Highly effective nanoparticle removal in plant-based water filters†
Received
13th February 2023
, Accepted 30th June 2023
First published on 10th July 2023
Abstract
There is emerging discussion over the advantages of nanotechnology and its prospective risks prompted by increasing reports of nanoparticle (NP) contamination of the environment and its potential impacts on human health. Water treatment facilities are a critical node for exposure to NP contamination but also offer an opportunity for their capture to minimize the exposure. Unfortunately, the typical drinking water treatment train is not suitable for the complete removal of NPs. In fact, the challenges facing the water treatment techniques with the removal of viruses (natural NPs) present a marked example of the energy-water nexus. Any upcoming regulations targeting the control of engineered and incidental NPs are bound to increase the burden on the available techniques. To address this emerging challenge, we established the feasibility of high-efficiency removal of man-made and natural NPs with a depth filter fabricated from plant-based materials. Using high-resolution analytical techniques that enable quick quantification of NP concentration, we showed that cotton fiber functionalized with cationic proteins from Moringa oleifera seeds achieve removals greater than 4
log10 (99.99%) for model nano-plastics and metal nanoparticles. Our results also show that the removals achieved are consistent under a range of pH values and salinities typical to drinking water treatment. The proposed filters in this study show promise as a low-cost and sustainable solution for the capture of NP contamination at loading rates typical to conventional water treatment.
Environmental significance
Harmful nanoparticles (NPs) are perpetually released into the atmosphere due to intentional and unintentional human activity. Conventional filtration and disinfection are not designed for the removal of inorganic nanoparticles whereas alternate membrane technologies face challenges in terms of initial and operational costs. There is a lack of accessible solutions based on natural materials to overcome this challenge which can have detrimental health effects. In this study, we show that a depth filter made of plant-based materials can achieve high removal of various nanoparticles. Due to the ease of fabrication and potential for gravity-driven operation, the proposed filters have the potential for application in low-resource settings.
|
Introduction
There is a growing concern about the presence of excess nanoparticles (NPs) in water systems and related health risks.1,2 This issue is driven by the high usage of engineered nanoparticles (ENPs), such as metal nanoparticles and carbon nanotubes, in everyday applications.3,4 Additionally, weathering of plastic and painted surfaces or combustion can cause the unintentional release of NPs, such as soot and nano-plastics termed incidental nanoparticles (INPs).5,6 Multiple studies indicate the potential toxicological effects NPs can have on human health.4,7 Thus, there is an emerging need for control of nano-contaminants such as nano-plastics and colloidal metals released into source waters perpetually by natural and human activity.
This interest in nano-contaminants may seem new, but NPs have been affecting human health from the earliest of times. For example, natural nanoparticles (NNPs), such as rotavirus and norovirus that cause widespread disease outbreaks are considered high-risk water contaminants.8 Generally, the high reactivity and penetration capability stemming from their small size along with difficulties with measurement obscure our understanding of the biological impact of ENPs and INPs. In contrast, viruses have been studied ubiquitously with well-established surrogates and analytical techniques.9 Therefore, we propose that virus surrogates can be used as surrogates to devise strategies for the removal of ENPs and INPs. To this end, the removal efficiencies of the proposed filters in this study for inorganic nanoparticles were compared to that of a virus surrogate.
Although virus removal and inactivation have been studied extensively in water treatment, currently no mandates exist for the removal of ENPs. There is a lack of focus on developing novel techniques for their removal due to (i) the low concentrations of ENPs and INPs in water compared to NNPs, re-shifting the focus onto NNP removal; (ii) the expected high financial burden to replace legacy equipment, and (iii) the multi-barrier approach commonly used in water treatment, that apportions the removal of NPs to either coagulation and sedimentation or disinfection technologies.10
Despite the looming threat of NP contamination, there is currently no single solution to protect humans from exposure. Drinking water treatment offers the opportunity for risk mitigation as inhalation and ingestion of harmful ENPs and INPs released into the atmosphere through water and air is an important route for exposure.11 Conventional water treatment techniques including filtration and coagulation, even when used in tandem, achieve inconsistent removals approximately in the range of 1–98% based on the literature (Table S1†).11–13 Alternate solutions, especially advanced membrane filtration, are proposed as a promising solution to curb NP contamination.11,14,15 Due to the wide range of NPs with various physical and chemical characteristics, it is unlikely that a single technique can achieve complete removal. Additionally, with the emerging focus on the sustainability of clean water production, it is essential to develop novel techniques with low energy requirements.16
Here, we demonstrate the feasibility of using an affinity-based depth filter fabricated from readily available plant-based materials to achieve high-efficiency nanoparticulate removal. These filters were designed to leverage the water purification activity of cationic proteins from Moringa oleifera (MO) seeds. The MO tree and its seed proteins were shown to be an ideal candidate for water treatment due to its wide availability and lack of cytopathic effects.17,18 The MO seeds contain cationic proteins MO coagulant protein (MO2.1) and MO chitin-binding protein (MoCBP) with established antifungal and coagulant activities.17,19,20 In our earlier studies, we developed and tested the capability of MO-functionalized natural fiber filters to achieve energy-efficient pathogen removal from water.21 In this study, the application of the MO-functionalized filter technology was extended to an array of NPs under practically relevant conditions to build a novel platform technology that can be applied at community or point-of-use scales. The proposed MO-functionalized filters were shown to achieve >5
log10, >4
log10, and 6.86 ± 0.04
log10 removals of 200 nm polystyrene nanoparticles (INPs), 50 nm silver nanoparticles (ENPs), and T7 bacteriophage (NNPs), respectively, exhibiting the potential to achieve highly effective nanoparticle removal. In addition to establishing the feasibility to achieve high removals, results show that the removals achieved are consistent at loading rates typical to water treatment (10 m h−1) under a range of salinities (1–20 mM NaCl) and pH values (5.5–8.5). Lastly, we demonstrated the capability of regeneration by washing the filters with 600 mM NaCl solution to detach the proteins and attached nanoparticles before re-functionalizing fibers with MO proteins.
Materials and methods
Moringa oleifera seeds
Moringa oleifera (MO) seeds were purchased from the Echo Global Farm in Florida, United States. The seeds have been stored at −80 °C for long-term storage. Once opened for use, they have been stored at room temperature in a sealed bag for the preparation of MO-functionalized cotton filters. Note that crushed seed powder was prepared freshly for each experiment. The storage at room temperature and preparation of fresh seed powder was followed to ensure the conditions in this study closely replicate field scale operation.
Cotton fiber
The unprocessed cotton used as a filter substrate was purchased from a local store. The link for purchase is available in ESI Materials and methods.† The chemical and surface characterization of cotton used, available in our previous study,21 shows that the cotton fiber purchased from the local store matches the characteristics of a standard cotton sample.
Model nanoparticles for filtration experiments
To test the removal of ENPs and INPs using MO-functionalized cotton filters, we used two commercially available nanoparticles.
(1) Carboxylate-modified polystyrene latex particles with 200 nm diameter from Invitrogen™ (sPsL particles).
(2) Polyvinyl-pyrrolidone-coated silver nanoparticles with 50 nm diameter from NanoComposix, San Diego (AgNPs).
In addition to the inorganic nanoparticles used to test the MO-functionalized cotton filters, T7 bacteriophage was used as a surrogate for natural nanoparticles to establish the high removal efficiency. T7 bacteriophage is a double-stranded DNA virus with an icosahedral head of ∼60 nm and a noncontractile tail approximately 20 nm long and 10 nm wide22 widely used in biological research.22,23 In addition to the low risk of infection and well-established protocols, T7 bacteriophage has been used as a surrogate for human viruses making it an ideal surrogate for filtration experiments.24,25 The T7 bacteriophage filtration, culture, and quantification protocols used in this study are available in the ESI Materials and methods.†
MO-functionalized cotton filter preparation
Readily available glass chromatography columns from Bio-Rad Laboratories, Inc. were used to prepare the filters. Approximately six cotton balls weighing ∼3.5 g were immersed in deionized (DI) water for 10 minutes. The wet cotton fiber was then packed into a glass chromatography column with dimensions of 1.5 cm inner diameter and 10 cm length using a plunger. The height of the cotton column thus prepared was measured to be ∼8 cm.
To functionalize the cotton filter with MO proteins, 100 mL of water extract from dry Moringa seeds was pumped through each column at a flow rate of 2 mL min−1. MO water extract was prepared by mixing 2 g of freshly ground unshelled MO seed with 100 mL of DI water for 5 minutes. After mixing, the solution was filtered sequentially through a 1.5 μm glass fiber filter (Whatman) and 0.22 μm PVDF filter (Millipore) to remove the excess seed material.
Quantification of nanoparticle removal with dynamic light scattering analysis
Standard filtration experiments were used to quantify the nanoparticle removal efficiency of MO-functionalized cotton filters. After preparation, a MO-functionalized cotton filter was equilibrated with 100 mL of the background buffer, 1 mM NaCl solution, at the required flow rate. Then the influent solution containing either ∼1010 #/mL of 200 nm sPsL particles or 50 nm AgNPs dispersed in the background buffer was filtered at a constant flow rate. The effluent solution was collected into two fractions of 50 mL from the 100 mL of influent passed through the filter. Experimental log removal efficiency (LRE) of the filters was quantified using eqn (1), where C and C0 are the nanoparticle concentrations in the effluent and influent samples. The concentration of the nanoparticles was measured using a calibration curve developed on dynamic light scattering (DLS). |  | (1) |
To establish the feasibility of NP removal, experiments were performed at a flow rate of 2 mL min−1. Unfunctionalized cotton filters prepared using the same procedure were used as a negative control. To understand the effect of flow rate on the nanoparticle removal, column experiments were performed at varying flow rates in the range of 2 mL min−1 to 60 mL min−1.
Nanoparticle concentration measurement using dynamic light scattering
Dynamic Light Scattering (DLS) is a standard method used for the quantification of the size and zeta potential of sub-micron particles. To quantify the nanoparticle removal efficiency quickly and accurately, a calibration curve was developed on Zetasizer Nano ZS to correlate the number of photons measured per second in a specific sample to its concentration. The count rate or the number of photons detected per second, usually monitored as kilo counts per second, is a key result of a DLS measurement. Note that to achieve a consistent count rate across measurements, two measurement parameters, the intensity of the laser and the distance of the detector to the sample, need to be held constant. For a Zetasizer Nano ZS, these parameters are represented by the attenuator index and measurement position. For achieving calibration curves, we measured varying concentrations of sPsL particles and AgNPs with a fixed measurement position of 4.65 mm and attenuator indices of 8 and 11.
Quantification of nanoparticle removal with high-resolution analysis
As the concentration of the effluent samples from MO-functionalized cotton is below the limit of detection of standard DLS analysis, we used alternate techniques to validate the removal efficiency from the DLS analysis. In the case of sPsL particles, the effluent sample was concentrated by a factor of 10 by ultracentrifuging 40 mL of the sample at 200
000 g for 30 minutes and resuspending in 4 mL of 1 mM NaCl. The 10 times concentrated effluent was analyzed to quantify the removal efficiencies. For silver nanoparticles (AgNPs), Inductively Coupled Plasma Mass Spectrometry (ICP-MS) is a high-resolution analysis used frequently in the literature.26,27 Additional filtration experiments similar to those described earlier were performed with AgNPs dispersed in 1 mM NaCl at a concentration of 1 mg mL−1. The concentration of AgNPs in the influent and effluent samples was measured by using ICP-MS after diluting to the appropriate concentration into a 2% HNO3 solution in DI water.
Scanning electron microscopy
To test the hypothesis that the NPs tested were physically removed by the MO-functionalized cotton filters, we used scanning electron microscopy (SEM) to visualize the adsorption of sPsL particles and AgNPs on the surface of MO-functionalized cotton. The MO-functionalized cotton fiber with adsorbed sPsL particles and AgNPs was prepared by filtering 100 mL of 1010 #/mL sPsL particles and 1 mg mL−1 of AgNPs with a MO-functionalized cotton filter at 2 mL min−1. Individual fibers from the top of the column were picked with clean tweezers and dried at 35 °C. Dried cotton samples were coated with a gold/palladium mixture using EMS Sputter Coater and SEM images were acquired using Quanta 650 ESEM (FEI) and Hitachi S-5500 SEM/STEM based on the particle size at an acceleration voltage of 5 kV to 15 kV.
Size and zeta potential of the nano-contaminants
The size and zeta potential of ∼1010 #/mL, ∼1011 #/mL, and ∼1010 PFU mL−1 of sPsL 200 nm particles, 50 nm AgNPs, and T7 bacteriophage dispersed in 1 mM NaCl were measured using Zetasizer Nano ZS. T7 bacteriophage samples were buffer exchanged using 50 kDa AMICON spin columns to switch the buffer from culture media to 1 mM NaCl without decreasing the concentration. After buffer exchange, the samples were filtered through a 0.22 μm syringe filter to remove any coagulated viruses that can affect the analysis. Note that unbuffered 1 mM NaCl was used as a dispersant for the size and zeta potential measurements to capture the behavior of the contaminants under the conditions used for filtration experiments performed for initial feasibility and electron microscopy.
Quantification of nanoparticle removal dependence on pH and salt concentration
After the nanoparticle removal capability of MO-cotton filters was established at various loading rates in 1 mM NaCl, additional filtration experiments were conducted at a flow rate of 30 mL min−1 by dispersing the nanoparticles in DI water with 2 mM, 5 mM, and 10 mM NaCl. To study the effect of pH, 10 times diluted phosphate-buffered saline (PBS) adjusted to the required pH (5.5, 6.5, 7.5, and 8.5) was used as the dispersant, and filtration experiments were run at 30 mL min−1. A 1010 #/mL influent concentration of 200 nm sPsL nanoparticles was used for all the experiments performed to study the effect of salt concentration and pH.
Regeneration feasibility of MO-functionalized cotton
For the proposed filters in this study to be sustainable in practice it is important to establish a regeneration procedure to reuse the cotton substrate. Previous studies have shown that when washed with 600 mM NaCl solution, MO proteins can be desorbed from the surface as the underlying electrostatic interactions between the substrate and cationic proteins will decrease.21,28,29 Following this hypothesis, experiments were conducted to wash the MO-functionalized filters with 600 mM NaCl and then re-functionalize them with 100 mL MO serum as described earlier. The nanoparticle removal efficiency was quantified at a flow rate of 30 mL min−1 with 1010 #/mL 200 nm sPsL particles dispersed in 0.1XPBS buffer pH 7 over three cycles of washing and regeneration.
Results and discussion
Moringa oleifera functionalized cotton filters achieve high-efficiency nanoparticle removal
The key finding of this study is that depth filters packed with cotton functionalized with MO seed cationic proteins achieve nanoparticle removal orders of magnitude higher compared to the unfunctionalized cotton filters. To establish the NP removal efficiency of MO-functionalized cotton filters, calibration curves developed using Dynamic Light Scattering analysis (DLS) were used to quantify the influent and effluent concentrations. Results from the DLS calibration for the two inorganic nanoparticles tested in this study (Fig. S1†) show that when operated at the highest resolution possible i.e., with an unattenuated laser, the lowest concentrations detectable for the sPsL particles and AgNPs are 1 × 106 #/mL and 1 × 107 #/mL respectively. Standard column experiments performed with MO-functionalized cotton filters and unfunctionalized cotton filters show that at a flow rate of 2 mL min−1 which corresponds to a superficial velocity of 0.68 m h−1, MO-functionalized cotton filters achieve significantly higher removals compared to the unfunctionalized cotton filters (Fig. 1A). As the concentration of the samples was below the limit of detection, the results show that MO-functionalized cotton filters achieve removals >4
log10 (99.99%) and >3
log10 (99.9%) for sPsL particles and AgNPs compared to the −0.03 ± 0.01
log10 and −0.00 ± 0.01
log10 achieved by the unfunctionalized cotton. The high efficiency reported here is the first report of nanoparticle removal with a simple plant material-based filter and is significantly higher than conventional water treatment reported in the literature as shown in Table S1†.11,12,30
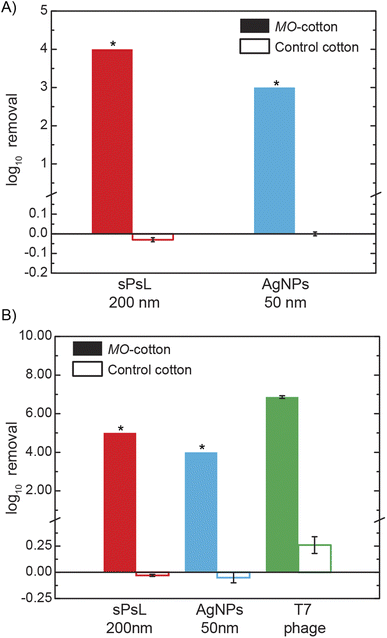 |
| Fig. 1
Moringa oleifera protein functionalized cotton filters achieve high-efficiency removal of nano contaminants tested in this study. (a) Experimental log10 removal of 1010 #/mL sPsL particles and AgNPs using MO-functionalized cotton filters compared to that of unfunctionalized filters at a flow rate of 2 mL min−1 showed MO-functionalized cotton filters achieve >4 log10 and >3 log10 removal compared to insignificant removal achieved by unfunctionalized cotton filters based on the calibration curves developed using Dynamic Light Scattering analysis to measure their concentration. (b) Experimental log10 removal of 1010 #/mL sPsL particles and AgNPs using MO-functionalized fiber filters compared to that of uncoated fiber filters at a flow rate of 2 mL min−1 showed MO-functionalized cotton filters achieve >5 log10 and >4 log10 using higher-resolution analysis for concentration measurement. The sPsL particle effluent was concentrated by a factor of 10 using centrifugation and inductively coupled plasma mass spectrometry was used for measuring silver concentration. MO-functionalized cotton filters also show 6.86 ± 0.04 log10 removal of T7 bacteriophage at a flow rate of 2 mL min−1 *Indicates that the effluent concentration was below the limit of detection which indicates that the actual removal, in this case, could be higher than the reported values. Error bars represent the standard deviation calculated from three independent measurements. | |
Note that the filtration experiments performed here aim to establish the high filtration removal of MO-cotton filters proposed using a highly concentrated influent solution (1010 #/mL). Traditionally, breakthrough experiments are conducted with influent concentrations typical in the environment, but such experiments are not feasible for NPs due to a lack of techniques for quantifying low concentrations, and long-term filtration experiments at high concentrations would be cost-intensive. The high-resolution techniques used for effluent concentration quantification in this study can only measure the lowest concentrations in the range of 106 #/mL. As discussed in the following sections the filtration mechanism of the proposed filters is based on electrostatic interactions and the sticking coefficient calculations based on experimental data and clean bed filtration theory indicate high attachment efficiency of MO cotton (Table S2†). This indicates that the MO cotton filters would be effective against low concentrations of NPs as the influent concentration is not a determining factor for removal efficiency according to clean bed filtration theory.31,32
Higher-resolution analyses including ICP-MS and infectivity assays corroborate the removals quantified using DLS analysis
After establishing the high nanoparticle removal of MO-functionalized cotton filters using DLS analysis which enables quick screening, alternate analytical techniques, and contaminants were used to ascertain the results. First, the removal efficiency of AgNPs was quantified using ICP-MS to analyze the concentration of silver in the samples. The results confirmed that when an influent solution of 1 mg mL−1 (1 ppm) of AgNPs was filtered through a MO-functionalized cotton filter, effluent concentration was lower than the limit of detection of ICP-MS, 0.1 ppb (>4
log10). As ICP-MS analysis is ineffective for the quantification of sPsL particles, the effluent from the filtration experiments was concentrated by a factor of 10 and reanalyzed with DLS to confirm the removal. As shown in Fig. 1B, ICP-MS and DLS analysis on concentrated effluent showed that MO-functionalized filters achieve >4
log10 and >5
log10 removal for AgNPs and sPsL particles which agrees with initial experiments (Fig. 1A).
Additionally, T7 bacteriophage with well-established quantification techniques was used as a surrogate for natural nanoparticles/viruses.22 Using a double-layer agar infectivity assay, the T7 removal efficiency of MO-functionalized cotton filters was analyzed to compare it to the inorganic NPs. Fig. 1B results show a removal efficiency of 6.86 ± 0.04
log10 in MO-functionalized cotton filters. Overall, multiple high-resolution analyses show that MO-functionalized cotton filters can achieve highly efficient nanoparticulate removal from water.
Scanning electron microscopy and zeta potential measurements indicate the physical removal of nanoparticles based on electrostatic interactions
Based on the results discussed to this point, it is evident that MO proteins adsorbed on the surface of cotton are responsible for the removal of nanoparticles. The cationic nature of MO seed proteins is reported widely in the literature, and we showed in previous studies that two cationic proteins, MO2.1 and MoCBP, adsorb onto the surface of cotton.17,19,29,33 SDS-PAGE electrophoresis and mass spectrometry were performed to confirm the presence of MoCBP on the surface of functionalized cotton (Fig. S2 and Table S3†). SEM analysis was used to confirm the physical attachment of nanoparticles to MO-functionalized cotton to show that the electrostatic interactions between the MO proteins and the nanoparticles were responsible for the removal. Fig. 2A and B show sPsL particles and AgNPs adsorbed on the filter substrate after filtration indicating strong interaction. Note that some AgNPs were found to be attached to the unfunctionalized cotton surface as well (Fig. 2B), but the density of particles was much lower compared to the MO-cotton at the same resolution confirming the effect of the MO proteins. Additionally, zeta potential measurements of the contaminants show a negative surface charge under the filtration conditions Fig. 2D. The negative surface charge of the NPs supports the hypothesis that favorable interactions exist between them and MO-functionalized cotton. In contrast, there is no discernible correlation between the size of the contaminants tested and their removal, suggesting that the removal achieved in MO-functionalized cotton filters is not dominated by size exclusion (Fig. 1B and 2C).
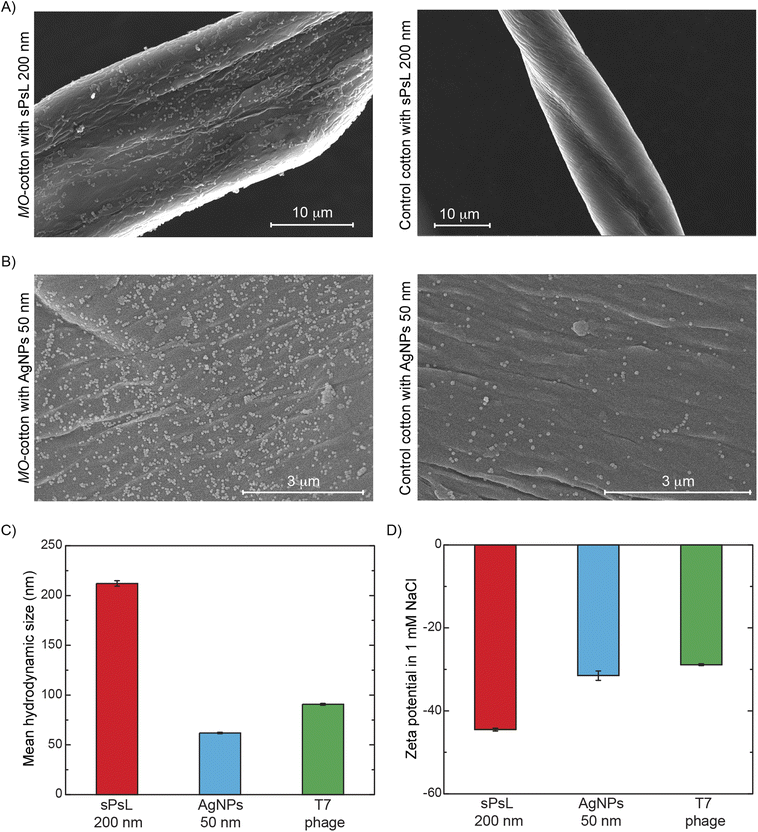 |
| Fig. 2
Moringa oleifera protein functionalized cotton filters achieve removal of nano contaminants due to electrostatic interactions between proteins adsorbed on the filter substrate and the contaminants. (A and B) Scanning electron microscopy images of MO-functionalized cotton samples taken from a filter after filtering sPsL particles and AgNPs shows the adherence of the same to the surface of MO-functionalized cotton indicating favorable interactions between the MO-cotton and the nano-contaminants tested. (C) DLS size analysis of the nano-contaminants tested in the study. The average diameter of sPsL particles, AgNPs, and T7 bacteriophage was measured to be 212.13 ± 2.77 nm, 61.89 ± 0.72 nm, and 90.71 ± 0.87 nm respectively. (D) Zeta potential measurements using DLS for the nano-contaminants tested in the study. The average zeta potential of sPsL particles, AgNPs, and T7 was measured to be −44.50 ± 0.36 mV, −31.50 ± 1.14 mV, and −28.87 ± 0.21 mV respectively. | |
MO-functionalized cotton filters can be operated at loading rates typical in conventional water treatment
Once the effectiveness of MO-functionalized cotton filters was established, experiments were conducted at varying flow rates to study the effect of flow rate on removal. The results showed that the proposed filters can achieve >99% removal at superficial velocities typical to rapid sand filtration used widely in drinking water treatment (∼10–15 m h−1). MO-cotton filters achieve 2.80 ± 0.13
log10 removal of sPsL particles at a superficial velocity of 16.98 m h−1 and 2.29 ± 0.21
log10 removal of AgNPs at a superficial velocity of 20.37 m h−1 (Fig. 3A and B). This indicates that MO-cotton filters can potentially be used as an alternative or in addition to rapid sand filtration in conventional water treatment. To study the effect of the background water matrix on the removal efficiency of MO-cotton filters, column experiments were performed in the presence of divalent cations and anions (Mg2+, Ca2+, SO42−, and SiO32−) with Na2SiO3, Na2SO4, MgCl2, and CaCl2 as background buffers in addition to a groundwater sample (Fig. S3 and S4†). The results show that MO-cotton filters achieve 1.43 ± 0.10, 2.55 ± 0.08, 3.23 ± 0.11, and >3.71
log10 removals respectively in the presence of Na2SiO3, Na2SO4, MgCl2, and CaCl2 compared to >4
log10 in the presence of monovalent ions (NaCl). Even though there was a slight decrease in performance due to divalent anions, the groundwater matrix with a total organic content of 2.21 ± 0.43 mg L−1 (Table S4†) did not show any effect on performance. The effect of individual divalent ions on the removal mechanism needs to be studied further. Additionally, future studies are required to study the fouling behavior and interaction with other contaminants present in realistic water samples.
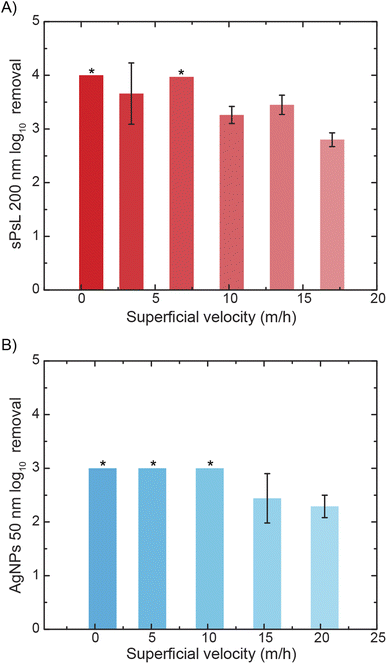 |
| Fig. 3 MO-functionalized cotton filters show high efficiencies of nanoparticle removal at superficial velocities typical in drinking water treatment. (A) Experimental log10 removal of sPsL particles using MO-functionalized cotton filters at various flowrates in the range of 2 mL min−1 to 50 mL min−1 showed that MO-cotton filters achieve 2.80 ± 0.13 log10 removal of sPsL particles at a flow rate of up to 50 mL min−1 which corresponds to a superficial velocity of 16.98 m h−1 (B) Experimental log10 removal of AgNPs using MO-functionalized cotton filters at various flowrates in the range of 2 mL min−1 to 60 mL min−1 showed that MO-cotton filters achieve 2.29 ± 0.21 log10 removal of AgNPs at a flow rate of up to 60 mL min−1 which corresponds to a superficial velocity of 20.37 m h−1. When compared to the typical operating conditions of two widely used conventional filters, rapid sand filtration (5–15 m h−1) and slow sand filtration (0.1–0.4 m h−1), MO-functionalized cotton filters show the potential to be applicable in conventional water treatment. *Indicates that the effluent concentration was below the limit of detection which indicates that the actual removal, in this case, could be higher than the reported values. Error bars represent the standard deviation calculated from three independent measurements. | |
Clean bed filtration models are used ubiquitously in literature to quantify the sticking coefficient or attachment efficiency of contaminants to filter media.31,32 Sticking coefficient values are an important tool that enables the design of filter dimensions to meet the removal requirements. From the removal efficiencies achieved by the MO-cotton filters at the highest flow rates tested in this study, the sticking coefficient values for sPsL particles and AgNPs are calculated to be 0.13 and 0.08. These values are orders of magnitude higher than the sticking coefficient values of the sPsL particles and AgNPs in unfunctionalized cotton filters (Table S2†). The increased attachment efficiency of the nanoparticles represents the strong interaction with the MO proteins as described earlier.21
MO-functionalized cotton filters exhibit consistent removals under a range of salt concentrations and pH values typical to drinking water treatment
The removal efficiency of MO-cotton filters against 200 nm sPsL particles was tested at 30 mL min−1 across a range of salinities and pH values encouraged by their performance at high loading rates. Filtration experiments performed with salt concentrations in the range of 1–20 mM NaCl showed that MO-cotton filters achieve ∼4
log10 removal of plastic nanoparticles in the salinities typical to surface water purification which indicates that the proposed filters have the potential for application in drinking water treatment (Fig. 4A). Further experiments were performed using pH adjusted 10 times diluted PBS buffer (pH values of 5.5, 6.5, 7.5, and 8.5) as the dispersant. These results demonstrate that pH conditions show no significant effect on the removal efficiency achieved (Fig. 4B). Overall, the effect of salinity and pH on the favorable interactions between NPs and MO proteins was found to be negligible in the background conditions that represent surface water treatment. Although the potential of MO-functionalized filters is established by performing filtration experiments at various conditions during this study, future studies are necessary to study the effect of realistic water matrices on the efficiency and life of the proposed filters.
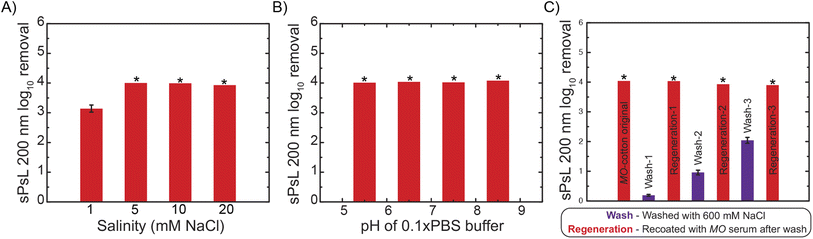 |
| Fig. 4 MO-functionalized cotton filters achieve high efficiencies of nanoparticle removal at various salt concentrations and pH values in addition to regeneration capability. (A) Experimental log10 removal of sPsL particles using MO-functionalized cotton filters at various salt concentrations in the range of 1 mM NaCl to 20 mM NaCl and a flow rate of 30 mL min−1 showed that MO-cotton filters achieve high removal of sPsL particles in conditions pertinent to surface water treatment. (B) Experimental log10 removal of sPsL particles using MO-functionalized cotton filters at various pH values s in the range of 5.5–8.5 and a flow rate of 30 mL min−1 showed a negligible effect of pH on the removal efficiency. pH-adjusted 0.1XPBS buffer was used for these experiments. (C) Experimental log10 removal of sPsL particles using MO-cotton filters at a flow rate of 30 mL min−1 over cycles of regeneration shows that the removal efficiency was consistent up to three cycles. MO-cotton filters achieved >4 log10 removals compared to the washed cotton columns that achieved removals of 0.19 ± 0.03 log10, 0.96 ± 0.08 log10, and 2.04 ± 0.10 log10 during the 1st, 2nd, and 3rd cycles respectively. *Indicates that the effluent concentration was below the limit of detection which indicates that the actual removal could be higher than the reported values. Error bars represent the standard deviation from three independent measurements. | |
MO-cotton filters can be generated up to three cycles by washing with 600 mM NaCl and re-functionalizing with MO serum
It is important to establish robust protocols for the regeneration of the proposed filters so the cotton substrate can be reused to improve the sustainability of the technique. To this end, we hypothesized that washing with 600 mM NaCl would desorb the absorbed nanoparticles and MO proteins by nullifying the electrostatic interactions at high salt concentrations inspired by past literature.28 The cotton filters can then be re-functionalized with MO proteins using the same procedure used to prepare the original MO-cotton to re-use them for filtration. To test this hypothesis, three cycles of regeneration were executed on MO-cotton filters by quantifying the removal of 200 nm sPsL particles at each step. These results indicate that MO-cotton filters achieve consistent removals >4
log10 over the three cycles and that regeneration with 600 mM NaCl wash is a robust process to improve the sustainability of MO-cotton filters in practice (Fig. 4C). The removals achieved by washed cotton increased significantly over the three cycles which could be either due to trace amounts of MO proteins or nanoparticles left on the substrate during the wash step that could result in the removal of particles, but it is important to note that this behavior did not show any pronounced effect on the removals achieved after re-functionalization.
Future efforts are necessary to study the maximum cycles of regeneration possible, any potential effects on pore blockage over time, and residual proteins and nanoparticles after the wash cycles to optimize the process for re-use. More importantly, MO-functionalized cotton filters exhibit various advantages in terms of water treatment such as low energy requirement and dry storage stability in addition to regeneration capability as demonstrated earlier.21 These properties make them a promising technique for drinking water treatment in various scenarios.
Conclusion
In conclusion, this work establishes the feasibility of nanoparticle removal at high efficiency using filters built with plant-based materials. The filtration experiments performed at various flowrates, salinity conditions, and pH conditions demonstrate that MO-cotton filters have the potential for applications in surface water treatment and merit further investigation to identify challenges and considerations to integrate them into conventional filtration. Further, electron microscopy and protein characterization techniques were used to explore the filtration mechanism in this study. Due to the electrostatic nature of the identified it is highly possible that organic matter in water would cause fouling during operation. Experiments performed with various divalent cations, divalent anions, and groundwater samples as background matrices suggest that divalent anions could decrease the removal efficiency slightly but overall, the MO cotton filters are effective in removing nanoparticles from a realistic groundwater matrix. Detailed future studies with various background matrices and pre-filtration steps to reduce potential fouling would be necessary for ensuring sustained long-term operation. Additionally, preliminary analysis showed that a household filter with 10 L day−1 capacity can be fabricated for a cost of $ 5–10 in both the US and India.21 Although future work is necessary to understand their performance in the field scale with realistic water, the proposed technology of MO-cotton filters offers a low-energy and accessible solution for the growing nanoparticle contamination of water sources.
Author contributions
L. S., S. V., and M. K. conceived and designed the research. L. S., S. D., and M. D., performed the experiments with the assistance of R. P. M., S. P., and Y.-M. T. L. S., S. V., and M. K. analyzed the data. L. S., S. V., and M. K. co-wrote the paper with editing help and comments from S. D., M. D., Y.-M. T., R. P. M., and D. G.
Conflicts of interest
There are no conflicts to declare.
Acknowledgements
The authors would like to thank Echo Global Farm, Florida for providing Moringa oleifera seeds. This work was supported by funding from the US National Science Foundation through the grants CBET-12022971, CBET-2027731, and CBET-1946392.
References
- D. Mitrano, Nanoplastic should be better understood, Nat. Nanotechnol., 2019, 14, 299 CrossRef PubMed.
-
S. Adhikari, S. Mandal, D.-H. Kim and A. K. Mishra, An overview of treatment technologies for the removal of emerging and nanomaterials contaminants from municipal and industrial wastewater, Emerging and Nanomaterial Contaminants in Wastewater, 2019, pp. 3–40 Search PubMed.
- A. D. Maynard, R. J. Aitken, T. Butz, V. Colvin, K. Donaldson and G. Oberdörster,
et al., Safe handling of nanotechnology, Nature, 2006, 444(7117), 267–269 CrossRef CAS PubMed.
-
M. R. Wiesner, G. V. Lowry, P. Alvarez, D. Dionysiou and P. Biswas, Assessing the Risks of Manufactured Nanomaterials, ACS Publications, 2006 Search PubMed.
- D. M. Mitrano, P. Wick and B. Nowack, Placing nanoplastics in the context of global plastic pollution, Nat. Nanotechnol., 2021, 16(5), 491–500 CrossRef CAS PubMed.
- A. Azimzada, J. M. Farner, M. Hadioui, C. Liu-Kang, I. Jreije and N. Tufenkji,
et al., Release of TiO2 nanoparticles from painted surfaces in cold climates: characterization using a high sensitivity single-particle ICP-MS, Environ. Sci.: Nano, 2020, 7(1), 139–148 RSC.
- R. H. Waring, R. Harris and S. Mitchell, Plastic contamination of the food chain: A threat to human health?, Maturitas, 2018, 115, 64–68 CrossRef CAS PubMed.
- K. E. Jones, N. G. Patel, M. A. Levy, A. Storeygard, D. Balk and J. L. Gittleman,
et al., Global trends in emerging infectious diseases, Nature, 2008, 451(7181), 990–993 CrossRef CAS PubMed.
- B. R. McMinn, N. J. Ashbolt and A. Korajkic, Bacteriophages as indicators of faecal pollution and enteric virus removal, Lett. Appl. Microbiol., 2017, 65(1), 11–26 CrossRef CAS PubMed.
- P. Westerhoff, A. Atkinson, J. Fortner, M. S. Wong, J. Zimmerman and J. Gardea-Torresdey,
et al., Low risk posed by engineered and incidental nanoparticles in drinking water, Nat. Nanotechnol., 2018, 13(8), 661–669 CrossRef CAS PubMed.
- Z. Ma, X. Yin, X. Ji, J.-Q. Yue, L. Zhang and J.-J. Qin,
et al., Evaluation and removal of emerging nanoparticle contaminants in water treatment: a review, Desalin. Water Treat., 2016, 57(24), 11221–11232 CrossRef.
- T. E. A. Chalew, G. S. Ajmani, H. Huang and K. J. Schwab, Evaluating Nanoparticle Breakthrough during Drinking Water Treatment, Environ. Health Perspect., 2013, 121(10), 1161–1166 CrossRef PubMed.
- E. Arkhangelsky, I. Levitsky and V. Gitis, Considering energy efficiency in filtration of engineering nanoparticles, Water Sci. Technol.: Water Supply, 2017, 17(5), 1212–1218 CAS.
- A. C. C. Bortolassi, S. Nagarajan, B. de Araújo Lima, V. G. Guerra, M. L. Aguiar and V. Huon,
et al., Efficient nanoparticles removal and bactericidal action of electrospun nanofibers membranes for air filtration, Mater. Sci. Eng., C, 2019, 102, 718–729 CrossRef CAS PubMed.
- D. A. Gopakumar, D. Pasquini, M. A. Henrique, L. C. de Morais, Y. Grohens and S. Thomas, Meldrum's Acid Modified Cellulose Nanofiber-Based Polyvinylidene Fluoride Microfiltration Membrane for Dye Water Treatment and Nanoparticle Removal, ACS Sustainable Chem. Eng., 2017, 5(2), 2026–2033 CrossRef CAS.
- Y. Liu, M. Hejazi, P. Kyle, S. H. Kim, E. Davies and D. G. Miralles,
et al., Global and regional evaluation of energy for water, Environ. Sci. Technol., 2016, 50(17), 9736–9745 CrossRef CAS PubMed.
- A. Ndabigengesere, K. S. Narasiah and B. G. Talbot, Active agents and mechanism of coagulation of turbid waters using Moringa oleifera, Water Res., 1995, 29(2), 703–710 CrossRef CAS.
- P. M. Ferreira, A. F. Carvalho, D. F. Farias, N. G. Cariolano, V. M. Melo and M. G. Queiroz,
et al., Larvicidal activity of the water extract of Moringa oleifera seeds against Aedes aegypti and its toxicity upon laboratory animals, An. Acad. Bras. Cienc., 2009, 81, 207–216 CrossRef PubMed.
- J. M. Gifoni, J. T. Oliveira, H. D. Oliveira, A. B. Batista, M. L. Pereira and A. S. Gomes,
et al., A novel chitin-binding protein from Moringa oleifera seed with potential for plant disease control, Pept. Sci., 2012, 98(4), 406–415 CrossRef CAS PubMed.
- J. X. Neto, M. L. Pereira, J. T. Oliveira, L. C. Rocha-Bezerra, T. D. Lopes and H. P. Costa,
et al., A chitin-binding protein purified from Moringa oleifera seeds presents anticandidal activity by increasing cell membrane permeability and reactive oxygen species production, Front. Microbiol., 2017, 8, 980 CrossRef PubMed.
- L. Samineni, S. De Respino, Y.-M. Tu, R. Chowdhury, R. P. Mohanty and H. Oh,
et al., Effective pathogen removal in sustainable natural fiber Moringa filters, npj Clean Water, 2022, 5(1), 27 CrossRef CAS.
- A. Rosenberg, K. Griffin, F. W. Studier, M. McCormick, J. Berg and R. Novy,
et al., T7Select phage display system: a powerful new protein display system based on bacteriophage T7, Innovations, 1996, 6, 1–6 Search PubMed.
- S. Kilcher and M. J. Loessner, Engineering bacteriophages as versatile biologics, Trends Microbiol., 2019, 27(4), 355–367 CrossRef CAS PubMed.
-
M. M. Mesquita and M. B. Emelko, Bacteriophages as Surrogates for the Fate and Transport of Pathogens in Source Water and in Drinking Water Treatment Processes, InTech, 2012 Search PubMed.
- C. Lytle, S. Tondreau, W. Truscott, A. Budacz, R. Kuester and L. Venegas,
et al., Filtration sizes of human immunodeficiency virus type 1 and surrogate viruses used to test barrier materials, Appl. Environ. Microbiol., 1992, 58(2), 747–749 CrossRef CAS PubMed.
- D. M. Mitrano, A. Barber, A. Bednar, P. Westerhoff, C. P. Higgins and J. F. Ranville, Silver nanoparticle characterization using single particle ICP-MS (SP-ICP-MS) and asymmetrical flow field flow fractionation ICP-MS (AF4-ICP-MS), J. Anal. At. Spectrom., 2012, 27(7), 1131–1142 RSC.
- R. J. Peters, Z. H. Rivera, G. van Bemmel, H. J. Marvin, S. Weigel and H. Bouwmeester, Development and validation of single particle ICP-MS for sizing and quantitative determination of nano-silver in chicken meat, Anal. Bioanal. Chem., 2014, 406(16), 3875–3885 CAS.
- B. A. Nordmark, T. M. Bechtel, J. K. Riley, D. Velegol, S. B. Velegol and T. M. Przybycien,
et al., Moringa oleifera Seed Protein Adsorption to Silica: Effects of Water Hardness, Fractionation, and Fatty Acid Extraction, Langmuir, 2018, 34(16), 4852–4860 CrossRef CAS PubMed.
- L. Samineni, B. Xiong, R. Chowdhury, A. Pei, L. Kuehster and H. Wang,
et al., 7 log virus removal in a simple functionalized sand filter, Environ. Sci. Technol., 2019, 53(21), 12706–12714 CrossRef CAS PubMed.
- H.-H. Chang, T.-J. Cheng, C.-P. Huang and G.-S. Wang, Characterization of titanium dioxide nanoparticle removal in simulated drinking water treatment processes, Sci. Total Environ., 2017, 601, 886–894 CrossRef PubMed.
- N. Tufenkji and M. Elimelech, Correlation equation for predicting single-collector efficiency in physicochemical filtration in saturated porous media, Environ. Sci. Technol., 2004, 38(2), 529–536 CrossRef CAS PubMed.
- B. E. Logan, D. Jewett, R. Arnold, E. Bouwer and C. O'Melia, Clarification of clean-bed filtration models, J. Environ. Eng., 1995, 121(12), 869–873 CrossRef CAS.
- S. De Respino, L. Samineni, Y.-M. Tu, H. Oh and M. Kumar, Simultaneous Removal of Oil and Bacteria in a Natural Fiber Filter, Environ. Sci. Technol. Lett., 2021, 9(1), 77–83 CrossRef.
|
This journal is © The Royal Society of Chemistry 2023 |
Click here to see how this site uses Cookies. View our privacy policy here.