Polybrominated diphenyl ethers in the grey-headed gull (Larus cirrocephalus) and African sacred ibis (Threskiornis aethiopicus)
Received
4th May 2023
, Accepted 19th August 2023
First published on 4th October 2023
Abstract
PBDEs have been studied in different types of birds from varying ecosystems, however, few of the studies have focused particularly on scavenger birds. It is important to study these birds since many scavenging birds forage on sites close to human population. The present study investigated PBDE concentrations in the grey-headed gull (Larus cirrocephalus) and African sacred ibis (Threskiornis aethiopicus) scavenging on a landfill site in Pretoria, South Africa. The detection frequencies of PBDEs in soil, leachate and feather samples ranged from nd to 100%, with the higher PBDE congeners generally displaying higher detection frequencies. Soil samples indicated 100% detection frequency for all the congeners, while the detection frequencies of PBDEs in leachate samples ranged from “not detected” for BDE-209 to 100% for BDE-28 and -183. In the feathers of grey-headed gulls and sacred ibises, seven of the eight PBDEs investigated were detected at high frequencies, 87.5–100% and 28–100% respectively. The mean PBDE concentrations detected in soil samples ranged from 1.23 ng g−1 for BDE-183 to 5.12 ng g−1 for BDE-209. The 45–150 μm particle size fraction yielded higher concentrations of PBDEs than the coarser 250–500 μm fraction. The concentrations of PBDEs in the leachate samples ranged from 0.49 g L−1 for BDE-153 to 1.68 g L−1 for BDE-100. The dominant congeners were BDE-47, -100 and -183, while the least dominant congener was BDE-153. The grey-headed gull had ∑7BDE 47, 99, 100, 153, 154, 183 and 209 ranging in total from 44.40 to 143.45 ng g−1 (median = 95.10 ng g−1). The ∑7PBDEs for the African sacred ibis ranged from 70.31 to 608.41 ng g−1 (median = 179.08 ng g−1). Spearman's correlation test revealed no significant relationship between beak length and pollutant burden in L. cirrocephalus feathers, suggesting that pollutant accumulation is not a function of age for these birds. Pearson correlation analysis was carried out for ∑7 PBDEs versus leachate (r = 0.65), soil (r = 0.52) and feather (r = 0.77) with a statistical significance of p < 0.05. The observed positive correlation may suggest a possible influence of leachate and soil on PBDE concentrations in the feathers. These findings indicated high PBDE concentrations in comparison with similar studies carried out in other parts of the world. This suggested that the avian species investigated in this study may be impacted as a result of PBDE contamination, which may also have similar negative effects on other wildlife and human populations that are exposed to the Ondestepoort landfill.
Environmental significance
Of all the different types of flame retardants available, brominated flame retardants, particularly polybrominated diphenyl ethers (PBDEs), were preferred because of their low costs and high efficiency as fire retardants. Penta-BDE was mostly used in polyurethane foam while octa-BDE and deca-BDE were used in the casings of electrical and electronic equipment and high impact polystyrene and in polypropylene fabric respectively. Until recently, PBDEs were identified to be toxic, bioaccumulative and persistent, and consequently, they were listed as persistent organic pollutants (POPs) under the Stockholm Convention. The continued usage of the various formulations is now either heavily regulated or has been completely stopped. Additionally, PBDEs are also lipophilic and, therefore, capable of accumulating in the fatty tissues of living systems where they can disrupt the endocrine system. Due to their bioaccumulation ability, they are also capable of biomagnifications within both aquatic and terrestrial food chains. There are no known studies with a specific focus on determining PBDE concentrations in living scavenger birds, considering the fact that birds are recognized as suitable biological markers for environmental contamination. A study of selected bird species that commonly make use of municipal refuge disposal sites would provide valuable insights concerning PBDE contamination in biota in such environments. The prevalence of PBDEs in the feathers of two avian species that frequent a domestic landfill site in Pretoria, South Africa was investigated. In the grey-headed gull and African sacred ibis bird species studied, seven of the eight PBDE congeners were detected very frequently. These findings indicated high PBDE concentrations in comparison with similar studies carried out in other parts of the world, strongly suggesting that the avian species investigated may be impacting affected birds, and implies similar negative effects on other wildlife and human populations that are exposed to the Ondestepoort landfill.
|
1. Introduction
Of all the different types of flame retardants available, brominated flame retardants were particularly desirable because of their low costs and high efficiency until recently when their toxic, bioaccumulative and persistent characteristics were identified. Previously, they were extensively used in the manufacturing industry since the 1960s. There are four main classes of brominated flame retardants, namely tetrabromobisphenol A, polybrominated biphenyls, polybrominated diphenyl ethers (PBDEs) and hexabromocyclododecane. PBDEs were the most widely used commercially and were sold in three main types of commercially available mixtures: penta-BDE, octa-BDE and deca-BDE.1 Penta-BDE was mostly used in polyurethane foam while octa-BDE and deca-BDE were used in the casings of electrical and electronic equipment and high impact polystyrene and in polypropylene fabric respectively.1
Despite their effectiveness as flame retardants, PBDEs were listed as persistent organic pollutants under the Stockholm Convention, and the continued use of the various formulations is either heavily regulated or has been completely stopped. For example, manufacturers in the US discontinued the production of octa- and penta-PBDE in 2004.2 PBDEs are additive flame retardants, which implies that they can easily volatilize or leach from their parent products to the surrounding environment. This ‘leaching’ can start as early as the day of manufacture, and it generally increases with time as the parent product ages and degrades. Additionally, PBDEs can enter the environment through emissions from the manufacturing process. PBDEs are also very stable in the environment, i.e., they do not break down easily and, therefore, tend to be quite persistent, hence their classification as persistent organic pollutants.3 They are also lipophilic and, therefore, capable of accumulating in the fatty tissues of living systems.4 PBDEs have been identified in different types of animals, both terrestrial and aquatic.5 They distribute easily within animals, as evidenced by their presence in different types of tissues such as muscle and liver, as well as in urine and faeces. In humans, PBDEs have been found in blood, hair, nails, milk, liver and adipose tissues.6 Due to their bioaccumulation ability, they are also capable of biomagnification in the food chain.7
Several studies have demonstrated biological activity of certain PBDEs highlighting the fact that these compounds are capable of adversely affecting the biological systems.8–10 Specific health risks associated with these PBDEs are hormonal imbalances, developmental neurotoxicity and cancer. Considering the potential risks associated with this group of compounds, the need for regular monitoring is quite evident.
Avian wildlife has frequently been used as an indicator of chemical pollution exposure and toxicity for assessing the health of aquatic ecosystems. Specifically, monitoring programs using bird tissue, blood and egg samples have supplied valuable data on spatial and temporal trends of organohalogen contaminants in areas of environmental concern. The presence of PBDEs in bird eggs was first reported, following the successful use of birds to monitor the levels of metallic pollutants in the air.11 Since then, studies on birds have been expanded to determine the effects of different types of feathers, gender, offspring and even regional and spatial trends.12 For pollution studies, higher trophic predatory birds have been recommended as assessment targets because of their ability to integrate pollutants over an extensive area.13 Certain gull (Laridiae) species such as the Great Lakes herring gull (Larus argentatus) have been identified as key sentinels of freshwater basin contamination in densely populated and industrialized regions.14,15 In Norway, the glaucous gull (Larus hyperboreus) served as a biomonitor species in the Arctic marine environment.16–18
To date, two known studies on PBDEs have been carried out on South African wildlife. The first was reported in the eggs of various bird species sampled from different parts of South Africa, with the highest levels found in a sacred ibis egg.19 A subsequent study found low levels of PBDEs in tiger fish from Lake Pongolapoort.20 These studies show that PBDEs are present in South African wildlife and diet. However, much work remains to establish the true extent of their spread, particularly in relation to birds that scavenge on landfill sites close to human population. Whereas PBDEs have been studied in different types of birds from varying ecosystems, few of these studies have focused particularly on scavenger birds. Scavenger birds are birds which are able to live off materials that are not traditional bird food. Most birds feed on particular things and, as such, birds can be classified according to what they eat, such as different plant parts (e.g. berries, flowers), amphibians, crustaceans, meat, fish, and others.21 To a large extent, the availability of such foods also determines the habitat of such birds. Scavengers are unique in the sense that they are not closed in their food selection, and are, therefore, able to accommodate a wider range of foods. In the case of landfill scavenger birds, they have perfected the art of finding enough food to survive from polluted sites littered with domestic waste.
In this study, two species of birds that frequent the Ondestepoort landfill in Pretoria, South Africa, were investigated for accumulation of PBDEs. The grey-headed gull, also known as the grey-hooded gull, is a small gull that breeds patchily in South America and sub-Saharan Africa, including Madagascar. It is one of the major types of gulls in South Africa, and it can be found in and ‘around’ freshwater, inland lakes throughout the country.22 Grey-headed gulls are opportunistic feeders, mainly eating aquatic invertebrates like insects and mollusks, and small vertebrates like fish and frogs.23 They also feed on the eggs of herons and cormorants, and are known to inhabit refuse dumps and feed on waste material such as offal.23 The African sacred ibis (Threskiornis aethiopicus) is a larger bird that is native to sub-Saharan Africa, excluding Madagascar and south eastern Iraq.24 It is distinguished by its white body and black head, neck, legs and feet. The African sacred ibis inhabits a wide range of habitats, including freshwater wetlands, salt pans, dams, mangroves, rivers in open forested areas, and cultivated fields. It is an opportunistic feeder, mainly carnivorous. It eats insects, worms, bird and reptile eggs, crustaceans, frogs, lizards, small mammals and carrion. Certain populations also forage around human environments like refuse dumps, abattoirs and farmyards, with some becoming reliant on these areas as a food source. African sacred ibises can live for up to 20 years, and would be ideal for long term studies on BFR pollutants.25
These bird species are carnivorous birds native to sub-Saharan Africa, and are quite prevalent around South Africa. They also belong to stable populations; therefore, their use in monitoring studies such as this one should not pose a threat to their respective populations. Information obtained on the presence and levels of these pollutants in the selected birds can give an indication of environmental pollution levels as it relates to other wildlife and even human beings in the region.
While several studies have investigated the presence of PBDEs in various environmental matrices such as soil and river water,26,27 fewer studies have investigated PBDE concentrations in living things – particularly on the African continent. Also, there are no known studies with a specific focus on determining PBDE concentrations in live scavenger birds, and birds are recognized as suitable biological markers for environmental contamination.28 A study of selected bird species that commonly make use of municipal refuge disposal sites would provide valuable insights concerning PBDE contamination in biota in such environments. The present study, therefore, aimed at verifying whether PBDEs are present within avian species that frequent the Ondestepoort landfill in Pretoria, South Africa; the select species were the grey-headed gull (Larus cirrocephalus) and African sacred ibis (Threskiornis aethiopicus).
2. Materials and methods
Pure standards (1.2 mL of 50 mg L−1) of each certified standard solution of nine PBDE congeners (BDE-28, 47, 99, 100, 118, 153, 154, 183, and 209) were purchased from Wellington Laboratories (Guelph, Ontario, Canada). Silica gel (100–200 mesh), sodium sulphate (purity 99.9%), glass wool and HPLC grade solvents: acetone, hexane, dichloromethane, toluene, methanol, nonane and isooctane (Sigma Aldrich, Germany) were purchased from Industrial Analytical Pty. Ltd., South Africa.
2.1 Study area
Gauteng province is one of the northern/highveld provinces in South Africa, housing two of the country's largest cities, Pretoria and Johannesburg which are highly industrialized and densely populated. Like most urbanized centres worldwide, it has good infrastructure and basic amenities, including functional municipal refuse landfills strategically positioned within the province. Ondestepoort is one of such landfills, situated to the north of the nation's administrative capital, Pretoria. This landfill site receives domestic and industrial waste material from the residential and industrial/business locations surrounding the site. It is also a goldmine for recyclers, who spend their days collecting reusable material such as plastics, metals, wood, tiles, tyres and paper for re-sale to interested individuals and organizations. Previous studies show that the Ondestepoort landfill does contain significant levels of PBDEs, as well as other organohalogen and metallic pollutants.29 In addition to the recyclers who work on the site, a number of bird species also frequent the site, scavenging among the waste for edible matter, and drinking from on-site leachate ponds and monitoring water bodies. Fig. 1 shows a map of the study area.
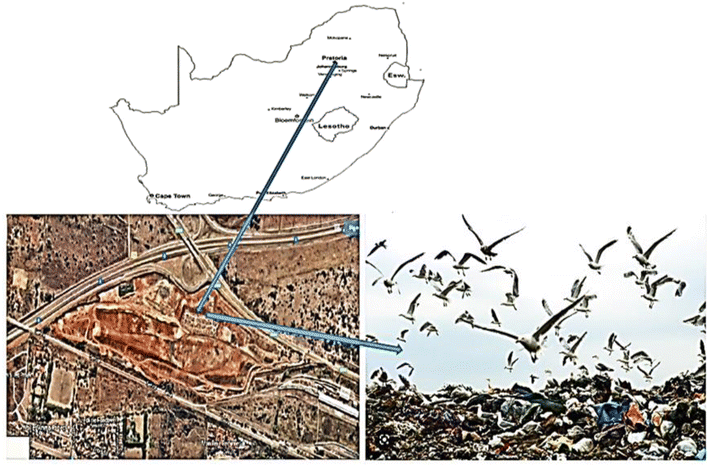 |
| Fig. 1 Map of South Africa (top) showing Pretoria and the Ondestepoort landfill (centre) site in Pretoria. | |
2.2 Sample collection
A total of 30 feather samples were obtained from live birds successfully trapped using a baited noose carpet hidden beneath the sand (Fig. 2). The feathers were collected between August and November 2016, from live birds in their natural habitat. Trapped birds were retrieved as soon as they were ensnared, and sampling was carried out immediately by snipping at the base of the feather using a sharp pair of scissors, as close to the umbilicus without harming the birds. In order to ensure that the same bird was not sampled more than once, all sampled birds were visibly marked by colouring the underside of one wing feather using a permanent marker. Also, before any newly trapped birds were sampled, the feathers were inspected to determine whether the bird had been sampled before. Four primary flight feathers – two from each wing – were collected from each bird, pooled and stored in aluminium foil and transported to the laboratory. The feather samples were stored in a cool, dry cupboard until treatment and analysis. The mass and beak length of each sampled bird was also recorded for age and gender estimation. The average beak lengths were 3.82 ± 0.3 cm, and 13.7 ± 3.30 cm for L. cirrocephalus and T. aethiopicus respectively, while the average masses were 0.41 ± 0.15 kg, and 1.31 ± 0.22 kg respectively.
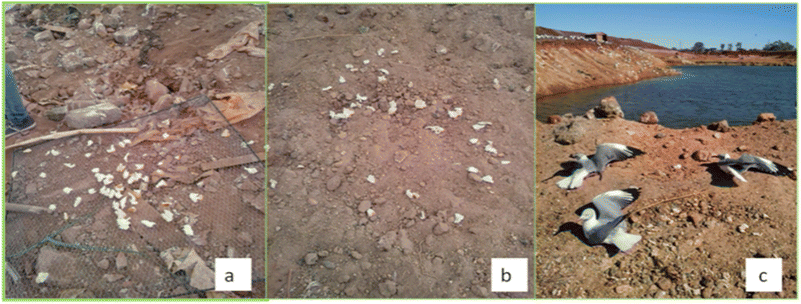 |
| Fig. 2 (a) Noose carpet with bait; (b) hidden noose carpet and (c) trapped grey-headed gull (Larus cirrocephalus). | |
Although the primary aim of the study was to determine the presence and concentrations of PBDEs in two species of birds, it was deemed necessary to collect soil and leachate samples from the landfill site in order to obtain background levels of PBDEs. Consequently, soil and leachate samples were collected. Soil samples (n = 9) were collected randomly from various locations around the landfill site. A stainless steel ladle was used to first clear the surface, and then scoop out ∼2 kg of the underlying soil at a depth of ≥3 cm into a labelled aluminium foil wrap. Each sample was carefully wrapped and placed in a sterile cooler box for transportation to the laboratory where they were stored at 4 °C for further processing. The samples were sieved into fractions of 45–150 μm and 250–500 μm for processing, in order to investigate the effects, if any, of particle sizes on pollutant burdens.
Leachate samples were also collected from one edge of the run-off pond, where the water was safely accessible within the landfill site. Leachate samples were collected into thoroughly washed and acetone rinsed and finally oven dried 2.5 L brown glass bottles. After clearing the surface of visible floating debris, each bottle was rinsed with some of the leachate and then filled by dipping the glass bottle at an angle into the water body until it was filled to the brim. Each bottle was then capped tightly and transported to the laboratory, where the pH was measured and adjusted to ∼3 by dropwise addition of concentrated hydrochloric acid, with subsequent shaking. The samples were then refrigerated at 4 °C until extraction and analysis.
2.3 Chemicals, reagents and their purification
Materials used for the determination of some selected polybrominated diphenyl ethers frequently detected in most matrices included the following: Strata florisil 500 mg mL−l, ultra-pure water was dispensed from Labostar ultrapure water equipment, copper powder (purity 99.98%) from Saarchem (Pty) Ltd., Muldersdrift, South Africa), silica gel (100–200 mesh), sodium sulphate (purity 99.9%), glass wool and HPLC grade solvents: acetone, hexane, dichloromethane, toluene, isooctane and methanol (Sigma Aldrich (Chemie GmbH, Steinheim, Germany), and 50 mL of nonane (purity 99.8%, Sigma Aldrich, product of Switzerland) were purchased from Industrial Analytical Pty. Materials such as silica gel, sodium sulphate and copper powder were activated before use by heating in a muffle furnace at 450 °C for 16 h. Acidic silica was prepared by adding 44 g of silica and concentrated H2SO4 and stirred until fine powder was achieved; meanwhile, basic silica was prepared by mixing 30 g of 1 N NaOH and stirred into fine powder. Copper powder was activated by soaking in 6 N HCl for 3 min and then rinsed thoroughly with ultrapure water followed by methanol and kept under toluene until use. Preparation of standards and serial dilution of working standards were done in a fume hood. 1.2 mL of 50 mg L−1 of each certified standard solution of 10 PBDE (28, 47, 100, 99, 153, 154, 183 and 209) congeners were purchased from Wellington Laboratories (Guelph, Ontario, Canada).
2.4 Recovery test and sample preparation
One hundred micro litres of 3.3 ng μL−1 PBDE surrogate standards were dissolved in 5 mL acetone and then spiked into triplicate 200 mL leachate samples and left to stand in a 45 °C water bath for 24 h for equilibration. The mixture was, thereafter, extracted using a liquid–liquid extraction method with 3 × 20 mL dichloromethane as the extraction solvent for 10 min. The extract was collected and concentrated to 1 mL using a rotary evaporator (Buchi Rotavapor, R-210, supplied by Labotec, South Africa), which was cleaned before GCMS analysis. The observed recovery ranged from 80 to 110%. Similarly for solids, triplicate 5 g sodium sulphate fortified with 100 μL PBDE surrogate dissolved in acetone was spiked into sodium sulphate. The impregnated salt was transferred into a glass conical flask, 2 g activated copper was added into the mixture and subjected to ultrasonication extraction using hexane/DCM (4
:
1, v/v) in a water bath of 45 °C for 24 h equilibration, with each extraction running for 15 min. Recoveries observed ranged from 85 to 101%.
The feathers were washed three times with distilled water, dried overnight at room temperature, and cut into pieces of ∼1 mm using stainless steel scissors. Accurately weighed feathers (500 ± 1 mg) were incubated at 45 °C in 4 M HCl (10 mL) for 24 h, after which a solvent mixture of 4
:
1 n-hexane/dichloromethane (10 mL) was added. The mixture was agitated using ultrasonication (Elmasonic S40H, 60 s) and allowed to stand in a 45 °C water bath for a further 24 h. Ultrasonication-assisted extraction was done using 3 × 5 mL of 4
:
1 hexane/DCM, with each extraction running for 15 min in the ultrasonicator. The combined organic fraction was concentrated using rotary evaporation to approximately 1 mL followed by clean up.
Bulk soil was air dried for 24 h at ambient temperature and sieved using mesh sieves into the following particle size categories: 45–150 μm and 250–500 μm. Sieved soil samples were prepared as described by Sánchez-Brunete et al.30 and K. K. Kefeni and J. O. Okonkwo31 with slight modification. Briefly, 5 g of soil and 1.5 g anhydrous sodium sulphate were weighed into brown glass vials, covered and extracted using the ultrasonication technique with hexane/DCM (4
:
1) (3 × 5 mL) as the extraction solvent, for 15 min per run. The combined extracts were filtered using Whatman filter paper into round-bottomed flasks and concentrated by rotary evaporation to ∼1 mL followed by clean up.
Leachate samples taken from the refrigerator were allowed to stand at room temperature until they had attained ambient temperature. After thoroughly shaking the bottle to homogenize the contents, triplicate sub-samples of 200 mL leachate samples were left to stand in a 45 °C water bath for 24 h for equilibration, and, thereafter, subjected to liquid–liquid extraction using 3 × 20 mL of dichloromethane (DCM) for 10 min. After extraction, the organic layers were combined and concentrated to ∼1 mL using a rotary evaporator. Extracts were cleaned up and prepared for instrumental analysis in the same manner as the soil samples.
All clean-up processes were performed using a 230 mm Pasteur pipette column filled with acidified silica (40% H2SO4, 3 cm) and anhydrous sodium sulphate (3 cm) separated by glass wool. The packed column was preconditioned with acetone (∼5 mL) and rinsed with 3
:
1 hexane/DCM (∼5 mL) and the extracts eluted using ∼10 mL of 3
:
1 hexane/DCM. The eluents were concentrated to near dryness under N2 to 250 μL. Before injection, BDE-118 (10 μL of 2.5 ng μL−1) was added as a quantitation internal standard and 1 μL was injected into the GC-MS. The method was validated with the recovery of surrogate standards 13C12 BDE-139 (90%) and BDE-209 (81%). Isooctane was fortified with a BDE-118 internal standard at 167 μg mL−1 for instrumental analysis.
2.5 Instrumental analysis
Prepared samples were analyzed using an Ultra-trace 2010 Shimadzu GC equipped with a QP2010 Ultra mass spectrometer operated in EI mode for PBDEs (28, 47, 100, 99, 153, 154, 183 and 209). Analysis was done in selective ion monitoring (SIM) mode, using a 15 m DB-5 (USA) column. The column diameter and packing thickness were 0.25 mm and 0.10 μm, respectively. The oven temperature programme was started at 90 °C (1 min), ramped up to 160 °C at 15 °C min−1, ramped again to 300 °C at 20 °C min−1, and finally to 310 °C at 10 °C min−1; it remained at 310 °C for 8 min. Helium (99.999% purity) was used as the carrier gas, and the flow rate was kept constant at 1.5 mL min−1. The ion source, injector and transfer line temperatures were set at 250, 270 and 315 °C, respectively.
2.5 Quality control
All sample preparation processes were carried out under conditions of reduced lighting to minimize the chances of degradation, particularly for the more highly brominated congeners. Storage was done in brown glass containers or aluminium foil wrap. Glass wool, silica gel and sodium sulphate used for sample clean-up were baked at 450 °C for 16 h to remove impurities, and then allowed to cool in desiccators. The silica gel and sodium sulphate were stored in pre-cleaned glass containers which were sealed to minimise hydration and contamination. Glass wool was wrapped in aluminium foil and stored in a desiccator for the same reasons. Internal standard calibration of the GC-MS was done using BDE-118 and a nine-level calibration curve. Retention times of unknown analytes were matched with those of the standards, and quantification was carried out by manually integrating the peaks from the molecular and reference ions generated.
Prior to sample analysis and after, an initial solvent blank, a laboratory performance standard check (linearity of the calibration curve) was performed using individual and mixtures of the most common brominated flame retardants. This was to ensure proper performance of the GC-MS. Check standard was run after every five samples to monitor retention time deviation. The use of surrogate standards during extraction and after clean-up was done to ensure accuracy. Retention times matched those of the standards and quantification was done by monitoring the molecular and reference ions using both internal and external methods. The limit of detection was taken as greater than 3 times the signal-to-noise ratio and the limit of quantification as 10 times the signal to noise ratio. For all the congeners, good linear regression of 0.999 was obtained. LOD values ranged from 0.02 to 0.0.05 ng μL−1 for liquid samples and 0.05 to 0.1 ng g−1 for solid samples; meanwhile, the LOQ ranged from 0.1 to 0.2 ng μL−1 for liquid samples and 0.5 to 0.9 ng g−1 for solid samples. Each sample was analyzed three times to facilitate proper statistical examination. Microsoft Office Excel 2007 was used for statistical analysis, except for correlation tests, which were done using SPSS.
3. Results and discussion
3.1 Detection frequencies of PBDEs in soil, leachate and feather samples
The detection frequencies of PBDEs in soil, leachate and feathers are shown in Table 1. As can be seen in Table 1, the detection frequencies ranged from nd to 100%, with the higher PBDE congeners generally displaying higher detection frequencies. Soil samples indicated 100% detection frequency for all the congeners, while the detection frequencies of PBDEs in leachate samples ranged from “not detected” for BDE-209 to 100% for BDE-28 and -183. In the feathers of grey-headed gulls and sacred ibises, seven of the eight PBDEs investigated were detected at high frequencies, 87.5–100% and 28–100% respectively. It is worth noting that BDE-28 was not detected in the feather of any of the birds.
Table 1 Detection frequencies (%) of PBDE congeners in soil, leachate and feather samples from the Ondestepoort landfill
PBDE congener |
Soil |
Leachate |
Feathers |
GHGa |
ASIa |
GHG = grey-headed gull; ASI = African sacred ibis; nd = not detected.
|
BDE-28 |
100 |
100 |
nd |
nd |
BDE-47 |
100 |
67 |
87.5 |
28.6 |
BDE-99 |
100 |
89 |
87.5 |
100 |
BDE-100 |
100 |
78 |
100 |
100 |
BDE-153 |
100 |
89 |
90.6 |
100 |
BDE-154 |
100 |
89 |
84.4 |
100 |
BDE-183 |
100 |
100 |
100 |
100 |
BDE-209 |
100 |
nd |
100 |
100 |
3.2 Soil samples
Soil samples were analyzed for PBDEs in two particle size fractions, 45–150 μm and 250–500 μm. As shown in Fig. 3, all the PBDEs investigated were detected in 100% of the soil samples analyzed. The mean PBDE concentrations detected ranged from 1.23 ng g−1 for BDE-183 to 5.12 ng g−1 for BDE-209. Apart from BDE-183 and -209, the finer 45–150 μm particle size fraction yielded higher concentrations of PBDEs than the coarser 250–500 μm fraction.
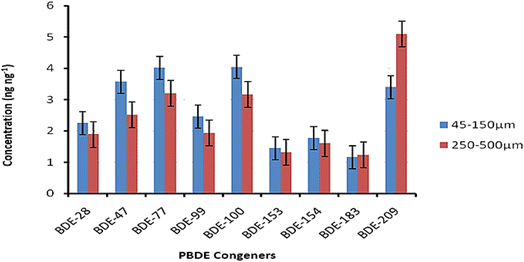 |
| Fig. 3 PBDE concentrations in soil samples from the Ondestepoort landfill. | |
3.3 Leachate samples
The concentrations of PBDEs in the leachate samples ranged from 0.49 g L−1 for BDE-153 to 1.68 g L−1 for BDE-100 (Fig. 4). The dominant congeners were BDE-47, -100 and -183, while the least dominant congener was BDE-153. BDE-209 was not detected in the leachate samples. The leachate results from the present study were compared with previous work in landfill sites in the city of Tshwane.32 PBDE concentrations of 9.793 ng L−1 for Temba, and 7.230 ng L−1 and 4.009 ng L−1 for Hatherly and Soshanguve landfill sites, respectively, were reported. In another study, a concentration of 2240 ng L−1 of PBDEs was reported in leachates from a landfill in the city of Cape Town, South Africa.33 The aforementioned concentrations are far higher than the concentrations observed in the present study. The observed lower concentrations observed in the present study compared to previous works32,33 may indicate less use and dumping of PBDE-containing products as well as ongoing intensive recycling of wastes in South Africa. Further comparison to findings from other regions was also conducted. In a study, a concentration range of 4–352 ng L−1 was reported from selected MSWLs in Northern China.34 A value of 4 ng L−1 was found in some seven MSWLs in Japan.35 Furthermore, a PBDE concentration range of 4–12 ng L−1 was reported in treated leachate effluent and a maximum of 234 ng g−1 for ∑8 PBDEs in top soil of one MSWL in Shanghai, China.36 That BDE-209 was predominant in leachates and soil samples from China was not surprising because China has been identified as one of the highest producers of deca-BDE with high consumption.37 High PBDE concentrations were reported in leachate samples from 28 landfills across Canada with a range of 1020–21
300 ng L−1.38 The highest concentration of 3.7–133
000 ng L−1 was reported in MSWL leachates from major cities in selected Asian countries.39
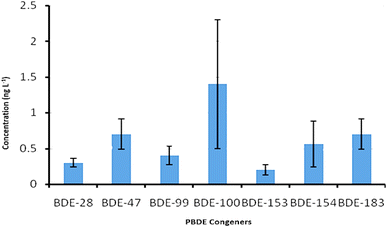 |
| Fig. 4 PBDE concentrations in leachate samples from the Ondestepoort landfill. | |
3.4 Feather samples
Feather samples from trapped birds were analysed to determine the presence and extent of PBDE contamination in the selected species. The samples analyzed contained significant amounts of PBDEs. Cumulative PBDE concentrations in feathers from individual grey-headed gulls and sacred ibises are shown in Fig. 5 and 6 respectively. Of the eight PBDEs investigated, seven were detected in grey-headed gulls and sacred ibises. BDE-28 was not detected in either of the species. In almost all sample sets, PBDE-209 had the highest detection levels. The grey-headed gull had ∑7BDE 47, 99, 100, 153, 154, 183 and 209 ranging in total from 44.40 to 143.45 ng g−1 (median = 95.10 ng g−1). Congener burdens followed the pattern BDE-209 > BDE-100 > BDE-47 > BDE-153. Fig. 5 shows the cumulative totals obtained for each grey-headed gull.
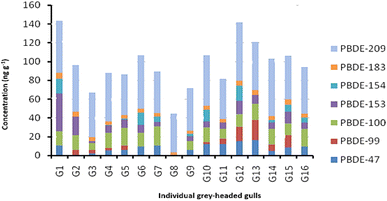 |
| Fig. 5 Cumulative PBDE concentrations in grey-headed gull feathers. | |
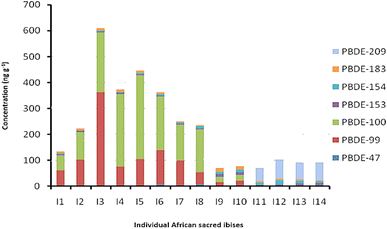 |
| Fig. 6 Cumulative PBDE concentrations in African sacred ibis feathers. | |
Fig. 6 shows the cumulative concentrations of PBDEs obtained for each sacred ibis. The ∑7PBDEs ranged from 70.31 to 608.41 ng g−1 (median = 179.08 ng g−1). There are two distinct congener distribution patterns, which are consistent with different sampling times, as the first samples were obtained in the spring of 2016, while the last four were obtained in the summer of 2016. The observed different PBDE loads can be explained as follows: the Africa sacred ibis is an opportunistic feeder, mainly carnivorous and it inhabits a wide range of habitats in search of food, including freshwater wetlands, salt pans, dams, mangroves, rivers in open forested areas, and cultivated fields. It eats insects, worms, birds and reptile eggs, crustaceans, frogs, lizards, small mammals and carrion. Certain populations also forage around human environments like refuse dumps, abattoirs and farmyards, with some becoming reliant on these areas as a food source. This feeding behaviour may have contributed to the observed different PBDE loads. Although not investigated in this study, heavy rainfall during summer which can deposit contaminants into different environmental compartments including habitats for the African ibis may have also contributed to the observed different PBDE loads.
That PBDEs were identified in the feathers and in environmental samples strongly suggests that the PBDE contamination observed in the birds can be attributed to their immediate environment. A number of studies have revealed correlations between environmental and biological pollutant burdens in several species of biota.12,40–43 Both the PBDE values obtained in the present study are generally higher than those reported in other studies on bird feathers. However, they were comparable to PBDE levels found in bird eggs of three insectivorous species from Belgium44 and eggs of various bird species obtained from different parts of South Africa.19 This is possibly because the birds in this study spend a lot of time in the highly polluted Ondestepoort landfill environment. The Ondestepoort landfill receives large amounts of building material waste, which probably includes PBDE-treated products. PBDE levels in feathers in this study were much higher than the levels reported in other studies (Table 2) conducted on feathers in both adult45 and nestling birds sampled in different parts of Europe.46 While studies of organohalogen contaminants in feathers are limited, several studies have been carried out on bird eggs.19,47,48
Table 2 Comparative analysis of median and ranges of PBDEs in feathers from various studies
Bird species |
PBDEs (ng g−1) |
28 |
47 |
99 |
100 |
153 |
154 |
183 |
Ref. |
T. aethiopicus, feathers |
2.48 |
0.40 |
19.10 |
10 |
9.94 |
9.94 |
13.23 |
This study |
2.42–2.54 |
nd–0.80 |
16.16–22.04 |
2.20–22.04 |
9.70–10.17 |
9.70–10.18 |
12.71–13.74 |
L. cirrocephalus, feathers |
13 |
9.6 |
54 |
18.6 |
7.6 |
6.8 |
4.1 |
This study |
12.5–14.5 |
6.4–16.4 |
nd–21.4 |
7.4–20 |
2.2–40.4 |
nd–16.4 |
3.2–6.2 |
White-tailed eagle body feathers |
|
5.6 |
0.75 |
|
|
|
|
Jaspers et al. 2011 |
|
0.39–22 |
0.09–5.4 |
|
|
|
|
Common magpie, urban |
|
0.3 |
0.17 |
|
|
|
|
Jaspers et al. 2009 |
|
0.12–0.86 |
0.09–1.07 |
|
|
|
|
Common buzzard, outer vane |
|
1.02 |
0.79 |
0.28 |
|
|
|
Jaspers et al. 2007 |
There is also growing data for organohalogen pollutants in human hair, particularly for individuals that are exposed either occupationally49,50 or by virtue of residential location.51 Hair and feathers are both keratinous tissues that are capable of trapping pollutants sourced internally (via the blood stream) and externally (by direct deposition from the environment). This property sets them apart from other types of tissues, which only get pollutants deposited via the blood stream, and may or may not accumulate them, depending on the physiology of the particular organ. That explains why differential accumulation levels have been observed in different tissues and organs from the same animal.1,52 With hair and feathers, pollutants deposited internally remain capable of reflecting pollution levels in the bird or person for as long as the hair strand or feather is attached, which can be months or even years. External pollutant deposits on human hair would usually get stripped with regular washing, but pollutants in bird feathers and wild animals' fur can accumulate better and remain for much longer, since they do not clean as frequently and/or thoroughly as human beings do.
In a study, the concentrations, total body burdens and compositional patterns of a large suite of persistent organohalogens were investigated in liver, blood and whole body homogenate samples of adult glaucous gulls from Svalbard, Norway.18 ∑PBDEs in liver samples (51.5 ± 15.9 ng g−1) were about 10 times less than the ∑PBDEs in blood samples (522 ± 154 ng g−1 ww). The whole body homogenates processed with feathers yielded – on average – lower PBDE levels than the homogenates processed without feathers, although they were in the same order of magnitude. This indicates a higher density of PBDE contaminants in the body tissue than in the feathers, which is not surprising, considering the fact that PBDEs would naturally circulate within the body before they can be transferred to be attached to tissues like feathers and claws. When conducting studies on pollutants in feathers, it is important to remember that pollutants sourced from the bloodstream may vary – both in magnitude and profile – from pollutants deposited externally. The total PBDE burdens may be even higher than measured due to the potential for additional congeners, not measured here but found in other bird studies, to be present.18
A nonparametric (Spearman's) correlation test was conducted and the results revealed no significant relationship between beak length and pollutant burden in L. cirrocephalus feathers, suggesting that pollutant accumulation is not a function of age for these birds. However, the same test revealed strong correlations at p < 0.01 between 47 and 154 (r = 0.856); 154 and 183 (r = 0.766); and 153 and 183 (r = 0.765). This could indicate a similar source, since BDE-153, -154 and -183 are all constituents of the octa-BDE technical mixture. Pearson correlation analysis between PBDE concentrations in leachate, soil and feather samples was performed. Correlation values for ∑7 PBDEs in leachate versus soil (r = 0.65), soil versus feather (r = 0.52) and leachate versus feather (r = 0.77) with a statistical significance (p < 0.05) were observed. The observed positive correlation may suggest a possible influence of leachate and soil on PBDE concentrations in the feathers.
Most previous studies on BFRs in feathers have focused on tail feathers, which are likely to contain elevated levels of pollutants due to their close proximity to the preen gland. In a study,53 PBDE levels in blood and feathers were in the same order of magnitude, and the levels found in preen oil were up to 12.5 times the concentrations found in the feathers. Grooming behaviour of birds (preening) is easily able to transfer polluted dust and preen oil to their beaks and nostrils, from where they can be inhaled or ingested into the system. Domestic cats have also exhibited high levels of PBDEs, and this has been attributed – to some extent – to their ‘preening’ tendencies, as well as their limited ability to metabolize PBDEs.54 It has been suggested54 that with the current and projected increase in African urbanisation and demand for consumer goods, there is substantial potential for concentrations of PBDEs and other POP chemicals to rise in the near future. There is, therefore, a need for long-term monitoring of concentrations of these chemicals in outdoor and indoor environments at a range of locations across the African continent. This should be designed to assess spatial trends and human exposure via inhalation and incidental dust ingestion, as well as facilitating elucidation of temporal trends of POPs in Africa and evaluation of the impacts of measures to reduce concentrations.
4. Conclusion
PBDEs were detected in soil, leachate and feather samples collected from the Ondestepoort landfill site in Pretoria, South Africa. 100% detection frequency was observed in soil samples for all the congeners, whereas the detection frequencies of PBDEs in leachate samples ranged from “not detected” for BDE-209 to 100% for BDE-28 and -183. In the grey-headed gulls and sacred ibises, seven of the eight PBDEs were detected at the following frequencies, 87.5–100% and 28–100% respectively. The grey-headed gull had ∑7BDE 47, 99, 100, 153, 154, 183 and 209 ranging in total from 44.40 to 143.45 ng g−1 (median = 95.10 ng g−1). The ∑7PBDEs for the African sacred ibis ranged from 70.31 to 608.41 ng g−1 (median = 179.08 ng g−1). These findings indicated high PBDE concentrations in comparison with similar studies carried out in other parts of the world, strongly suggesting that the PBDE levels observed in the avian species investigated in this study may be impacting affected birds, and implies similar negative effects on other wildlife and human populations that are exposed to the Ondestepoort landfill.
Author contributions
Conceptualization: Prof. O. J. Okonkwo. Data curation: Prof. O. J. Okonkwo, Prof. R. Jansen and Ms C. Emereole. Formal analysis: Ms C. Emereole. Funding acquisition: Prof. O. J. Okonkwo. Investigation: Prof. O. J. Okonkwo, Prof. R. Jansen and Ms C. Emereole. Methodology: Prof. O. J. Okonkwo, Prof. R. Jansen and Ms C. Emereole. Project administration: Prof. O. J. Okonkwo and Prof. R. Jansen. Resources: Prof. O. J. Okonkwo and Prof. R. Jansen. Software: Tshwane University of Technology. Supervision: Prof. O. J. Okonkwo and Prof. R. Jansen. Validation: Prof. O. J. Okonkwo, Prof. R. Jansen and Ms C. Emereole. Visualization: Prof. O. J. Okonkwo, Prof. R. Jansen and Ms C. Emereole. Writing – original draft: Ms C. Emereole and Prof. O. J. Okonkwo. Writing – review & editing: Ms C. Emereole, Prof. O. J. Okonkwo and Prof. R. Jansen.
Conflicts of interest
There is no conflict of interest.
Acknowledgements
The authors are indebted to Tshwane University of Technology, Pretoria, South Africa for financial support to Ms C. Emereole for her MSc programme in Environmental Management.
References
- S. Voorspoels, A. Covaci, P. Lepom, V. L. B. Jaspers and P. Schepens, Levels and distribution of polybrominateddiphenyl ethers in various tissues of birds of prey, Environ. Pollut., 2006, 144(1), 218–227 CrossRef CAS PubMed.
-
L. Stiffler. They are everywhere, they accumulate and they spread, The Seattle Post Intelligentser, March 28, 2007 Search PubMed.
- P. O. Darnerud, M. Aune, L. Larsson, S. Lignell, T. Mutshatshi, J. O. Okonkwo, B. Botha and N. Agyei, Levels of brominated flame retardants and other pesistent organic pollutants in breast milk samples from Limpopo province, South Africa, Sci. Total Environ., 2011, 409(19), 4048–4053 CrossRef CAS PubMed.
- A. P. Daso, J. O. Okonkwo, R. Jansen, P. B. Forbes, A. Kotzé and E. R. Rohwer, Polybrominated diphenyl ethers (PBDEs) in eggshells of the Southern Ground-hornbill (Bucorvus leadbeateri) and Wattled Crane (Bugeranus carunculatus) in South Africa, Chemosphere, 2015, 11, 284–292 CrossRef PubMed.
- I. Eulaers, V. L. B. Jaspers, R. Pinxten, A. Covaci and M. Eens, Legacy and current-use brominated flame retardants in the Barn Owl, Sci. Total Environ., 2014, 472, 454–462 CrossRef CAS PubMed.
- A. Covaci, S. Voorspoels, L. Roosens, W. Jacobs, R. Blust and H. Neels, Polybrominateddiphenyl ethers (PBDEs) and polychlorinated biphenyls (PCBs) in human liver and adipose tissue samples from Belgium, Chemosphere, 2008, 73(2), 170–175 CrossRef CAS PubMed.
- J. P. Wu, X. J. Luo, Y. Zhang, M. Yu, S. J. Chen, B. X. Mai and Z. Y. Yang, Biomagnification of polybrominateddiphenyl ethers (PBDEs) and polychlorinated biphenyls in a highly contaminated freshwater food web from South China, Environ. Pollut., 2009, 157(3), 904–909 CrossRef CAS PubMed.
- S. N. Kuriyama, C. E. Talsness, K. Grote and I. Chahoud, Developmental exposure to low-dose PBDE-99: effects on male fertility and neurobehaviour in rat offspring, Environ. Health Perspect., 2005, 56, 49–154 Search PubMed.
- T. A. McDonald, A perspective on the potential health risks of PBDEs, Chemosphere, 2002, 46(5), 745–755 CrossRef CAS.
-
J. B. Herbstman, A. Sjödin, M. Kurzon, S. A. Lederman, R. S. Jones, V. Rauh, L. L. Needham, D. Tang, M. Niedzwiecki, R. Y. Wang and F. Perera. Everyday Environ Toxins: Children’s Exposure Risks, 2015, vol. 12, pp. 223–238 Search PubMed.
- U. Sellström, A. Bignert, A. Kierkegaard, L. Häggberg, C. A. De Wit, M. Olsson and B. Jansson, Temporal trend studies on tetra-and pentabrominateddiphenyl ethers and hexabromocyclododecane in guillemot egg from the Baltic Sea, Environ. Sci. Technol., 2003, 37(24), 5496–5501 CrossRef PubMed.
- V. L. Jaspers, A. Covaci, P. Deleu and M. Eens, Concentrations in bird feathers reflect regional contamination with organic pollutants, Sci. Total Environ., 2009, 407(4), 1447–1451 CrossRef CAS PubMed.
- R. W. Furness and K. C. Camphuysen, Seabirds as monitors of the marine environment, ICES J. Mar. Sci., 1997, 54(4), 726–737 CrossRef.
- C. E. Hebert, R. J. Norstrom, J. Zhu and C. R. Macdonald, Historical changes in PCB patterns in Lake Ontario and Green Bay, Lake Michigan, 1971 to 1982, from herring gull egg monitoring data, J. Great Lakes Res., 1999, 25(1), 220–233 CrossRef CAS.
- R. J. Norstrom, M. Simon, J. Moisey, B. Wakeford and D. C. Weseloh, Geographical distribution (2000) and temporal trends (1981-2000) of brominated diphenyl ethers in Great Lakes herring gull eggs, Environ. Sci. Technol., 2002, 36(22), 4783–4789 CrossRef CAS PubMed.
- T. R. Barrett, S. JU and G. W. Gabrielsen, Recent changes in levels of persistent organochlorines and mercury in eggs of seabirds from the Barents Sea, Environ. Pollut., 1997, 92(1), 13–18 CrossRef PubMed.
- T. N. Savinova, A. Polder, G. W. Gabrielsen and J. U. Skaare, Chlorinated hydrocarbons in seabirds from the Barents Sea area, Sci. Total Environ., 1995, 160, 497–504 CrossRef.
- J. Verreault, W. A. Gebbink, L. T. Gauthier, G. W. Gabrielsen and R. J. Letcher, Brominated flame retardants in glaucous gulls from the Norwegian Arctic: more than just an issue of polybrominateddiphenyl ethers, Environ. Sci. Technol., 2007, 41(14), 4925–4931 CrossRef CAS PubMed.
- A. Polder, B. Venter, J. U. Skaare and H. Bouwman, Polybrominated diphenyl ethers and HBCD in bird eggs of South Africa, Chemosphere, 2008, 73(2), 148–154 CrossRef CAS PubMed.
- V. Wepene, N. Smit, A. Covaci, S. Dyke and L. Bervoets, Seasonal bioaccumulation of organohalogens in tigerfish, HydrocynusvittatusCastelnau, from Lake Pongolapoort, South Africa, Bull. Environ. Contam. Toxicol., 2012, 88(2), 277–282 CrossRef PubMed.
- P. O. Darnerud, Toxic effects of brominated flame retardants in man and in wildlife, Environ. Int., 2003, 29(6), 841–853 CrossRef CAS PubMed.
-
L. Oxley, Gulls in and around South Africa, http://www.adventures.co.za/gulls.htm, viewed, 6/11/2016 Search PubMed.
-
P. A. R. Hockey, W. R. J. Dean and P. J. Ryan, Roberts – Birds of Southern Africa, The Trustees of the John Voelcker Bird Book Fund, VIIth ed, 2005, Cape Town Search PubMed.
- E. Mariussen, E. Steinnes, K. Breivik, T. Nygård, M. Schlabach and J. A. Kålås, Elevated levels of polybrominated diphenyl ethers (PBDEs) in fish from Lake Mjøsa, Norway, Sci. Total Environ., 2008, 404(1), 162–170 CrossRef CAS PubMed.
-
WildscreenArkive 2016 African sacred ibis fact file, Downloaded from http://www.arkive.org on 07/11/2016 Search PubMed.
-
BirdLife International 2016 IUCN Red List for birds, Downloaded from http://www.birdlife.org on 07/11/2016 Search PubMed.
-
A. Elliot, E. F. J. Garcia, P. Boesman, In: J. Del Hoyo, A. EllioT, J. Sargatal, D. A. Christie and D. Juana, E. Juana, Handbook of the Birds of the World Alive [online], Barcelona, 2018, Lynx Edicions. Available from: https://www.hbw.com/node/52744Accessed 20–9 2018] Search PubMed.
-
M. S. De Villiers, Birds and Environmental Change: Building an Early Warning System in South Africa, Africa, South African National Biodiversity Institute, Pretoria, South Africa Search PubMed.
- O. I. Olukunle and O. J. Okonkwo, Concentration of novel brominated flame retardants and HBCD in leachates and sediments from selected municipal solid waste landfill sites in Gauteng Province, South Africa, Waste Manage., 2015, 43, 300–306 CrossRef CAS PubMed.
- C. Sánchez-Brunete, E. Miguel and J. L. Tadeo, Determination of organochlorine pesticides in sewage sludge by matrix solid-phase dispersion and gas chromatography-mass spectrometry, Talanta, 2006, 70(5), 1051–1056 CrossRef PubMed.
- K. K. Kefeni and J. O. Okonkwo, Distribution of polybrominated diphenyl ethers and dust particle size fractions adherent to skin in indoor dust, Pretoria, South Africa, Environ. Sci. Pollut. Res., 2014, 21(6), 4376–4386 CrossRef CAS PubMed.
- D. O. Odusanya, O. J. Okonkwo and B. Botha, Polybrominated diphenyl ethers (PBDEs) in leachates from selected landfill sites in South Africa, Waste Manage., 2008, 29(1), 96–102 CrossRef PubMed.
- A. Daso, O. Fatoki, J. Odendaal and O. Olujimi, Polybrominated diphenyl ethers (PBDEs) and 2,2′,4,4′,5,5′-hexabromobiphenyl (BB-153) in landfill leachate in Cape Town, South Africa, Environ. Monit. Assess., 2012, 185(1), 431–439 CrossRef PubMed.
- Y. Li, J. LI and C. Deng, Occurrence, characteristics and leakage of polybrominated diphenyl ethers in leachate from municipal solid waste landfills in China, Environ. Pollut., 2014, 184(0), 94–100 CrossRef CAS PubMed.
- M. Osako, Y.-J. Kim and S.-I. Sakai, Leaching of brominated flame retardants in leachate from landfills in Japan, Chemosphere, 2004, 57(10), 1571–1579 CrossRef CAS PubMed.
- K. Huang, J. Guo, K.-F. Lin, X.-Y. Zhou, J.-X. Wang and P. Zhou, Distribution and temporal
trend of polybrominated diphenyl ethers in one Shanghai municipal landfill, China, Environ. Sci. Pollut. Res., 2013, 20, 5299–5308 CrossRef CAS PubMed.
- Li J. Chen, L. Liu and N. Zhao, Polybrominated diphenyl ethers fate in China: A review with an emphasis on environmental contamination levels, human exposure and regulation, J. Environ. Manage., 2012, 113, 22–30 CrossRef PubMed.
- B. Li, M. N. Danon-Schaffer, L. Y. Li, M. G. Ikonomou and J. R. Grace, Occurrence of PFCs and PBDEs in landfill leachates from across Canada. Water, Water, Air, Soil Pollut., 2012, 223, 3365–3372 CrossRef CAS.
- C. S. Kwan, H. Takada, K. Mizukawa, M. Torii, T. Koike and R. Yamashita, PBDEs in leachates from municipal solid waste dumping sites in tropical Asian countries: phase distribution and debromination, Environ. Sci. Pollut. Res., 2013, 20, 4188–4204 CrossRef CAS PubMed.
- B. Jansson, R. Andersson, L. Asplund, K. Litzen, K. Nylund, U. Sellströom, U. B. Uvemo, C. Wahlberg, U. Wideqvist, T. Odsjö and M. Olsson, Chlorinated and brominated persistent organic compounds in biological samples from the environment, Environ. Toxicol. Chem., 1993, 12(7), 1163–1174 CrossRef CAS.
- R. A. Hites, Polybrominated diphenyl ethers in the environment and in people: a meta-analysis of concentrationsEnviron, Sci. Technol., 2004, 38(4), 945–956 CrossRef CAS PubMed.
- V. L. B. Jaspers, S. Voorspoels, A. Covaci, G. Lepoint and M. Eens, Evaluation of the usefulness of bird feathers as a non-destructive biomonitoring tool for organic pollutants: a comparative and meta-analytical approach, Environ. Int., 2007, 33(3), 328–337 CrossRef CAS PubMed.
- P. Ondarza, M. Gonzalez, G. Fillmann and K. Mglioranza, PBDEs, PCBs and organochlorine pesticides distribution in edible fish from Negro River basin, Argentinean Patagonia, Chemosphere, 2014, 94, 135–142 CrossRef CAS PubMed.
- T. Dauwe, E. Van den Steen, V. L. B. Jaspers, K. Maes, A. Covaci and M. Eens, Interspecific differences in concentrations and congener profiles of chlorinated and brominated organic pollutants in three insectivorous bird species, Environ. Int., 2009, 35(2), 369–375 CrossRef CAS.
- I. Eulaers, A. Covaci, D. Herzke, M. Eens, C. Sonne, T. Moum, L. Schnug, S. A. Hanssen, T. V. Johnsen, J. O. Bustnes and V. L. B. Jaspers, A first evaluation of the usefulness of feathers of nestling predatory birds for non-destructive biomonitoring of persistent organic pollutants, Environ. Int., 2011, 37(3), 622–630 CrossRef CAS PubMed.
- L. T. Gauthier, C. E. Hebert, D. C. Weseloh and R. J. Letcher, Dramatic changes in the temporal trends of polybrominateddiphenyl ethers (PBDEs) in herring gull eggs from the Laurentian Great Lakes: 1982–2006Environ, Sci. Technol., 2008, 42(5), 1524–1530 CrossRef CAS PubMed.
- D. Chen and R. C. Hale, A global review of polybrominateddiphenyl ether flame retardant contamination in birds, Environ. Int., 2010, 36(7), 800–811 CrossRef CAS PubMed.
- J. Zheng, K. H. Chen, X. J. Luo, X. Yan, C. T. He, W. J. Yu, G. C. Hu, X. W. Peng, M. Z. Ren, Z. Y. Yang and B. X. Mai, Polybrominateddiphenyl ethers (PBDEs) in paired human hair and serum from e-waste recycling workers: source apportionment of hair PBDEs and relationship between hair and serum, Environ. Sci. Technol., 2013, 48(1), 791–796 CrossRef PubMed.
- Z. Tang, Q. Huang, J. Cheng, Y. Yang, J. Yang, W. Guo, Z. Nie, N. Zeng and L. Jin, Polybrominateddiphenyl ethers in soils, sediments, and human hair in a plastic waste recycling area: a neglected heavily polluted area, Environ. Sci. Technol., 2014, 48(3), 1508–1516 CrossRef CAS PubMed.
- G. Zhao, Z. Wang, M. H. Dong, K. Rao, J. Luo, D. Wang, J. Zha, S. Huang and Y. Xu, PBBs, PBDEs, and PCBs levels in hair of residents around e-waste disassembly sites in Zhejiang Province, China, and their potential sources, Sci. Total Environ., 2008, 397(1), 46–57 CrossRef CAS PubMed.
- Q. Xian, K. Ramu, T. Isobe, A. Sudaryanto, X. Liu, Z. Gao, S. Takahashi, H. Yu and S. Tanabe, Levels and body distribution of polybrominateddiphenyl ethers (PBDEs) and hexabromocyclododecanes (HBCDs) in freshwater fishes from the Yangtze River, China, Chemosphere, 2008, 71(2), 268–276 CrossRef CAS PubMed.
- V. L. Jaspers, A. Covaci, P. Deleu, H. Neels and M. Eens, Preen oil as the main source of external contamination with organic pollutants onto feathers of the common magpie (Pica pica), Environ. Int., 2008, 34(6), 741–748 CrossRef CAS PubMed.
- W. Guo, J. S. Park, Y. Wang, S. Gardner, C. Baek, M. Petreas and K. Hooper, High polybrominateddiphenyl ether levels in California house cats: house dust a primary source?, Environ. Toxicol. Chem., 2012, 31(2), 301–306 CrossRef CAS PubMed.
- O. E. Akinrinade, W. Stubbings, M. Abdallah, O. Ayejuyo, R. Alani and S. Harrad, Status of brominated flame retardants, polychlorinated biphenyls, and polycyclic aromatic hydrocarbons in air and indoor dust in Africa, Emerging Contam., 2020, 6, 405–420 CrossRef.
|
This journal is © The Royal Society of Chemistry 2023 |
Click here to see how this site uses Cookies. View our privacy policy here.