Variability in air quality, ozone formation potential by VOCs, and associated air pollution attributable health risks for Delhi's inhabitants†
Received
22nd May 2024
, Accepted 29th June 2024
First published on 15th July 2024
Abstract
The present long-term study has been conducted with dual objectives: firstly, to monitor the spatio-temporal variation of ambient air quality parameters and secondly, to evaluate the impact of air pollutants on the Delhi population. Five years (January 2019 to December 2023) of data of six key pollutants (PM10, PM2.5, NO2, O3, Benzene, and Toluene) were collected by continuous ambient air quality monitoring stations, obtained from the Central Pollution Control Board portal. The impact of air pollutants on human health was assessed using different indices and the AirQ+ model developed by the World Health Organization (WHO). Additionally, the ozone formation potential (OFP) of benzene and toluene was evaluated. The findings of the study revealed that the concentrations of PM10 and PM2.5 exceeded both national and global guidelines across all the sites throughout the study period. Notably, industrial sites were classified as the severe category according to the National Air Quality Index. At industrial sites, the OFP of volatile organic compounds (VOCs) was observed to be higher in comparison to commercial sites. The AirQ+ model analysis in the health risk assessment indicated a strong association between PM10 exposure and mortality from respiratory (91.36%) and chronic bronchitis (90.85%) diseases. Additionally, long-term PM2.5 exposure was linked to an increased risk of stroke (65%) and circulatory (63.83%) mortality.
Environmental significance
Long-term study to assess the impact of air pollution on human health in Delhi, the capital city of India (fastest emerging economy of the world). The concentrations of particulate matter (PM10 and PM2.5) exceeded the Indian and WHO ambient air quality standards. Concentrations of volatile organic compounds (benzene and toluene) were significant at the industrial sites. A health risk assessment using the AirQ+ model demonstrated a significant association between PM10 exposure and mortality, predominantly attributed to chronic bronchitis and respiratory diseases in the Delhi region.
|
1. Introduction
Exposure to environmental pollutants has been associated with chronic diseases and mortality, posing a major threat to public health.1,2 Recently, the prevalence of air pollution-associated diseases has increased on local, regional, and global scales. The majority of anthropogenic air pollutants are derived from vehicular and industrial emissions, fossil fuel combustion, mining activities, cooking fuels, and construction works.3,4 These sources form a complex mixture of toxic pollutants such as particulate matter (PM) and air pollutants including nitrogen dioxide, carbon monoxide, sulfur dioxide, and ozone. Several lines of evidence (epidemiological and clinical studies) have demonstrated that air pollutants exert deleterious effects on human health including systemic inflammation, elevated peripheral blood pressure, cardiovascular events, hypercoagulability, and may alter the cardiac autonomic nervous system.1,5–7 However, the exact mechanisms by which pollutants exert these effects are not fully understood.
Every year, air pollution accounts for 7 million deaths due to joint effects of ambient and household air pollution, impacting 99% of the global population living in areas that exceed World Health Organization guidelines.8,9 Particulate Matter (PM) serves as a key component of air pollution, categorized by its aerodynamic size. Fine particles such as PM2.5 possess the ability to penetrate deep into the respiratory system. Therefore, they contribute to a wide range of health effects including pulmonary and cardiovascular morbidity and mortality.10 Several studies all over the world have demonstrated that the particulate matter released by vehicles poses a significant risk to our environmental health.11 Factors such as high urban population, an increasing number of motor vehicles, traffic density, and vehicle characteristics are severely exacerbating the impact of atmospheric pollutants on the urban environments.12,13 In developing countries like India, solid fuels such as coal or biomass are the major fuel sources used for cooking and heating. These activities result in the emission of hazardous gases such as nitrogen oxide, sulphur oxides, carbon monoxide, ammonia, ozone, and volatile organic compounds (VOCs).14,15 In the atmosphere, VOCs play an important role in the formation of ozone (O3). BTEX (benzene, toluene, ethylbenzene, and xylene) react with atmospheric nitrate and hydroxyl radical to form aldehydes, peroxyacetyl nitrates (PAN), and secondary aerosols. BTEX has been identified as a hazardous substance by the United States Environmental Protection Agency (USEPA). Among them, benzene poses a significant concern to human health due to its carcinogenic properties.16
Particulate matter (PM10 and PM2.5) are indispensable criteria pollutants to assess the ambient air quality of any region and to calculate the Air Quality Index (AQI). Also, significant concentrations of particulate matter were observed in the city in past studies.17–20 Prominent sources of nitrogen dioxide in the ambient air are vehicular and industrial emissions and higher concentrations signify anthropogenic activities. Also, NO2 acts as a precursor of tropospheric ozone (ground-level ozone), which causes serious environmental and health hazards.21–27 Earlier studies have shown the adverse impact of benzene and toluene on human health.28 Therefore, these six air pollutants (PM10, PM2.5, NO2, O3, benzene, and toluene) were considered for the present study.
Delhi, the capital of India, has been declared as one of the most polluted city globally by the World Health Organization (WHO).29 Based on PM2.5 concentration, the latest IQ Air report has ranked Delhi as the most polluted capital city among 106 countries. Previous studies have identified the large transport sector, which has the highest vehicle stock in the country, as a major source of emissions.30 It has experienced dramatic growth in traffic density due to the increased rate of new vehicle production and extended industrial sectors, contributing as a primary source of ambient air quality in Delhi.31 Though, the Government has formulated and implemented a variety of measures to address pollution including compressed natural gas (CNG) for public transport vehicles, implementation of Bharat Stage (BS) norms for public and private vehicles, regular monitoring to check fuel adulteration, promotion of e-mobility, etc.18,32–36 The previously reported studies are fragmented and short-term with limited pollutants. Thus, the present long-term study aimed to investigate the impact of air pollution on human health in the Delhi region. To assess the risk, we have measured the six potent air pollutants at four different Delhi regions and predicted the health risk. These findings lend support to the view that air pollution is linked with serious harmful effects on human health which further contributes to the global burden of diseases.
2. Materials and method
2.1. Study region
Delhi city (28.61 °N and 77.23 °E, 216 m above mean sea level), the capital city of India is spread over 1484 sq. km and caters to over 32 million population. According to the Koppen classification, the city's climate is a humid subtropical climate with a dry winter (Cwa).37 The Indian Meteorological Department categorically divides Delhi's seasons into winter (January–February), pre-monsoon (March to May), summer-monsoon (June–September), and post-monsoon (October–December) seasons.25 The city witnesses extreme temperatures between winter (5 °C to 10 °C) and summer (45 °C or more) seasons. River Yamuna, Indo-Gangetic alluvial plains, Delhi Ridge (green cover), and Aravalli hill ranges are the geographical characteristics of the city, where around 21.86% of the geographical area is forest-covered. Delhi-National Capital Territory (Delhi-NCT), borders with the neighboring states (Uttar Pradesh, Haryana and Rajasthan), and is collectively known as Delhi-National Capital Region (Delhi-NCR). Researchers have earlier mentioned that the total road length in Delhi is 33
198 km with 864 signalized and 418 blinkers traffic intersections, forming 1749 km road density (road length per 100 km2). Delhi has different clusters of residential/institutional, industrial, and commercial establishments with other hotspots having high traffic interjections. The city has witnessed a continuous rise in vehicular density and different industrial units over the past few years (Tables ST1 and ST2†) (ESI†).20,31,38 Delhi comes among the most polluted cities in the world, being the most degraded urban air quality.39,40
2.2. Study period and parameters
In this long-term study, twenty-four-hour ambient air quality data of six pollutants (PM10, PM2.5, NO2, ozone (O3), benzene, and toluene) were downloaded from the Central Pollution Control Board (CPCB) portal (https://www.airquality.cpcb.gov.in/ccr/#/login) from January 01, 2019 to December 31, 2023 (sixty-months). The above-said data are being collected by real-time continuous ambient air quality monitoring stations (CAAQMS) established at different locations in the city by the Delhi Pollution Control Committee (DPCC). PUSA (S1) and Okhla Phase II (S4) are categorized as industrial sites as these sites have clusters of industrial units of various scales. National stadium (S2) lies in the central part of the city where various govt. organizations, departments, and establishments are situated. Similarly, Dwarka Sector 8 (S3) is a suburban area of the city that has observed tremendous infrastructural and commercial growth in the recent past. Sites S2 and S3 fall under the commercial category as these regions have residential and commercial complexes, education institutions, hospitals, govt. offices etc. All the selected sites for the present study witness dense traffic load, interjections, and large population density. The details of the sampling stations and studied parameters are given in Table 1. Ozone, benzene, and toluene were considered to assess the impact assessment on human health in the present study. The present study investigates the ozone formation potential of VOCs and assesses the health risks posed by the studied parameters on human health.
Table 1 Sampling stations and parametersa
Station code |
Station name |
Coordinates (DDS) |
Region |
PM10 (μg m−3) (BAM) |
PM2.5 (μg m−3) (BAM) |
NO2 (μg m−3) (chemiluminescence) |
O3 (μg m−3) |
Benzene (μg m−3) |
Toluene (μg m−3) |
DD – degree decimal; R – range; M – mean; SD – standard deviation; BAM – beta ray attenuation method.
|
S1 |
PUSA |
28.639645 N |
Industrial |
R: 46.94–394.13 |
R: 18.52–234.75 |
R: 12.34–83.65 |
R: 3.0–70.25 |
R: 0.34–11.73 |
R: 3.00–53.00 |
77.146263 E |
M: 207.11 |
M: 94.42 |
M: 49.54 |
M: 23.6 |
M: 4.43 |
M: 24.13 |
SD: 94 |
SD: 65.65 |
SD: 19.74 |
SD: 13.4 |
SD: 3.07 |
SD: 14.14 |
S2 |
National stadium |
28.611381 N |
Commercial |
R: 48.2–366.0 |
R: 23.35–242.6 |
R: 14.26–91.74 |
R: 10.18–65.21 |
R: 0.06–12.14 |
R: 1.03–58.21 |
77.237738 E |
M: 191.25 |
M: 95.13 |
M: 39.50 |
M: 25 |
M: 2.85 |
M: 12.44 |
SD: 83.40 |
SD: 62.6 |
SD: 19.3 |
SD: 12.5 |
SD: 2.75 |
SD: 12.33 |
S3 |
Dwarka sector 8 |
28.576552 N |
Commercial |
R: 60.76–456.34 |
R: 24.50–268 |
R: 13.23–76.85 |
R: 6–77. 5 |
R: 0.25–7.0 |
R: 1.51–45.80 |
77.076574 E |
M: 248.74 |
M: 104.6 |
M: 35 |
M: 28 |
M: 2.6 |
M: 14.53 |
SD: 104.72 |
SD: 70.3 |
SD: 16 |
SD: 15.7 |
SD: 1.92 |
SD: 13.11 |
S4 |
Okhla phase II |
28.530785 N |
Industrial |
R: 56.3–409.88 |
R: 20.24–260.26 |
R: 15.63–110.10 |
R: 14.34–54.61 |
R: 0.94–8.0 |
R: 11–103.68 |
77.271255 E |
M: 212.74 |
M: 99.85 |
M: 51.1 |
M: 29 |
M: 4.0 |
M: 38.44 |
SD: 95.38 |
SD: 71.37 |
SD: 22.74 |
SD: 10.0 |
SD: 1.92 |
SD: 19.13 |
PM10 and PM2.5 concentrations were analyzed by Beta Ray Attenuation Method (BAM 1020/Spirant BAM 1020; Met One, USA/Ecotech, Australia) while nitrogen dioxide (NO2) concentrations were analyzed with the Chemiluminescence method (Serinus 44; Ecotech Australia).20 It's important to note that Continuous Ambient Air Quality Monitoring Stations (CAAQMS) were temporarily closed for maintenance at various locations for several days throughout the study period. We have factored these closures into our calculations. AirQ+ software tool for health risk assessment of air pollution was downloaded from World Health Organization (WHO) (https://www.who.int/europe/tools-and-toolkits/airq---software-tool-for-health-risk-assessment-of-air-pollution). Origin Pro 2022 was used for graph plotting (Fig. 1).
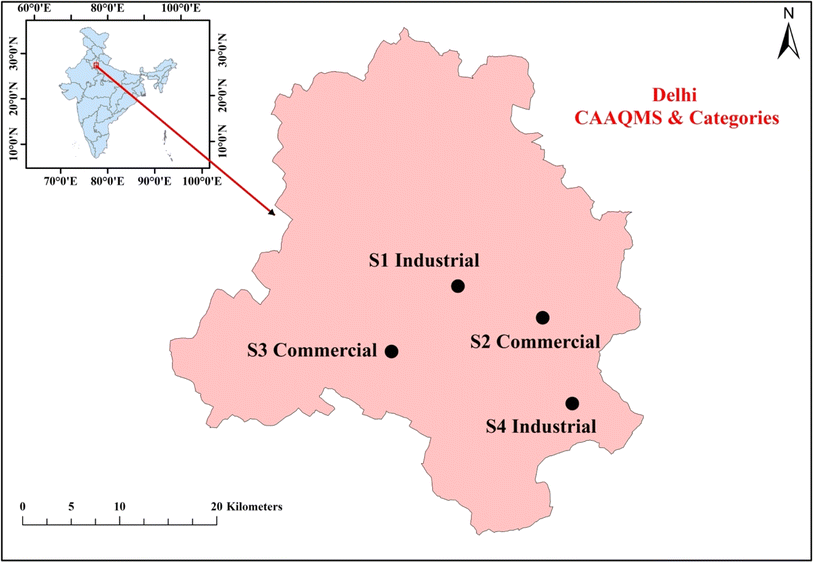 |
| Fig. 1 Continuous Ambient Air Quality Monitoring Stations (CAAQMS) and their categories. | |
2.3. Ozone formation potential
Volatile organic compounds (VOCs) are important reactants for photochemical smog and ozone formation. The reaction between the OH radical and VOCs produces aryl peroxy radicals, which react with NO to form NO2 and aryloxy radicals. Nitrogen dioxide acts as a precursor of ozone, contributing to the formation of O3 in the ambient atmosphere. Degradation of aryloxy radicals also helps in the formation of ozone. The ozone formation potential (OFP) has been calculated using the following equation:
OFP = MIR coefficient × C |
where, MIR is the maximum incremental reactivity scale and C is the ambient concentration (μg m−3) of VOC. The MIR coefficient of benzene and toluene are 0.42 and 2.7, respectively.41,42
2.4. Health risk assessment
2.4.1. Daily, lifetime exposure and integrated lifetime cancer risk (ILTCR).
Assessing the health risks associated with the pollutants under study is crucial for predicting potential health impacts resulting from elevated pollutant concentrations. Previous studies have demonstrated that air pollution may significantly contribute to the development of various diseases such as cardiovascular issues, stroke, respiratory ailments, and exacerbate existing co-morbidities. It has been documented that benzene exhibits a carcinogenic effect, whereas toluene and ozone are considered non-carcinogenic. The inhalation pathway has been considered to assess daily exposure of the human population:43,44
where, E is daily exposure (mg kg−1 d−1); C is exposed concentration of pollutant (mg m−3); IR is the inhalation rate (0.83 m3 h−1); ED is exposure duration (10 hours day−1) and BW is the average body weight of human body (70 kg).
Based on calculated daily exposed concentration (E), lifetime exposure concentration (EL) was obtained using the following equation:42,43
EL = E × (D/7) × (WK/52) × (YE/YL) |
where,
EL (mg kg
−1 d
−1) is lifetime exposure,
D is days per week exposure (7 days), WK is weeks of exposure (52 weeks), YE is years of exposure (15 years) and YL is years in lifetime (75 years).
Obtained values of EL were used to calculate the integrated life time cancer risk (ILTCR) for benzene using the equation given below:42
ILTCR = EL (mg kg−1 d−1) × CPF (mg kg−1 d−1) |
where, CPF is the carcinogenic potency factor (cancer slope factor). The CPF value for benzene is 0.0273.
45
As discussed elsewhere, the hazard quotient (HQ) for non-malignant conditions also depicts the risk assessment. If, HQ is more than 1, it falls under the category of “adverse noncarcinogenic effect of concern”. When HQ is equal to or less than 1, it is considered as “acceptable level”. The HQ may be calculated as:46
where,
CAVG is yearly average concentration of the pollutant (benzene and toluene) and R
fC is the reference concentration of the studied pollutants. The R
fC for benzene and toluene are 0.03 mg m
−3 and 5 mg m
−3, respectively.
2.4.2. Risk assessment using AirQ+ software tool.
AirQ+ model was used in the present study to compute the health impact assessment (attributed to PM2.5, PM10, NO2 and ozone), burden of disease (attributed to PM2.5) and risk analysis (attributed to carcinogenic evaluation of benzene) of the selected sites (S1, S2, S3 and S4). The software was designed and developed by WHO to quantify short-term and long-term effects of air pollutants on human health, concerning population of a particular region. The software estimated the attributable proportion, attributable cases per 100
000 population at risk, cut off and relative risk (RR). Relative risk estimates the magnitude of an association between exposure and disease. The RR is provided with 95% confidence interval in AirQ+ software. The attributable proportion (AP) described as the fraction of the health outcome, can be calculated using the following equation, as discussed elsewhere.47
where, RR = relative risk for the health endpoint and P = fraction of the population under exposure.
In the present study, the long-term effects of attributed pollutants were studied by choosing 100% population at risk in the AirQ+ tool. The input data include the average annual concentration of each selected pollutant, the total population of the site and respective area in sq. km. The output data analyzed include estimated attributed proportion in percentage and relative risk.
3. Results and discussion
3.1. Spatio-temporal variations of pollutants
Finer and coarse particulate matter, originating from natural (e.g., pollen grains and soil particles etc.) and anthropogenic sources (vehicular emissions; biomass, solid waste & fossil fuel burning; road dust etc.), not only adversely affect human health but also, they are the carrier of various hazardous pollutants and heavy metals.19,48,49 It has been well-established that nitrogen dioxide is emitted from various man-made sources (vehicular emissions, industries etc.) and is a precursor of various secondary pollutants, including tropospheric ozone and also imposes adverse impacts on human health.21,23,25,26 The highest and lowest average concentrations of coarser particulates (PM10) were recorded at S3 (248.74 ± 104.72 μg m−3) and S2 (191.25 ± 83.40 μg m−3), respectively. Similarly, the highest average concentration of PM2.5 was observed at S3 (104.6 ± 70.3 μg m−3) while the lowest concentration was 94.42 ± 65.65 μg m−3 at S1. Despite being an industrial and institutional site, the lowest concentration of PM2.5 at S1 (PUSA) could be attributed to the presence of green patches in the area. As anticipated, the highest concentrations of PM10 and PM2.5 at S3 (Dwarka Sector 8) clearly indicate intense anthropogenic activities and high traffic interjections in the sub-urban area and hence, S3 may be considered as a hotspot in terms of particulate matter concentration.20 The average concentrations of particulate matter [(PM10 212.74 ± 95.38 μg m−3) and (PM2.5 100 ± 71.37 μg m−3)] were found at significant levels at S4 due to a wide range of industrial and anthropogenic activities and high traffic movement. Seasonal variations of particulate matter showed a consistent trend during the entire period of sixty-months. The highest concentrations of PM10 and PM2.5 were observed during post-monsoon (October–December) and winter (January and February) seasons (Fig. 2). Garg and Gupta (2020) also recorded similar trend. The cold and dense air column in post-monsoon-winter season obstructs the vertical mixing and hence, pollutants get trapped in the lower atmosphere. The concentration of particulate matter started decreasing sharply from March to September (pre-monsoon and summer-monsoon) as wind flow, air circulation, vertical mixing and precipitation dilute and wash away the particulate matter concentration in the atmosphere.20 The average annual concentrations of coarse and fine particulate matter exceeded the national and WHO standards (Table ST3†). Therefore, particulate matter mean concentrations recorded in the present study depict poor air quality. Earlier also, higher concentrations of particulate matter were reported by different workers.17–19 According to a study by Khan et al. (2023), in 2019, the average annual concentration of PM2.5 was 108.2 ± 18.5 μg m−3, which decreased to 94.8 ± 19.2 μg m−3 in 2020, and then slightly increase to 105.7 ± 21.1 μg m−3 in 2021 across Delhi.50 In another study, investigation conducted by Chen et al. (2019), average annual concentration of PM2.5 during 2015–2018 over Delhi was 110–140 μg m−3 (the major source being the traffic and domestic sector). Similarly, the concentration of PM10 in Delhi was 217.6 ± 22.8 μg m−3 in 2019, 180.5 ± 28.4 μg m−3 in 2020 and 214.0 ± 28.6 μg m−3 in 2021.40 High concentration of particulate matter was observed during winter months (November to January) in all the selected years.50
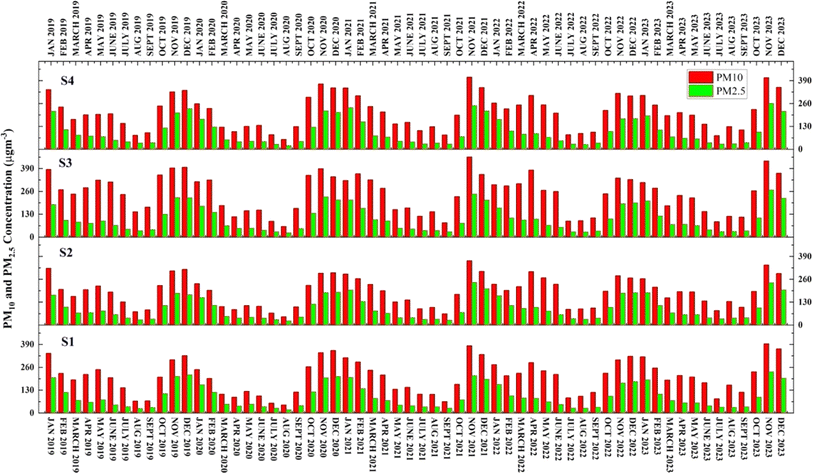 |
| Fig. 2 Concentration of PM10 and PM2.5 at different sites during the study period. | |
In this paper, we compared the levels of environmental pollutants (PM10, PM2.5, NO2, and O3) at four different sites in Delhi. It was found that PM2.5 and PM10 levels were higher at all four sites in comparison to NO2 and O3 as per the CPCB guidelines which may pose a significant risk for the Delhi population (Fig. 3 and 4). In this analysis, it is evident from the results that only 2% of the study duration meets the criteria for good air quality at site 1 and site 2 for PM10 levels which were completely absent at sites 3 and 4. All the sites showed more than 50% of air quality in the moderate category whereas around 25% in the poor air category in terms of PM10 concentration. Dwarka (S3) exhibited 17% and 3% PM10 levels in the very poor and severe category, respectively (Fig. 3). On the other hand, PM2.5 levels indicated that around 26% of air quality in the range of poor across all the sites throughout the observed period. The analysis revealed that sites S1, S3, and S4 have been classified under the severe PM2.5 air category (Fig. 3). Interestingly, NO2 and O3 levels were observed in the good and satisfactory category across the period at all four sites (Fig. 4).
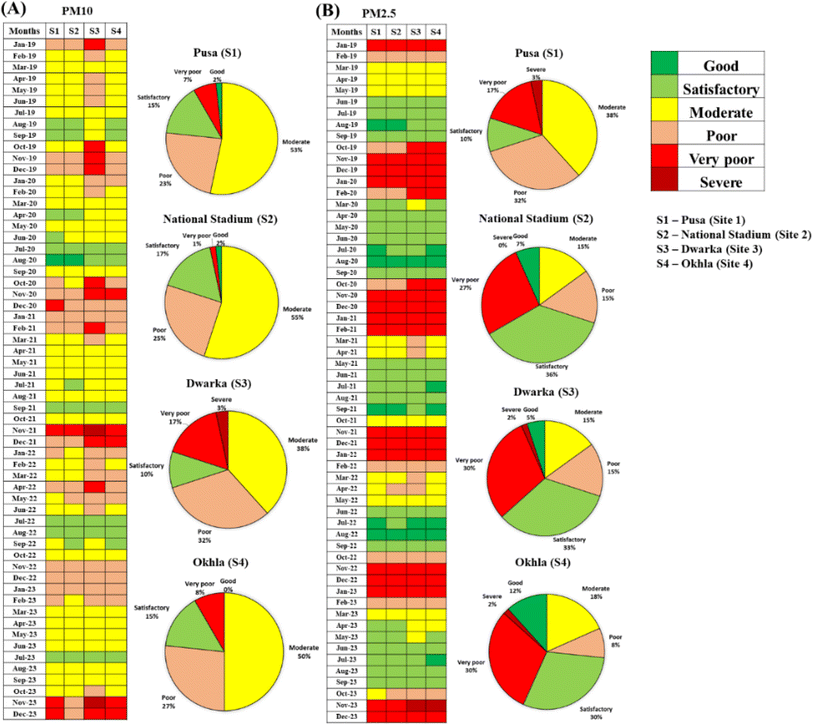 |
| Fig. 3 Distribution of PM10 (A) and PM2.5 (B) levels across the study period at four different sites in Delhi, India. Different colors represent the air quality color scaling of levels of environmental pollutants as per the Central Pollution Control Board (CPCB) guidelines. | |
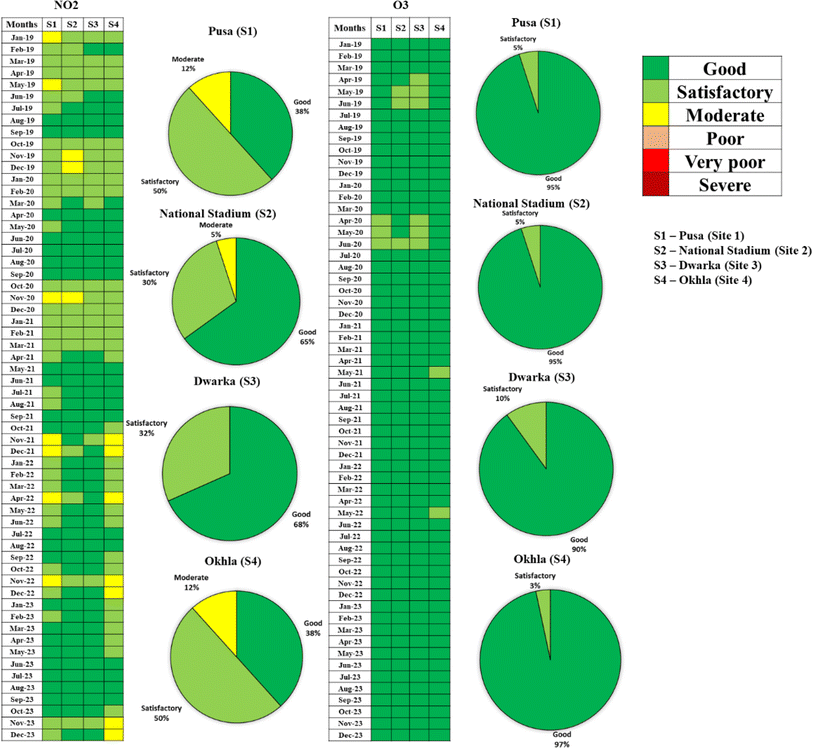 |
| Fig. 4 Distribution of NO2 and O3 levels across the study period at four different sites in Delhi, India. Different colors represent the air quality color scaling of levels of environmental pollutants as per the Central Pollution Control Board (CPCB) guidelines. | |
Emissions from the transportation sector and industries are the primary anthropogenic sources of nitrogen dioxide in the ambient atmosphere. The highest mean NO2 concentration was recorded at S4 (51.07 ± 22.74 μg m−3) followed by S1 (49.54 ± 19.74 μg m−3) and S2 (39.5 ± 19.3 μg m−3) and least mean concentration was observed at S3 (35 ± 16 μg m−3). Seasonal variations showed that NO2 concentrations were highest during post-monsoon and winter seasons (October–February) (due to obstructed vertical mixing and dense cold air column) followed by pre-monsoon – summer (dilution of pollutants due to air circulation) and monsoon seasons (March–September) (precipitation washes away the pollutants in the ambient atmosphere). Similar results have been reported by other researchers in the recent past. In a report by Khan et al. 2023, the annual average NOx level in Delhi was 51.9 ± 5.1 μg m−3 in 2019, 47.2 ± 8.1 μgm−3 in 2020, and 53.7 ± 8.2 μg m−3 in 2021.50 The highest concentration of NOx was observed in the month of December and January in all years except during lockdown years.
Tropospheric ozone is a serious pollutant and potential oxidant and may cause severe health impacts.22,27 The highest and lowest mean concentration of ozone was recorded at S4 (51.07 ± 22.74 μg m−3) and S1 (23.6 ± 13.4 μg m−3), respectively. Sites S2 and S3 also observed notable concentrations of tropospheric ozone. Nitrogen dioxide is a proven precursor of tropospheric ozone and hence may significantly contribute in the ground level ozone formation. However, concentration of ozone was found significantly higher as compared to corresponding NO2 concentrations at the studied sites during different time of the study period. This indicates that ozone must have originated from the primary sources and VOCs also contributed significantly in the ozone formation (secondary pollutant). The annual concentration of surface ozone in Delhi was reported to be 33.0 ± 3.0 μg m−3 in 2019, 34.5 ± 2.9 μg m−3 in 2019 and 30.1 ± 2.1 μg m−3 in 2021.50 The concentration of ozone was lower in winter months (November to January) and monsoon season (July to September) due to less insolation and cloud cover. The concentration started increasing from the month of March and reached maximum concentration during the pre-monsoon season (May and June). The value decreases in the monsoon season and was lowest during peak monsoon time. And after monsoon, surface ozone levels again started increases in month of October due to clear sky and high insolation.
Benzene (carcinogenic) and toluene (non-carcinogenic) are classic examples of volatile organic compounds (VOCs) that originate mainly from anthropogenic sources like petroleum products, fuel combustion, paint industries, etc. Benzene and toluene negatively affect human health and have significant ozone formation potential (OFP).51 Higher concentrations of benzene were observed at S1 (4.43 ± 3.07 μg m−3) and S4 (3.98 ± 1.92 μg m−3) while S2 (2.85 ± 2.75 μg m−3) and S3 (2.6 ± 1.92 μg m−3) had comparatively lower concentrations of benzene. Mean concentrations of benzene at all the study sites were lower than the national ambient air quality guidelines (5 μg m−3) given by CPCB. The highest toluene concentration was also recorded at S4 (38.44 ± 19.13 μg m−3) and S1 (24.13 ± 14.14 μg m−3) whereas lower concentrations were observed at S2 (12.44 ± 12.33 μg m−3) and S3 (14.53 ± 13.11 μg m−3). The concentration of toluene increased tremendously at S4 from April 2020 till December 2023. This depicts the rise in the intensity of anthropogenic sources of toluene at S4 (industrial site). This may also cause a rise in toluene OFP in the area. As with other air pollutants, the concentration of VOCs showed a decreasing seasonal trend i.e., highest in the post-monsoon-winter season (October–February), lower in pre-monsoon (March–May), and least in summer-monsoon (June–September). Benzene and toluene have higher residential periods, low atmospheric reactivity, and longer half-life (benzene 9.4 days; toluene 02 days).42,43 Therefore, even at lower concentrations, they are persistent in nature. In the present study, higher concentrations of VOCs were noticed from 2019–2022 but were significantly low during 2023.
3.2. Ozone formation potential (OFP)
In the present study, the ozone formation potential (OFP) of benzene and toluene has been calculated by using maximum incremental reactivity (MIR). Results revealed that toluene has higher OFP than benzene (Fig. 6A and B). Toluene OFP was recorded highest at S4 (industrial site) from April 2020 to December 2023. This was due to a significant increase in toluene concentration at S4 during the same period (Fig. 5). However, toluene OFP at S1 (industrial site) was highest during the initial phase (October 2019 to February 2020) of the study and reached to the second highest level following S4 during the later phase of the study. Almost similar trend was shown by benzene OFP graph (Fig. 6B) where OFP at S2 was highest in the initial phases but benzene OFP at S1 and S4 dominated nearly throughout the study period. As discussed earlier, benzene has a longer half-life and residential period but shows less atmospheric reactivity, even the lower concentration of benzene and toluene can significantly contribute to tropospheric ozone. Results revealed that the OFP for benzene in the present study was lower than that observed in Agra42 and Gorakhpur43 but higher OFP for toluene was recorded at both sites (Table 2).
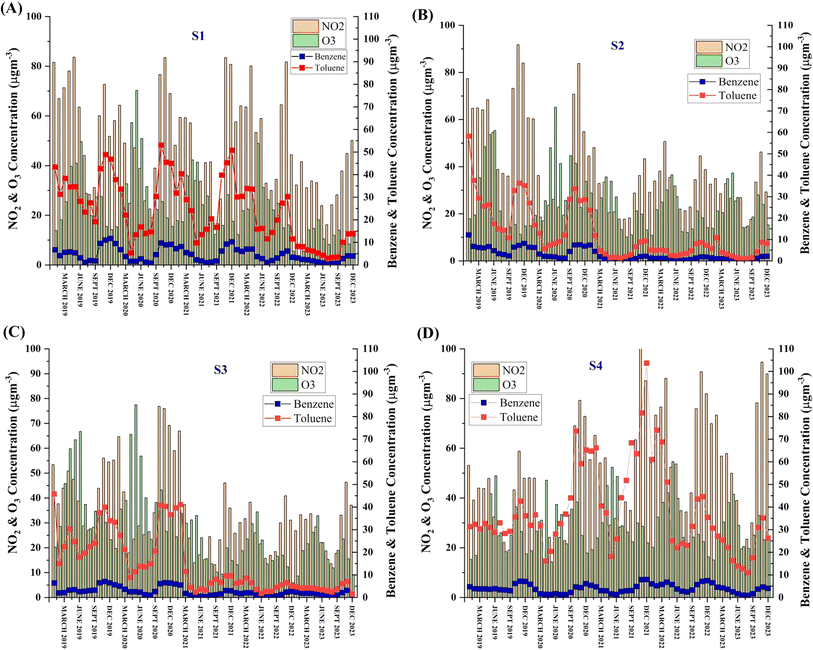 |
| Fig. 5 Concentration of gaseous pollutants (NO2 and O3) at four different sites of Delhi (S1, S2, S3 and S4). | |
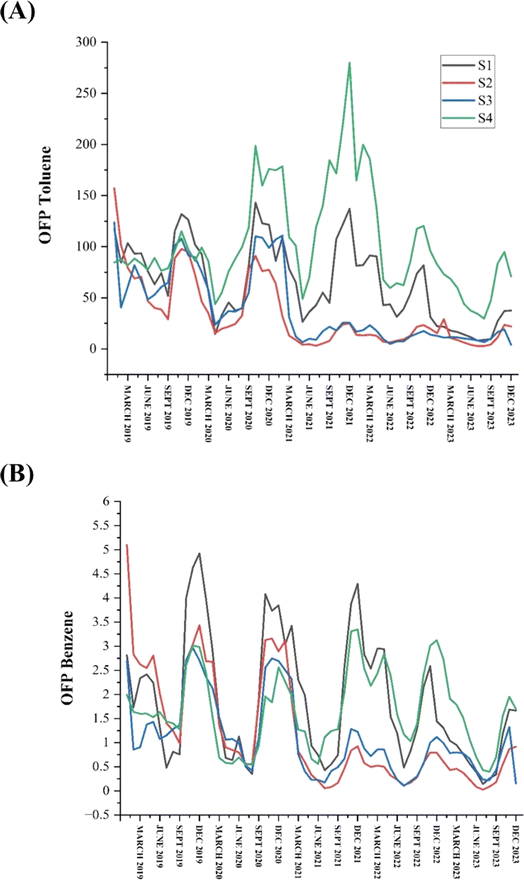 |
| Fig. 6 Ozone formation potential of toluene (A) and benzene (B). | |
Table 2 Comparative analysis of pollutant concentration, OFP, ILTCR and HQ
City |
Pollutant range |
OFP |
ILTCR (benzene) (×10−6) |
HQ benzene (×10−2) |
HQ toluene (×10−2) |
Ref. |
NO2 (μg m−3) |
PM10 (μg m−3) |
PM2.5 (μg m−3) |
O3 (μg m−3) |
Benzene (μg m−3) |
Toluene (μg m−3) |
Benzene |
Toluene |
Delhi (India) |
12.34–110.10 |
46.94–456.34 |
24.5–268 |
3–77.5 |
0.06–12.14 |
1.03–103.68 |
0.03–5.1 |
2.78–280 |
1.7–2.93 |
8–31 |
0.24–0.76 |
Present study |
Agra (India) |
— |
— |
— |
— |
33.4 |
42.4 |
8.1 |
103.9 |
12.5 |
68 |
0.8 |
Kumari et al., 2021 (ref. 42) |
Gorakhpur (India) |
— |
— |
— |
— |
2.6–73.7 |
8.28–108.24 |
4.9–20.1 |
75.7–149.8 |
19 |
97 |
0.7 |
Masih et al. 2016 (ref. 43) |
Zahedan city, Iran |
— |
— |
— |
— |
5.75–2622.83 |
19.97–4025.90 |
— |
— |
— |
0.2153 |
1.397 |
Kamani et al., 2023 (ref. 51) |
Eskişehir, Turkey |
30.9 |
— |
— |
89.82 |
1.23 |
6.11 |
— |
— |
— |
0.88–1.7 |
< 1 |
Demirel et al., 2014 (ref. 52) |
Tehran, Iran |
— |
— |
— |
— |
3.4–25.5 |
10–85.6 |
— |
— |
0.013–0.54 |
0.113–0.871 |
0.002–0.017 |
Yousefian et al., 2020 (ref. 53) |
Significantly higher concentration of ground-level ozone at S1 and S4, followed by S2 shows a good agreement with the OFP results (Fig. 5). This clearly shows that a rise in VOCs concentration increases the ground-level ozone concentration, which may eventually cause an upsurge in the dual exposure (VOC and ozone) linked health risk.
3.3. Health risk assessment
As previously discussed, exposure to tropospheric ozone can have detrimental impacts on human health. The highest effective lifetime exposure of ozone was found at sites S3 (0.64 × 10−4) and S4 (0.64 × 10−4), whereas significantly lower values were observed at sites S2 (0.46 × 10−4) and S1 (0.48 × 10−4). In this study, we also calculated the lifetime exposure for VOCs. As expected, the effective lifetime exposure value for benzene was significantly high at industrial sites S4 (0.92 × 10−4) and S1 (0.88 × 10−4). Similarly, the highest values of effective lifetime exposure values for toluene were observed at the S4 (8.6 × 10−4) and S1 (5.4 × 10−4) sites. In the present study, we also assessed the potential carcinogenic (CR) and non-carcinogenic risks associated with benzene and toluene. This study found that the highest mean integrated lifetime cancer risk (ILTCR) for benzene was at S1 (2.93 × 10−6), followed by S4 (2.62 × 10−6) and S2 (2.42 × 10−6) while the least mean value of ILTCR was found at S3 (1.7 × 10−6). The HQ was in the acceptable range throughout the study for benzene and toluene. The highest and lowest mean HQ benzene values were found at S4 (0.31) and S3 (0.085) respectively. Similarly, the highest and lowest mean HQ toluene values were observed at S4 (0.76 × 10−2) and S2 (0.24 × 10−2) respectively. The HQ values observed for benzene and toluene at all sites during the study period were comparatively lower than in other studies.28,42 A significant level of HQ benzene was observed at Gorakhpur while lower ranges were recorded in different studies conducted in Iran and Egypt (Table 2). These high levels may pose an effect on the health of commuters and people residing near this area.
3.4. Health risk assessment using AirQ+ tool
In this study, we found that levels of PM2.5 and PM10 were higher at all four sites in Delhi than NO2 and O3. Therefore, the health impact assessment of PM2.5 and PM10 was performed using the World Health Organization (WHO) AirQ+ software to estimate the health risk. The death rate data in the city was obtained from the govt. report.54 The health impact assessment was carried out by evaluating the mortality rate due to the burden of diseases with the effect of air pollutants. The estimated attributable proportion of mortality with long-term exposure to PM2.5 and PM10 from 2019 to 2023 is shown in Table 3a and b.
Table 3 (a) Health impact assessment of PM2.5 in Delhi using the AirQ+ model. (b) Health impact assessment of PM10 in Delhi using the AirQ+ model
(a) |
Impact assessment (PM2.5) |
2019 |
2020 |
2021 |
2022 |
Mortality |
Estimated attributable proportion |
Relative risk (RR) |
Estimated attributable proportion |
Relative risk (RR) |
Estimated attributable proportion |
Relative risk (RR) |
Estimated attributable proportion |
Relative risk (RR) |
Central% (lower%–upper%) |
Central (RR) (lower–upper) |
Central% (lower%–upper%) |
Central (RR) (lower–upper) |
Central% (lower%–upper%) |
Central (RR) (lower–upper) |
Central% (lower%–upper%) |
Central (RR) (lower–upper) |
Lung cancer (LC), acute lower respiratory infection (ALRI), chronic obstructive pulmonary disease (COPD), ischaemic heart disease (IHD), non-accidental deaths (NCDs + ALRI). |
Natural causes (age 30+ years) |
52.78% (43.34–56.84%) |
2.12 (1.76–2.32) |
49.02% (39.96–52.97%) |
1.96 (1.67–2.13) |
52.73% (43.29–56.79%) |
2.12 (1.76–2.31) |
49.92% (40.76–53.90%) |
2.00 (1.69–2.17) |
Circulatory (age 30+ years) |
63.85% (56.84–72.12%) |
2.77 (2.32–3.59) |
59.90% (52.97–68.25%) |
2.49 (2.13–3.15) |
63.80% (56.79–72.08%) |
2.76 (2.31–3.58) |
60.85% (53.90–69.19%) |
2.55 (2.17–3.25) |
Respiratory (age 30+ years) |
60.51% (25.04–80.08%) |
2.53 (1.33–5.02) |
56.59% (22.80–76.52%) |
2.30 (1.30–4.26) |
60.46% (25.01–80.04%) |
2.53 (1.33–5.01) |
57.53% (23.32–77.40%) |
2.35 (1.30–4.42) |
LC |
33.61% (25.09–41.21%) |
1.51 (1.34–1.70) |
32.98% (24.77–40.42%) |
1.49 (1.33–1.68) |
33.60% (25.09–41.21%) |
1.51 (1.33–1.70) |
33.15% (24.76–40.65%) |
1.50 (1.33–1.68) |
ALRI |
37.63% (27.60–46.88%) |
1.60 (1.38–1.88) |
35.63% (25.71–45.04%) |
1.55 (1.35–1.82) |
37.60% (27.58–46.86%) |
1.60 (1.38–1.88) |
36.11% (26.15–45.54%) |
1.57 (1.35–1.84) |
COPD |
45.56% (38.42–52.82%) |
1.84 (1.62–2.12) |
43.30% (36.09–50.55%) |
1.76 (1.56–2.02) |
45.53% (38.39–52.80%) |
1.84 (1.62–2.12) |
43.84% (36.65–51.14%) |
1.78 (1.58–2.05) |
IHD |
56.16% (45.56–64.54%) |
2.28 (1.84–2.82) |
55.98% (45.55–64.50%) |
2.27 (1.84–2.82) |
56.16% (45.56–64.54%) |
2.28 (1.84–2.82) |
56.06% (45.55–64.56%) |
2.55 (2.28–2.82) |
Stroke |
65.00% (54.83–72.32%) |
2.86 (2.21–3.61) |
64.83% (54.52–72.11%) |
2.84 (2.20–3.59) |
65.00% (54.83–72.32%) |
2.86 (2.21–3.61) |
64.91% (54.69–72.23%) |
2.85 (2.21–3.60) |
(NCDs + ALRI) |
41.70% (33.36–48.99%) |
1.72 (1.50–1.96) |
40.05% (31.95–47.19%) |
1.67 (1.47–1.89) |
41.68% (33.35–48.97%) |
1.71 (1.50–1.96) |
40.46% (32.30–47.63%) |
1.68 (1.48–1.91) |
(b) |
Impact assessment (PM10) |
2019 |
2020 |
2021 |
2022 |
Mortality |
Estimated attributable proportion |
Relative risk (RR) |
Estimated attributable proportion |
Relative risk (RR) |
Estimated attributable proportion |
Relative risk (RR) |
Estimated attributable proportion |
Relative risk (RR) |
Central% (lower%–upper%) |
Central (RR) (lower–upper) |
Central% (lower%–upper%) |
Central (RR) (lower–upper) |
Central% (lower%–upper%) |
Central (RR) (lower–upper) |
Central% (lower%–upper%) |
Central (RR) (lower–upper) |
Chronic bronchitis |
90.85% (57.16–97.63%) |
10.93 (2.33–42.15) |
84.66% (48.55–94.68%) |
6.52 (1.94–18.78) |
89.63% (55.22–97.12%) |
9.64 (2.23–34.66) |
90.04% (55.85–97.29%) |
10.04 (2.26–36.90) |
Post neonatal infant |
57.16% (34.82–76.83%) |
2.33 (1.53–4.32) |
48.55% (28.50–68.22%) |
1.94 (1.40–3.15) |
55.22% (33.34–74.99%) |
2.23 (1.50–4.00) |
55.85% (33.82–75.59%) |
2.26 (1.51–4.10) |
Bronchitis in children |
81.05% (0.00–97.67%) |
5.28 (1.00–42.93) |
72.85% (0.00–94.75%) |
3.68 (1.00–19.05) |
79.33% (0.00–97.16%) |
4.84 (1.00–35.27 |
79.89% (0.00–97.34%) |
4.97 (1.00–37.55) |
Natural causes |
57.16% (47.21–71.61%) |
2.33 (1.89–3.52) |
48.55% (39.40–62.74%) |
1.94 (1.65–2.68) |
55.22% (45.42–69.68%) |
2.23 (1.83–3.30) |
55.85% (46.00–70.31%) |
2.26 (1.85–3.37) |
IHD |
71.61% (19.35–87.25%) |
3.52 (1.24–7.84) |
62.74% (15.51–80.11%) |
2.68 (1.18–5.03) |
69.68% (18.44–85.80%) |
3.30 (1.23–7.04) |
70.31% (18.73–86.28%) |
3.37 (1.23–7.29) |
Respiratory |
91.36% (71.61–97.67%) |
11.58 (3.52–42.93) |
85.34% (62.74–94.75%) |
6.82 (2.68–19.05) |
90.18% (69.68–97.16%) |
10.19 (3.30–35.27) |
90.58% (70.31–97.34%) |
10.61 (3.37–37.55) |
LC |
81.05% (57.16–92.87%) |
5.28 (2.33–14.03) |
72.85% (48.55–87.39%) |
3.68 (1.94–7.93) |
79.33% (55.22–91.82%) |
4.84 (2.23–12.22) |
79.89% (55.85–92.17%) |
4.97 (2.26–12.77) |
Table 3a and b represents the impact assessment associated with long-term exposure to different concentrations of PM2.5 (Table 3a) and PM10 (Table 3b) among the Delhi population from 2019 to 2023. In this health assessment, it was observed that PM2.5 exposure was linked to a higher risk of stroke-related mortality (65%, RR-2.86), followed by circulatory (63.85%, RR-2.77), respiratory (60.51%, RR-2.53), ischemic heart disease (IHD, 56.16%, RR-2.28), natural causes (52.78%, RR-2.12), chronic obstructive pulmonary disease (COPD, 45.56%, RR-1.84), non-accidental deaths (NCDs + ALRI, 41.70%, RR-1.72), acute lower respiratory infection (ALRI, 37.63%), and lung cancer (LC, 33.61%, RR-1.51) consistently in Delhi throughout the study period. On the other hand, exposure to PM10 is strongly associated with mortality related to respiratory issues (91.36%, RR-11.58) and chronic bronchitis (90.85%, RR-10.93), followed by lung cancer (81.05%, RR-5.28), and bronchitis in children (81.05%, RR-5.28). These results are consistent with other studies.55,56
In the Burden of disease analysis of PM2.5, we observed a consistent trend similar to the impact assessment of PM2.5 (Table ST4†). We estimated that long-term exposure to ambient PM2.5 contributed to stroke followed by IHD, COPD, and ALRI respectively.57 has also estimated a similar trend with long-term exposure to PM2.5 and showed 36.2% of mortality from cerebrovascular disease (stroke), followed by ischemic heart disease (IHD), lung cancer (LC), chronic obstructive pulmonary disease (COPD), and acute lower respiratory infection (ALRI).
The strengths of this study were that all air pollution data have been collected from trusted government organization (CPCB). This study is a long-term study (2019–2023) that contains four air pollution monitoring sites in Delhi with six air pollutants (PM2.5, PM10, NO2, O3, benzene, toluene) that provide a better demonstration of the effects of air pollution in comparison to previous studies. This study has some limitations. In this study, we used four monitoring stations in specific urban locations which represent the total exposure of people to pollutants and the impact can be a result of the cumulative effects of pollutants rather than individual pollutants. This study also failed to explain the exact mechanism by which pollutants contribute to the development of diseases.
4. Conclusion
Five-year data, collected by real-time continuous ambient air quality monitoring stations (CAAQMS) and obtained from the CPCB portal were used for the present long-term study to assess the impact of air pollution on human health in Delhi, the capital city of India. Results revealed that out of all the pollutants, the concentrations of particulate matter (PM10 and PM2.5) exceeded the Indian and WHO ambient air quality standards. As expected, the highest concentrations of the pollutants were recorded in post-monsoon and winter seasons, due to the calm atmosphere and hindrance in vertical mixing. The concentrations of volatile organic compounds (benzene and toluene) were significant at the industrial sites. Higher ILTCR value was observed at S3 which is a cause of concern for the local population. Health risk assessment using AirQ+ model demonstrated a significant association between PM10 exposure and mortality, predominantly attributed to chronic bronchitis and respiratory diseases in Delhi region.
Declaration of generative AI and AI-assisted technologies in the writing process
During the preparation of this work the author(s) have not used any AI tools.
Data availability
The data sets for research were downloaded from the Central Pollution Control Board portal (https://airquality.cpcb.gov.in/ccr/), an open access platform.
Author contributions
Dr Saurabh Sharma: manuscript writing, review and editing, data curation and analysis, figures and graph preparation, graphical abstract preparation. Dr Anjum Singhal: graphical abstract preparation, AirQ+ analysis and manuscript review. Dr V. Venkat Ramanan: conceptualization, investigation, and manuscript review. Ms Pawan Verma: data handling, data curation, analysis and manuscript review. Dr Mayank Pandey: conceptualization, manuscript writing, review and editing, data curation, analysis, map and graph preparation.
Conflicts of interest
The authors declare that they have no known competing financial interests or personal relationships that could have appeared to influence the work reported in this paper.
Acknowledgements
Authors did not get any funding from any govt. or non-govt. agency/institution for the present study. The authors are thankful to Central Pollution Control Board for providing necessary data and information to conduct this study. Authors are grateful to P. G. D. A. V. College (Evening) and IGNOU for providing necessary infrastructure for successfully completing this research work.
References
- I. Manisalidis, E. Stavropoulou, A. Stavropoulos and E. Bezirtzoglou, Environmental and Health Impacts of Air Pollution: A Review, Front. Public Health, 2020, 8, 14 CrossRef PubMed.
- S. S. Shetty, D. D, H. S, S. Sonkusare, P. B. Naik, S. Kumari N and H. Madhyastha, Environmental pollutants and their effects on human health, Heliyon, 2023, 9, e19496 CrossRef CAS PubMed.
- F. Perera, Pollution from Fossil-Fuel Combustion is the Leading Environmental Threat to Global Pediatric Health and Equity: Solutions Exist, Int. J. Environ. Res. Public Health, 2018, 15, 16 CrossRef PubMed.
-
F. Popescu, I. Ionel, F. Popescu and I. Ionel, in Air Quality, IntechOpen, 2010 Search PubMed.
- A. Ghorani-Azam, B. Riahi-Zanjani and M. Balali-Mood, Effects of air pollution on human health and practical measures for prevention in Iran, J. Res. Med. Sci., 2016, 21, 65 CrossRef PubMed.
- J. G. F. Hogervorst, N. Madhloum, N. D. Saenen, B. G. Janssen, J. Penders, C. Vanpoucke, I. De Vivo, K. Vrijens and T. S. Nawrot, Prenatal particulate air pollution exposure and cord blood homocysteine in newborns: Results from the ENVIRONAGE birth cohort, Environ. Res., 2019, 168, 507–513 CrossRef CAS PubMed.
- K.-H. Kim, S. A. Jahan and E. Kabir, A review on human health perspective of air pollution with respect to allergies and asthma, Environ. Int., 2013, 59, 41–52 CrossRef CAS PubMed.
- W. Hill, E. L. Lim, C. E. Weeden, C. Lee, M. Augustine, K. Chen, F.-C. Kuan, F. Marongiu, E. J. Evans, D. A. Moore, F. S. Rodrigues, O. Pich, B. Bakker, H. Cha, R. Myers, F. van Maldegem, J. Boumelha, S. Veeriah, A. Rowan, C. Naceur-Lombardelli, T. Karasaki, M. Sivakumar, S. De, D. R. Caswell, A. Nagano, J. R. M. Black, C. Martínez-Ruiz, M. H. Ryu, R. D. Huff, S. Li, M.-J. Favé, A. Magness, A. Suárez-Bonnet, S. L. Priestnall, M. Lüchtenborg, K. Lavelle, J. Pethick, S. Hardy, F. E. McRonald, M.-H. Lin, C. I. Troccoli, M. Ghosh, Y. E. Miller, D. T. Merrick, R. L. Keith, M. Al Bakir, C. Bailey, M. S. Hill, L. H. Saal, Y. Chen, A. M. George, C. Abbosh, N. Kanu, S.-H. Lee, N. McGranahan, C. D. Berg, P. Sasieni, R. Houlston, C. Turnbull, S. Lam, P. Awadalla, E. Grönroos, J. Downward, T. Jacks, C. Carlsten, I. Malanchi, A. Hackshaw, K. Litchfield, J. DeGregori, M. Jamal-Hanjani and C. Swanton, Lung adenocarcinoma promotion by air pollutants, Nature, 2023, 616, 159–167 CrossRef CAS PubMed.
- S. Philip, R. V. Martin, G. Snider, C. L. Weagle, A. van Donkelaar, M. Brauer, D. K. Henze, Z. Klimont, C. Venkataraman, S. K. Guttikunda and Q. Zhang, Anthropogenic fugitive, combustion and industrial dust is a significant, underrepresented fine particulate matter source in global atmospheric models, Environ. Res. Lett., 2017, 12, 044018 CrossRef.
- P. Thangavel, D. Park and Y.-C. Lee, Recent Insights into Particulate Matter (PM2.5)-Mediated Toxicity in Humans: An Overview, Int. J. Environ. Res. Public Health, 2022, 19, 7511 CrossRef CAS PubMed.
- K. Zhang and S. Batterman, Air pollution and health risks due to vehicle traffic, Sci. Total Environ., 2013, 307–316 CrossRef CAS PubMed.
- A. Kumar and R. K. Mishra, Human health risk assessment of major air pollutants at transport corridors of Delhi, India, J. Transp. Health, 2018, 10, 132–143 CrossRef.
- R. K. Mishra, A. Pandey, G. Pandey and A. Kumar, The effect of odd-even driving scheme on PM2.5 and PM1.0 emission, Transp. Res. D, 2019, 67, 541–552 CrossRef.
- P. Chankapure, P. Doggali, K. Motghare, S. Waghmare, S. Rayalu, Y. Teraoka and N. Labhsetwar, Coal and Biomass Based Fuels in Rural India: Emissions and Possibility of Their Control, J. Novel Carbon Resour. Sci., 2011, 4, 8–12 Search PubMed.
- I. Mohapatra, S. C. Das and S. Samantaray, Health impact on women using solid cooking fuels in rural area of Cuttack district, Odisha, J. Family Med. Prim. Care, 2018, 7, 11–15 CrossRef PubMed.
- L. Falzone, A. Marconi, C. Loreto, S. Franco, D. A. Spandidos and M. Libra, Occupational exposure to carcinogens: Benzene, pesticides and fibers (Review), Mol. Med. Rep., 2016, 14, 4467–4474 CrossRef CAS PubMed.
- G. Beig, D. M. Chate, S. D. Ghude, A. S. Mahajan, R. Srinivas, K. Ali, S. K. Sahu, N. Parkhi, D. Surendran and H. R. Trimbake, Quantifying the effect of air quality control measures during the 2010 Commonwealth Games at Delhi, India, Atmos. Environ., 2013, 80, 455–463 CrossRef CAS.
- S. K. Goyal, S. V. Ghatge, P. Nema and S. M. Tamhane, Understanding urban vehicular pollution problem vis-a-vis ambient air quality-case study of a megacity (Delhi, India), Environ. Monit. Assess., 2006, 119, 557–569 CrossRef CAS PubMed.
- S. M. L. Hama, P. Kumar, R. M. Harrison, W. J. Bloss, M. Khare, S. Mishra, A. Namdeo, R. Sokhi, P. Goodman and C. Sharma, Four-year assessment of ambient particulate matter and trace gases in the Delhi-NCR region of India, Sustain. Cities Soc., 2020, 54, 102003 CrossRef.
- M. Pandey, M. P. George, R. K. Gupta, D. Gusain and A. Dwivedi, Impact of COVID-19 induced lockdown and unlock down phases on the ambient air quality of Delhi, capital city of India, Urban Clim., 2021, 39, 100945 CrossRef PubMed.
- B. Bishoi, A. Prakash and V. K. Jain, A Comparative Study of Air Quality Index Based on Factor Analysis and US-EPA Methods for an Urban Environment, Aerosol Air Qual. Res., 2009, 9, 1–17 CrossRef CAS.
- J. Currie, M. Neidell and J. F. Schmieder, Air pollution and infant health: Lessons from New Jersey, J. Health Econ., 2009, 28, 688–703 CrossRef PubMed.
- Y. Gao, S. Wang, C. Zhang, C. Xing, W. Tan, H. Wu, X. Niu and C. Liu, Assessing the impact of urban form and urbanization process on tropospheric nitrogen dioxide pollution in the Yangtze River Delta, China, Environ. Pollut., 2023, 336, 122436 CrossRef CAS PubMed.
- A. Garg and N. C. Gupta, The Great Smog Month and Spatial and Monthly Variation in Air Quality in Ambient Air in Delhi, India, J. Health Pollut., 2020, 10, 200910 CrossRef PubMed.
- D. Ghosh, S. Lal and U. Sarkar, Variability of tropospheric columnar NO2 and SO2 over eastern Indo-Gangetic Plain and impact of meteorology, Air Qual., Atmos. Health, 2017, 10, 565–574 CrossRef CAS.
- A. Kumar and P. Goyal, Forecasting of air quality in Delhi using principal component regression technique, Atmos. Pollut. Res., 2011, 2, 436–444 CrossRef CAS.
- D. Kong, J. Liang and C. Liu, Invisible enemy: The health impact of ozone, China Econ. Rev., 2022, 72, 101760 CrossRef.
- A. Garg and N. C. Gupta, A comprehensive study on spatio-temporal distribution, health risk assessment and ozone formation potential of BTEX emissions in ambient air of Delhi, India, Sci. Total
Environ., 2019, 659, 1090–1099 CrossRef CAS PubMed.
- A. Garg, A. Kumar and N. C. Gupta, Comprehensive study on impact assessment of lockdown on overall ambient air quality amid COVID-19 in Delhi and its NCR, India, J. Hazard. Mater. Lett., 2021, 2, 100010 CrossRef CAS PubMed.
- A. Dutta and W. Jinsart, Air pollution in Delhi, India: It's status and association with respiratory diseases, PLoS One, 2022, 17, e0274444 CrossRef CAS PubMed.
- P. Kumar, S. Gulia, R. M. Harrison and M. Khare, The influence of odd–even car trial on fine and coarse particles in Delhi, Environ. Pollut., 2017, 225, 20–30 CrossRef CAS PubMed.
- V. P. Aneja, A. Agarwal, P. A. Roelle, S. B. Phillips, Q. Tong, N. Watkins and R. Yablonsky, Measurements and analysis of criteria pollutants in New Delhi, India, Environ. Int., 2001, 27, 35–42 CrossRef CAS PubMed.
- A. B. Chelani and S. Devotta, Air quality assessment in Delhi: before and after CNG as fuel, Environ. Monit. Assess., 2007, 125, 257–263 CrossRef CAS PubMed.
- C. N. H. Doll and O. Balaban, A methodology for evaluating environmental co-benefits in the transport sector: application to the Delhi metro, J. Cleaner Prod., 2013, 58, 61–73 CrossRef.
- P. S. Khillare, T. Agarwal and V. Shridhar, Impact of CNG implementation on PAHs concentration in the ambient air of Delhi: A comparative assessment of pre- and post-CNG scenario, Environ. Monit. Assess., 2008, 147, 223–233 CrossRef CAS PubMed.
- K. Ravindra, E. Wauters, S. K. Tyagi, S. Mor and R. Van Grieken, Assessment of air quality after the implementation of compressed natural gas (CNG) as fuel in public transport in Delhi, India, Environ. Monit. Assess., 2006, 115, 405–417 CrossRef CAS PubMed.
- D. Mazzeo, N. Matera, P. De Luca, C. Baglivo, P. Maria Congedo and G. Oliveti, Worldwide geographical mapping and optimization of stand-alone and grid-connected hybrid renewable system techno-economic performance across Köppen-Geiger climates, Appl. Energy, 2020, 276, 115507 CrossRef.
- Economic Survey of Delhi 2022-23, Planning Department, https://delhiplanning.delhi.gov.in/planning/economic-survey-delhi-2022-23, accessed 2 July 2024.
- P. Balyan, C. Ghosh, A. K. Sharma and B. D. Banerjee, Health Effects of Air Pollution among Residents of Delhi: A Systematic Review, Int. J. Health Sci., 2018, 8, 273–282 Search PubMed.
- Y. Chen, O. Wild, E. Ryan, S. K. Sahu, D. Lowe, S. Archer-Nicholls, Y. Wang, G. McFiggans, T. Ansari, V. Singh, R. S. Sokhi, A. Archibald and G. Beig, Mitigation of PM2.5 and ozone pollution in Delhi: a sensitivity study during the pre-monsoon period, Atmos. Chem. Phys., 2020, 20, 499–514 CrossRef CAS.
- W. P. L. Carter, Development of Ozone Reactivity Scales for Volatile Organic Compounds, Air Waste, 1994, 44, 881–899 CrossRef CAS.
- S. Kumari, N. Baghel, A. Lakhani and K. M. Kumari, BTEX and formaldehyde levels at a suburban site of Agra: Temporal variation, ozone formation potential and health risk assessment, Urban Clim., 2021, 40, 100997 CrossRef.
- A. Masih, A. S. Lall, A. Taneja and R. Singhvi, Inhalation exposure and related health risks of BTEX in ambient air at different microenvironments of a terai zone in north India, Atmos. Environ., 2016, 147, 55–66 CrossRef CAS.
- Guidelines for Ecological Risk Assessment.
- The Risk Assessment Information System, https://rais.ornl.gov/index.html, accessed 2 July 2024.
- T. Tunsaringkarn, W. Siriwong, A. Rungsiyothin and S. Nopparatbundit, Occupational exposure of gasoline station workers to BTEX compounds in Bangkok, Thailand, Int. J. Occup. Environ. Med., 2012, 3, 117–125 CAS.
- H. A. Arregocés, R. Rojano and G. Restrepo, Health risk assessment for particulate matter: application of AirQ+ model in the northern Caribbean region of Colombia, Air Qual., Atmos. Health, 2023, 16, 897–912 CrossRef PubMed.
- D. S. Bisht, U. C. Dumka, D. G. Kaskaoutis, A. S. Pipal, A. K. Srivastava, V. K. Soni, S. D. Attri, M. Sateesh and S. Tiwari, Carbonaceous aerosols and pollutants over Delhi urban environment: Temporal evolution, source apportionment and radiative forcing, Sci. Total Environ., 2015, 521–522, 431–445 CrossRef CAS PubMed.
- M. Pandey, A. K. Pandey, A. Mishra and B. D. Tripathi, Speciation of carcinogenic and non-carcinogenic metals in respirable suspended particulate matter (PM10) in Varanasi, India, Urban Clim., 2017, 19, 141–154 CrossRef.
- A. A. Khan, K. Garsa, P. Jindal, P. C. S. Devara, S. Tiwari and P. B. Sharma, Demographic Evaluation and Parametric Assessment of Air Pollutants over Delhi NCR, Atmosphere, 2023, 14, 1390 CrossRef CAS.
- H. Kamani, M. Baniasadi, H. Abdipour, L. Mohammadi, S. Rayegannakhost, H. Moein and A. Azari, Health risk assessment of BTEX compounds (benzene, toluene, ethylbenzene and xylene) in different indoor air using Monte Carlo simulation in Zahedan city, Iran, Heliyon, 2023, 9, e20294 CrossRef CAS PubMed.
- G. Demirel, Ö. Özden, T. Döğeroğlu and E. O. Gaga, Personal exposure of primary school children to BTEX, NO2 and ozone in Eskişehir, Turkey: Relationship with indoor/outdoor concentrations and risk assessment, Sci. Total Environ., 2014, 473–474, 537–548 CrossRef CAS PubMed.
- F. Yousefian, M. S. Hassanvand, R. N. Nodehi, H. Amini, N. Rastkari, M. Aghaei, M. Yunesian and K. Yaghmaeian, The concentration of BTEX compounds and health risk assessment in municipal solid waste facilities and urban areas, Environ. Res., 2020, 191, 110068 CrossRef CAS PubMed.
-
Annual Report on Registration of Births and Deaths in Delhi, Directorate of Economics and Statistics & Office of Chief Registrar (Births and Deaths), Govt. of NCT of Delhi, 2022, https://des.delhi.gov.in/sites/default/files/inline-files/annual_report_2022.pdf Search PubMed.
- P. K. Hopke, S. S. Hashemi Nazari, M. Hadei, M. Yarahmadi, M. Kermani, E. Yarahmadi and A. Shahsavani, Spatial and Temporal Trends of Short-Term Health Impacts of PM2.5 in Iranian Cities; a Modelling Approach (2013-2016), Aerosol Air Qual. Res., 2018, 18, 497–504 CrossRef CAS.
- D. J. Naghan, A. Neisi, G. Goudarzi, M. Dastoorpoor, A. Fadaei and K. A. Angali, Estimation of the effects PM2.5, NO2, O3 pollutants on the health of Shahrekord residents based on AirQ+ software during (2012–2018), Toxicol Rep, 2022, 9, 842–847 CrossRef CAS PubMed.
- S. Faridi, M. Shamsipour, M. Krzyzanowski, N. Künzli, H. Amini, F. Azimi, M. Malkawi, F. Momeniha, A. Gholampour, M. S. Hassanvand and K. Naddafi, Long-term trends and health impact of PM2.5 and O3 in Tehran, Iran, 2006–2015, Environ. Int., 2018, 114, 37–49 CrossRef CAS PubMed.
|
This journal is © The Royal Society of Chemistry 2024 |