Characterization and dermal bioaccessibility of residual- and listed PFAS ingredients in cosmetic products†
Received
20th October 2023
, Accepted 24th December 2023
First published on 4th January 2024
Abstract
As a large group of chemicals with diverse properties, per- and polyfluoroalkyl substances (PFAS) have found extensive application throughout consumer products, including cosmetics. Little is known about the importance of dermal uptake as a human exposure pathway for PFAS. Here we investigate a suite of listed-ingredient and residual PFAS in cosmetic products, along with their dermal bioaccessibility using in vitro incubations with artificial sweat. Concentrations of volatile listed ingredients (including cyclic perfluorinated alkanes, perfluorinated ethers, and polyfluorinated silanes) in three products ranged from 876–1323 μg g−1, while polar listed ingredients (i.e., polyfluoroalkyl phosphate esters [PAPs]) in a single product occurred at up to 2427 μg g−1 (6
:
2/6
:
2 diPAP)). Residual perfluoroalkyl carboxylic acids (PFCAs) were also measured at concentrations ranging from 0.02–29 μg g−1. When listed ingredients were included, our targeted analysis accounted for up to 103% of the total fluorine, while highlighting ambiguous and/or incorrect International Nomenclature of Cosmetic Ingredient (INCI) names used in several products. Bioaccessibility experiments revealed that residual PFCAs readily partitioned to artificial sweat (bioaccessible fractions ranging from 43–76% for detectable substances) while listed ingredients (i.e., PAPs and neutral/volatile PFAS) displayed negligible partitioning. This work provides new insight into the occurrence of PFAS in cosmetic products, while furthering our understanding on their mechanisms of dermal uptake.
Environmental significance
Per- and polyfluoroalkyl substances (PFAS) occur as both listed and residual ingredients in cosmetic products, but many of these substances remain unquantified using current methods. Through development and application of new analytical methods, the present work quantified a suite of previously overlooked volatile PFAS for the first time, and used these concentrations to close the fluorine mass balance in several cosmetic products. Collectively, these data help to improve the accuracy of PFAS environmental emission estimates from cosmetics, in particular with regards to emission of volatile PFAS to the atmosphere. Moreover, an assessment of dermal bioaccessibility of both listed- and residual PFAS in cosmetics provides improved understanding of the physical–chemical properties favouring dermal uptake, and ultimately the significance of dermal uptake as an exposure pathway to PFAS.
|
Introduction
Per- and polyfluoroalkyl substances (PFAS) are a large group of anthropogenic fluorinated compounds characterized by the presence of at least one perfluorinated methyl (–CF3) or methylene (–CF2–) group.1 Perfluoroalkyl chains impart unique physicochemical properties on a molecule, including combined lipophobicity/hydrophobicity and resistance to thermal and biological degradation.2 These properties have contributed to the extensive use of PFAS across numerous consumer products, for example in cosmetics, food packaging, and plastics.3 Ultimately, the widespread use of PFAS, together with their persistence and mobility have resulted in near ubiquitous contamination of humans and the environment globally.4
PFAS that are intentionally added to cosmetic products function as surfactants, emulsifiers, solvents, and conditioning and viscosity agents.5 Studies seeking to quantify these substances have focused mostly on polyfluoroalkyl phosphate esters (PAPs), which have been reported at sum concentrations of up to 1080 μg g−1 in cosmetic products from Sweden, the US and Canada.5–8 Residual impurities, such as perfluoroalkyl acids (PFAAs) and fluorotelomer-based PFAA-precursors, have also been observed at several orders of magnitude lower concentrations than PAPs (i.e. 0.45–5900 ng g−1).5–9 Given the diversity of PFAS in cosmetics — many of which are currently not captured by conventional analytical techniques — total fluorine (TF) and extractable organic fluorine (EOF)-based measurements have also been applied, revealing concentrations of up to 14
000 μg F g−1 in some products.5,7,8 However, these approaches have several drawbacks, for example interference from inorganic fluorine in the case of TF, and poor extraction efficiency for certain PFAS in the case of EOF.7 In particular when it comes to volatile listed PFAS ingredients, new analytical methods must be developed before these substances can be quantified in cosmetics products.
For the general human adult population, diet is widely considered to be the most important exposure pathway.10 Drinking water has also been shown to contribute in communities with a contaminated water supply11 and ingestion/inhalation of dust/indoor air may be important in households that regularly apply PFAS-containing carpet treatments.12,13 The importance of dermal uptake to overall PFAS exposure, however, is much less clear.14 For example, a Norwegian study examining pathways of human exposure suggested that dermal exposure to known PFAS in consumer products represented only ∼0.3% (median; range 0.2–7%) of total PFAS exposure.15 Other recent epidemiological studies have reported strong positive associations between several PFAS in serum and the use of skin care products (e.g. sunscreen, cosmetics and facial cleaner),16,17 suggesting that dermal exposure may represent a significant portion of overall PFAS exposure.
Lab-based experiments have also provided evidence that PFAS may be absorbed by the skin. Dermal absorption is a 2-step process involving partitioning of a chemical from a product into the skin-surface film liquid (SSFL) (i.e. bioaccessibility), followed by permeation through the skin (i.e. bioavailability). Bioaccessibility is highly influenced by the composition of the SSFL as well as substance-specific physicochemical properties.18,19 For example, the bioaccessibility of perfluoroalkyl acids (PFAAs) from textiles to sweat was reported to be significant (24–90%), but decreased with increasing perfluoroalkyl chain length.20 A similar trend was observed from dust, where bioaccessibility fractions of 54–92% and 61–77% were observed for PFCAs and perfluoroalkyl sulfonic acids (PFSAs) using 1
:
1 sebum/sweat.19 In that work, the presence of cosmetic products had a small effect on bioaccessibility for some PFAS, while increasing the percentage of sebum significantly enhanced bioaccessibility, but mostly for longer chain length PFCAs. Early work investigating dermal uptake of ammonium perfluorooctanoate (PFOA) concluded that only a small portion was bioavailable (0.048 ± 0.01%),21 but was later shown to be much greater (up to 69%) at lower pH, when the neutral (acid) species is dominant.22 However, since normal stratum corneum pH typically ranges from 4.5–5.5 (compared to a pKa for PFOA of ∼0.5 (ref. 23 and 24)), it is expected that PFAAs would exist primarily in the ionized form, rendering dermal uptake under normal physiological conditions less likely. Nevertheless, subsequent studies performed without pH adjustment showed absorption of significant quantities of PFOA, including in a human volunteer exposed via fortified sunscreen (∼1.6% absorbed),25 and in rodents exposed via a solution in acetone (∼3% absorbed).26 The latter study also showed that short-chain PFAS, especially perfluoroheptanoate (PFHpA, 15-16% absorbed) and perfluorobutane sulfonate (PFBS, 15–18% absorbed), were more easily absorbed than their long-chain homologues.26
Despite the evidence for dermal uptake of PFAS, there remains a paucity of data on absorption of PFAS occurring in cosmetics, in particular listed ingredients, which can have significantly different physical–chemical properties compared to PFAAs.26 To the best of our knowledge, only a single study has investigated absorption of polyfluoroalkyl phosphate esters (PAPs; known listed ingredients in cosmetics), which demonstrated that PAPs are less efficiently absorbed than PFAAs. For neutral PFAS, direct dermal uptake from the gas phase has also been predicted.27
The present work investigated a wide range of residual- and listed PFAS ingredients in cosmetic products, many of which are determined here for the first time. These measurements were combined with determination of TF and EOF (the latter performed using both MeOH and toluene-based extractions) in order to assess fluorine mass balance. Thereafter, we incubated the cosmetics with artificial sweat in order to evaluate bioaccessibility of the PFAS ingredients and residuals. Collectively, this work provides new insight into the occurrence of PFAS in cosmetic products while furthering our understanding on their mechanism of dermal uptake.
Materials and methods
Standards and reagents
Perfluorohexane (99% purity), methyl perfluorobutyl ether (99%; unspecified mixture of linear and isobutyl isomers), perfluorodecalin (95% purity), perfluoro-1,3-dimethylcyclohexane (80% purity) and HPLC grade toluene (≥99.8% purity) were purchased from Sigma Aldrich. Perfluoroperhydrophenanthrene (80% purity) was purchased from Avantor®. Linear isomer standards used for targeted PFAS analysis included perfluorobutanoate (PFBA), perfluoropentanoate (PFPeA), perfluorohexanoate (PFHxA), PFHpA, PFOA, perfluorononanoate (PFNA), perfluorodecanoate (PFDA), perfluoroundecanoate (PFUnDA), perfluorododecanoate (PFDoDA), perfluorotridecanoate (PFTriDA), perfluorotetradecanoate (PFTeDA), perfluorohexadecanoate (PFHxDA), perfluorooctadecanoate (PFOcDA), PFBS, perfluorohexane sulfonate (PFHxS), PFOS, perfluorodecane sulfonate (PFDS), phosphoric acid 3,3,4,4,5,5,6,6,6-nonafluorohexyl ester (4
:
2 monoPAP), 1H,1H,2H,2H-perfluorooctylphosphate (6
:
2 monoPAP), 1H,1H,2H,2H-perfluorodecylphosphate (8
:
2 monoPAP), mono[2-(perfluorodecyl)ethyl] phosphate (10
:
2 monoPAP), bis(3,3,4,4,5,5,6,6,6-nonafluorohexyl) hydrogen phosphate (4
:
2/4
:
2 diPAP), bis(1H,1H,2H,2H-perfluorooctyl) phosphate (6
:
2/6
:
2 diPAP), (1H,1H,2H,2H-perfluorodecyl) phosphate (6
:
2/8
:
2 diPAP) and bis(1H,1H,2H,2H-perfluorodecyl) phosphate (8
:
2/8
:
2 diPAP) were purchased from Wellington Laboratories (Guelph, Canada). Isotopically-labeled standards were also purchased from Wellington (see ESI for details†). Solvents and reagents used for extraction and LC-MS analytical procedures were of analytical grade and purchased as follows: methanol, acetonitrile and ammonium acetate from Merck, sodium hydroxide, acetic acid and hydrochloric acid (37%) from Sigma Aldrich. Supelclean™ ENVI-Carb™ powder was obtained from Supelco, Sigma Aldrich. Argon and oxygen gases were of purity grade 5.0 and MilliQ water obtained from Millipore (Merck, TOC of 3 ppb, conductivity of 18.2 MΩ).
Cosmetic product selection and experimental approach
In our recent study, an inventory of PFAS ingredients and their frequency of occurrence in cosmetic products within Europe was determined.7 From this work, a total of six cosmetic products were selected for more extensive characterization, including targeted analysis, EOF determination, and bioaccessibility assessment. The products were selected based on high levels of TF (reported previously)7 and structural diversity of PFAS in the lists of ingredients. All cosmetic products were purchased from local stores in Stockholm in September 2020 and contained at least one PFAS (except for a PFAS-free sample, used as blank) in their ingredient lists. An overview of the samples, fluorinated ingredients and product names as listed on the packaging is provided in Table 1.
Table 1 List of sample IDs, product and brand names, listed PFAS ingredient (as written on the product), and corresponding PFAS targeted in the present work
Sample ID |
Product name |
Brand/company |
Listed ingredient(s) |
Target PFAS based on listed ingredient(s)a |
Monitored across all products.
Not measurable by methods used in the present work.
Monitored without an authentic standard.
|
Concealer |
CC C'est magic anti-redness skin enhancer |
L'Oréal Paris |
Perfluorooctyl triethoxysilane |
Triethoxy(3,3,4,4,5,5,6,6,7,7,8,8,8-tridecafluorooctyl)silane |
Foundation/BB cream |
Fresh nude foundation SPF 15, sahara light 030 |
The body shop |
Ammonium C6-16 perfluoroalkylethyl phosphate |
4 : 2 monoPAP, 6 : 2 monoPAP, 8 : 2 monoPAP, 10 : 2 monoPAP, 4 : 2/4 : 2 diPAP, 4 : 2/6 : 2 diPAPc, 6 : 2/6 : 2 diPAP, 6 : 2/8 : 2 diPAP and 8 : 2/8 : 2 diPAP |
Loose powder |
Loose powder |
Inglot |
Polyperfluoromethylisopropyl etherb |
— |
Mask 2 |
Water drench hyaluronic micro-bubbling cloud mask |
Peter thomas roth |
Methyl perfluorobutyl ether |
1,1,1,2,2,3,3,4,4-Nonafluoro-4-methoxybutane |
Methyl perfluoroisobutyl ether |
2-[Difluoro(methoxy)methyl]-1,1,1,2,3,3,3-heptafluoropropane |
Perfluorohexane |
1,1,1,2,2,3,3,4,4,5,5,6,6,6-Tetradecafluorohexaneb |
Perfluoroperhydrophenanthrene |
1,1,2,2,3,3,4,4,4a,4b,5,5,6,6,7,7,8,8,8a,9,9,10,10,10a-Tetracosafluorophenanthrene |
Perfluorodecalin |
1,1,2,2,3,3,4,4,4a,5,5,6,6,7,7,8,8,8a-Octadecafluoronaphthalene |
Perfluorodimethylcyclohexane |
1,1,2,2,3,3,4,5,5,6-Decafluoro-4,6-bis(trifluoromethyl)cyclohexane |
Mask 3 |
Bubblesheet oxygenating deep cleanse mask |
Glamglow |
Methyl perfluorobutyl ether |
1,1,1,2,2,3,3,4,4-Nonafluoro-4-methoxybutane |
Treatment |
Perfect hair day |
Living proof |
— |
— |
Monitored residuals: C4–C14 PFCAs, C4, C6, C8, C10 PFSAs |
Our target analysis sought to capture all listed PFAS ingredients in each product, with the exception of loose powder (which listed a polymer – polyperfluoromethylisopropyl ether), and foundation/BB cream (which listed “ammonium C6-16 perfluoroalkylethyl phosphate”), for which we included a suite of PAPs (4
:
2, 6
:
2, 8
:
2 and 10
:
2 monoPAPs, 4
:
2, 4
:
2/6
:
2 [monitored without an authentic standard], 6
:
2, 6
:
2/8
:
2 and 8
:
2/8
:
2 diPAPs). In addition, a suite of PFAAs (i.e. C4–C14 PFCAs, C4, C6, C8, C10 perfluoroalkyl sulfonic acids [PFSAs]) which we suspected may occur as residuals, were monitored. A comparison of listed ingredients vs. monitored targets is provided in Table 1.
Dermal bioaccessibility assay
The dermal bioaccessibility assay was performed at the University of Birmingham using a previously established method that approximates wet skin conditions (i.e. 60 mg cosmetics/6 mL sweat).18,19,28 Artificial sweat was prepared according to a previously reported US patent and pH adjusted to 5.3 ± 0.1 (normal human skin pH). Table S1 in the ESI† lists the compounds used in the preparation of artificial sweat.29 Sebum was not included because the method described in the US patent applies a mixture of chloroform/methanol as solvents for sebum ingredients, which may influence PFAS partitioning under our bioaccessibility test conditions. The bioaccessibility assay was carried out as follows: a glass tube containing 60 mg of cosmetic and 6 mL of sweat was gently agitated at 32–34 °C (average temperature of human skin surface) with glass covered magnetic beads for 1 h. At the end of the exposure, the two phases were separated by centrifugation. After centrifugation (3900 rpm, 20 min), the supernatant synthetic sweat was transferred to a clean PP tube. The supernatant and residuals were sealed in polypropylene and glass tubes, respectively, and shipped to Stockholm University for analysis. All of the experiments were performed at least in triplicate. Procedural blanks (artificial sweat only; n = 4) were also included.
Sample handling and instrumental analysis
Extraction of cosmetics and sweat.
Separate extraction and instrumental analysis methods were used for ionizable/polar and neutral/volatile PFAS. The polar extraction procedure for cosmetics was as follows: about 5 mL of methanol was added to a ∼0.1 g sample of cosmetic product which was then vortexed and ultrasonicated for 30 minutes at room temperature. The supernatant was transferred into a new tube following centrifugation (2000 rpm, 5 minutes). The procedure was repeated and the supernatants were combined. Thereafter, the solvent was evaporated under a gentle stream of nitrogen to approximately 1 mL. 100 μL of the concentrated extract were weighed and transferred to a 1.5 mL Eppendorf tube containing 25 mg of graphitized carbon (Supelclean ENVI-Carb) and 50 μL glacial acetic acid. The tubes were vortexed and centrifuged (10
000 rpm, 10 minutes) before transferring the supernatant to a new Eppendorf tube. At this point, the extract was divided into two portions: 500 μL was set aside for EOFpolar analysis while 100 μL was spiked with 50 μL of an isotopically labelled standard solution (20 pg μL−1) for target PFAS quantification. Samples were processed in triplicate and all sample extracts were stored in a freezer until the day of analysis. To quantify polar PFAS in artificial sweat, samples were fortified with IS and then analysed directly by LC-MS/MS. All sweat samples were measured in triplicate except for sweat from masks 2 and 3, which were measured without replication due to an insufficient amount of sample.
The EOFnon-polar extraction procedure involved addition of toluene (2.5 mL) to a ∼0.1 g sample of cosmetic, which was subsequently vortexed and ultrasonicated (30 minutes at room temperature) followed by centrifugation (2000 rpm, 5 minutes). After separating the supernatant, the extraction was repeated with an additional 2.5 mL of toluene. Supernatants were combined and the extract was sealed and stored in a refrigerator for no more than 48 h prior to analysis to minimize loss of volatiles. All samples were prepared in triplicate. For quantifying non-polar PFAS in sweat, samples (1 mL) were shaken together with toluene (1 mL) in a glass tube. Thereafter, the mixture was left to stand for 5 minutes and the organic phase transferred to a separate glass tube for analysis. All samples were prepared in triplicates without internal standards in order to facilitate comparisons to CIC measurements (i.e. EOFnon-polar). Further details on the sweat extraction procedure can be found in the extraction and analysis procedures section of the ESI.†
LC-MS/MS analysis of polar PFAS.
Extracts (5 μL) were injected onto an Acquity ultra performance liquid chromatograph (UPLC) (Waters Corp., Milford, MA) equipped with a BEH C18 guard (5 × 2.1 mm, 1.7 mm particle size) and an analytical (50 × 2.1 mm, 1.7 mm) column operated at 40 °C. To increase sensitivity and improve chromatographic peak shape, 1-methylpiperidine (1 MP) was added to the mobile phase, as described previously.30 The composition of the mobile phase and details on the gradient and the flow rate can be found in Table S2.† Detection of PFAS was carried out using a Xevo TQ-S triple quadrupole mass spectrometer (Waters Corp, Milford, MA) operated in negative electrospray ionization mode according to a method reported previously.10 The capillary voltage was set to 3 kV and the desolvation and source temperature were set to 350 °C and 150 °C, respectively. The desolvation and cone gas flows were set to 150 L h−1 and 650 L h−1, respectively. Further information on MS parameters are presented in Table S3.† Quantification of PFCAs and PAPs was performed using MassLynx 4.1 (Waters), via an 8-point calibration curve ranging from 0.02 to 100 pg μL−1. Analytes lacking an analogous isotope labelled standard were quantified using the internal standard with the closest retention time. In cases where peak areas exceeded the highest point on the calibration curve, the extract was diluted by a factor of 100, and fortified with additional internal standard prior to re-analysis.
GC-ECD analysis of non-polar PFAS.
While gas chromatography coupled to mass spectrometry (GC-MS) is widely used for analysis of semi-volatile PFAS, our initial attempts at utilizing this technique were hampered by co-elution of the solvent (toluene) with the target analytes, which required removal of the solvent delay. Unfortunately, this resulted in rapid degradation of the filament; indicating that this approach could not be utilized for more than a few samples at a time. Gas chromatography – electron capture detection (GC-ECD), on the other hand, does not utilize a filament (and therefore does not require a solvent delay), and is a selective and sensitive detection technique for halogenated substances. Moreover, non-halogenated solvents (e.g. toluene) are not detectable by ECD, thereby eliminating the possibility of interferences caused by the solvent. While ECD has the disadvantage that it cannot resolve co-eluting substances using mass-to-charge ratios, this only posed a problem for one product (mask 2) which contained a mixture of PFAS ingredients which were difficult to baseline resolve (see results and discussion).
Instrumental analysis was performed using an Agilent 6890 gas chromatograph (GC) equipped with a Thermo TG-5MS capillary column (60 m, 250 μm, 0.25 μm) and coupled to an ECD. The oven temperature program was started at 70 °C and held for 5 min, then increased to 90 °C and held for 5 min at 4 °C min−1 rate. Then, the temperature was increased to 290 °C and held for 13 min at 15 °C min−1 rate and finally ended at 290 °C (see details in Table S4, ESI†). The injection volume was 2 μL and the carrier gas (helium) was set at a flow rate of 1.6 mL min−1. The ECD make-up gas (80/10 argon/methane) was set at a flow rate of 25 mL min−1. Standard calibration curves (500, 100, 50, 20, 10, 1 and 0.5 μg mL−1) were prepared in toluene and were then used for the quantification of targeted PFAS. Standards were subjected to GC-ECD within 48 h of preparation due to high volatility of substances and peak areas were manually integrated.
TF and EOF determination by CIC
TF data for all cosmetic products and EOFpolar data from mask 2, concealer and foundation/BB cream were previously reported in Pütz et al..7 EOF measurements for remaining samples are reported for the first time here. Briefly, 0.1–1 mg of solid sample (i.e. neat cosmetic) or 100 μL of liquid (i.e. methanol, toluene or sweat) was placed into a ceramic boat containing glass wool for better dispersion. To minimize background contamination, all boats, including glass wool, were baked prior to use. The samples were combusted in a combustion furnace (HF-201, Mitsubishi) at 1100 °C under a flow of oxygen (400 mL min−1) and argon mixed with water vapor (200 mL min−1) for approximately 6 min. Combustion gases were absorbed in Milli-Q water during the entire length of the combustion process using a gas absorber unit (GA-210, Mitsubishi). A 100 μL aliquot of the absorption solution was subsequently injected onto a Dionex Integrion high performance ion chromatograph (Thermo Fisher Scientific) equipped with an anion exchange column (Dionex IonPac AS19 2 × 50 mm guard column and 2 × 250 mm analytical column, 7.5 mm particle size) operated at 30 °C. Chromatographic separation was achieved by running a gradient of aqueous hydroxide mobile phase ramping from 8 to 60 mM at a flow rate of 0.25 mL min−1, and fluoride were detected by a conductivity detector. The retention time of fluoride was approximately 6.36 min.
Quality control – cosmetics and sweat samples
Quality control associated with cosmetic TF measurements as well as EOFpolar measurements for mask 2, concealer and foundation/BB cream are reported in detail elsewhere.7 Briefly, the accuracy and precision of TF determinations were confirmed through triplicate combustions of a certified reference material (CRM; BCR®-461, fluorine in clay), which produced an average percent recovery of 90% (2.5% relative standard deviation [RSD], n = 3). For TF, EOFpolar and EOFnon-polar measurements, background contamination was assessed from blanks (boat blanks and procedural blanks, respectively) which were analyzed along with samples. All TF data were blank subtracted and the limits of detection (LODs) were calculated as 3 times the standard deviation of the blanks. A standard mixture of PFOS and PFOA (0.7398 μg F mL−1) which was measured repeatedly in the same sequence as real samples displayed good agreement with theoretical concentrations (average recoveries of 97% ± 5% RSD, [n = 4] and 107% ± 3.5% RSD, [n = 4] for EOFpolar and EOFnon-polar analyses, respectively) indicating that the response measured on the CIC was both specific to fluorine and reproducible.
To evaluate the accuracy and precision of targeted measurements of polar PFAS, replicates of a PFAS-free cosmetic product were extracted and analysed with and without fortification of a suite of polar PFAS (i.e. 10 ng of individual PFAS; n = 3; Table S6†). Percent recovery for most substances fell within the range of 64–99% (6–35% RSD) with the exception of PFTeDA, PFBS, 4
:
2, 8
:
2, and 10
:
2 monoPAP, and 4
:
2/4
:
2 and 6
:
2/8
:
2 diPAP, which had lower recoveries (range 13–44%, RSD 6–54%). For sweat samples, fortification was performed into artificial sweat in the presence of a PFAS-free cosmetic (10 ng of individual PFAS; n = 3). Recoveries for most substances fell within the range of 85–95%, with the exception of PFTeDA which displayed a lower recovery of 29% and 4
:
2/4
:
2 diPAP with 138% as an outlier. Overall, recoveries from synthetic sweat were slightly higher than those obtained from the cosmetic matrix for PFCAs and PAPs, suggesting that bioaccessibility may be somewhat overestimated for these substances. Limits of detection (LODs) were determined using the concentration obtained from the lowest calibration point with a well-shaped peak displaying an intensity >1000 and a signal-to-noise (S/N) ratio of >3 and converted to a weight-based concentration (e.g. ng g−1) using the average sample weight.
For QC of PFAS analysed by GC-ECD, the spike/recovery experiment was repeated but with a suite of non-polar PFAS (12.5 μg of individual substances; n = 3, Table S7†). Percent recoveries ranged from 44% to 82% (4–16% RSD) in cosmetics and 56% to 84% (2–20% RSD) in sweat samples, indicating somewhat variable accuracy but good precision in both matrices. In both matrices, perfluorooctyltriethoxysilane consistently produced among the lowest recoveries (44%), while perfluoroperhydrophenanthrene had the highest recovery (82%). The low boiling point/high vapor pressure of some substances (see Table S5†) may have contributed to low recoveries in some cases due to evaporative losses. Notably, low recoveries for perfluorooctyltriethoxysilane (44%) in cosmetics and perfluorodecalin (56%) in sweat may lead to potential under-reporting of bioaccessibility by up to 56% and 43%, respectively. Despite these discrepancies, the recoveries were deemed acceptable, especially since internal standards were not utilized for correcting procedural losses. LODs were determined using 3 times standard deviation of concentration in procedural blank plus average concentration (n = 3). Concentrations < LOD were substituted by LOD/2 and were not blank-corrected. The LOD values for cosmetics and sweat can be found in Tables S8 and S9 in ESI.†
Data handling
To enable comparisons to TF and EOFpolar/non-polar data, targeted PFAS concentrations were converted to fluorine equivalents (CF_PFAS; ng F g−1) using the following Eq. 1: | CF_PFAS = nF × AF/MWPFAS × CPFAS | (Eq. 1) |
where CPFAS and nF are the concentration and number of fluorine atoms for a given target, AF is the atomic weight of fluorine (19.0 g mol−1), and MWPFAS is the molecular weight of the target (g mol−1). Once the concentrations were converted to fluorine equivalents (i.e. ng F g−1), they were summed to obtain polar/non-polar ΣCF_PFAS concentrations, which were directly comparable to EOFpolar/non-polar and TF measurements.
Bioaccessibility fraction (fbioaccessibile; %) was calculated using Eq. 2.
|  | (Eq. 2) |
Results and discussion
Inaccuracies in INCI names revealed by targeted analysis
Target PFAS determined by either LC-MS/MS (MeOH extraction) or GC-ECD (toluene extraction) were not detected in 2 out of 6 products, namely treatment (which did not contain listed PFAS ingredients), and loose powder (which contained a fluorinated polymer which was not measurable by our methods). For the remaining products, the listed PFAS ingredient(s) generally aligned with substances on our target list, albeit with some notable exceptions. Foundation/BB cream, which listed “ammonium C6–16 perfluoroalkylethyl phosphate” as an ingredient, contained 6
:
2 monoPAP, 4
:
2/6
:
2 diPAP, and 6
:
2/6
:
2 diPAP at average concentrations of 151 ± 20 μg g−1, 18 ± 1 μg g−1, and 2427 ± 304 μg g−1, respectively. Mono- and diPAP homologues with chain lengths shorter than 4
:
2 or larger than 6
:
2 were not observed. Concentrations of PAPs observed here are consistent with prior measurements of foundation/BB cream cosmetic samples in Sweden which disclosed PAPs on their ingredients lists5 but nearly ∼1000-fold higher than observations in North American cosmetics where ingredients were undisclosed.8 Health Canada has indicated that “ammonium C6-16 perfluoroalkylethyl phosphate” corresponds to “Phosphoric acid esters with 2-(perfluoro-C6-16-alkyl) ethanol, ammonium salt” (i.e. PAPs),31 but not whether the substance is a mono- or diPAP. Our analysis revealed the occurrence of both mono- and di-esters with chain lengths as short as 4
:
2, neither of which are specified by the INCI name. We cannot rule out that historical versions of this ingredient contained longer chain length homologues (i.e. up to C16) and were subsequently modified along with increased regulation of long chain PFAAs and PFAA-precursors; nevertheless, our analysis clearly shows that the current INCI name is poorly aligned with the actual substances used in the product.
In the case of Concealer, the listed ingredient was “perfluorooctyl triethoxysilane”, yet upon measuring this substance by GC-ECD (toluene extraction) we observed a small discrepancy between the retention time of the sample and that of the standard (14.46 min vs. 14.50 min, respectively; Fig. 1). To assess whether the apparent retention time shift was due to a matrix effect, or a mismatch between the substance in the sample and the standard, the sample extract was fortified with a standard and re-measured. The standard addition revealed two peaks, confirming that the fluorinated ingredient in Concealer was slightly different to the analytical standard (Fig. 1). Based on the close retention time to the standard of perfluorooctyl triethoxysilane, and the fact this substance is stated on the product's ingredient list, we suspect that the structure in the product may be an isomer. Semi-quantification of the peak using our perfluorooctyltriethoxysilane standard revealed concentrations of 164 ± 45 μg g−1, but this value remains tentative due to the mismatch between sample and standard. Overall, our observations in both concealer and foundation cream highlight the inaccuracy of some INCI names.
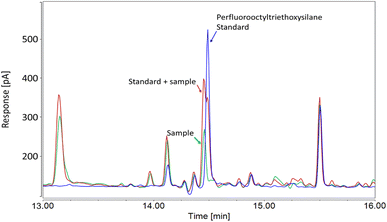 |
| Fig. 1 Comparison of GC-ECD chromatograms for an authentic standard of perfluorooctyl triethoxysilane (blue trace), concealer extract (green trace), and the concealer extract fortified with an authentic standard of perfluorooctyl triethoxysilane (red trace). | |
Mask 2, which listed six PFAS ingredients (i.e. methyl perfluorobutyl ether, methyl perfluoroisobutyl ether, perfluoro-1,3-dimethylcyclohexane, perfluorodecalin, perfluoroperhydrophenanthrene, and perfluorohexane) also exhibited the highest sum PFAS concentrations (1452 ± 248 μg g−1). Among the listed PFAS ingredients, perfluorodecalin was the most prevalent (841 ± 121 μg g−1), followed by perfluoro-1,3-dimethylcyclohexane (505 ± 100 μg g−1) and perfluoroperhydrophenanthrene (106 ± 27 μg g−1). Perfluorohexane was unfortunately not quantifiable using this method, likely due to its extremely high volatility. Quantification of the two methyl perfluorobutyl ether isomers were also hampered by co-elution with each other and with perfluoro-1,3-dimethylcyclohexane. However, since the slope of the combined calibration curve for the methyl perfluorobutyl ether isomers was over an order of magnitude lower than the other targets, we assumed that the observed peak was primarily associated with perfluoro-1,3-dimethylcyclohexane. The exact contributions of perfluorohexane and the two methyl perfluorobutyl ether isomers remains unclear.
Analysis of mask 3 revealed two isomers of methyl perfluorobutyl ether, consistent with our analytical standard, at ∑concentrations of 876 ± 93 μg g−1. The observation of two isomers was surprising considering that the ingredient list only specifies a single PFAS (methyl perfluorobutyl ether), and because INCI names exist for both the linear and isopropyl isomers (as observed on the ingredient list for mask 2).
PFCA residuals were also observed in all samples, with the exception of loose powder (polymeric listed ingredient) and treatment (PFAS-free), with sum concentrations ranging from 0.099 μg g−1 (concealer) to 29.31 μg g−1 (mask 2). PFSAs were not observed in any samples. Mask 2 and 3 contained exclusively PFBA (29.3 and 22.47 μg g−1, respectively), while concealer contained a range of short-chain PFCAs (C4–C6; 0.024–0.05 μg g−1). Foundation/BB cream was the only product to contain a large range of chain lengths (C4–C8 PFCAs; 0.02–1.29 μg g−1). While the few samples investigated here did not allow us to draw associations between listed ingredient and residuals, we note that several prior studies have reported occurrence of PFCAs in products listing polyfluoroalkyl ethers, silicon-based PFAS, or PAPs in their ingredients lists.5,6,9,32 However, further research is necessary to determine whether PFCAs in these products originate as impurities from manufacturing or possibly transformation products.
Non-polar extractions help to close the fluorine mass balance in cosmetic products
TF concentrations in cosmetics investigated here were previously reported in Pütz et al. (2022) and were above detection limits for all but treatment (which was PFAS-free). While TF measurements are effective for capturing all PFAS in a sample, they can be confounded by inorganic sources of fluorine, such as synthetic mica, which are known to occur in cosmetic products. EOF measurements offer an effective means of removing inorganic fluorine, but are strongly influenced by the extraction procedure. For this reason, both EOFpolar and EOFnonpolar concentrations were determined in the present work (Fig. 3) (Table S11, ESI†). Among the 5 products listing PFAS ingredients, EOFpolar only exceeded EOFnonpolar concentrations in foundation/BB cream, which was not surprising considering that this was the only product containing polar listed ingredients (i.e. PAPs). Foundation/BB cream displayed a nearly closed fluorine mass balance, with comparable TF and EOFpolar concentrations (3307 vs. 3106 μg F g−1, respectively), and only slightly lower ∑PFASpolar concentrations (1613.6 μg F g−1; primarily due to PAPs). A closed fluorine mass balance was also observed for mask 3, with roughly equivalent concentrations of TF, EOFnon-polar and ∑PFASnon-polar (595.6, 377.9, and 598.9 μg F g−1, respectively). Concentrations for EOFpolar and ∑PFASpolar in this product were much lower (<14 μg F g−1) and did not contribute significantly to the fluorine mass balance. Mask 2 displayed similar results to mask 3 (i.e. EOFnon-polar ≈ ∑PFASnon-polar >> EOFpolar > ∑PFASpolar), with the exception that concentrations of TF were much higher than EOFnon-polar (i.e. 10
581.0 μg F g−1vs. 935.6 μg F g−1, respectively). While we cannot rule out potential losses of volatile PFAS during the extraction procedure, a more likely explanation for this difference is due to non-extractable fluorine occurring in mica listed among the product's ingredients.
Concealer was the only sample displaying consistent TF and EOFnon-polar concentrations (797 vs. 731 μg F g−1, respectively) but much lower ∑PFASnon-polar concentrations (79.3 μg F g−1). This gap in the organofluorine mass balance could be attributed to the discrepancy between the listed ingredient and standard used to quantify it (i.e. perfluorooctyl triethoxysilane; see discussion above) and/or the occurrence of additional, as-of-yet unrecognized PFAS that were not captured by our targeted analyses.
Finally, loose powder, which listed a polymeric ingredient (polyperfluoromethyl isopropyl ether), displayed concentrations of EOFnon-polar which were several orders of magnitude below TF (i.e. 38 vs. 6006 μg F g−1, respectively). This difference could be from a combination of either poor extraction efficiency of the polymer in toluene and/or the presence of synthetic fluorphlogopite, which was listed among the product's ingredients. Since the listed ingredient was not targeted here, it was not surprising that ∑PFASnon-polar concentrations were non-detectable.
Occurrence of PFAS in sweat
None of the non-polar/volatile PFAS measurable by GC-ECD were detectable in sweat samples produced from bioaccessibility experiments, which was not surprising considering their poor water solubility (Table 2). Based on their volatility (Table S5†), these substances can reasonably be expected to evaporate during application/use and are likely to persist in the environment. However, given the paucity of data surrounding these substances, their fate and behavior in the environment remains unclear. In the case of polar/non-volatile PFAS measurable by LC-MS, PAPs were not detectable but PFCA homologues from C4–C7 were observed in sweat samples obtained from incubation with foundation/BB cream (0.001–0.01 μg g−1), while PFBA was observed in sweat incubated with masks 2 and 3 (0.17 and 0.15 μg g−1 respectively; Fig. 2).
Table 2 The fbioaccessibility[%] of studied cosmetic samples. Results are presented as average ± SD (n = 3)a
Cosmetic products |
PFAS type |
Quantified PFAS |
Individual fbioaccessible [%] |
Water solubility (mol L−1) |
ND: Non-detectable concentrations in sweat. Estimate based on detection limit. N/A: Not available.
n = 1.
|
Mask 2 |
Residual |
PFBA |
56.6b |
3.46 |
Ingredient |
Perfluoro-1,3-dimethyl cyclohexane |
ND (<0.07 ± 0.015) |
0.000002 |
Ingredient |
Perfluorodecalin |
ND (<0.04 ± 0.005) |
0.000007 |
Ingredient |
Perfluoroperhydrophenanthrene |
ND (<0.63 ± 0.2) |
0.0000007 |
Mask 3 |
Residual |
PFBA |
66.2b |
3.46 |
Ingredient |
Methyl perfluorobutyl ether |
ND (<7.20) |
0.0003 |
Concealer |
Ingredient |
Perfluorooctyltriethoxysilane |
ND (<35.10) |
0.0001 |
Foundation/BB cream |
Residual |
PFBA |
56.1 ± 18.7 |
3.46 |
Residual |
PFPeA |
69.5 ± 15.1 |
0.0005 |
Residual |
PFHxA |
75.5 ± 9.70 |
0.0034 |
Residual |
PFHpA |
42.8 ± 14.3 |
0.0013 |
Residual |
PFOA |
ND (<97.8 ± 19.0) |
0.0006 |
Ingredient |
6 : 2 monoPAP |
ND (<0.026 ± 0.003) |
0.0057 |
Ingredient |
4 : 2/6 : 2 diPAP |
ND (<0.26 ± 0.01) |
0.003 |
Ingredient |
6 : 2/6 : 2 diPAP |
ND (<0.0019 ± 0.0002) |
0.000005 |
Loose powder |
N/A |
N/A |
N/A |
N/A |
Treatment (PFAS-free) |
N/A |
N/A |
N/A |
N/A |
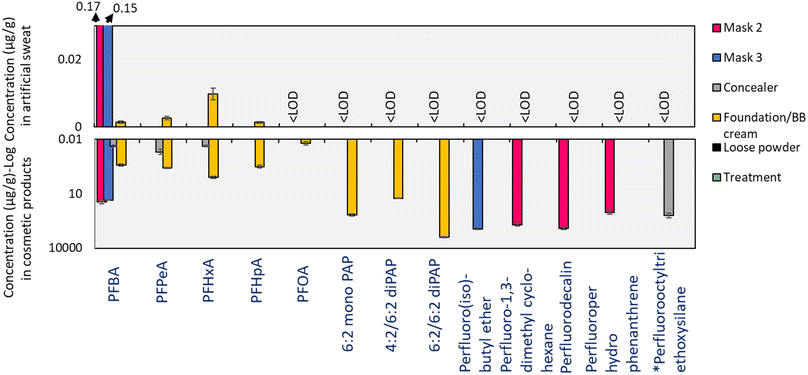 |
| Fig. 2 The average concentration of residuals-and listed-PFAS partitioned in (top) artificial sweat; (bottom) in cosmetic products (LODsweat = 0.00035 μg g−1). Error bars show the standard deviations (n = 3). *Measurement is considered semi-quantitative due to slight differences in standard used for quantification. | |
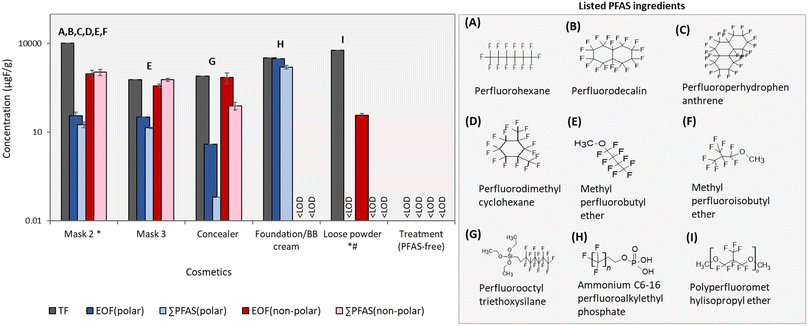 |
| Fig. 3 Fluorine mass balance showing TF, EOF and targeted PFAS concentrations (μg F g−1 sample) on a logarithmic scale. Letters (A–I) above each bar correspond to listed ingredients shown in the box on the right. The targeted PFAS (∑PFAS) concentrations were converted to fluorine concentrations for comparative purposes. (LODTF = 35.45 μg F g−1, LODEOF(polar) = 0.33 μg F g−1, LODEOF(non-polar) = 7.1 μg F g−1, LOD∑PFAS(polar) = 0.0015 μg F g−1, LOD∑PFAS(non-polar) = 0.13 μg F g−1). *mica listed among ingredients; #synthetic fluorphlogopite listed among ingredients. | |
Bioaccessibility of residuals exceeds that of listed PFAS ingredients
Due to the large number of non-detects in artificial sweat, worst-case estimates of bioaccessibility were calculated by substituting the concentration in sweat with the value of the LOD (Table 2). This produced reasonable estimates of bioaccessibility for most non-polar PFAS (i.e. <0.002% [6
:
2/6
:
2 diPAP] to <0.63% [perfluoroperhydrophenanthrene]) but unreasonably high estimates for methyl perfluorobutyl ether (<7.20%) and perfluorooctyl triethoxysilane (<35.10%) due to higher detection limits for these substances by GC-ECD. With the use of more sensitive analytical methods, it is expected that these estimates will improve.
In contrast to non-polar listed PFAS, residual PFCAs measured in both sweat and cosmetics exhibited much greater bioaccessibility (Table 2), with fractions ranging from 43% (PFHpA) to 75% (PFHxA). These values are generally lower than prior estimates of bioaccessibility from residual PFCAs in textiles (range 83–87.5% for C5–C8 PFCAs; 100% sweat) and dust (81–87%; C5–C7 PFCAs; 100% sweat), possibly due to differences in the composition of artificial sweat or matrix (i.e. cosmetics vs. textile and dust) used in each study.19,20 While Ragnarsdóttir et al. reported decreasing bioaccessibility with increasing chain-length, this trend was only observable for chain lengths ranging from C8–C14 in 100% sweat; below that (i.e. C4–C7), no trend was observed, consistent with the present work. Notably, in our work the bioaccessibility of PFBA (the only substance for which bioaccessibility was determined from multiple products) was fairly consistent (i.e. 56% from foundation/BB cream, 56.6% from mask 2, and 66.17% from mask 3). This suggests that the matrix had relatively little effect on bioaccessibility of PFBA, again consistent with Ragnarsdóttir et al., who also reported that bioaccessibility remained relatively consistent for this target in the presence of foundation, moisturizer, and deodorant, and only slightly lower in the presence of sunscreen. For other targets, bioaccessibility reportedly increased or decreased, depending on the cosmetic.19
Implications for dermal exposure to PFAS in cosmetics
This study was the first to quantify non-polar PFAS in cosmetic products, while highlighting the importance of alternative extraction procedures to close organofluorine mass balance. Several incorrect/ambiguous INCI names were identified, highlighting challenges associated with chemical characterization in consumer products. While listed PFAS ingredients appear relatively non-bioaccessible compared to residual PFCAs using artificial sweat, these substances may still pose a risk, either from direct dermal absorption from the gas phase (as predicted by Kissel et al., for volatile PFAS), or indirect exposure following transformation in the environment to persistent PFAAs.27 In comparison, PFAAs were readily bioaccessible, indicating that despite occurring at orders of magnitude lower concentrations than listed ingredients, they can nevertheless be relevant for dermal exposure. Clearly, the main limitation in this study was that our SSFL composition did not include sebum, which was previously shown to enhance bioaccessibility, in particular for more hydrophobic PFAS.19 The reason for not including sebum was that it was only available in a mixture of chloroform and methanol, which we expected would bias the partitioning behaviour of PFAS, in particular non-polar substances. Moreover, several prior studies19,20 on bioaccessibility have utilized 100% sweat, providing an opportunity for comparisons between studies. The bioaccessibility in vitro assay was limited to an incubation time of 1 hour, which may not accurately reflect the usage patterns of short- or long-term skincare products. To address this limitation, future work should include a wider range of incubation times. Furthermore, sorption and/or volatilisation may have occurred during incubation, contributing to losses of some listed PFAS ingredients. Finally, we note that our results are primarily relevant for post-application conditions, i.e. after the product has dried on the surface of the skin. When first applied as a wet product, some PFAS may already be bioaccessible from the product itself. Direct absorption from cosmetic products, together with the role of sebum on bioaccessibility, require further investigation for PFAS.
Conflicts of interest
There are no conflicts to declare.
Acknowledgements
This project has received funding from the European Union’s Horizon 2020 research and innovation programme under Marie Skłodowska-Curie Action Grant Agreement 860665 (PERFORCE3) and from Swedish Research Council FORMAS under Grant Agreement 2018-00930.
References
- Z. Wang, A. M. Buser, I. T. Cousins, S. Demattio, W. Drost and O. Johansson,
et al., A New OECD Definition for Per- and Polyfluoroalkyl Substances, Environ. Sci. Technol., 2021, 55(23), 15575–15578 CrossRef CAS PubMed.
- E. M. Sunderland, X. C. Hu, C. Dassuncao, A. K. Tokranov, C. C. Wagner and J. G. Allen, A review of the pathways of human exposure to poly- and perfluoroalkyl substances (PFASs) and present understanding of health effects, J. Exposure Sci. Environ. Epidemiol., 2019, 29(2), 131–147 CrossRef CAS PubMed.
- J. Glüge, M. Scheringer, I. T. Cousins, J. C. DeWitt, G. Goldenman and D. Herzke,
et al., An overview of the uses of per- and polyfluoroalkyl substances (PFAS), Environ. Sci.: Processes Impacts, 2020, 22(12), 2345–2373 RSC.
- A. O. De Silva, J. M. Armitage, T. A. Bruton, C. Dassuncao, W. Heiger-Bernays and X. C. Hu,
et al., PFAS Exposure Pathways for Humans and Wildlife: A Synthesis of Current Knowledge and Key Gaps in Understanding, Environ. Toxicol. Chem., 2021, 40(3), 57–631 CrossRef PubMed.
- L. Schultes, R. Vestergren, K. Volkova, E. Westberg, T. Jacobson and J. P. Benskin, Per- and polyfluoroalkyl substances and fluorine mass balance in cosmetic products from the Swedish market: implications for environmental emissions and human exposure, Environ. Sci.: Processes Impacts, 2018, 20(12), 1680–1690 RSC.
- K. J. Harris, G. Munoz, V. Woo, S. Sauvé and A. A. Rand, Targeted and Suspect Screening of Per- and Polyfluoroalkyl Substances in Cosmetics and Personal Care Products, Environ. Sci. Technol., 2022, 56(20), 14594–14604 CrossRef CAS PubMed.
- K. W. Pütz, S. Namazkar, M. Plassmann and J. P. Benskin, Are cosmetics a significant source of PFAS in Europe? product inventories, chemical characterization and emission estimates, Environ. Sci.: Processes Impacts, 2022, 24(10), 1697–1707 RSC.
- H. D. Whitehead, M. Venier, Y. Wu, E. Eastman, S. Urbanik and M. L. Diamond,
et al., Fluorinated Compounds in North American Cosmetics, Environ. Sci. Technol. Lett., 2021, 8(7), 538–544 CrossRef CAS.
- Y. Fujii, K. H. Harada and A. Koizumi, Occurrence of perfluorinated carboxylic acids (PFCAs) in personal care products and compounding agents, Chemosphere, 2013, 93(3), 538–544 CrossRef CAS PubMed.
- W. A. Gebbink, U. Berger and I. T. Cousins, Estimating human exposure to PFOS isomers and PFCA homologues: The relative importance of direct and indirect (precursor) exposure, Environ. Int., 2015, 74, 160–169 CrossRef CAS PubMed.
- G. Johanson, I. Gyllenhammar, C. Ekstrand, A. Pyko, Y. Xu and Y. Li,
et al., Quantitative relationships of perfluoroalkyl acids in drinking water associated with serum concentrations above background in adults living near contamination hotspots in Sweden, Environ. Res., 2023, 219, 115024 CrossRef CAS PubMed.
- S. Beesoon, S. J. Genuis, J. P. Benskin and J. W. Martin, Exceptionally High Serum Concentrations of Perfluorohexanesulfonate in a Canadian Family are Linked to Home Carpet Treatment Applications, Environ. Sci. Technol., 2012, 46(23), 12960–12967 CrossRef CAS PubMed.
- K. Winkens, J. Koponen, J. Schuster, M. Shoeib, R. Vestergren and U. Berger,
et al., Perfluoroalkyl acids and their precursors in indoor air sampled in children's bedrooms, Environ. Pollut., 2017, 222, 423–432 CrossRef CAS PubMed.
- O. Ragnarsdóttir, M. A. E. Abdallah and S. Harrad, Dermal uptake: An important pathway of human exposure to perfluoroalkyl substances?, Environ. Pollut., 2022, 307, 119478 CrossRef PubMed.
- S. Poothong, E. Papadopoulou, J. A. Padilla-Sánchez, C. Thomsen and L. S. Haug, Multiple pathways of human exposure to poly- and perfluoroalkyl substances (PFASs): From external exposure to human blood, Environ. Int., 2020, 134, 105244 CrossRef CAS PubMed.
- A. Colles, L. Bruckers, E. Den Hond, E. Govarts, B. Morrens and T. Schettgen,
et al., Perfluorinated substances in the Flemish population (Belgium): Levels and determinants of variability in exposure, Chemosphere, 2020, 242, 125250 CrossRef CAS PubMed.
- E. Thépaut, H. A. A. M. Dirven, L. S. Haug, B. Lindeman, S. Poothong and M. Andreassen,
et al., Per- and polyfluoroalkyl substances in serum and associations with food consumption and use of personal care products in the Norwegian biomonitoring study from the EU project EuroMix, Environ. Res., 2021, 195, 110795 CrossRef PubMed.
- G. Pawar, M. A. E. Abdallah, E. V. de Sáa and S. Harrad, Dermal bioaccessibility of flame retardants from indoor dust and the influence of topically applied cosmetics, J. Exposure Sci. Environ. Epidemiol., 2017, 27(1), 100–105 CrossRef CAS PubMed.
- O. Ragnarsdóttir, M. A. E. Abdallah and S. Harrad, Dermal bioaccessibility of perfluoroalkyl substances from household dust; influence of topically applied cosmetics, Environ. Res., 2023, 238, 117093 CrossRef PubMed.
- Y. Wu, G. Z. Miller, J. Gearhart, G. Peaslee and M. Venier, Side-chain fluorotelomer-based polymers in children car seats, Environ. Pollut., 2021, 268, 115477 CrossRef CAS PubMed.
- W. J. Fasano, G. L. Kennedy, B. Szostek, D. G. Farrar, R. J. Ward and L. Haroun,
et al., Penetration of Ammonium Perfluorooctanoate Through Rat and Human Skin In Vitro, Drug Chem. Toxicol., 2005, 28(1), 79–90 CrossRef CAS PubMed.
- J. Franko, B. J. Meade, H. F. Frasch, A. M. Barbero and S. E. Anderson, Dermal Penetration Potential of Perfluorooctanoic Acid (PFOA) in Human and Mouse Skin, J. Toxicol. Environ. Health, Part A, 2012, 75(1), 50–62 CrossRef CAS PubMed.
- J. H. Johansson, H. Yan, U. Berger and I. T. Cousins, Water-to-air transfer of branched and linear PFOA: Influence of pH, concentration and water type, Emerging Contam., 2017, 3(1), 46–53 CrossRef.
- L. Vierke, U. Berger and I. T. Cousins, Estimation of the Acid Dissociation Constant of Perfluoroalkyl Carboxylic Acids through an Experimental Investigation of their Water-to-Air Transport, Environ. Sci. Technol., 2013, 47(19), 11032–11039 CrossRef CAS PubMed.
- K. Abraham and B. H. Monien, Transdermal absorption of 13C4-perfluorooctanoic acid (13C4-PFOA) from a sunscreen in a male volunteer – What could be the contribution of cosmetics to the internal exposure of perfluoroalkyl substances (PFAS)?, Environ. Int., 2022, 169, 107549 CrossRef CAS PubMed.
- Q. Chen, S. Yi, Q. Ye, Y. Zhu, W. Zhong and L. Zhu, Insights into the Dermal Absorption, Deposition, and Elimination of Poly- and Perfluoroalkyl Substances in Rats: The Importance of Skin Exposure, Environ. Sci. Technol., 2022, 56(23), 16975–16984 CrossRef CAS PubMed.
- J. C. Kissel, I. A. Titaley, D. J. Muensterman and J. A. Field, Evaluating Neutral PFAS for Potential Dermal Absorption from the Gas Phase, Environ. Sci. Technol., 2023, 57(12), 4951–4958 CrossRef CAS PubMed.
- H. Ertl and W. Butte, Bioaccessibility of pesticides and polychlorinated biphenyls from house dust: in-vitro methods and human exposure assessment, J. Exposure Sci. Environ. Epidemiol., 2012, 22(6), 574–583 CrossRef CAS PubMed.
-
A. B. Stefaniak and C. J. Harvey, Artificial Skin Surface Film Liquids, US Pat., US20080311613A1, 2008, https://patents.google.com/patent/US20080311613A1/en-2008.
- S. Ullah, T. Alsberg and U. Berger, Simultaneous determination of perfluoroalkyl phosphonates, carboxylates, and sulfonates in drinking water, J. Chromatogr. A, 2011, 1218(37), 6388–6395 CrossRef CAS PubMed.
-
Government of Canada, Natural Health Products Ingredients Database, 2023, https://webprod.hc-sc.gc.ca/nhpid-bdipsn/search-rechercheReq.do Search PubMed.
- B. Zhu, W. Jiang, W. Wang, Y. Lin, T. Ruan and G. Jiang, Occurrence and Degradation Potential of Fluoroalkylsilane Substances as Precursors of Perfluoroalkyl Carboxylic Acids, Environ. Sci. Technol., 2019, 53(9), 4823–4831 CrossRef CAS PubMed.
|
This journal is © The Royal Society of Chemistry 2024 |