerging household and food waste management was encouraged to meet the global legal frameworks for food security. Furthermore, traditional waste management poses multiple challenges to achieving sustainable development goals. These challenges include the generation of greenhouse gases, higher costs without value-added products, the release of high temperatures, and various air pollutants.118–120 Although several conventional approaches such as composting and vermicomposting, combustion, or incineration with proper landfilling may have a positive correlation with sustainable economic and environmental aspects, the commercialization of waste valorization through conventional management of food waste is still limited.117,120
Anaerobic digestion, composting, source degradation, and in situ conversion of several wastes, including food waste, food processing waste, and agricultural residues, have been reported as effective schemes for the conversion of food waste into biofertilizers.121 However, the resultant biofertilizers can replace a portion of the applied synthetic fertilizers in a waste valorization approach. Similarly, a contemporary study noticed four sustainable livestock waste valorization approaches. The approaches included anaerobic co-digestion and mono-digestion using biological drivers, gasification, and pyrolysis (thermal technology) to replace conventional landfilling and incineration strategies.122 The integrated food waste management through valorization was anticipated by both institutional bodies and consumers' participation. A survey evaluated Italian consumers' willingness and overall perception toward food waste valorization. The study depicted a comprehensive scenario between the consumers and concerned authorities to participate actively in sustainable food waste management through various valorization techniques, either by conventional or emerging approaches.123 Furthermore, a combination of sustainable, environmentally friendly, renewable, and inexpensive approaches to waste management toward a circular bioeconomy has been suggested.124 According to the findings of several conceptual, behavioral, empirical, and cohesive studies, global optimization and synchronization efforts have been made to establish a circular bioeconomy through food waste valorization. To date, both traditional and contemporary methods of food waste valorization have been merged to create an integrated waste management plan within a European context that also applies to developed nations worldwide. However, the emerging enzymatic conversion of food waste into value-added compounds has become a priority in the sustainable food waste valorization race in the industrially driven regions of the world.74,75,117
6. Conversion of value-added products from horticulture waste (HW)
Horticultural waste (HW) is an unavoidable waste generated during the harvesting of fruits and vegetables from the field and during the processing of fruits and vegetables in the food industry.125,126 Several variables and procedures in ‘farm to fork’ food web chains (i.e., harvesting techniques, processing factors, peeling factors, and feedstock heterogeneity) can influence the cohort of HW derived from fruits and vegetables.127,128 These HWs act as reservoirs for numerous bioactive molecules and extractable value-added products.129 Good numbers of recent research and review papers have documented the perspective of a ‘waste to value’ strategy toward sustainable management of horticultural and agricultural wastes.33,126,130–132 Although waste from fruits and vegetables is regarded as a possible source for various new value-added goods, the untapped potential of sustainable techniques for this ‘waste to value’ approach has yet to be fully explored.125,133 Therefore, the bioconversion and extraction of high-value products from fruit and vegetable wastes should be meticulously explored Fig. 3.
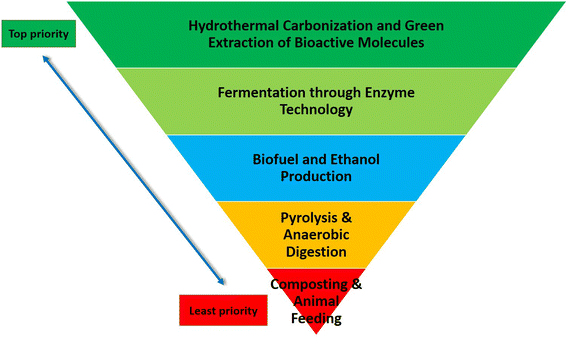 |
| Fig. 3 A hypothetical hierarchy pyramid of food waste management and the valorization approach deciphering the least priority to top priority approaches. This figure depicts the vital points concerning policy implications toward advanced food waste recycling. | |
Generally, waste derived from fruits and vegetables is the key harbor of many significant biomolecules, including carbohydrates, proteins, lipids, and volatiles.134,135 If fruit and vegetable waste valorization is not correctly managed, these valuable but unusable or unrecognized bioactive compounds may be lost. However, several conventional and advanced approaches for the sustainable bioconversion of HW into value-added products have been reported.126,136 HW is heterogeneous and largely composed of peels and seeds of fruits and vegetables.137 Thus, a major bioactive molecule of HW is either an essential oil (flavoring products) or a preservative (secondary metabolites). In addition, the pulp of HW may contain other bioactive materials such as carotenoids, sugars, and vegetative tissues, including lignocellulose fibers.125–137 Despite the potential of HW, its sustainable bioconversion and utilization in large-scale biorefineries are limited.138 Among the bioactive compounds derived from fruit and vegetable wastes, organic acids have been the most reported.127 For instance, citric acid has been extracted from banana and citrus peel wastes, apple pomace wastes, etc.139 Succinic acid, and lactic acid have been extracted from wheat bread and apple pomace wastes,140 respectively.
HW, particularly fruit and vegetable waste (FVW), is a rich source of bioactive materials and dietary fibers compared to edible portions.141 Consequently, a large portion of inedible and unavoidable FVW remains untapped for the extraction of high-value compounds compared to the edible portions of horticultural crops.129,142 Several emerging high-value bioactive compounds such as polyphenols, biofuels, and biodiesel have been extracted from agricultural residues and horticultural waste.68,137,143 Amongst the high-value compounds, essential oils, carotenoids, phenolic compounds, saponins, sterols, phytic acids, and polyphenols have been extensively studied and extracted from the HW derived from fruits and vegetables.129,144 In addition, several antioxidants and antimicrobial activities of the extracted bioactive molecules from FVW suppressed Listeria-oriented foodborne diseases in humans.129,145 Likewise, the by-products of fruits and vegetables have been considered potential feedstocks for extracting several dietary fibers.146
Several classical and modern extraction approaches have been found to be effective in extracting these potential high-value compounds from HW.147 Traditional extraction methods such as vigorous grinding, maceration, drenching, Soxhlet, heating, and supercritical fluid extractions are highly discouraged during sustainable bioconversion of fruit and vegetable wastes, owing to high energy inputs and cost-effectiveness concerns.148–150 Eventually, several modern extraction methods have replaced older techniques and have emerged as green and sustainable approaches for converting agricultural waste into valuable resources. These methods include microwave-assisted, ultrasound-assisted, pressurized solvents, enzymatic extraction, negative pressure extraction, and high-pressure homogenization.99,147 In contrast, uncertain byproduct generation during conventional extraction approaches is doubtful.147 So, alternative green extraction solvents are beneficial and sustainable with regard to uncertain toxicity.151,152 However, anaerobic digestion (AD) is considered a robust approach for the effective bioconversion of FVW into value-added products.152 Thus, the adoption of advanced and sustainable extraction strategies for the efficient bioconversion of HW will open new avenues for food waste valorization (Table 2).
Table 2 The conversion of value-added products from horticulture waste
Food processing sector |
Types of food waste |
Products |
Conversion techniques |
Application |
Ref. |
Fruit and vegetables processing sector |
Peels, pulp, pomace, stalks, leaves, seeds, cores, unharvested parts, spoiled and damaged parts |
Polyphenols, organic acids, carotenoids, anthocyanin, flavonoids, dietary fibers, tocopherol, vitamins, aeromantic compounds, phytochemicals, stilbenes, lignans, pectin, cellulose, nutrients (N, P, K) |
Drying, solid-state fermentation, solvent extraction, ultrasound, enzyme and microwave-assisted extraction, pressurized liquid extraction, supercritical CO2 extractions, aerobic digestion, and composting |
Food fortification |
153–159
|
Enhance nutritional value and oxidative stability |
Novel functional food ingredients |
Extracted oils in the cosmetics and food industries |
Promote the growth and development of cells production of probiotics, food additives, flavor enhancers, chewing gum, and stabilizers for oil–water emulsions |
Nutraceuticals, functional foods, baked goods such as muffins and brownies, as well as packaging materials |
Biodegradable and edible plastics |
Enzyme production, cancer prevention, cardio, and digestive disease prevention |
Production of biogas, animal feed, fertilizer, organic acids, enzymes, and bioactive compounds |
Dairy industry |
Dairy processing water, dairy sludge & effluent, whey, dissolved organic compounds, lipids, carbohydrates, and organic substances |
Whey and whey permeate, bio-plastics, biofuels, bioenergy, organic acids, peptides |
Physicochemical treatment, micro- and nano-filtration, aerobic and anaerobic digestion, advanced biotechnological processes, coagulation, fermentation, aerated lagoons |
Production of functional food, emulsifier, single-cell protein, polysaccharides, lipase, citric acid, lactic acid, propionic acid, xanthan gum, whey protein isolate, polyhydroxyalkanoates, polyhydroxybutyrates, alcoholic beverages, ethanol |
160–165
|
Animal products processing |
Skin, blood, gut, horns, bones, liver, lung, kidney, tendons, brains, spleen, and internal organs, poultry feather |
Nutrients, fiber, energy, meat powder, peptides or biopeptides, collagen, essential amino acids, saturated and unsaturated fatty acids, biodiesel, fibreboard, bioplastic films |
Solid state fermentation (SSF), enzymatic & non-enzymatic hydrolysis, aerobic digestion, chemical treatment, water extraction, mincing, concentration, colloid milling, homogeneity, and spray drying |
Utilization as animal feed, biodiesel, or biogas production, the development of dietetic items such as chitosan, the extraction of natural colors, and the incorporation of collagen into cosmetics |
166–169
|
It is a highly valuable reservoir of many essential trace minerals |
The potential applications of this substance include its use as a protein supplement, a feasible alternative to milk, a supplementary source of lysine, and a stabilizer for vitamins |
Fish, seafood, seaweed processing |
Scale, bone, muscle, liver, gut, head, blood skin, processing water seaweed, oyster and crustaceans' shells |
Protein, peptide, collagen, gelatin, chitin and chitosan, alginate, agar, and carrageenan, fish meal, fish oil, fertilizer/manure, fish silage, fish sauce |
Solid state fermentation (SSF), enzymatic hydrolysis, chemical treatment |
The production of functional foods, nutraceuticals, antifreeze compounds, surimi, fish protein hydrolysates, fish protein concentrates, fish enzymes, gelatin, collagen, carotenoids, chitin, chitosan, glucosamine, and fermented fish products is undertaken in the manufacturing industry |
170–174
|
7. Integrated biorefinery: an innovative approach
Integrated valorization methods involve a combination of various waste treatment and conversion processes to maximize the value extracted from waste streams, including food waste. These integrated approaches aim to enhance resource efficiency, reduce waste, and support sustainability. Integrated valorization methods can include a combination of biological, chemical, and thermal processes as well as the recovery and utilization of valuable byproducts. Over the last few years, advanced strategies have explored HW, specifically FVW, for the production of high-value bioactive compounds, bioenergy, and biofuels.175,176 The ‘waste-to-energy’ concept was adopted for biomass conversion from FVW into bioenergy and biofuels.125,126,131 Several potential ‘waste-to-energy’ approaches, such as landfilling, composting, anaerobic digestion, enzymatic conversion, etc., have been documented for efficient biorefinery of HW.126 Among the adopted approaches, anaerobic digestion (AD) is the most efficient for large-scale industrial bioconversion of fruit and vegetable wastes.152 However, various value-added products, including bioenergy, biofuel, biohydrogen, biodiesel, bioethanol, and volatile fatty acids, have been extracted from horticultural wastes.125,177,178 Similarly, a previous observation179 noted the potential of agri-food wastes for the sustainable management of wastewater through bioconversion of FVW biomass.
The biorefinery of HW (i.e., pineapple waste into value-added products, and synthesis of nanoparticles from horticultural wastes) has been documented in previous studies.180,181 Likewise, a previous study182 documented the potential of carrot peel waste for the bioconversion of pectin and carotene through advanced biorefinery approaches. In addition, another study183 demonstrated the conversion of greenhouse horticultural waste into value-added products through bioconversion and biorefinery approaches. These approaches can effectively valorize the unavoidable yet potential food waste and waste streams through the concept of bioeconomy. However, a recent study in Singapore reported the potential of HW for bioconversion into biochar through gasification strategies.184 In the biorefinery approach, HW biomass can be converted into bioenergy or biofuels through various approaches, including acidogenesis, solventogenesis, fermentation, microbial bioconversion, and electrolysis.185,186 Microbial fuel cells have the ability to produce bioelectricity by oxidizing organic chemicals. On the other hand, bioplastics can be generated by fermenting or bioconverting fruit and vegetable wastes that are abundant in lignin and other fibers. Microorganisms or enzymes can be utilized to decompose the complicated organic compounds into simpler molecules such as sugars, which can subsequently be subjected to additional processing to produce sustainable bioplastics.125,130
Numerous studies have reported sole biorefinery approaches for the efficient bioconversion of HW into value-added energy or fuels.180,181,187 However, owing to cost-effectiveness, incomplete bioconversion, and sustainability for large-scale applications, these single biorefineries are not suitable. Thus, an integrated biorefinery will open a new research area to solve this problem during the holistic and cost-effective integrated management of fruit and vegetable wastes.99,133,187 An integrated biorefinery coupled with green extraction approaches will enhance the bioeconomy through the sustainable circular economy of horticultural waste valorization.33,138,143,150,188
8. Immobilized enzymes for food waste conversion
Food waste valorization is an attempt to convert discarded waste materials into valuable products such as biopolymers, biofuels, and biocompounds. Numerous advanced chemical and microbial extractions of valuable biopolymers from different food waste streams have been reviewed.72 However, both free and immobilized enzymes have been reported to convert food waste into valuable bioactive molecules as alternatives to conventional methods of food waste valorization.189,190 Utilizing immobilized enzymes in food waste valorization presents multiple advantages, including operational stability, reusability, and resilience to temperature and pH fluctuations. These attributes enhance the efficiency and effectiveness of bioconversion processes for food waste streams.174,175 The industrial application of various immobilized enzymes was documented for application lenience over free enzymes. To enhance the efficient conversion of organic waste into valuable products, researchers have explored various immobilization techniques, including physical adsorption, entrapment, covalent bonding, and crosslinking. These methods have been applied using diverse support media such as alginate, glass beads, agar, and clay minerals.190,191 The food waste stream is a rich source of diverse carbohydrates including polysaccharides and disaccharides. Carbohydrates derived from the food waste stream can be effectively converted to bioactive galactosides through the immobilized enzyme β-galactosidase on a suitable nanocatalytic support surface.192 The novel enzyme β-galactosidase, derived from different microbial strains, can also serve as an immobilized enzyme when paired with an appropriate support surface. This combination enables the synthesis of a wide range of disaccharides from dairy-waste materials.189 Similarly, pectin is one of the most important carbohydrates derived from vegetable waste streams. Pectin synthesis was facilitated using an immobilized pectin-degrading enzyme (derived from Bacillus licheniformis) on agar–agar support media.193 Immobilized enzyme-based pectin synthesis is a sustainable approach over other conventional chemical techniques owing to the low cost and reusability of enzyme immobilization Fig. 4.
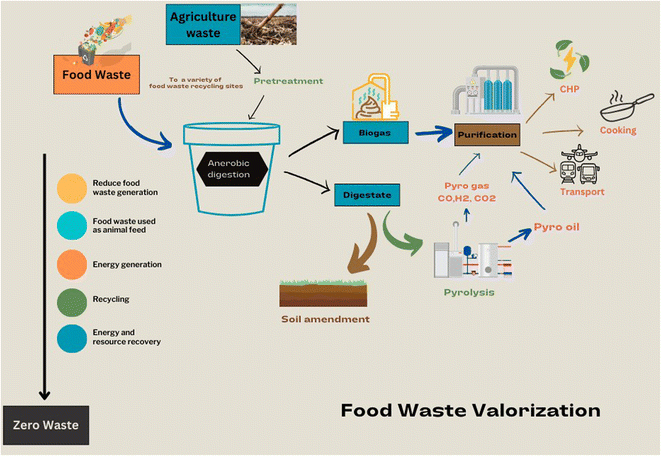 |
| Fig. 4 An updated valorization approach, including the integrated biorefinery for the sustainable recycling of food waste and agro-food wastes toward the zero waste campaign. | |
Chitin is a major carbohydrate in fish and crustacean waste streams. The dual reactor-based uninterrupted production of chitosan-based chitooligosaccharides by an oligosaccharide-synthesizing enzyme immobilized on support media was documented.194 The selection of support media depends on the enzyme sources, enzyme-binding sites, and adsorption capacity of the support media. A better combination of support media and enzymes may lead to sustainable and economical synthesis of bioactive compounds from organic food waste.191–193 In the management of industrial waste, the use of cost-effective and adsorptive support surfaces, along with the reusability of immobilized ligninolytic enzymes, has been identified as an emerging and innovative technological approach.195 The immobilization process is advantageous because of the diverse tolerance of pH, temperature, and storage conditions of enzymes for enhanced and sustainable application for tackling food waste management.191,194 However, biodiesel production from the industrial food waste stream (oil, fat, and greases) is rendered by lipase. A previous study explored the potential of nanotechnology by utilizing lipase immobilization on carbon nanotubes for sustainable and continuous biodiesel synthesis from crude vegetable oil.196 In a pioneering investigation, the cost-effective and highly efficient catalytic potential of vegetable oil waste was observed in biodiesel production. This was achieved by immobilizing lipase on dendrimer-coated magnetic carbon nanotubes.197 Therefore, the immobilization of enzymes has become an attractive option over other conventional chemical and microbial approaches owing to their robustness, economic feasibility, and environmental sustainability for the potential management of food waste streams into valuable bioactive compounds Fig. 5.
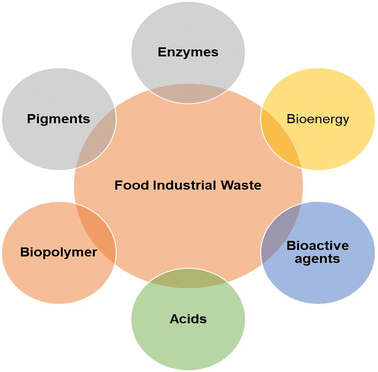 |
| Fig. 5 An overview of the industrial food waste valorization into value added products. | |
9. Industrial food waste valorization for tackling waste streams
In the food industry context, “food industrial wastes” most often refer to sections of leftovers produced as a result of food manufacturing processes. They are composed of a broad array of organic components that are discarded after production. Industrial food waste collected from diverse sectors, including fruit and vegetable processing, oil processing, paper manufacturing, and dairy processing industries, represents a potential substrate for the bioprocessing of a wide variety of products. These products include pigments, enzymes, acids, biofuels, bioactive agents, and biopolymers, with applications spanning the industrial and therapeutic domains.
A comprehensive list of industrial food waste valorization and the sustainable conversion of waste into wealth is listed in Table 3.
Table 3 The valorization of food waste, especially industrial food waste, to value-added products through microbial strains
Products categories |
Products |
Food industrial waste |
Microorganism |
Process |
Ref. |
Pigments |
Carotenoids |
Rice powder |
Bacillus clausii
|
Solid state fermentation |
197–203
|
Lycopene |
Soybean meal |
Rhodopseudomonas faecalis
|
Solid state fermentation |
Phycocyanin |
Sugarcane vinasse |
Aphanothece microscopic
|
— |
Prodigosin |
Cassava wastewater |
Serratia marcescens UCP 1549 |
— |
Red pigment |
Corn cob |
Monascus purpureus KACC 42430 |
Solid state fermentation |
Lycopene |
Tomato peel and seeds |
— |
Supercritical CO2 extraction |
Enzymes |
Cellulase |
Lignocellulosic biomass |
Pleurotus ostreatus
|
Solid state fermentation |
203–206
|
Pectinase |
Lemon peel pomace |
Aspergillus niger
|
Solid state fermentation |
Protease |
Pulse flour, corn, vegetable pills, gram husk |
Nocardiopsis alba OM-4 |
Solid state fermentation |
Lipase |
Rape seed oil |
Aspergillus sp. DPUA 1727 |
Solid state fermentation |
Xylanase |
Liquefied wheat bran |
Aspergillus niger
|
Submerged fermentation |
Bioactive agents |
Phenolic compounds |
Pineapple waste |
Kluyveromyces marxiansus NRRL Y-8281 |
Solid state fermentation |
207–211
|
Phenolic compounds |
Fig by-products using |
Aspergillus niger HT4 |
Solid state fermentation |
Gallic acid and ferulic acid |
Rice bran |
Rizhopus oryzae
|
Solid state fermentation |
Caffeic acid, naringenin, daidzein |
Soybean meals |
— |
Microwave-assisted extraction |
Ellagitannins, punicalagin, and punicalin |
Pomegranate peels |
— |
Ultrasound-assisted extraction |
Biopolymer |
Chitosan |
Corn steep liquor |
Rhizopus arrhizus UCP 402 |
Fermentation |
212
|
Pectin |
Jackfruit peel |
— |
Sonication microwave synergistic extraction |
213
|
Acids |
Lactic acid |
Mango peels |
Lactobacillus casei
|
Hydrolyzed steam explosion |
214
|
Propionic acid |
Cheese whey |
Propionibacterium acidipropionici
|
— |
215
|
Fumaric acid |
Food waste |
Rhizopus oryzae
|
Hydrolyzed |
216
|
Bioenergy |
Biodiesel |
Waste cooking olive oil |
Aspergillus niger
|
Fermentation |
213–216
|
Bioethanol |
Sugarcane bagasse |
Saccharomyces cerevisiae
|
Solid state fermentation |
Bioethanol |
Rice straw |
Saccharomyces cerevisiae
|
Fermentation |
10. Future opportunities and challenges
Sustainable management of food waste (FW), including horticultural waste (HW) and domestic kitchen waste, through bioconversion and biorefineries, has emerged as a potential technique. However, according to previous studies, several research uncertainties still exist as barriers to the sustainable conversion of FW into value-added products. Establishing an industrial biorefinery for the bioconversion of food waste into valuable compounds is a major challenge related to sustainability, cost-effectiveness, environmental considerations, and technological obstacles. The design and implementation of industrial biorefinery plants and bioreactors are currently in the early stages of research and development. Alternatively, several pilot experiments are ongoing, but a cost–benefit analysis has not yet been performed. As a result, pilot-phase biorefineries have been identified as potential alternatives for sustainable handling of food waste. However, assurance of industrial and commercial sustainability has not yet been confirmed. Therefore, the integration of a comprehensive biorefinery could offer a comprehensive solution to address the current challenges associated with the sustainable management of hazardous waste (HW), utilizing biorefinery and circular economic principles. Conversely, previous research has documented several emerging extraction technologies and methodologies designed to extract valuable products from both industrial and household food waste and waste streams, holding potential for value-added applications.
However, green extraction approaches and sustainable integrated biorefineries have yet to be investigated. Therefore, meticulous adaptive and pilot studies must be conducted to establish a sustainable and effective biorefinery for extracting value-added products and bioenergy through a circular economy using fruit and vegetable wastes.
In contrast, food waste recycling and management were not separated but included municipal waste management in developing countries.49,213 Thus, there is scope for waste separation and further valorization of food waste in developing countries. In particular, kitchen and industrial wastes are poorly managed because of insufficient technical knowledge and research data. Poor food waste management can create several environmental pollutants, including greenhouse gases and toxic environmental contaminants (e.g., toxic metals and pesticides). Although the thermal processing of FW (incineration) and biochemical processing (anaerobic digestion) is a well-adopted valorization or management process to tackle industrial and domestic food waste, the sustainable and green extraction of food waste for bioactive molecules is still in the pilot phase. Thus, establishing integrated and sustainable valorization approaches, including biorefinery, is a promising option for recycling and managing industrial and domestic food waste. The following recommendations were suggested for the effective and sustainable management of global food waste (Fig. 6):
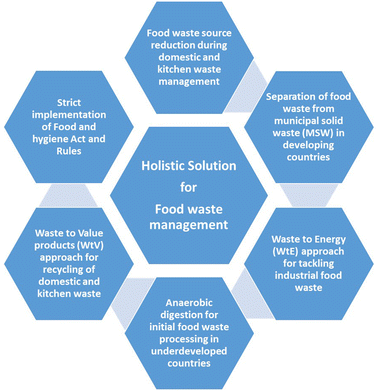 |
| Fig. 6 A policy advocacy and holistic recommendation concerning further opportunities regarding food waste management including advanced valorization and strict enforcement of rules and regulations. | |
(1) Minimizing waste generation during kitchen food processing and domestic use can be achieved through source reduction. This approach designates unavoidable waste materials, including potato and carrot peels, citrus rinds, and pomace fruits, as food waste. Consequently, a substantial portion of the food waste generated during food processing and cooking can be effectively managed through source reduction practices.
(2) Separating food waste from municipal solid waste is a vital step, particularly in populous nations, where food waste is often disposed of together with other waste. This practice is essential for efficient food waste management in unplanned disposal settings, which are common in various Asian and African countries.
(3) Recent years have seen the adoption of advanced and sustainable approaches, broadly categorized as (a) Waste to Energy (WtE) and (b) Waste to Value product (WtV). These multifaceted processes aim to recycle and convert food waste into valuable resources and present viable options for exploration on a global scale, including the local food industry.
(4) Finally, anaerobic digestion should be implemented to tackle the bulk of unavoidable food waste on the municipal pilot and industrial scales via the strict enforcement of global acts and rules concerning food waste recycling and sustainable management.
11. Conclusion
In summary, food waste valorization (FWV) is a promising sustainable approach for addressing global food waste and its conversion into value-added products. Food waste (FW) is generally derived from domestic or industrial waste streams. Despite the valuable sources of bioactive molecules, food waste (FW) and waste streams have been underexplored for sustainable conversion owing to a lack of awareness and comprehensive research efforts. Although developed countries and giant food companies are concerned about sustainable recycling and converting FW into wealth through various contemporary valorization approaches, FWV is limited in global scale and small-scale food industries. Therefore, a large portion of FW remains untouched and can be dumped with municipal solid waste. As a result, FW has become a global concern because of the unplanned management of other solid waste. The inherent feature of FW is mediated by anaerobic digestion, which leads to odorous waste instead of the sustainable conversion from waste to wealth. In this critical appraisal, we suggested a hypothetical waste pyramid to scrutinize the most preferable options for the sustainable conversion of global food waste. The integrated biorefinery is a promising option for industrial food waste management. Domestic and kitchen wastes should be managed separately with the initiative of waste separation from municipal solid waste. Traditionally, food waste has not been studied because of its potential as a resource recovery feedstock. However, several studies have suggested that diversified food waste is a potential, yet untapped source of bioactive molecules and bioenergy. Thus, an advanced valorization process can extract or convert value products from food waste. The integration of life cycle assessment (LCA) and hydrothermal conversion (HTC) plays a vital role in optimizing the overall output from waste streams. Advanced research is also in the initial stages of pilot experiments. Thus, further combined and adaptive research and scale-up of existing pilot studies should be explored toward meeting the UN's sustainable development goals (SDGs). Finally, the adoption of an advanced and sustainable valorization approach can significantly contribute to promoting a circular bioeconomy, effectively addressing the global macro issue of food waste.
Author contributions
Conceptualization; writing the draft: Aniruddha Sarker, Raju Ahmmed; writing and reviewing, data curation: S. M. Ahsan, Juwel Rana, Mithun Kumar Ghosh, and Rakhi Nandi.
Conflicts of interest
“There are no conflicts to declare”.
Acknowledgements
The lead authors are grateful to all contributing authors for their support and cooperation.
References
-
J. Gustavsson, C. Cederberg, U. Sonesson, R. Van Otterdijk, and A. Meybeck, Global Food Losses and Food Waste – Extent, Causes and Prevention, Food and Agriculture Organization of the United Nations, Rome, 2011 Search PubMed.
- M. F. Bellemare, M. Çakir, H. H. Peterson, L. Novak and J. Rudi, On the measurement of food waste, Philos. Trans. R. Soc., B, 2017, 365(1554), 3065–3081, DOI:10.1093/ajae/aax034.
- J. Parfitt, M. Barthel and S. Macnaughton, Food waste within food supply chains: quantification and potential for change to 2050, Philos. Trans. R. Soc., B, 2010, 365, 3065–3081, DOI:10.1098/rstb.2010.0126.
- C. M. Ajila, M. Aalami, K. Leelavathi and U. J. S. Prasada Rao, Mango peel powder: A potential source of antioxidant and dietary fiber in macaroni preparations, Innovative Food Sci. Emerging Technol., 2010, 11(1), 219–224, DOI:10.1016/j.ifset.2009.10.004.
- P. Thiviya, A. Gamage, R. Kapilan, O. Merah and T. Madhujith, Single cell protein production using different fruit waste: a review, Separations, 2022, 9(7), 178, DOI:10.3390/separations9070178.
- A. Nayak and B. Bhushan, An overview of the recent trends on the waste valorization techniques for food wastes, J. Environ. Manage., 2019, 233, 352–370, DOI:10.1016/j.jenvman.2018.12.041.
-
S. D. Sadhu, M. Garg, and A. Kumar, Major Environmental Issues and New Materials, in New Polymer Nanocomposites for Environmental Remediation, Elsevier, 2018, pp. 77–97, DOI:10.1016/B978-0-12-811033-1.00004-4.
- E. M. Alexandre, S. A. Moreira, L. M. Castro, M. Pintado and J. A. Saraiva, Emerging technologies to extract high added value compounds from fruit residues: Sub/supercritical, ultrasound-, and enzyme-assisted extractions, Food Rev. Int., 2018, 34(6), 581–612, DOI:10.1080/87559129.2017.1359842.
- J. Azmir, I. S. M. Zaidul, M. M. Rahman, K. M. Sharif, A. Mohamed, F. Sahena, M. H. A. Jahurul, K. Ghafoor, N. A. N. Norulaini and A. K. M. Omar, Techniques for extraction of bioactive compounds from plant materials: A review, J. Food Eng., 2013, 117(4), 426–436, DOI:10.1016/j.jfoodeng.2013.01.014.
- A. K. Jha and N. Sit, Extraction of bioactive compounds from plant materials using a combination of various novel methods: A review, Trends Food Sci. Technol., 2022, 119, 579–591, DOI:10.1016/j.tifs.2021.11.019.
- A. Saini, P. S. Panesar and M. B. Bera, Valorization of fruits and vegetables waste through green extraction of bioactive compounds and their nanoemulsions-based delivery system, Bioresources and Bioprocessing, 2019, 6(1), 1–12, DOI:10.1186/s40643-019-0261-9.
- G. Garcia-Garcia, J. Stone and S. Rahimifard, Opportunities for waste valorisation in the food industry–A case study with four UK food manufacturers, J. Clean. Prod., 2019, 211, 1339–1356, DOI:10.1016/j.jclepro.2018.11.269.
- R. Sindhu, E. Gnansounou, S. Rebello, P. Binod, S. Varjani, I. S. Thakur, R. B. Nair and A. Pandey, Conversion of food and kitchen waste to value-added products, J. Environ. Manage., 2019, 241, 619–630, DOI:10.1016/j.jenvman.2019.02.053.
- H. S. Ng, P. E. Kee, H. S. Yim, P. T. Chen, Y. H. Wei and J. C. W. Lan, Recent advances on the sustainable approaches for conversion and reutilization of food wastes to valuable bioproducts, Bioresour. Technol., 2020, 302, 122889, DOI:10.1016/j.biortech.2020.122889.
- N. Mahato, K. Sharma, M. Sinha and M. H. Cho, Citrus waste derived nutra-/pharmaceuticals for health benefits: Current trends and future perspectives, J. Funct. Foods, 2018, 40, 307–316, DOI:10.1016/j.jff.2017.11.015.
- B. Prakash, A. Kujur, P. P. Singh, A. Kumar and A. Yadav, Plants-derived bioactive compounds as functional food ingredients
and food preservative, J. Nutr. Food Sci., 2017, 1, 4 Search PubMed.
- A. Baiano, Recovery of biomolecules from food wastes—A review, Molecules, 2014, 19(9), 14821–14842, DOI:10.3390/molecules190914821.
- H. Bagherian, F. Z. Ashtiani, A. Fouladitajar and M. Mohtashamy, Comparisons between conventional, microwave-and ultrasound-assisted methods for extraction of pectin from grapefruit, Chem. Eng. Process., 2011, 50(11–12), 1237–1243, DOI:10.1016/j.cep.2011.08.002.
- O. Gligor, A. Mocan, C. Moldovan, M. Locatelli, G. Crişan and I. C. Ferreira, Enzyme-assisted extractions of polyphenols–A comprehensive review, Trends Food Sci. Technol., 2019, 88, 302–315, DOI:10.1016/j.tifs.2019.03.029.
- K. Kumar, S. Srivastav and V. S. Sharanagat, Ultrasound assisted extraction (UAE) of bioactive compounds from fruit and vegetable processing by-products: A review, Ultrason. Sonochem., 2021, 70, 105325, DOI:10.1016/j.ultsonch.2020.105325.
- H. Cai, S. You, Z. Xu, Z. Li, J. Guo, Z. Ren and C. Fu, Novel extraction methods and potential applications of polyphenols in fruit waste: a review, J. Food Meas. Charact., 2021, 15, 3250–3261, DOI:10.1007/s11694-021-00901-1.
- J. Singh and I. Ordoñez, Resource recovery from post-consumer waste: important lessons for the upcoming circular economy, J. Clean. Prod., 2016, 134, 342–353, DOI:10.1016/j.jclepro.2015.12.020.
- R. Kirkman and N. Voulvoulis, The role of public communication in decision making for waste management infrastructure, J. Environ. Manage., 2017, 203, 640–647, DOI:10.1016/j.jenvman.2016.06.002.
- R. E. Marshall and K. Farahbakhsh, Systems approaches to integrated solid waste management in developing countries, Waste Manage., 2013, 33(4), 988–1003, DOI:10.1016/j.wasman.2012.12.023.
-
D. C. Wilson, L. Rodic, P. Modak, R. Soos, A. Carpintero, K. Velis, M. Iyer, and O. Simonett, Global Waste Management Outlook, UNEP, 2015 Search PubMed.
- Y. Geng, J. Fu, J. Sarkis and B. Xue, Towards a national circular economy indicator system in China: an evaluation and critical analysis, J. Clean. Prod., 2012, 23(1), 216–224, DOI:10.1016/j.jclepro.2011.07.005.
- K. Ravindra, K. Kaur and S. Mor, System analysis of municipal solid waste management in Chandigarh and minimization practices for cleaner emissions, J. Clean. Prod., 2015, 89, 251–256, DOI:10.1016/j.jclepro.2014.10.036.
- M. Ilić and M. Nikolić, Drivers for development of circular economy–A case study of Serbia, Habitat Int., 2016, 56, 191–200, DOI:10.1016/j.habitatint.2016.06.003.
- E. C. Rada, L. A. Istrate, V. Panaitescu, M. Ragazzi, T. M. Cirlioru and T. Apostol, A comparison between different scenarios of Romanian municipal solid waste treatment before landfilling, Environ. Eng. Manage. J., 2010, 9(4), 589–596 CrossRef CAS.
- G. Ionescu, E. C. Rada and L. I. Cioca, Municipal solid waste sorting and treatment schemes for the maximization of material and energy recovery in a latest eu member, Environ. Eng. Manage. J., 2015, 14(11), 2537–2544 CrossRef.
- K. H. Esbensen and C. Velis, Transition to circular economy requires reliable statistical quantification and control of uncertainty and variability in waste, Waste Manage. Res., 2016, 34(12), 1197–1200, DOI:10.1177/0734242X16680911.
- P. R. More, A. R. Jambrak and S. S. Arya, Green, environment-friendly and sustainable techniques for extraction of food bioactive compounds and waste valorization, Trends Food Sci. Technol., 2022, 128, 296–315, DOI:10.1016/j.tifs.2022.08.016.
- Q. Jin, L. Yang, N. Poe and H. Huang, Integrated processing of plant-derived waste to produce value-added products based on the biorefinery concept, Trends Food Sci. Technol., 2018, 74, 119–131, DOI:10.1016/j.tifs.2018.02.014.
- D. A. Teigiserova, L. Hamelin and M. Thomsen, Towards transparent valorization of food surplus, waste and loss: Clarifying definitions, food waste hierarchy, and role in the circular economy, Sci. Total Environ., 2020, 706, DOI:10.1016/j.scitotenv.2019.136033.
-
IFPRI, Global Food Policy Report, Washington, DC, 2016 Search PubMed.
- H. Hartikainen, L. Mogensen, E. Svanes and U. Franke, Food waste quantification in primary production – the Nordic countries as a case study, Waste Manage., 2018, 71, 502–511, DOI:10.1016/j.wasman.2017.10.026.
-
United Nations Environment Programme, https://wedocs.unep.org/20.500.11822/25194, accessed 10 August, 2023.
- C. Chen, A. Chaudhary and A. Mathys, Nutritional and environmental losses embedded in global food waste, Resour., Conserv. Recycl., 2020, 160, 104912, DOI:10.1016/j.resconrec.2020.104912.
- M. Springmann, K. Wiebe, D. Mason-D'Croz, T. B. Sulser, M. Rayner and P. Scarborough, Health and nutritional aspects of sustainable diet strategies and their association with environmental impacts: a global modelling analysis with country-level detail, Lancet Planet. Health, 2018, 2, e451–e461, DOI:10.1016/S2542-5196(18)30206-7.
- W. Willett, J. Rockström, B. Loken, M. Springmann, T. Lang, S. Vermeulen and C. J Murray, Food in the Anthropocene: the EAT–Lancet Commission on healthy diets from sustainable food systems, Lancet, 2019, 393, 447–492, DOI:10.1016/S0140-6736(18)31788-4.
- B. Grizzetti, U. Pretato, L. Lassaletta, G. Billen and J. Garnier, The contribution of food waste to global and European nitrogen pollution, Environ. Sci. Policy, 2013, 33, 186–195, DOI:10.1016/j.envsci.2013.05.013.
- L. Ma, Z. Bai, W. Ma, M. Guo, R. Jiang, J. Liu, O. Oenema, G. L. Velthof, A. P. Whitmore, J. Crawford, A. Dobermann, M. Schwoob and F. Zhang, Exploring future food provision scenarios for China, Environ. Sci. Technol., 2019, 53, 1385–1393, DOI:10.1021/acs.est.8b04375.
- A. Usubiaga, I. Butnar and P. Schepelmann, Wasting food, wasting resources: potential environmental savings through food waste reductions, J. Ind. Ecol., 2018, 22, 574–584, DOI:10.1111/jiec.12695.
- S. Jribi, H. Ben Ismail, D. Doggui and H. Debbabi, COVID-19 virus outbreak lockdown: What impacts on household food wastage?, Environ. Dev. Sustain., 2020, 22(5), 3939–3955, DOI:10.1007/s10668-020-00740-y.
-
S. Otles, S. Despoudi, and C. Bucatariu and C. Kartal, Food Waste Recovery: Processing Technologies and Industrial Techniques, Elsevier, 2015, DOI:10.1016/B978-0-12-800351-0.00001-8.
- H. S. Ng, P. E. Kee, H. S. Yim, P. T. Chen, Y. H. Wei and J. Chi-Wei Lan, Recent advances on the sustainable approaches for conversion and reutilization of food wastes to valuable bioproducts, Bioresour. Technol., 2020, 302, DOI:10.1016/j.biortech.2020.122889.
-
R. Sindhu, P. Binod, R. B. Nair, S. Varjani, A. Pandey, and E. Gnansounou, Current Developments in Biotechnology and Bioengineering: Resource Recovery from Wastes, Elsevier, 2020, DOI:10.1016/B978-0-444-64321-6.00009-4.
- M. Sharma, Z. Usmani, V. K. Gupta and R. Bhat, Valorization of fruits and vegetable wastes and by-products to produce natural pigments, Crit. Rev. Biotechnol., 2021, 41(4), 535–563, DOI:10.1080/07388551.2021.1873240.
- A. Sarker, J. E. Kim, A. R. M. T. Islam, M. Bilal, M. R. J. Rakib, R. Nandi, M. M. Rahman and T. Islam, Heavy metals contamination and associated health risks in food webs—a review focuses on food safety and environmental sustainability in Bangladesh, Environ. Sci. Pollut. Res., 2022, 29, 3230–3245, DOI:10.1007/s11356-021-17153-7.
-
M. M. H. Tipu, A. Baroi, J. Rana, S. Islam, R. Jahan, M. S. Miah and M. Asaduzzaman, Potential Applications of Nanotechnology in Agriculture: A Smart Tool for Sustainable Agriculture, in Agricultural Development in Asia: Potential Use of Nano-Materials and Nano-Technology, IntechOpen, 2021, DOI:10.5772/intechpen.78083.
-
G. N. Tanjina Hasnat, Sources and Impacts of Emerging Contaminants in Agroecosystems, Sustainable Agriculture Reviews 50: Emerging Contaminants in Agriculture, Springer, Cham, 2021, DOI:10.1007/978-3-030-63249-6_1.
- A. Sarker, T. Islam, S. Rahman, R. Nandi and J. E. Kim, Uncertainty of pesticides in foodstuffs, associated environmental and health risks to humans—a critical case of Bangladesh with respect to global food policy, Environ. Sci. Pollut. Res., 2021, 28, 54448–54465, DOI:10.1007/s11356-021-16042-3.
- A. Sarker, R. Nandi, J. E. Kim and T. Islam, Remediation of chemical pesticides from contaminated sites through potential microorganisms and their functional enzymes: Prospects and challenges, Environ. Technol. Innovation, 2021, 23, 101777, DOI:10.1016/j.eti.2021.101777.
-
V. K. Singh, R. Singh, and E. Lichtfouse, Sustainable Agriculture Reviews 50: Emerging Contaminants in Agriculture, Springer Nature, 2021, DOI:10.1016/j.snb.2014.02.016.
- O. Alshaarawy, H. Elbaz and M. Andrew, The association of urinary polycyclic aromatic hydrocarbon biomarkers and cardiovascular disease in the US population, Environ. Int., 2016, 89(90), 174–178, DOI:10.1016/j.envint.2016.02.006.
- Y. Cao, B. Yang, Z. Song, H. Wang, F. He and X. Han, Wheat straw biochar amendments on the removal of polycyclic aromatic hydrocarbons (PAHs) in contaminated soil, Ecotoxicol. Environ. Saf., 2016, 130, 248–255, DOI:10.1016/j.ecoenv.2016.04.033.
- A. White, P. Bradshaw, A. Herring, S. Teitelbaum, J. Beyea and S. Stellman, Exposure to multiple sources of polycyclic aromatic hydrocarbons and breast cancer incidence, Environ. Int., 2016, 89–90, 185–192, DOI:10.1016/j.envint.2016.02.009.
- T. Hu, J. Zhang, C. Ye, L. Zhang, X. Xing, Y. Zhang and Q. Zhang, Status, source and health risk assessment of polycyclic aromatic hydrocarbons (PAHs) in soil from the water-level-fluctuation zone of the Three Gorges Reservoir, J. Geochem. Explor., 2017, 172, 20–28, DOI:10.1016/j.gexplo.2016.09.012.
- D. Dey and M. K. Gupta, Use of essential oils for insect pest management-a review, Innovative Farming, 2016, 1(2), 21–29 Search PubMed.
-
K. Lah, Effects of pesticides on human health, in Toxipedia, 2011, http://www.toxipedia.org/display/toxipedia/Effects+of+Pesticides+on+Human+Health Search PubMed.
- S. Khalid, M. Shahid, B. Murtaza, I. Bibi, M. Natasha and N. K. Niazi, A critical review of different factors governing the fate of pesticides in soil under biochar application, Sci. Total Environ., 2020, 711, 134645, DOI:10.1016/j.scitotenv.2019.134645.
- V. Yadav, A. Sarker, A. Yadav, A. O. Miftah, M. Bilal and H. M. N. Iqbal, Integrated biorefinery approach to valorize citrus waste: A sustainable solution for resource recovery and environmental management, Chemosphere, 2022, 293, 133459, DOI:10.1016/j.chemosphere.2021.133459.
- A. Sarker, M. A. Al Masud, D. M. Deepo, K. Das, R. Nandi, M. W. R. Ansary, A. R. M. T. Islam and T. Islam, Biological and green remediation of heavy metal contaminated water and soils: A state-of-the-art review, Chemosphere, 2023, 332, 138861, DOI:10.1016/j.chemosphere.2023.138861.
- A. Sarker, D. M. Deepo, R. Nandi, J. Rana, S. Islam, S. Rahman, M. N. Hossain, M. S. Islam, A. Baroi and J. E. Kim, A review of microplastics pollution in the soil and terrestrial ecosystems: A global and Bangladesh perspective, Sci. Total Environ., 2020, 733, 139296, DOI:10.1016/j.scitotenv.2020.139296.
- M. R. J. Rakib, A. Sarker, K. Ram, M. G. Uddin, T. R. Walker, T. Chowdhury, J. Uddin, M. U. Khandaker, M. M. Rahman and A. M. Idris, Microplastic Toxicity in Aquatic Organisms and Aquatic Ecosystems: a Review, Water, Air, Soil Pollut., 2023, 234, 52, DOI:10.1007/s11270-023-06062-9.
-
J. Kaur, Sustainable Agriculture Reviews 50: Emerging Contaminants in Agriculture, ed. V. Kumar Singh, R. Singh and E. Lichtfouse, Springer, Cham, vol. 50, 2021, pp. 133–176, DOI:10.1007/978-3-030-63249-6_6.
- M. S. Islam, K. Phoungthong, A. R. M. T. Islam, M. M. Ali, A. Sarker, M. H. Kabir and A. M. Idris, Present status and mitigation approaches of arsenic in the environment of Bangladesh: A critical review, Int. J. Environ. Sci. Technol., 2023, 20, 13883–13894, DOI:10.1007/s13762-023-04956-z.
- A. S. Sharma, S. Bhatia, A. Verma, S. Soni and N. Batra, Insights on sustainable approaches for production and applications of value-added products, Chemosphere, 2021, 286, 131623, DOI:10.1016/j.chemosphere.2021.131623.
- S. Christiaens, D. Uwibambe, M. Uyttebroek, B. Droogenbroeck, A. M. Van Loey and M. E. Hendrickx, Pectin characterisation in vegetable waste streams: A starting point for waste valorisation in the food industry, LWT–Food Sci. Technol., 2015, 61(2), 275–282, DOI:10.1016/j.lwt.2014.12.054.
- R. Redcorn, S. Fatemi and A. S. Engelberth, Comparing End-Use Potential for Industrial Food-Waste Sources, Engineering, 2018, 4(3), 371–380, DOI:10.1016/j.eng.2018.05.010.
- A. Badgett and A. Milbrandt, A summary of standards and practices for wet waste streams used in waste-to-energy technologies in the United States, Renewable Sustainable Energy Rev., 2020, 117, 109425, DOI:10.1016/j.rser.2019.109425.
- S. Ranganathan, S. Dutta, J. A. Moses and C. Anandharamakrishnan, Utilization of food waste streams for the production of biopolymers, Heliyon, 2020, 6, e04891, DOI:10.1016/j.heliyon.2020.e04891.
- I. A. Udugama, L. A. H. Petersen, F. C. Falco, H. Junicke, A. Mitic, X. F. Alsina, S. S. Mansouri and K. V. Gernaey, Resource recovery from waste streams in a water-energy-food nexus perspective: Toward more sustainable food processing, Food Bioprocess Technol., 2020, 119, 133–147, DOI:10.1016/j.fbp.2019.10.014.
- M. Bilal and H. M. N. Iqbal, Sustainable bioconversion of food waste into high-value products by immobilized enzymes to meet bio-economy challenges and opportunities – A review, Food Res. Int., 2019, 123, 226–240, DOI:10.1016/j.foodres.2019.04.066.
- P. Loizia, N. Neofytou and A. A. Zorpas, The concept of circular economy strategy in food waste management for the optimization of energy production through anaerobic digestion, Environ. Sci. Pollut. Res., 2019, 26(15), 14766–14773, DOI:10.1007/s11356-018-3519-4.
- J. C. M. Barreira, A. A. Arraibi and I. C. F. R. Ferreira, Bioactive and functional compounds in apple pomace from juice and cider manufacturing: Potential use in dermal formulations, Trends Food Sci. Technol., 2019, 90, 76–87, DOI:10.1016/j.tifs.2019.05.014.
- M. de Andrade Lima, D. Charalampopoulos and A. Chatzifragkou, Optimisation and modelling of supercritical CO2 extraction process of carotenoids from carrot peels, J. Supercrit. Fluids, 2018, 133, 94–102, DOI:10.1016/j.supflu.2017.09.028.
- A. M. I. Encalada, C. D. Pérez, S. K. Flores, L. Rossetti, E. N. Fissore and A. M. Rojas, Antioxidant pectin enriched fractions obtained from discarded carrots (Daucus carota L.) by ultrasound-enzyme assisted extraction, Food Chem., 2019, 289, 453–460, DOI:10.1016/j.foodchem.2019.03.078.
- F. O. Ogutu and T.-H. Mu, Ultrasonic degradation of sweet potato pectin and its antioxidant activity, Ultrason. Sonochem., 2017, 38, 726–734, DOI:10.1016/j.ultsonch.2016.08.014.
- T. K. Varun, S. Senani, N. Jayapal, J. Chikkerur, S. Roy, V. B. Tekulapally, M. Gautam and N. Kumar, Extraction of chitosan and its oligomers from shrimp shell waste, their characterization and antimicrobial effect, Vet. World, 2017, 10(2), 170–175, DOI:10.14202/vetworld.2017.170-175.
- D. Barba, F. Beolchini, D. Cifoni and F. Veglió, Whey protein concentrate production in a pilot scale two-stage diafiltration process, Sep. Sci. Technol., 2001, 36(4), 587–603, DOI:10.1081/SS-100102948.
- M. Masmoudi, S. Besbes, M. Chaabouni, C. Robert, M. Paquot, C. Blecker and H. Attia, Optimization of pectin extraction from lemon by-product with acidified date juice using response surface methodology, Carbohydr. Polym., 2008, 74(2), 185–192, DOI:10.1016/j.carbpol.2008.02.003.
- P. Niemi, C. B. Faulds, J. Sibakov, U. Holopainen, K. Poutanen and J. Buchert, Effect of a milling pre-treatment on the enzymatic hydrolysis of carbohydrates in brewer's spent grain, Bioresour. Technol., 2012, 116, 155–160, DOI:10.1016/j.biortech.2012.04.043.
- X. Wang, Q. Chen and X. Lü, Pectin extracted from apple pomace and citrus peel by subcritical water, Food Hydrocolloids, 2014, 38, 129–137, DOI:10.1016/j.foodhyd.2013.12.003.
- C. Hernández, J. Ascacio-Valdés, H. De la Garza, J. Wong-Paz, C. N. Aguilar, G. C. Martínez-Ávila, C. Castro-López and A. Aguilera-Carbó, Polyphenolic content, in vitro antioxidant activity and chemical composition of extract from Nephelium lappaceum L. (Mexican rambutan) husk, Asian Pac. J. Trop. Med., 2017, 10(12), 1201–1205, DOI:10.1016/j.apjtm.2017.10.030.
- F. J. Herraiz, M. D. Raigón, S. Vilanova, M. D. García-Martínez, P. Gramazio, M. Plazas, A. Rodríguez-Burruezo and J. Prohens, Fruit composition diversity in land races and modern pepino (Solanum muricatum) varieties and wild related species, Food Chem., 2016, 203, 49–58, DOI:10.1016/j.foodchem.2016.02.035.
- N. Mahato, K. Sharma, M. Sinha and M. H. Cho, Citrus waste derived nutra-/pharmaceuticals for health benefits: Current trends and future perspectives, J. Funct. Foods, 2018, 40, 307–316, DOI:10.1016/j.jff.2017.11.015.
- M. Sójka, K. Kołodziejczyk, J. Milala, M. Abadias, I. Viñas, S. Guyot and A. Baron, Composition and properties of the polyphenolic extracts obtained from industrial plum pomaces, J. Funct. Foods, 2015, 12, 168–178, DOI:10.1016/j.jff.2014.11.015.
- C. Torres-León, R. Rojas, J. C. Contreras-Esquivel, L. Serna-Cock, R. E. Belmares-Cerda and C. N. Aguilar, Mango seed: Functional and nutritional properties, Trends Food Sci. Technol., 2016, 55, 109–117, DOI:10.1016/j.tifs.2016.06.009.
- H. T. Vu, C. J. Scarlett and Q. V. Vuong, Phenolic compounds within banana peel and their potential uses: A review, J. Funct. Foods, 2018, 40, 238–248, DOI:10.1016/j.jff.2017.11.006.
- H. F. B. Lasta, L. Lentz, N. Mezzomo and S. R. S. Ferreira, Supercritical CO2 to recover extracts enriched in antioxidant compounds from beetroot aerial parts, Biocatal. Agric. Biotechnol., 2019, 19, 101169, DOI:10.1016/j.bcab.2019.101169.
- P. H. Santos, D. H. Baggio Ribeiro, G. A. Micke, L. Vitali and H. Hense, Extraction of bioactive compounds from feijoa (Acca sellowiana (O. Berg) Burret) peel by low and high-pressure techniques, J. Supercrit. Fluids, 2019, 145, 219–227, DOI:10.1016/j.supflu.2018.12.016.
- M. Thomas, A. Badr, Y. Desjardins, A. Gosselin and P. Angers, Characterization of industrial broccoli discards (Brassica oleracea var. italica) for their glucosinolate, polyphenol and flavonoid contents using UPLC MS/MS and spectrophotometric methods, Food Chem., 2018, 245, 1204–1211, DOI:10.1016/j.foodchem.2017.11.021.
- J. J. Vulić, T. N. Ćebović, J. M. Čanadanović-Brunet, G. S. Ćetković, V. M. Čanadanović, S. M. Djilas and V. T. Tumbas Šaponjac,
In vivo and in vitro antioxidant effects of beetroot pomace extracts, J. Funct. Foods, 2014, 6, 168–175, DOI:10.1016/j.jff.2013.10.003.
- Z.-G. Wu, H.-Y. Xu, Q. Ma, Y. Cao, J.-N. Ma and C.-M. Ma, Isolation, identification and quantification of unsaturated fatty acids, amides, phenolic compounds and glycoalkaloids from potato peel, Food Chem., 2012, 135(4), 2425–2429, DOI:10.1016/j.foodchem.2012.07.019.
- D. Ballesteros-Vivas, G. Álvarez-Rivera, S. J. Morantes, A. del P. Sánchez-Camargo, E. Ibáñez, F. Parada-Alfonso and A. Cifuentes, An integrated approach for the valorization of mango seed kernel: Efficient extraction solvent selection, phytochemical profiling and antiproliferative activity assessment, Food Res. Int., 2019, 126, 108616, DOI:10.1016/j.foodres.2019.108616.
- L. Klavins, J. Kviesis, I. Nakurte and M. Klavins, Berry press residues as a valuable source of polyphenolics: Extraction optimisation and analysis, LWT, 2018, 93, 583–591, DOI:10.1016/j.lwt.2018.04.021.
- V. Lavelli and S. Corti, Phloridzin and other phytochemicals in apple pomace: Stability evaluation upon dehydration and storage of dried product, Food Chem., 2011, 129(4), 1578–1583, DOI:10.1016/j.foodchem.2011.06.011.
- E. J. Cho, L. T. P. Trinh, Y. Song, Y. G. Lee and H.-J. Bae, Bioconversion of biomass waste into high value chemicals, Bioresour. Technol., 2020, 298, 122386, DOI:10.1016/j.biortech.2019.122386.
- A. Sharma, V. Vivekanand and R. P. Singh, Solid-state fermentation for gluconic acid production from sugarcane molasses by Aspergillus niger ARNU-4 employing tea waste as the novel solid support, Bioresour. Technol., 2008, 99(9), 3444–3450, DOI:10.1016/j.biortech.2007.08.006.
- K. Piwowarek, E. Lipińska and E. Hać-Szymańczuk, Possibility of using apple pomaces in the process of propionic-acetic fermentation, Electron. J. Biotechnol., 2016, 23, 1–6, DOI:10.1016/j.ejbt.2016.07.004.
- R. K. Das, S. K. Brar and M. Verma, A fermentative approach towards optimizing directed biosynthesis of fumaric acid by Rhizopus oryzae 1526 utilizing apple industry waste biomass, Fungal Biol., 2015, 119(12), 1279–1290, DOI:10.1016/j.funbio.2015.10.001.
- W. Dessie, W. Zhang, F. Xin, W. Dong, M. Zhang, J. Ma and M. Jiang, Succinic acid production from fruit and vegetable wastes hydrolyzed by on-site enzyme mixtures through solid state fermentation, Bioresour. Technol., 2018, 247, 1177–1180, DOI:10.1016/j.biortech.2017.08.171.
- G. S. Dhillon, S. K. Brar, M. Verma and R. D. Tyagi, Apple pomace ultrafiltration sludge – A novel substrate for fungal bioproduction of citric acid: Optimisation studies, Food Chem., 2011, 128(4), 864–871, DOI:10.1016/j.foodchem.2011.03.107.
- A. Karthikeyan and N. Sivakumar, Citric acid production by Koji fermentation using banana peel as a novel substrate, Bioresour. Technol., 2010, 101(14), 5552–5556, DOI:10.1016/j.biortech.2010.02.063.
- M. Patsalou, K. K. Menikea, E. Makri, M. I. Vasquez, C. Drouza and M. Koutinas, Development of a citrus peel-based biorefinery strategy for the production of succinic acid, J. Clean. Prod., 2017, 166, 706–716, DOI:10.1016/j.jclepro.2017.08.039.
- P. Pal and J. Nayak, Development and analysis of a sustainable technology in manufacturing acetic acid and whey protein from waste cheese whey, J. Clean. Prod., 2016, 112, 59–70, DOI:10.1016/j.jclepro.2015.07.085.
- P. Niemi, T. Tamminen, A. Smeds, K. Viljanen, T. Ohra-aho, U. Holopainen-Mantila, C. B. Faulds, K. Poutanen and J. Buchert, Characterization of Lipids and Lignans in Brewer's Spent Grain and Its Enzymatically Extracted Fraction, J. Agric. Food Chem., 2012, 60(39), 9910–9917, DOI:10.1021/jf302684x.
- I. Dalmolin, M. A. Mazutti, E. A. C. Batista, M. A. A. Meireles and J. V. Oliveira, Chemical characterization and phase behaviour of grape seed oil in compressed carbon dioxide and ethanol as co-solvent, J. Chem. Thermodyn., 2010, 42(6), 797–801, DOI:10.1016/j.jct.2010.02.003.
-
S. A. Socaci, A. C. Farcas, D. C. Vodnar, and M. Tofana, Food Wastes as Valuable Sources of Bioactive Molecules, in Superfood and Functional Food – the Development of Superfoods and Their Roles as Medicine, InTech, 2017, DOI:10.5772/66115.
- N. Boussetta, J.-L. Lanoisellé, C. Bedel-Cloutour and E. Vorobiev, Extraction of soluble matter from grape pomace by high voltage electrical discharges for polyphenol recovery: Effect of sulphur dioxide and thermal treatments, J. Food Eng., 2009, 95(1), 192–198, DOI:10.1016/j.jfoodeng.2009.04.030.
- C. D. Pereira, O. Diaz and A. Cobos, Valorization of by-products from ovine cheese manufacture: clarification by thermocalcic precipitation/microfiltration before ultrafiltration, Int. Dairy J., 2002, 12(9), 773–783, DOI:10.1016/S0958-6946(02)00070-5.
- D.-S. Tang, Y.-J. Tian, Y.-Z. He, L. Li, S.-Q. Hu and B. Li, Optimisation of ultrasonic-assisted protein extraction from brewer's spent grain, Czech J. Food Sci., 2010, 28(1), 9–17, DOI:10.17221/178/2009-CJFS.
- E. Vieira, M. A. M. Rocha, E. Coelho, O. Pinho, J. A. Saraiva, I. M. P. L. V. O. Ferreira and M. A. Coimbra, Valuation of brewer's spent grain using a fully recyclable integrated process for extraction of proteins and arabinoxylans, Ind. Crops Prod., 2014, 52, 136–143, DOI:10.1016/j.indcrop.2013.10.012.
- J. Bustamante, S. van Stempvoort, M. García-Gallarreta, J. A. Houghton, H. K. Briers, V. L. Budarin, A. S. Matharu and J. H. Clark, Microwave assisted hydro-distillation of essential oils from wet citrus peel waste, J. Clean. Prod., 2016, 137, 598–605, DOI:10.1016/j.jclepro.2016.07.108.
- P. Tongnuanchan and S. Benjakul, Essential Oils: Extraction, Bioactivities, and Their Uses for Food Preservation, J. Food Sci., 2014, 79(7), R1231–R1249, DOI:10.1111/1750-3841.12492.
- I. Esparza, N. Jiménez-Moreno, F. Bimbela, C. Ancín-Azpilicueta and L. M. Gandía, Fruit and vegetable waste management: Conventional and emerging approaches, J. Environ. Manage., 2020, 265, DOI:10.1016/j.jenvman.2020.110510.
- K. Schanes, K. Dobernig and B. Gözet, Food waste matters – A systematic review of household food waste practices and their policy implications, J. Cleaner Prod., 2018, 182, 978–991, DOI:10.1016/j.jclepro.2018.02.030.
- N. Ferronato, E. C. Rada, M. A. Gorritty Portillo, L. I. Cioca, M. Ragazzi and V. Torretta, Introduction of the circular economy within developing regions: A comparative analysis of advantages and opportunities for waste valorization, J. Environ. Manage., 2019, 230, 366–378, DOI:10.1016/j.jenvman.2018.09.095.
- K. L. Ong, G. Kaur, N. Pensupa, K. Uisan and C. S. K. Lin, Trends in food waste valorization for the production of chemicals, materials and fuels: Case study South and Southeast Asia, Bioresour. Technol., 2018, 248, 100–112, DOI:10.1016/j.biortech.2017.06.076.
- C. Du, J. J. Abdullah, D. Greetham, D. Fu, M. Yu, L. Ren, S. Li and D. Lu, Valorization of food waste into biofertiliser and its field application, J. Cleaner Prod., 2018, 187, 273–284, DOI:10.1016/j.jclepro.2018.03.211.
- F. Kanani, M. D. Heidari, B. H. Gilroyed and N. Pelletier, Waste valorization technology options for the egg and broiler industries: A review and recommendations, J. Cleaner Prod., 2020, 262, DOI:10.1016/j.jclepro.2020.121129.
- M. Borrello, F. Caracciolo, A. Lombardi, S. Pascucci and L. Cembalo, Consumers’ perspective on circular economy strategy for reducing food waste, Sustainability, 2017, 9(1), 141, DOI:10.3390/su9010141.
- T. M. W. Mak, X. Xiong, D. C. W. Tsang, I. K. M. Yu and C. S. Poon, Sustainable food waste management towards circular bioeconomy: Policy review, limitations and opportunities, Bioresour. Technol., 2020, 297, DOI:10.1016/j.biortech.2019.122497.
- S. Dahiya, A. N. Kumar, J. Shanthi Sravan, S. Chatterjee, O. Sarkar and S. V. Mohan, Food waste biorefinery: Sustainable strategy for circular bioeconomy, Bioresour. Technol., 2018, 248, 2–12, DOI:10.1016/j.biortech.2017.07.176.
- K. S. Ganesh, A. Sridhar and S. Vishali, Utilization of fruit and vegetable waste to produce value-added products: Conventional utilization and emerging opportunities-A review, Chemosphere, 2021, 287, 132221, DOI:10.1016/j.chemosphere.2021.132221.
- D. A. Teigiserova, L. Hamelin and M. Thomsen, Review of high-value food waste and food residues biorefineries with focus on unavoidable wastes from processing, Resour., Conserv. Recycl., 2019, 149, 413–426, DOI:10.1016/j.resconrec.2019.05.003.
- A. S. Sodhi, N. Sharma, S. Bhatia, A. Verma, S. Soni and N. Batra, Insights on sustainable approaches for production and applications of value added products, Chemosphere, 2021, 286, 131623, DOI:10.1016/j.chemosphere.2021.131623.
- J. P. Trigo, E. M. C. Alexandre, J. A. Saraiva and M. E. Pintado, High value-added compounds from fruit and vegetable by-products–Characterization, bioactivities, and application in the development of novel food products, Crit. Rev. Food Sci. Nutr., 2020, 60, 1388–1416, DOI:10.1080/10408398.2019.1572588.
- M. Carmona-Cabello, I. L. Garcia, D. Leiva-Candia and M. P. Dorado, Valorization of food waste based on its composition through the concept of biorefinery, Curr. Opin. Green Sustainable Chem., 2018, 14, 67–79, DOI:10.1016/j.cogsc.2018.06.011.
- A. Patel, K. Hrůzová, U. Rova, P. Christakopoulos and L. Matsakas, Sustainable biorefinery concept for biofuel production through holistic volarization of food waste, Bioresour. Technol., 2019, 294, 122247, DOI:10.1016/j.biortech.2019.122247.
- S. Plazzotta, M. Cottes, P. Simeoni and L. Manzocco, Evaluating the environmental and economic impact of fruit and vegetable waste valorisation: The lettuce waste study-case, J. Cleaner Prod., 2020, 262, DOI:10.1016/j.jclepro.2020.121435.
- M. S. M. Basri, N. N. A. K. Shah, A. Sulaiman, I. S. M. A. Tawakkal, M. Z. M. Nor, S. H. Ariffin, N. H. A. Ghani and F. S. M. Salleh, Progress in the valorization of fruit and vegetable wastes: Active packaging, biocomposites, by-products, and innovative technologies used for bioactive compound extraction, Polymers, 2021, 13(20), 3503, DOI:10.3390/polym13203503.
- L. A. Pfaltzgraff, M. De Bruyn, E. C. Cooper, V. Budarin and J. H. Clark, Food waste biomass: A resource for high-value chemicals, Green Chem., 2013, 15, 307–314, 10.1039/c2gc36978h.
-
Bioactive potential of fruit and vegetable wastes, in Advances in Food and Nutrition Research, ed. V. Coman, B. E. Teleky, L. Mitrea, G. A. Martău, K. Szabo, L. F. Călinoiu and D. C. Vodnar, Academic Press, Elsevier, 2020, vol. 91, pp. 157–225, DOI:10.1016/bs.afnr.2019.07.001.
- C. Fritsch, A. Staebler, A. Happel, M. A. C. Márquez, I. Aguiló-Aguayo, M. Abadias, M. Gallur, I. M. Cigognini, A. Montanari, M. J. López, F. Suárez-Estrella, N. Brunton, E. Luengo, L. Sisti, M. Ferri and G. Belotti, Processing, valorization and application of bio-waste derived compounds from potato, tomato, olive and cereals: A review, Sustainability, 2017, 9(8), 1–46, DOI:10.3390/su9081492.
- A. Sridhar, M. Ponnuchamy, P. S. Kumar, A. Kapoor, D. V. N. Vo and S. Prabhakar, Techniques and modeling of polyphenol extraction from food: a review, Environ. Chem. Lett., 2021, 19, 3409–3443, DOI:10.1007/s10311-021-01217-8.
- R. E. Jones, R. E. Speight, J. L. Blinco and I. M. O'Hara, Biorefining within food loss and waste frameworks: A review, Renewable Sustainable Energy Rev., 2021, 154, 111781, DOI:10.1016/j.rser.2021.111781.
-
N. Abu Yazid, Proteases from Protein-Rich Waste: Production by SSF, Downstream, Immobilisation onto Nanoparticles and Application on Protein Hydrolysis, PhD Thesis, Autonomous University of Barcelona, 2017 Search PubMed.
- E. Uckun Kiran, A. P. Trzcinski and Y. Liu, Platform chemical production from food wastes using a biorefinery concept, J. Chem. Technol. Biotechnol., 2015, 90(8), 1364–1379, DOI:10.1002/jctb.4551.
- H. Kowalska, K. Czajkowska, J. Cichowska and A. Lenart, What's new in biopotential of fruit and vegetable by-products applied in the food processing industry, Trends Food Sci. Technol., 2017, 67, 150–159, DOI:10.1016/j.tifs.2017.06.016.
- N. Jimenez-Moreno, I. Esparza, F. Bimbela, L. M. Gandía and C. Ancín-Azpilicueta, Valorization of selected fruit and vegetable wastes as bioactive compounds: Opportunities and challenges, Crit. Rev. Environ. Sci. Technol., 2020, 50(20), 2061–2108, DOI:10.1080/10643389.2019.1694819.
-
D. Ramesh, I. K. Muniraj, K. Thangavelu and S. Karthikeyan, Chemicals and Fuels Production from Agro Residues: A Biorefinery Approach, in Sustainable Approaches for Biofuels Production Technologies, ed. Srivastava N., Srivastava M., Mishra P., Upadhyay S., Ramteke P., Gupta V., Biofuel and Biorefinery Technologies, 2019, vol 7, Springer, Cham, DOI:10.1007/978-3-319-94797-6_3.
- M. Demirkol and Z. Tarakci, Effect of grape (Vitis labrusca L.) pomace dried by different methods on physicochemical, microbiological and bioactive properties of yoghurt, Lwt, 2018, 97, 770–777, DOI:10.1016/j.lwt.2018.07.058.
- A. Padayachee, L. Day, K. Howell and M. J. Gidley, Complexity and health functionality of plant cell wall fibers from fruits and vegetables, Crit. Rev. Food Sci. Nutr., 2017, 57(1), 59–81, DOI:10.1080/10408398.2013.850652.
- C. M. G. C. Renard, Extraction of bioactives from fruit and vegetables: State of the art and perspectives, LWT, 2018, 93, 390–395, DOI:10.1016/j.lwt.2018.03.063.
- V. Mozhiarasi, Overview of pretreatment technologies on vegetable, fruit and flower market wastes disintegration and bioenergy potential: Indian scenario, Chemosphere, 2021, 288, 132604, DOI:10.1016/j.chemosphere.2021.132604.
- H. Lu, V. Yadav, M. Bilal and H. M. N. Iqbal, Bioprospecting microbial hosts to valorize lignocellulose biomass – Environmental perspectives and value-added bioproducts, Chemosphere, 2021, 288, 132574, DOI:10.1016/j.chemosphere.2021.132574.
- J. Paini, V. Benedetti, S. S. Ail, M. J. Castaldi, M. Baratieri and F. Patuzzi, Valorization of Wastes from the Food Production Industry: A Review Towards an Integrated Agri-Food Processing Biorefinery, Waste Biomass Valorization, 2021, 13, 31–50, DOI:10.1007/s12649-021-01467-1.
- M. Herrero and E. Ibanez, Green extraction processes, biorefineries and sustainability: Recovery of high added-value products from natural sources, J. Supercrit. Fluids, 2018, 134, 252–259, DOI:10.1016/j.supflu.2017.12.002.
- M. Zia, S. Ahmed and A. Kumar, Anaerobic digestion (AD) of fruit and vegetable market waste (FVMW): potential of FVMW, bioreactor performance, co-substrates, and pre-treatment techniques, Biomass Convers. Biorefin., 2020, 12, 3573–3592, DOI:10.1007/s13399-020-00979-5.
-
P. Majumder and H. V. Annegowda, Fruit and vegetable by-products: novel ingredients for a sustainable society, in Valorization of Agri-Food Wastes and By-Products, Elsevier, 2021, pp. 133–156, DOI:10.1016/B978-0-12-824044-1.00006-4.
- F. Zhu, B. Du, L. Zheng and J. Li, Advance on the bioactivity and potential applications of dietary fibre from grape pomace, Food Chem., 2015, 186, 207–212, DOI:10.1016/j.foodchem.2014.07.057.
- F. Shahidi and P. Ambigaipalan, Phenolics and polyphenolics in foods, beverages and spices: Antioxidant activity and health effects – A review, J. Funct. Foods, 2015, 18, 820–897, DOI:10.1016/j.jff.2015.06.018.
- G. Ferrentino, Md. Asaduzzaman and M. M. Scampicchio, Current
technologies and new insights for the recovery of high valuable compounds from fruits by-products, Crit. Rev. Food Sci. Nutr., 2016, 1, 19, DOI:10.1080/10408398.2016.1180589.
- S. Ben-Othman, I. Jõudu and R. Bhat, Bioactives from Agri-Food Wastes: Present Insights and Future Challenges, Molecules, 2020, 25(3), 510, DOI:10.3390/molecules25030510.
- K. S. Ganesh, A. Sridhar and S. Vishali, Utilization of fruit and vegetable waste to produce value-added products: Conventional utilization and emerging opportunities-A review, Chemosphere, 2022, 287, 132221, DOI:10.1016/j.chemosphere.2021.132221.
- A. S. Sodhi, N. Sharma, S. Bhatia, A. Verma, S. Soni and N. Batra, Insights on sustainable approaches for production and applications of value added products, Chemosphere, 2022, 286, 131623, DOI:10.1016/j.chemosphere.2021.131623.
- V. Mozhiarasi and T. S. Natarajan, Slaughterhouse and poultry wastes: management practices, feedstocks for renewable energy production, and recovery of value added products, Biomass Convers. Biorefin., 2022 DOI:10.1007/s13399-022-02352-0.
- K. Spalvins, L. Zihare and D. Blumberga, Single cell protein production from waste biomass: comparison of various industrial by-products, Energy Procedia, 2018, 147, 409–418, DOI:10.1016/j.egypro.2018.07.111.
- P. S. Panesar, S. Kumari and R. Panesar, Biotechnological approaches for the production of prebiotics and their potential applications, Crit. Rev. Biotechnol., 2013, 33(4), 345–364, DOI:10.3109/07388551.2012.709482.
- S. Ram Kumar Pandian, V. Deepak, K. Kalishwaralal, J. Muniyandi, N. Rameshkumar and S. Gurunathan, Synthesis of PHB nanoparticles from optimized medium utilizing dairy industrial waste using Brevibacterium casei SRKP2: A green chemistry approach, Colloids Surf., B, 2009, 74(1), 266–273, DOI:10.1016/j.colsurfb.2009.07.029.
- S. Ozmihci and F. Kargi, Kinetics of batch ethanol fermentation of cheese-whey powder (CWP) solution as function of substrate and yeast concentrations, Bioresour. Technol., 2007, 98(16), 2978–2984, DOI:10.1016/j.biortech.2006.10.005.
- J. Parrondo, L. A. Garcia and M. Diaz, Production of an Alcoholic Beverage by Fermentation of Whey Permeate with Kluyveromyces fragilis I: Primary Metabolism, J. Inst. Brew., 2000, 106(6), 367–375, DOI:10.1002/j.2050-0416.2000.tb00527.x.
- M. Mesomo, M. F. Silva, G. Boni, F. F. Padilha, M. Mazutti, A. Mossi, D. de Oliveira, R. L. Cansian, M. Di Luccio and H. Treichel, Xanthan gum produced by Xanthomonas campestris from cheese whey: production optimisation and rheological characterisation, J. Sci. Food Agric., 2009, 89(14), 2440–2445, DOI:10.1002/jsfa.3743.
- K. Jayathilakan, K. Sultana, K. Radhakrishna and A. S. Bawa, Utilization of byproducts and waste materials from meat, poultry and fish processing industries: a review, J. Food Sci. Technol., 2012, 49(3), 278–293, DOI:10.1007/s13197-011-0290-7.
-
A. Mohan and J. M. Long, Valorization of wastes and by-products from the meat industry, in Valorization of Agri-Food Wastes and By-Products, Elsevier, 2021, pp. 457–474, DOI:10.1016/B978-0-12-824044-1.00010-6.
- F. Toldrá, M.-C. Aristoy, L. Mora and M. Reig, Innovations in value-addition of edible meat by-products, Meat Sci., 2012, 92(3), 290–296, DOI:10.1016/j.meatsci.2012.04.004.
- J. E. JE, Isolation of angiotensin I converting enzyme (ACE) inhibitor from fermented oyster sauce, Crassostrea gigas, Food Chem., 2005, 90(4), 809–814, DOI:10.1016/j.foodchem.2004.05.028.
- W.-K. Jung, B.-J. Lee and S.-K. Kim, Fish-bone peptide increases calcium solubility and bioavailability in ovariectomised rats, Br. J. Nutr., 2006, 95(1), 124–128, DOI:10.1079/BJN20051615.
- S.-K. Kim and E. Mendis, Bioactive compounds from marine processing byproducts – A review, Food Res. Int., 2006, 39(4), 383–393, DOI:10.1016/j.foodres.2005.10.010.
-
S.-K. Kim and J. Venkatesan, Introduction to Seafood Processing By-products, in Seafood Processing By-Products, Springer, New York, 2014, pp. 1–9, DOI:10.1007/978-1-4614-9590-1_1.
-
P. V. Suresh, T. G. Kudre, and L. C. Johny, Sustainable Valorization of Seafood Processing By-Product/Discard, in Waste to Wealth. Energy, Environment, and Sustainability, ed. R. Singhania, R. Agarwal, R. Kumar and R. Sukumaran, Springer, Singapore, 2022, pp. 111–139, DOI:10.1007/978-981-10-7431-8_7.
- M. Eriksson and J. Spangberg, Carbon footprint and energy use of food waste management options for fresh fruit and vegetables from supermarkets, Waste Manage., 2017, 60, 786–799, DOI:10.1016/j.wasman.2017.01.008.
- I. Chakravarty and S. A. Mandavgane, Valorization of fruit and vegetable waste for biofertilizer and biogas, J. Food Process Eng., 2021, 44(2), e13512, DOI:10.1111/jfpe.13512.
- A. Marone, G. Massini, C. Patriarca, A. Signorini, C. Varrone and G. Izzo, Hydrogen production from vegetable waste by bioaugmentation of indigenous fermentative communities, Int. J. Hydrogen Energy, 2012, 37(7), 5612–5622, DOI:10.1016/j.ijhydene.2011.12.159.
- N. Srivastava, M. Srivastava, E. F. AbduAllah, R. Singh, A. Hashem and V. K. Gupta, Biohydrogen production using kitchen waste as the potential substrate: A sustainable approach, Chemosphere, 2021, 271, 129537, DOI:10.1016/j.chemosphere.2021.129537.
- E. Matei, M. Râpă, A. M. Predescu, A. A. urcanu, R. Vidu, C. Predescu, C. Bobirica, L. Bobirica and C. Orbeci, Valorization of agri-food wastes as sustainable eco-materials for wastewater treatment: Current state and new perspectives, Materials, 2021, 14(6), 4581, DOI:10.3390/ma14164581.
- P. R. Ghosh, D. Fawcett, S. B. Sharma and G. E. J. Poinern, Production of high-value nanoparticles via biogenic processes using aquacultural and horticultural food waste, Materials, 2017, 10(8), 852, DOI:10.3390/ma10080852.
- S. Banerjee, R. Vijayaraghavan, A. F. Patti and A. Arora, Integrated Biorefinery Strategy for Valorization of Pineapple Processing Waste into High-Value Products, Waste Biomass Valorization, 2021, 13(1), 631–643, DOI:10.1007/s12649-021-01542-7.
- N. Jayesree, P. K. Hang, A. Priyangaa, N. P. Krishnamurthy, R. N. Ramanan, M. S. A. Turki, M. G. Charis and C. W. Ooi, Valorisation of carrot peel waste by water-induced hydrocolloidal complexation for extraction of carote and pectin, Chemosphere, 2021, 272, 129919, DOI:10.1016/j.chemosphere.2021.129919.
- A. D. Moreno, A. Duque, A. González, I. Ballesteros and M. J. Negro, Valorization of greenhouse horticulture waste from a biorefinery perspective, Foods, 2021, 10(4), 814, DOI:10.3390/foods10040814.
- S. Arora, J. Jung, M. Liu, X. Li, A. Goel, J. Chen, S. Song, C. Anderson, D. Chen, K. Leong, S. H. Lim, S. L. Fong, S. Ghosh, A. Lin, H. W. Kua, H. T. W. Tan, Y. Dai and C. H. Wang, Gasification biochar from horticultural waste: An exemplar of the circular economy in Singapore, Sci. Total Environ., 2021, 781, 146573, DOI:10.1016/j.scitotenv.2021.146573.
-
S. K. Panda and R. C. Ray, Microbial processing for valorization of horticultural wastes, in Environmental Microbial Biotechnology, Springer, Cham, 2015, pp. 203–221, DOI:10.1007/978-3-319-19018-1_11.
-
Utilization and management of horticultural waste, in Postharvest Technology of Perishable Horticultural Commodities, ed. M. G. Lobo and E., Dorta, Woodhead Publishing, Elsevier, 2019, 19, pp. 639–666, DOI:10.1016/B978-0-12-813276-0.00019-5.
- J. Villacís-Chiriboga, K. Elst, J. Van Camp, E. Vera and J. Ruales, Valorization of Byproducts from Tropical Fruits: Extraction Methodologies, Applications, Environmental, and Economic Assessment: A Review (Part 1: General Overview of the Byproducts, Traditional Biorefinery Practices,
and Possible Applications), Compr. Rev. Food Sci. Food Saf., 2020, 19(2), 405–447, DOI:10.1111/1541-4337.12542.
-
R. Salomone, A. Cecchin, P. Deutz, A. Raggi and L. Cutaia, Industrial Symbiosis for the Circular Economy: Operational Experiences, Best Practices and Obstacles to a Collaborative Business Approach, Springer, Cham, 2020, DOI:10.1007/978-3-030-36660-5.
- S. M. Andler and J. M. Goddard, Transforming food waste: how immobilized enzymes can valorize waste streams into revenue streams, Npj Sci. Food, 2018, 2(1), 19, DOI:10.1038/s41538-018-0028-2.
- J. Chapman, A. E. Ismail and C. Z. Dinu, Industrial applications of enzymes: Recent advances, techniques, and outlooks, Catalysts, 2018, 8(6), 20–29, DOI:10.3390/catal8060238.
- M. Bilal, M. Asgher, H. Cheng, Y. Yan and H. M. N. Iqbal, Multi-point enzyme immobilization, surface chemistry, and novel platforms: A paradigm shift in biocatalyst design, Crit. Rev. Biotechnol., 2019, 39(2), 202–219, DOI:10.1080/07388551.2018.1531822.
- H. U. Rehman, A. Aman, R. R. Zohra and S. A. U. Qader, Immobilization of pectin degrading enzyme from Bacillus licheniformis KIBGE IB-21 using agar-agar as a support, Carbohydr. Polym., 2014, 102, 622–626, DOI:10.1016/j.carbpol.2013.11.073.
- K. Banjanac, M. Carević, M. Ćorović, A. Milivojević, N. Prlainović, A. Marinković and D. Bezbradica, Novel β-galactosidase nanobiocatalyst systems for application in the synthesis of bioactive galactosides, RSC Adv., 2016, 6, 97216–97225, 10.1039/C6RA20409K.
- P. Santos-Moriano, J. M. Woodley and F. J. Plou, Continuous production of chitooligosaccharides by an immobilized enzyme in a dual-reactor system, J. Mol. Catal. B: Enzym., 2016, 133, 211–217, DOI:10.1016/j.molcatb.2016.09.001.
- M. Bilal, M. Asgher, R. Parra-Saldivar, H. Hu, W. Wang, X. Zhang and H. M. N. Iqbal, Immobilized ligninolytic enzymes: An innovative and environmental responsive technology to tackle dye-based industrial pollutants–a review, Sci. Total Environ., 2017, 576, 646–659, DOI:10.1016/j.scitotenv.2016.10.137.
- T. Tan, J. Lu, K. Nie, L. Deng and F. Wang, Biodiesel production with immobilized lipase: A review, Biotechnol. Adv., 2010, 28(5), 628–634, DOI:10.1016/j.biotechadv.2010.05.012.
- Y. Fan, Y. Fan, G. Wu, F. Su, K. Li, L. Xu, X. Han and Y. Yan, Lipase oriented-immobilized on dendrimer-coated magnetic multiwalled carbon nanotubes toward catalyzing biodiesel production from waste vegetable oil, Fuel, 2016, 178, 172–178, DOI:10.1016/j.fuel.2016.03.071.
- T. Korumilli and S. Mishra, Carotenoid production by Bacillus clausii using rice powder as the sole substrate: pigment analyses and optimization of key production parameters, J. Biochem. Technol., 2014, 5, 788–794 Search PubMed.
- S. Patthawaro, K. Lomthaisong and C. Saejung, Bioconversion of agro-industrial waste to value-added product lycopene by photosynthetic bacterium Rhodopseudomonas faecalis and its carotenoid composition, Waste Biomass Valorization, 2020, 11, 2375–2386, DOI:10.1007/s12649-018-00571-z.
- D. V. Morais and R. G. Bastos, Phycocyanin production by Aphanothece microscopica nageli in synthetic medium supplemented with sugarcane vinasse, Appl. Biochem. Biotechnol., 2019, 187, 129–139, DOI:10.1007/s12010-018-2811-6.
- H. W. Casullo de Araújo, K. Fukushima and G. M. C. Takaki, Prodigiosin Production by Serratia marcescens UCP 1549 Using Renewable-Resources as a Low Cost Substrate, Molecules, 2010, 15(10), 6931–6940, DOI:10.3390/molecules15106931.
- P. Velmurugan, H. Hur, V. Balachandar, S. Kamala-Kannan, K. J. Lee, S. M. Lee, J. C. Chae, P. J. Shea and B. T. Oh, Monascus pigment production by solid-state fermentation with corn cob substrate, J. Biosci. Bioeng., 2011, 112(6), 590–594, DOI:10.1016/j.jbiosc.2011.08.009.
- M. Khalil Kehili, L. M. Schmidt, W. Reynolds, A. Zammel, C. Zetzl, I. Smirnova, N. Allouche and S. Sayadi, Biorefinery cascade processing for creating added value on tomato industrial by-products from Tunisia, Biotechnol. Biofuels, 2016, 9, 261, DOI:10.1186/s13068-016-0676-x.
- H. A. Ruiz, R. M. Rodríguez-Jasso, R. Rodríguez, J. C. Contreras-Esquivel and C. N. Aguilar, Pectinase production from lemon peel pomace as support and carbon source in solid-state fermentation column-tray bioreactor, Biochem. Eng. J., 2012, 65, 90–95, DOI:10.1016/j.bej.2012.03.007.
-
F. Thakrar, D. Goswami and S. P. Singh, Production of an alkaline protease from Nocardiopsis Alba Om-4, a haloalkaliphilic actinobacteria in solid-state fermentation using agricultural waste products, in Proceedings of the National Conference on Innovations in Biological Sciences (NCIBS), SSRN, 2020 Search PubMed.
- M. V. Tacin, F. P. Massi, M. H. P. Fungaro, M. F. S. Teixeira, A. V. de Paula and V. de Carvalho Santos-Ebinuma, Biotechnological valorization of oils from agro-industrial wastes to produce lipase using Aspergillus sp. from Amazon, Biocatal. Agric. Biotechnol., 2019, 17, 369–378, DOI:10.1016/j.bcab.2018.11.013.
- C. C. Amorim, C. S. Farinas and E. A. Miranda, Liquefied wheat bran as carbon source and inducer in high-solids submerged cultivation of Aspergillus niger for xylanase production, Biocatal. Agric. Biotechnol., 2019, 21, 101346, DOI:10.1016/j.bcab.2019.101346.
- M. M. Rashad, A. E. Mahmoud, M. M. Ali, M. U. Nooman and A. S. Al-Kashef, Antioxidant and anticancer agents produced from pineapple waste by solid state fermentation, Int. J. Toxicol. Pharmacol. Res., 2015, 7, 287–296 Search PubMed.
- J. J. Buenrostro-Figueroa, M. Velázquez, O. Flores-Ortega, J. A. Ascacio-Valdés, S. Huerta-Ochoa, C. N. Aguilar and L. A. Prado-Barragán, Solid state fermentation of fig (Ficus carica L.) by-products using fungi to obtain phenolic compounds with antioxidant activity and qualitative evaluation of phenolics obtained, Process Biochem., 2017, 62, 16–23, DOI:10.1016/j.procbio.2017.07.016.
- C. G. Schmidt, L. M. Gonçalves, L. Prietto, H. S. Hackbart and E. B. Furlong, Antioxidant activity and enzyme inhibition of phenolic acids from fermented rice bran with fungus Rizhopus oryzae, Food Chem., 2014, 146, 371–377, DOI:10.1016/j.foodchem.2013.09.101.
- A. M. Goula and H. N. Lazarides, Integrated processes can turn industrial food waste into valuable food by-products and/or ingredients: the cases of olive mill and pomegranate wastes, J. Food Eng., 2015, 167, 45–50, DOI:10.1016/j.jfoodeng.2015.01.003.
- E. Oskoueian, N. Abdullah, R. Hendra and E. Karimi, Bioactive compounds, antioxidant, xanthine oxidase inhibitory, tyrosinase inhibitory and anti-inflammatory activities of selected agro-industrial by-products, Int. J. Mol. Sci., 2011, 12(12), 8610–8625, DOI:10.3390/ijms12128610.
- A. Cardoso, C. I. M. Lins, E. R. Dos Santos, M. C. F. Silva and G. M. Campos-Takaki, Microbial enhance of chitosan production by Rhizopus arrhizus using agroindustrial substrates, Molecules, 2012, 17, 4904–4914, DOI:10.3390/molecules17054904.
-
M. A. R. Mazumder, J. Rana, M. F. Jubayer, T. V. Ranganathan, and M. J. Ansari, Sonication microwave synergistic extraction of bioactive compounds from plant source, in Ultrasound and Microwave for Food Processing, Academic Press, 2023, pp. 239–267, DOI:10.1016/B978-0-323-95991-9.00013-8.
- G. Garai-Ibabe, I. Ibarburu, I. Berregi, O. Claisse, A. Lonvaud-Funel and A. Irastorza,
et al., Glycerol metabolism and bitterness producing lactic acid bacteria in cidermaking, Int. J. Food Microbiol., 2008, 121, 253–261, DOI:10.1016/j.ijfoodmicro.2007.11.004.
- J. C. Peinemann and D. Pleissner, Material utilization of organic residues, Appl. Biochem. Biotechnol., 2018, 184, 733–745, DOI:10.1007/s12010-017-2586-1.
- N. Cao, J. Du, C. S. Gong and G. T. Tsao, Simultaneous production and recovery of fumaric acid from immobilized Rhizopus oryzae with a rotary biofilm contactor
and an adsorption column, Appl. Environ. Microbiol., 1996, 62, 2926–2931, DOI:10.1128/aem.62.8.2926-2931.1996.
Footnote |
† These authors contributed equally to this work. |
|
This journal is © The Royal Society of Chemistry 2024 |
Click here to see how this site uses Cookies. View our privacy policy here.