DOI:
10.1039/D2VA00257D
(Perspective)
Environ. Sci.: Adv., 2023,
2, 151-161
Policy options to account for multiple chemical pollutants threatening biodiversity
Received
24th October 2022
, Accepted 2nd December 2022
First published on 5th December 2022
Abstract
Chemical pollution poses a threat to biodiversity on a global scale. This has been acknowledged in the Post-2020 Global Biodiversity Framework, which proposes to regulate the release of chemicals to the environment and names specific indicators focusing on pesticides, nutrients and plastic waste. We fully welcome the inclusion of these substances but would like to further emphasize that in order to protect biodiversity from hazardous chemicals, the scope of Target 7 should feature a wider range of pollutants that can contribute to biodiversity loss. We propose the inclusion of non-agricultural biocides, per- and polyfluoroalkyl substances (PFASs), toxic metal(loid)s, and endocrine-disrupting chemicals (EDCs). Furthermore, data on emerging pollutants (e.g., rare earth elements, toxic and persistent industrial chemicals, liquid crystal monomers, pharmaceuticals, personal care products) need to be regularly reviewed with the aim to integrate additional pollutants to Target 7 in the case of biodiversity risk. We suggest to amend Target 7 to postulate the aim for the overall reduction of chemical production and emissions, as well as the addition of the aforementioned substance groups of high concern to biodiversity for integration in the Post-2020 Global Biodiversity Framework. We further elaborate on different strategies for the reduction of emissions of hazardous chemicals through chemical simplification and grouping, reduction of chemicals with non-essential use, and innovative synthesis strategies (e.g., “benign-by-design”). In this context, the full life cycle of chemicals (i.e., production, use, and end of life) needs to be considered. Lastly, we propose to set up transparent data inventories, in cooperation with the industry, to inform about the production, transport and emissions of chemicals, which can serve as a basis for indicators related to monitoring the progress towards achieving the goals set under Target 7.
Environmental significance
Environmental pollution has been identified as major driver of global biodiversity loss. However, the impact of different chemical substance groups on biodiversity is often not fully accounted for in policy actions. In order to protect biodiversity from chemical pollution, a prioritization of chemicals that impact biodiversity is necessary. We fully endorse the inclusion of these substances already included in Target 7 of the Post-2020 Biodiversity Framework (pesticides, nutrients, plastic waste), but elaborate that in order to prevent biodiversity loss, the target should feature other groups of pollutants. Additionally, we present different approaches on how the emission of hazardous chemicals can be reduced and remaining emissions monitored.
|
Outline
Reducing chemical pollution is essential for protecting biodiversity and ecosystems globally.1 In this article, we draw the attention of policy makers, technical experts, and practitioners working in the field of biodiversity conservation to the global threat of chemical pollution. We support the inclusion of Target 7 on pollution in the current draft Post-2020 Global Biodiversity Framework.2 However, we note that the current draft of Target 7 with its accompanying headline indicators for monitoring emphasizes nutrients, pesticides and plastic waste only. These pollutants are indeed important contributing factors to the threat of biodiversity. Nonetheless, they are only a subset of chemical pollution that should be regulated and monitored to protect the environment and mitigate biodiversity loss. Our paper aims to clarify the implications of the current restricted scope of Target 7, and suggest a science-based extension in scope needed to address the contribution of the global releases of anthropogenic chemicals to the loss of biodiversity. We present several options that can guide policy actions on the interlinked issue of global biodiversity loss and chemical pollution. While many chemicals also impair human health3 and cause long-term costs to society,4 our discussion is restricted to ecological and ecotoxicological aspects. We bring the following items to the attention of delegates at the forthcoming meeting of the Conference of the Parties to the Convention on Biological Diversity (COP 15) in December 2022, which aims to finalize the Post-2020 Global Biodiversity Framework:
(1) An overemphasis on nutrients, pesticides and plastic waste, as expressed in the current draft of Target 7, is insufficient for a full understanding and tackling of the relationship between chemical pollution and global biodiversity loss.
(2) We therefore strongly recommend that at least several other major groups of hazardous chemicals should be explicitly included in Target 7.
(3) We recommend the Conference of the Parties to the Convention on Biological Diversity at its next meeting (COP 15) to trigger an in-depth analysis of linkages with other existing normative instruments such as the chemicals-related conventions on biodiversity conservation that would benefit achieving Target 7.
(4) We suggest suitable indicators and measures to reduce threats from anthropogenic chemicals to biodiversity.
(1) Need to expand Target 7 of the Post-2020 Global Biodiversity Framework
Environmental pollution by anthropogenic chemicals is a major agent of global change5 and an important driver of biodiversity loss.6 This has been recognized in the negotiations of the Post-2020 Global Biodiversity Framework,7 in which the current draft Target 7 addresses pollution and calls for the reduction of pesticide and nutrient inputs to the environment along with the elimination of plastic waste. However, to focus solely on these three chemical groups is insufficient for achieving protection because of the continuous rise in the production, use and release of a diverse and wide range of other chemicals. Specifically, chemical production has increased about 50 times from 1950 to 2010 and is expected to double by 2030, with respect to the production volumes of 2019.8 Production has resulted in an enormous variety of 350
000 chemicals and chemical mixtures that have been registered for production and use around the world.9 Evidence shows that chemical pollution, throughout the life cycle of chemicals, is causing direct and indirect impacts that can result in the instability of populations and community shifts10 in the wild, and thus, impair ecosystem services.11,12 Hazardous chemicals can also lead to the extinction of species, and these impacts may be increasingly exacerbated as the resilience of species is reduced because of additional stressors such as global warming.13,14 Thus, by excluding the immense variety of anthropogenic chemicals, the draft Global Biodiversity Framework fails to address their significant impact on biodiversity.
Sigmund et al.1 urged the negotiators of the Framework to broaden the scope of Target 7 to reflect the breadth and complexity of chemical pollution by considering, for example, toxic metals, chemicals used in industrial processes (such as solvents) and consumer products (such as flame retardants or impregnation agents), and pharmaceuticals, as well as the (often unknown) transformation products formed during production, use, and disposal of chemicals and related products. To achieve the 2030 mission of the Post-2020 Global Biodiversity Framework ‘to put biodiversity on a path to recovery for the benefit of the planet and people,’ it is critical to broaden the scope of chemical pollutants under the Framework. Only by combatting the multitude and multifaceted effects of anthropogenic chemicals and their distribution across the planet, may the 2050 Vision of ‘Living in Harmony with Nature’ be achieved.15 The complexity and pressing nature of the pollution problem may require strengthening and optimizing of existing collaborative links between relevant organizations and stakeholders, both within and outside the UN system.
(2) Groups of hazardous chemicals to explicitly include in Target 7
Pesticides, nutrients and plastics, as mentioned in Target 7, all have a distinct impact on global biodiversity as briefly summarized below. Thus, we fully welcome the inclusion of pesticides, nutrients, and plastics into the framework draft. However, Target 7 needs to be expanded to include additional groups of chemicals that cause environmental harm (Fig. 1). Several important groups for inclusion are outlined below. It is key to note that many chemical substances of high persistence can lead to almost irreversible, long-term global problems, similar to plastic pollution. Our strategy to prioritize chemicals of biodiversity concern to be included in Target 7 is based on the following criteria: (a) chemicals for which evidence of environmental harm has been demonstrated, (b) chemicals of high persistence, and (c) emerging contaminants (see Fig. 1), selected by periodic screening of the scientific literature on toxicity and persistence aspects. Scientific knowledge on the environmental harm of anthropogenic chemicals is evolving rapidly; hence, the list of chemicals for consideration under the Global Biodiversity Framework will therefore need to continue to evolve. Swift priority action regarding the production, use and regulation of these chemicals is warranted.
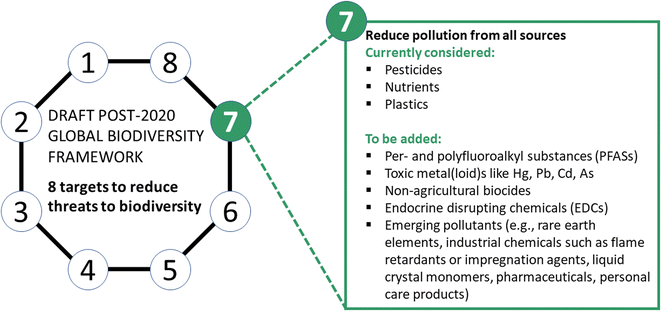 |
| Fig. 1 The eight targets for reducing threats to biodiversity of the draft Post-2020 Global Biodiversity Framework with Target 7 addressing environmental pollution. In the future, beside major hazardous chemicals listed also the exposure to “emerging” or “under-researched” pollutants like rare earth elements and other metals/metalloids, industrial chemicals such as flame retardants, liquid crystal monomers, pharmaceuticals and personal care products might need to be included. This is also true for high-production volume chemicals for which evidence of accumulation in the environment has been demonstrated. | |
Pesticides harm wildlife and ecosystems either by direct toxic effects or indirectly. Arguments to only prohibit the use of the most toxic pesticides fall short because of the indirect effects16–18 of substances, e.g., herbicides reducing the diversity and abundance of non-target flowering plants and corresponding loss of food and habitat resources for insects. Other biocides, not used for crop protection, are contained in many products and uses with high environmental releases, for instance, as disinfectants for human and veterinary hygiene (e.g., quaternary ammonium compounds) or as wood preservatives (e.g., creosote, pentachlorophenol, copper chromate). Non-agricultural biocides used to prevent fouling and material degradation (e.g., copper, isothiazolinones), leach from building facades and are used as preservants of personal care and cleaning products. These uses lead to non-point source releases to the environment, which are difficult to control but clearly impact exposed organisms.19,20 Due to their environmental impacts, non-agricultural biocides also should be included in Target 7.19
In aquatic media, excess nutrients from wastewater or run-off from agricultural land can lead to the formation of algal blooms which, if in surplus, create water bodies with little (hypoxia) or no oxygen, cause green muck that blocks sunlight and increase the turbidity of water, thereby reducing the ability of fish and other aquatic life to find food.21 As a result, entire populations may leave an area or even die. Certain types of harmful algae can create toxins that are detrimental to fish and other animals.22
Wildlife can be entangled by plastic materials and suffers from starvation because of ingested plastic debris.23,24 Weathering processes cause the fragmentation of plastics into microparticles, which can affect organisms by, for example physical injury, and impaired feeding and reproduction,25 not only by the particles themselves but also due to the release of additives such as endocrine disrupting phthalates.26 Plastics also accumulate in soils and can reach up to 0.1 weight percent of the organic matter, which can impact soil organisms.27 The sources of plastics include the use of plastic mulching, by deposition from the air and by agricultural use of biosolids.
We further recommend the integration of the following major groups of chemicals in Target 7, for which clear evidence of detrimental effects on wildlife has been compiled.
Per- and polyfluoroalkyl substances (PFASs)
PFASs comprise thousands of synthetic chemicals28 that are used in a huge variety of products, including pharmaceuticals, pesticides and a broad range of consumer products. Because of their high persistence, environmental mobility, bioaccumulation and biomagnification, many PFAS are found in all environmental compartments and biota, including humans, across the globe. PFAS exposure will continue for the forseeable future, even if their production and use would be phased out immediately.29
Currently, only a handful of PFASs are characterized for their toxicity, mainly perfluorooctanoic acid (PFOA) and perfluorooctane sulfonate (PFOS). Substantial data gaps still exist for the other members of the PFAS class, rendering a comprehensive evaluation of environmental risks impossible. Concentrations assumed to be safe for human health and/or the environment are exceedingly low, ranging from a few pg L−1 to the ng L−1 range.30 Because of this, it has been argued that current PFAS concentrations exceed planetary boundaries.31
Metal(loid)s such as arsenic, cadmium, lead and mercury
The provisional addition of mercury and other toxic metals to Target 7 was discussed in the meeting of the Open-ended Working Group on the Post-2020 Global Biodiversity Framework in June 2022. Evidence shows that the environmental impact of metals and metalloids on organisms,32–34 and impacts can be even higher for mixtures of various metals.35,36
Mercury's potential to significantly disrupt human and ecosystem health is well established for the past few decades, which has ultimately led to the Minamata Convention on Mercury.37 In brief, mercury is a highly toxic, non-degradable and mobile contaminant (some of its species can be transported in the atmosphere over very long distances) and its cycling in marine, freshwater and Arctic systems is well established.38–40 Elemental mercury is methylated by microorganisms, forming methylmercury as the most toxic form, which bioaccumulates and biomagnifies in the food web.33 Mercury concentrations in fish and wildlife across the globe often exceed human and wildlife health thresholds. All major vertebrate taxa are at risk of significant health impacts regarding physiological, behavioral, reproductive and survivorship endpoints.41–45 This raises significant concerns about its potential global effects on biodiversity.
These concerns also pertain to other toxic metal(loid)s like arsenic cadmium, and lead.
Arsenic is used as an alloying agent and in the processing of glass, pigments, textiles, paper, metal adhesives, wood preservatives and in the tanning process. It bioaccumulates in primary consumers and biomagnifies to higher-order organisms.46 Effects of arsenic include interference with the endocrine system and reproductive systems in fish, the formation of new blood vessels (angiogenesis),47 and induces oxidative stress.46–48 Due to its structural analogy to phosphate, arsenate may replace phosphorus in bones.49
Cadmium uses comprise batteries, alloys, coatings (electroplating), solar cells, plastic stabilizers, and pigments. Both in aquatic and terrestrial ecosystems, cadmium bioaccumulates and its effects on wildlife are often due to kidney damage50 and genotoxicity.51
Lead environmental levels rise in almost every country of the world because of its large use, for instance, in battery manufacturing and recycling and shot, bullets and fishing weights, as well as contamination from legacy sources such as lead-containing paint. This poses serious threats to humans and wildlife. Acute and chronic toxicity in birds results from the ingestion of lead either by primary ingestion of lead particles or secondary ingestion of contaminated food.52,53 Less information on lead poisoning is available for mammals, but a list of species at risk regarding haematological and cardiovascular effects, renal functions, and neurotoxicity has been collected.54
Endocrine-disrupting chemicals (EDCs)
EDCs interfere with the normal functioning of hormones in organisms, and thus, can cause multiple adverse effects at very low concentrations. As such, they are of high concern.55 Considerable evidence of reproductive and developmental harm linked to impairments in endocrine function in wildlife species exists, especially in environments contaminated by cocktails of EDCs. Studies show that EDCs harm the reproductive systems of vertebrate species such as polar bears56 and fish,57 and invertebrates58–60 such as snails and insects by impaired reproduction, biased sex ratios, decreased fertility, and a risk for a concomitant decline in species diversity and populations. It is therefore likely that EDCs contribute strongly to the decline in biodiversity, as shown by the example of the dramatic decline and local extinction of mollusc populations by an EDC, tributyltin, years ago in various parts of the world.61 Other examples of EDCs include organochlorines such as PCBs,62 phthalates,63 polybrominated diphenyl ethers,64 bisphenol A,65 ethinylestradiol66 and some PFASs.67,68
Emerging pollutants
It should be noted that the naming of specific groups of hazardous chemicals above for inclusion in Target 7 of the Global Biodiversity Framework does not imply that other chemicals are of no concern. As an example of chemicals used in consumer products that can readily enter the environment, sunscreens containing UV blockers have been detected in freshwater and the marine environment. The poorly biodegradable UV blocker octocrylene exerts high toxicity in chronic exposure studies on daphnids, and thus may impact population development directly and through food availability.69 A degradation product of octocrylene, i.e., benzophenone, leads to liver morbidity and homeostatic distress in mammals.70 Flame retardants are widely detected in soils and in aquatic systems where exposures can cause behavioural, neurological and physiological effects in organisms.71 Liquid crystal monomers, as another example of high-production-volume chemicals, are used in digital displays including mobile phones. They contain persistent, bioaccumulative and toxic chemicals.72,73 Numerous examples exist that pharmaceuticals and personal care products are of ecotoxicological concern in surface water,74,75 groundwater,76,77 marine biot a,78,79 rivers80,81 and lakes.82,83
While we have presented some examples, only limited ecotoxicological information is available for most of these chemicals,84 and no ecotoxicological information is available for the vast majority of the 350
000 chemicals and chemical mixtures registered for use on the market. Thus, there is currently no way to assess the environmental impact of the totality of chemicals registered for use globally. In many locations of the world, these and other chemicals occur in mixtures, which complicates the assessment of the environmental risk, both because of analytical challenges and the difficulty to consider the mixture effects on organisms.85 Also not discussed but of greater importance is that populations experience not only chemical stress, but also numerous other additional stresses such as temperature fluctuations, drought, salinization, and compaction of soils. These multiple stresses leave populations more vulnerable to toxicity from chemicals in their environment. Limited information is available on the safe levels of chemical exposures for populations experiencing multiple stressors. In this context safe levels for chemicals are environmental concentrations that are utilized in regulatory science to indicate concentrations that are accepted to define a level where no or negligible impacts are expected. These aspects on multiple stresses are not covered in the present article and may be challenging for inclusion in the current global biodiversity framework, but should be considered in future updates as relevant scientific evidence emerges.
(3) Lessons from global agreements to protect human and environmental health from chemical pollution
Need for linking biodiversity conservation to other environmental priorities
Several global multilateral environmental agreements (MEAs) have been established over the past decades to address specific issues of chemicals and waste management. For example, the Vienna Convention and the associated Montreal Protocol focus on the protection of the ozone layer from ozone-depleting substances, the Basel Convention on the control of hazardous waste and its disposal, the Stockholm Convention on persistent organic pollutants (POPs), and the Minamata Convention on the control of mercury. The Global Biodiversity Framework should establish links to these MEAs to manage the interlinked planetary crises (biodiversity loss and chemical pollution) in an integrated and holistic manner. Implementation of the MEAs and jointly developed solutions will not only provide numerous co-benefits, such as the conservation of elementary resources such as our soils, vegetation, and water, but also generate economic benefits such as clean drinking water and water for irrigation, protection of agricultural production from pollution, the health of human food supply by reducing societal costs for the cleaning of polluted media and the reduced need for treatment of diseases caused by environmental pollution.
In addition, the establishment of an intergovernmental science-policy panel on chemicals, waste and pollution prevention is now being negotiated.86 This panel should enable discussions amongst governmental representatives and policymakers, leading to global action on the sound management of chemicals and waste, and prevention of pollution. The panel can achieve this by improving knowledge, at a global scale, regarding the status of legacy and overlooked chemicals and waste problems. The knowledge should come from a strong scientific network engaging natural, social, legal and economy scientists to enable regular horizon scanning and early warning of emerging environmental issues. Such work should inform the Global Biodiversity Framework about emerging chemical threats to biodiversity when identified, individually but also jointly with the Intergovernmental Science-Policy Platform on Biodiversity and Ecosystem Services (IPBES). These bodies need to engage the participation of the wide scientific community including ecologists, ecotoxicologists, environmental chemists, sociologists, climate scientists, environmental lawyers, and economists. Neither the scientists nor research funders have fully recognized the potential of interdisciplinary cooperation. Only by interlinkage can we achieve political coherence for managing the triple planetary crises of climate change, biodiversity loss and environmental pollution.
Data inventories on chemical production, emission and use
In particular, knowledge about the distribution of chemicals in the environment needs to be improved to identify chemical pressure as a driver of biodiversity loss in specific areas and to determine local management options. Most monitoring data that exist and are compiled in inventories are from high-income countries, while data are particularly scarce for low- and medium-income countries. These latter countries have the least capacity for such investigations, and their biodiversity hotspots are at greatest threat of loss when associated with elevated environmental contaminants, e.g., the release of mercury from artisanal small-scale gold mining in the tropics.87 Existing data inventories such as the UNEP World Environmental Situation Room,88 the OECD Global Inventory of Pollutant Releases,89 and the International Environmental Specimen Bank Group,90 each focus on a fraction of pollutants and are available for only a small part of the world. For a prospective assessment of the effects of anthropogenic chemicals on biodiversity, additional open and transparent data inventories are needed that make available data on production volumes, emission inventories, use patterns and international transport. For improving the availability of such data, partnerships with companies need to be developed, including through updated chemical regulation.
(4) Steps to reduce biodiversity threats from hazardous chemicals
Reduction of emission of (hazardous) chemicals
Despite existing management of chemicals by national governments and MEAs, the planetary burden of chemical pollution is considerable (“A growing peril and potential catastrophic risk to humanity”)11 and societies currently do not invest sufficient resources to assess and manage the consequences of the ever-increasing diversity, production volumes and emission of chemicals.12 In response, we recommend to take the following three actions, as food for thought for the negotiation at the COP15: (1) a global capping of production and emissions of anthropogenic chemicals, (2) improvement of chemical management, and (3) development of less hazardous chemicals.
(1) The production volume and emissions of chemicals have been argued to be directly interlinked, resulting in steep increases of pollutant emissions following the gobal rising trends of chemical industry production.12 Consequently, the overall reduction of chemical production volume has been proposed as necessary measure to lower the total impact of the chemical burden on the environment.12 Reduction should start with chemicals of concern that have been identified by intergovernmental organisations or multilateral environmental agreements, including the Strategic Approach to International Chemicals Management (SAICM) and its successor. Additionally, the non-essential use of chemicals in many products, with a focus on consumer products, should be reduced.91 This would lower the overall production of chemicals, and in parallel, result in the design of materials suitable for recycling in a circular economy.92
In addition, the focus on reduction of hazardous chemicals should be expanded in Target 7. As detailed in section 2, next to pesticides, nutrients and plastic waste, Target 7 should also include toxic metal(loid)s, non-agricultural biocides, EDCs, and PFASs, at the very least. Furthermore, the Target 7 must be adaptable to include emerging pollutants. Reduction of hazardous chemicals can be achieved through reduction of production and specific capping of chemicals, restricting the use of chemicals or phasing out of chemicals. Because certain hazardous chemicals are essential for specific applications, such as some pharmaceuticals (e.g., anti-parasiticides) or toxic metals (e.g., cobalt, a carcinogen, used in batteries), an immediate and complete phase-out of such chemicals from essential-use applications is not possible. However, the global community should aim to use the absolute minimum amounts of hazardous chemicals following the essential-use concept.93 It is important to note that taking aggressive steps to limit the production, use and release of hazardous chemicals needs to consider impacts along the life cycle of these chemicals-from production, use, to disposal. For example, the regions with the greatest PFAS contamination are those where PFASs have been produced and/or used.94,95
In addition to taking a life-cycle approach, specific attention should be given to global consideration of chemical impacts on biodiversity, particularly because there is a dearth of information on impacts of chemicals on biodiversity from low- and middle-income countries. Some of these countries are biodiversity “hotspots.” Greater attention needs to be given to ensuring that all countries, regardless of income status and ability to produce data showing impacts, can protect their biodiversity. For example, many low- and middle-income countries are known to host metal mining and chemical production facilities which, due to financial limitations, often fail to apply state of the art technologies. For instance, modern operations in chemical production may require high temperatures or pressures, or use of innovative catalysts, all adding up to unaffordable high operating costs.96 In these regions of the world, the opportunity to bypass old, traditional chemical technologies and to foster more sustainable production is currently limited.
(2) Many governments throughout the world develop and implement strategies and concepts to protect environmental and human health from chemical pollution. These strategies can be expected to positively impact biodiversity and there exist some key concepts that should be considered as effective management tools for the regulation of hazardous chemicals. It has to be noted that the implementation of these strategies is often tailored to certain highly developed regions, and solutions will need to be adapted if they are to be expanded on a global scale including low-income regions.
The European Commission is currently working towards a zero-pollution ambition for 2050 to reduce air, water and soil pollution to levels no longer considered harmful to human and ecological health97 as a key deliverable of the European Green Deal. Part of the Green Deal is the EU's Chemicals Strategy for Sustainability (CSS),93 under which criteria for the future application of the essential-use concept across the EU chemical legislations shall be defined. This concept aims to phase out most harmful substances whenever possible and only allow their use if it is necessary for health or safety, or is critical for the functioning of society, and if no acceptable alternatives are available.93 This approach has been first defined and successfully applied through the Montreal Protocol, where it was used to phase out the use of ozone-depleting chlorofluorocarbons that did not fall under the defined concept of ‘essential use.’ Later, it has been adapted for possible application to the phase-out of PFASs.98
In addition, intermediate evaluation strategies that attempt at “chemical simplifications” and grouping of chemicals within classes have been described, which may assist in the risk assessment and management of chemicals.92,99 The grouping strategy aims to establish identification and regulation of hazardous chemical groups, which makes regulation faster and avoids regrettable substitutions of hazardous chemicals by equally problematic substitutes. Grouping is typically conducted based on the structural similarity of chemicals. With the emergence of novel, information-dense measurement methods such as ‘omics’ technologies, grouping based on the chemicals' observed modes of action (MOA) could be a valuable addition. This would enable chemicals to be classified according to their effects on biological organisms, which opens a door to linking chemical groups to impacts on biodiversity. This process can guide identification of chemicals posing the biggest threat to biodiversity, which should then be considered for regulation through the essential-use concept. The concepts and approaches for the evaluation and management of chemical pollution described here can serve as tools for international agreements aimed at the reduction of chemical pressure in relation to biodiversity loss.
(3) The development of safer chemicals that do not persist in the environment can be achieved, e.g., through the application of the benign-by-design concept, which is one of the green chemistry principles.100 The successful application of the benign-by-design concept has been demonstrated for widely used pharmaceuticals and chemicals such as β-blockers101 and ionic liquids.102 The development of less hazardous chemicals will in parallel lead to a reduction of pollution input into wastewater treatment facilities allowing them to become more effective in removing pollutants.103 Additionally, the Safe-and-Sustainable-by-Design (SSdB) approach for chemicals and materials93 constitutes a holistic strategy aimed at integrating chemical safety throughout their entire life cycle with the goal to minimize harmful effects on the environment, and can be considered as a strategy for the reduction of harmful chemicals that enter the environment. A framework for implementation of the approach that also defines criteria for sustainability and safety is currently in development by the European Commission104 under its Chemical Strategy for sustainability.
Amendment to draft Target 7
The current draft Target 7 of the Post-2020 Global Biodiversity Framework defines goals and indicators for the specifically mentioned substance groups, i.e., the reduction of pesticides and nutrients and the elimination of plastic waste. As part of our proposal to include further hazardous chemicals in Target 7 and to reduce overall chemical pressure on ecosystems, we suggest to amend the Target as follows: Overall reduction of chemical emissions.Reduce pollution of hazardous chemicals from all sources to levels that are not harmful to biodiversity, ecosystem functions or human health, including by reducing nutrients lost to the environment by at least half, pesticides by at least two thirds, eliminating the discharge of plastic waste, and reducing non-agricultural biocides, per- and polyfluoroalkyl substances (PFASs), endocrine-disrupting chemicals (EDCs), metal(loid)s such as mercury have to be reduced to the lowest amounts possible. Furthermore, regular horizon-scanning of data on emerging pollutants with the aim to integrate additional pollutants to Target 7 in the case of biodiversity risk.
Proposed indicators for effectiveness evaluation of progress towards achieving the amended Target 7
Effectiveness evaluation is crucial for the determination of the success for the improvement of environmental health. The Headline Indicators currently included in Target 7 are linked to existing indicators listed in the Global Indicator Framework for Sustainable Development Goals (SDGs).105 We propose that the indicators used in Target 7, specifically for the substances suggested to be amended, should not be evaluated exclusively through environmental monitoring data but also from assembling and maintaining inventories of chemical production and/or emissions. Example inventories include those for copper, polybrominated diphenyl ethers and PCBs.106–108 Material flow analysis can be used to track production, transboundary trade (export and import) and releases to the environment.109
In addition, we suggest considering the long-term efforts by multiple working groups of the Minamata Convention on Mercury, which have methodically identified potential environmental indicators and monitoring elements.110 The state of knowledge of published global biotic mercury concentrations is well-established and relevant monitoring programs are being identified. Therefore, this is a good example of contaminant monitoring that can be adapted and applied to the anthropogenic pollutants proposed as indicators in Target 7 as well. Suitable organisms as bioindicators for pollution should be selected based on the extensive work evaluating monitoring approaches for mercury under the Minamata Convention.111
Time to act is now!
With the aforementioned three recommendations on reduction of emission of (hazardous) chemicals, the amendment to Target 7 and indicators for effectiveness evaluation, we see opportunity to minimize hazardous effects of chemicals on the global biodiversity. We call on the negotiators and other stakeholders for a timely integration of the proposed additions to the Post-2020 Global Biodiversity Framework at the COP15 meeting in Montreal in December 2022.
Conflicts of interest
There are no conflicts to declare.
Acknowledgements
M. S. acknowledges funding by the CETOCOEN PLUS project (CZ.02.1.01/0.0/0.0/15_003/0000469), the project CETOCOEN EXCELLENCE (CZ.02.1.01/0.0/0.0/17_043/0009632), and RECETOX RI (LM2018121) financed by the Czech Ministry of Education, Youth and Sports. Z. W. gratefully acknowledges funding by the European Union under the Horizon 2020 Research and Innovation Programme (Project: ZeroPM, Grant Agreement Number 101036756).
References
- G. Sigmund, M. Ågerstrand, T. Brodin, M. L. Diamond, W. R. Erdelen and D. C. Evers,
et al., Broaden chemicals scope in biodiversity targets, Science, 2022, 376(6599), 1280 CrossRef CAS.
-
C. B. D. First, Draft of the Post-2020 Global Biodiversity Framework, UN Environment Programme, 2021, Report No. CBD/WG2020/3/3, https://www.cbd.int/doc/c/abb5/591f/2e46096d3f0330b08ce87a45/wg2020-03-03-en.pdf Search PubMed.
- R. Fuller, P. J. Landrigan, K. Balakrishnan, G. Bathan, S. Bose-O’Reilly and M. Brauer,
et al., Pollution and health: a progress update, Lancet Planet Health, 2022, 6(6), e535–e547 CrossRef PubMed.
- P. Grandjean and M. Bellanger, Calculation of the disease burden associated with environmental chemical exposures: application of toxicological information in health economic estimation, Environ Health, 2017, 16(1), 123 CrossRef PubMed.
- E. S. Bernhardt, E. J. Rosi and M. O. Gessner, Synthetic chemicals as agents of global change, Front Ecol Environ, 2017, 15(2), 84–90 CrossRef.
- K. Groh, C. vom Berg, K. Schirmer and A. Tlili, Anthropogenic Chemicals As Underestimated Drivers of Biodiversity Loss: Scientific and Societal Implications, Environ. Sci. Technol., 2022, 56(2), 707–710 CrossRef CAS.
-
CBD, A New Global Framework for Managing Nature through 2030, First detailed draft agreement debuts, 2021, http://www.cbd.int/article/draft-1-global-biodiversity-framework Search PubMed.
-
Environment UN, Global Chemicals Outlook II: from Legacies to Innovative Solutions, UNEP – UN Environment Programme, 2019, http://www.unep.org/resources/report/global-chemicals-outlook-ii-legacies-innovative-solutions Search PubMed.
- Z. Wang, G. W. Walker, D. C. G. Muir and K. Nagatani-Yoshida, Toward a Global Understanding of Chemical Pollution: A First Comprehensive Analysis of National and Regional Chemical Inventories, Environ. Sci. Technol., 2020, 54(5), 2575–2584 CrossRef CAS.
- M. Saaristo, T. Brodin, S. Balshine, M. G. Bertram, B. W. Brooks and S. M. Ehlman,
et al., Direct and indirect effects of chemical contaminants on the behaviour, ecology and evolution of wildlife, Proc R Soc B Biol Sci, 2018, 285(1885), 20181297 CrossRef PubMed.
- R. Naidu, B. Biswas, I. R. Willett, J. Cribb, B. Kumar Singh and C. Paul Nathanail,
et al., Chemical pollution: A growing peril and potential catastrophic risk to humanity, Environ. Int., 2021, 156, 106616 CrossRef CAS PubMed.
- L. Persson, B. M. Carney Almroth, C. D. Collins, S. Cornell, C. A. de Wit and M. L. Diamond,
et al., Outside the Safe Operating Space of the Planetary Boundary for Novel Entities, Environ. Sci. Technol., 2022, 56(3), 1510–1521 CrossRef PubMed.
- A. Schaeffer, W. Amelung, H. Hollert, M. Kaestner, E. Kandeler and J. Kruse,
et al., The impact of chemical pollution on the resilience of soils under multiple stresses: A conceptual framework for future research, Sci. Total Environ., 2016, 568, 1076–1085 CrossRef CAS.
- M. C. Rillig, M. Ryo, A. Lehmann, C. A. Aguilar-Trigueros, S. Buchert and A. Wulf,
et al., The role of multiple global change factors in driving soil functions and microbial biodiversity, Science, 2019, 366(6467), 886–890 CrossRef CAS PubMed.
-
Secretariat of the Convention on Biological Diversity, Long-Term Strategic Directions to the 2050 Vision for Biodiversity, Approaches to Living in Harmony with Nature and preparation for the post-2020 global biodiversity framework, 2018, https://www.cbd.int/doc/c/0b54/1750/607267ea9109b52b750314a0/cop-14-09-en.pdf Search PubMed.
- M. Hahn, A. Schotthöfer, J. Schmitz, L. A. Franke and C. A. Brühl, The effects of agrochemicals on Lepidoptera, with a focus on moths, and their pollination service in field margin habitats, Agric., Ecosyst. Environ., 2015, 207, 153–162 CrossRef CAS.
- N. T. Halstead, T. A. McMahon, S. A. Johnson, T. R. Raffel, J. M. Romansic and P. W. Crumrine,
et al., Community ecology
theory predicts the effects of agrochemical mixtures on aquatic biodiversity and ecosystem properties, Ecol. Lett., 2014, 17(8), 932–941 CrossRef PubMed.
- G. Vogel, Where have all the insects gone?, Science, 2017, 356(6338), 576–579 CrossRef CAS PubMed.
- S. Kresmann, A. H. R. Arokia, C. Koch and B. Sures, Ecotoxicological potential of the biocides terbutryn, octhilinone and methylisothiazolinone: Underestimated risk from biocidal pathways?, Sci. Total Environ., 2018, 625, 900–908 CrossRef CAS PubMed.
- S. Wieck, O. Olsson and K. Kümmerer, Possible underestimations of risks for the environment due to unregulated emissions of biocides from households to wastewater, Environ. Int., 2016, 94, 695–705 CrossRef CAS.
- C. A. Amorim and N. Moura A do, Ecological impacts of freshwater algal blooms on water quality, plankton biodiversity, structure, and ecosystem functioning, Sci. Total Environ., 2021, 758, 143605 CrossRef CAS PubMed.
- V. G. Christensen, R. P. Maki, E. A. Stelzer, J. E. Norland and E. Khan, Phytoplankton community and algal toxicity at a recurring bloom in Sullivan Bay, Kabetogama Lake, Minnesota, USA, Sci. Rep., 2019, 9(1), 16129 CrossRef.
- S. Kühn and J. A. van Franeker, Quantitative overview of marine debris ingested by marine megafauna, Mar. Pollut. Bull., 2020, 151, 110858 CrossRef.
- S. C. Gall and R. C. Thompson, The impact of debris on marine life, Mar. Pollut. Bull., 2015, 92(1), 170–179 CrossRef CAS PubMed.
-
N. Prinz and Š. Korez, Understanding How Microplastics Affect Marine Biota on the Cellular Level Is Important for Assessing Ecosystem Function: A Review, in YOUMARES 9 – the Oceans: Our Research, Our Future: Proceedings of the 2018 Conference for YOUng MArine RESearcher in Oldenburg, Germany, ed., Jungblut S., Liebich V. and Bode-Dalby M., Springer International Publishing, Cham, 2020, pp. 101–20, DOI:10.1007/978-3-030-20389-4_6.
- C. Cunha, J. Paulo, M. Faria, M. Kaufmann and N. Cordeiro, Ecotoxicological and biochemical effects of environmental concentrations of the plastic-bond pollutant dibutyl phthalate on Scenedesmus sp, Aquat. Toxicol., 2019, 215, 105281 CrossRef CAS.
- D. Kim, R. Cui, J. Moon, J. I. Kwak and Y. J. An, Soil ecotoxicity study of DEHP with respect to multiple soil species, Chemosphere, 2019, 216, 387–395 CrossRef CAS.
- Z. Wang, A. M. Buser, I. T. Cousins, S. Demattio, W. Drost and O. Johansson,
et al., A New OECD Definition for Per- and Polyfluoroalkyl Substances, Environ. Sci. Technol., 2021, 55(23), 15575–15578 CrossRef CAS.
- M. G. Evich, M. J. B. Davis, J. P. McCord, B. Acrey, J. A. Awkerman and D. R. U. Knappe,
et al., Per- and polyfluoroalkyl substances in the environment, Science, 2022, 375(6580), eabg9065 CrossRef CAS PubMed.
-
US Environmental Protection Agency, Lifetime Drinking Water Health Advisories for Four Perfluoroalkyl Substances, Environmental Protection Agency, 2022, Report No. 2022–13158 Search PubMed.
- I. T. Cousins, J. H. Johansson, M. E. Salter, B. Sha and M. Scheringer, Outside the Safe Operating Space of a New Planetary Boundary for Per- and Polyfluoroalkyl Substances (PFAS), Environ. Sci. Technol., 2022, 56(16), 11172–11179 CrossRef CAS PubMed.
- P. de Almeida Rodrigues, R. G. Ferrari, L. S. Kato, R. A. Hauser-Davis and C. A. Conte-Junior, A Systematic Review on Metal Dynamics and Marine Toxicity Risk Assessment Using Crustaceans as Bioindicators, Biol. Trace Elem. Res., 2022, 200(2), 881–903 CrossRef CAS PubMed.
- H. Ali, E. Khan and I. Ilahi, Environmental Chemistry and Ecotoxicology of Hazardous Heavy Metals: Environmental Persistence, Toxicity, and Bioaccumulation, J. Chem., 2019, 2019, e6730305 Search PubMed.
- M. Mayer-Pinto, A. J. Underwood, T. Tolhurst and R. A. Coleman, Effects of metals on aquatic assemblages: What do we really know?, J. Exp. Mar. Biol. Ecol., 2010, 391(1), 1–9 CrossRef CAS.
- E. L. Enserink, J. L. Maas-Diepeveen and C. J. Van Leeuwen, Combined effects of metals; an ecotoxicological evaluation, Water Res., 1991, 25(6), 679–687 CrossRef CAS.
-
M. A. A. Wijayawardena, M. Megharaj and R. Naidu, Chapter Three – Exposure, Toxicity, Health Impacts, and Bioavailability of Heavy Metal Mixtures, in Advances in Agronomy, ed., Sparks D. L., Academic Press, 2016, vol. 138, pp. 175–234, https://www.sciencedirect.com/science/article/pii/S0065211316300402 Search PubMed.
- D. C. Evers, S. E. Keane, N. Basu and D. Buck, Evaluating the effectiveness of the Minamata Convention on Mercury: Principles and recommendations for next steps, Sci. Total Environ., 2016, 569–570, 888–903 CrossRef CAS PubMed.
- K. L. Bowman, C. H. Lamborg and A. M. Agather, A global perspective on mercury cycling in the ocean, Sci. Total Environ., 2020, 710, 136166 CrossRef CAS PubMed.
- B. A. Branfireun, C. Cosio, A. J. Poulain, G. Riise and A. G. Bravo, Mercury cycling in freshwater systems - An updated conceptual model, Sci. Total Environ., 2020, 745, 140906 CrossRef CAS PubMed.
- A. Dastoor, H. Angot, J. Bieser, J. H. Christensen, T. A. Douglas and L. E. Heimbürger-Boavida,
et al., Arctic mercury cycling, Nat. Rev. Earth Environ., 2022, 3(4), 270–286 CrossRef CAS.
-
D. C. Evers, The Effects of Methylmercury on Wildlife: A Comprehensive Review and Approach for Interpretation in Encyclopedia of the Anthropocene, Elsevier, 2017, p. 2290 Search PubMed.
- R. Dietz, R. J. Letcher, J. Aars, M. Andersen, A. Boltunov and E. W. Born,
et al., A risk assessment review of mercury exposure in Arctic marine and terrestrial mammals, Sci. Total Environ., 2022, 829, 154445 CrossRef CAS PubMed.
- G. López-Berenguer, J. Peñalver and E. Martínez-López, A critical review about neurotoxic effects in marine mammals of mercury and other trace elements, Chemosphere, 2020, 246, 125688 CrossRef PubMed.
- K. E. Markham and F. Sangermano, Evaluating Wildlife Vulnerability to Mercury Pollution From Artisanal and Small-Scale Gold Mining in Madre de Dios, Peru, Trop. Conserv. Sci., 2018, 11, 1940082918794320 Search PubMed.
-
M. C. Whitney and D. A. Cristol, Impacts of Sublethal Mercury Exposure on Birds: A Detailed Review, in Reviews of Environmental Contamination and Toxicology, ed. de Voogt P., Springer International Publishing, Cham, 2018, vol. 244, pp. 113–63, DOI:10.1007/398_2017_4.
- B. Kumari, V. Kumar, A. K. Sinha, J. Ahsan, A. K. Ghosh and H. Wang,
et al., Toxicology of arsenic in fish and aquatic systems, Environ. Chem. Lett., 2017, 15(1), 43–64 CrossRef CAS.
- L. Z. Liu, Y. Jiang, R. L. Carpenter, Y. Jing, S. C. Peiper and B. H. Jiang, Role and Mechanism of Arsenic in Regulating Angiogenesis, PLoS One, 2011, 6(6), e20858 CrossRef CAS PubMed.
- J. C. Davey, J. E. Bodwell, J. A. Gosse and J. W. Hamilton, Arsenic as an Endocrine Disruptor: Effects of Arsenic on Estrogen Receptor–Mediated Gene Expression In Vivo and in Cell Culture, Toxicol. Sci., 2007, 98(1), 75–86 CrossRef CAS PubMed.
- C. R. Lage, A. Nayak and C. H. Kim, Arsenic ecotoxicology and innate immunity, Integr. Comp. Biol., 2006, 46(6), 1040–1054 CrossRef CAS PubMed.
- V. Hiatt and J. E. Huff, Environmental Impact of Cadmium: an Overview, Int. J. Env. Stud. U K, 1975, 7 CAS , Available from: https://www.osti.gov/etdeweb/biblio/7352927.
- M. D. Pavlaki, M. J. Araújo, D. N. Cardoso, A. R. R. Silva, A. Cruz and S. Mendo,
et al., Ecotoxicity and genotoxicity of cadmium in different marine trophic levels, Environ. Pollut., 1987, 215, 203–212 CrossRef.
- T. Grade, P. Campbell, T. Cooley, M. Kneeland, E. Leslie and B. MacDonald,
et al., Lead poisoning from ingestion of fishing gear: A review, Ambio, 2019, 48(9), 1023–1038 CrossRef CAS PubMed.
- J. C. Franson, S. P. Hansen, T. E. Creekmore, C. J. Brand, D. C. Evers and A. E. Duerr,
et al., Lead Fishing Weights and Other Fishing Tackle in Selected Waterbirds, Waterbirds, 2003, 26(3), 345–352 CrossRef.
-
E. C. H. A. Annex, XV Restriction Report Lead, Helsinki, 2021, Report No. 2.0, https://echa.europa.eu/documents/10162/da9bf395-e6c3-b48e-396f-afc8dcef0b21 Search PubMed.
- V. L. Marlatt, S. Bayen, D. Castaneda-Cortès, G. Delbès, P. Grigorova and V. S. Langlois,
et al., Impacts of endocrine disrupting chemicals on reproduction in wildlife and humans, Environ. Res., 2022, 208, 112584 CrossRef CAS PubMed.
- K. E. Pedersen, R. J. Letcher, C. Sonne, R. Dietz and B. Styrishave, Per- and polyfluoroalkyl substances (PFASs) – New endocrine disruptors in polar bears (Ursus maritimus)?, Environ. Int., 2016, 96, 180–189 CrossRef CAS.
- K. A. Kidd, P. J. Blanchfield, K. H. Mills, V. P. Palace, R. E. Evans and J. M. Lazorchak,
et al., Collapse of a fish population after exposure to a synthetic estrogen, Proc. Natl. Acad. Sci. U. S. A, 2007, 104(21), 8897–8901 CrossRef CAS PubMed.
- P. Matthiessen, J. R. Wheeler and L. Weltje, A review of the evidence for endocrine disrupting effects of current-use chemicals on wildlife populations, Crit. Rev. Toxicol., 2018, 48(3), 195–216 CrossRef CAS PubMed.
- T. F. Grilo and R. Rosa, Intersexuality in aquatic invertebrates: Prevalence and causes, Sci. Total Environ., 2017, 592, 714–728 CrossRef CAS PubMed.
- V. Cuvillier-Hot and A. Lenoir, Invertebrates facing environmental contamination by endocrine disruptors: Novel evidences and recent insights, Mol. Cell. Endocrinol., 2020, 504, 110712 CrossRef CAS PubMed.
-
Å. Bergman, J. J. Heindel, S. Jobling, K. Kidd, T. R. Zoeller and World Health Organization, State of the Science of Endocrine Disrupting Chemicals 2012, World Health Organization, 2013 Search PubMed.
- L. K. Akinola, A. Uzairu, G. A. Shallangwa and S. E. Abechi, A computational insight into endocrine disruption by polychlorinated biphenyls via non-covalent interactions with human nuclear receptors, Ecotoxicol. Environ. Saf., 2021, 214, 112086 CrossRef CAS PubMed.
- H. Hlisníková, I. Petrovičová, B. Kolena, M. Šidlovská and A. Sirotkin, Effects and Mechanisms of Phthalates' Action on Reproductive Processes and Reproductive Health: A Literature Review, Int. J. Environ. Res. Public Health, 2020, 17(18), 6811 CrossRef PubMed.
- F. Gorini, G. Iervasi, A. Coi, L. Pitto and F. Bianchi, The Role of Polybrominated Diphenyl Ethers in Thyroid Carcinogenesis: Is It a Weak Hypothesis or a Hidden Reality? From Facts to New Perspectives, Int. J. Environ. Res. Public Health, 2018, 15(9), 1834 CrossRef PubMed.
- B. S. Rubin, Bisphenol A: An endocrine disruptor with widespread exposure and multiple effects, J. Steroid Biochem. Mol. Biol., 2011, 127(1), 27–34 CrossRef CAS PubMed.
- M. Saaristo, C. P. Johnstone, K. Xu, M. Allinson and B. B. M. Wong, The endocrine disruptor, 17α-ethinyl estradiol, alters male mate choice in a freshwater fish, Aquat. Toxicol., 2019, 208, 118–125 CrossRef CAS PubMed.
- S. Kar, M. S. Sepúlveda, K. Roy and J. Leszczynski, Endocrine-disrupting activity of per- and polyfluoroalkyl substances: Exploring combined approaches of ligand and structure based modeling, Chemosphere, 2017, 184, 514–523 CrossRef CAS PubMed.
- S. S. White, S. E. Fenton and E. P. Hines, Endocrine disrupting properties of perfluorooctanoic acid, J. Steroid Biochem. Mol. Biol., 2011, 127(1), 16–26 CrossRef CAS PubMed.
- K. Duis, T. Junker and A. Coors, Review of the environmental fate and effects of two UV filter substances used in cosmetic products, Sci. Total Environ., 2022, 808, 151931 CrossRef CAS PubMed.
- C. A. Downs, J. C. DiNardo, D. Stien, A. M. S. Rodrigues and P. Lebaron, Benzophenone Accumulates over Time from the Degradation of Octocrylene in Commercial Sunscreen Products, Chem. Res. Toxicol., 2021, 34(4), 1046–1054 Search PubMed.
-
J. M. Ortega-Olvera, A. Mejía-García, H. Islas-Flores, M. D. Hernández-Navarro and L. M. Gómez-Oliván, Chapter Three - Ecotoxicity of emerging halogenated flame retardants, in Comprehensive Analytical Chemistry, ed., Oh J. E., Emerging Halogenated Flame Retardants in the Environment, Elsevier, 2020, vol. 88, pp. 71–105, https://www.sciencedirect.com/science/article/pii/S0166526X19301084 Search PubMed.
- J. Li, G. Su, R. J. Letcher, W. Xu, M. Yang and Y. Zhang, Liquid Crystal Monomers (LCMs): A New Generation of Persistent Bioaccumulative and Toxic (PBT) Compounds?, Environ. Sci. Technol., 2018, 52(9), 5005–5006 CrossRef CAS PubMed.
- H. Su, S. Shi, M. Zhu, D. Crump, R. J. Letcher and J. P. Giesy,
et al., Persistent, bioaccumulative, and toxic properties of liquid crystal monomers and their detection in indoor residential dust, Proc. Natl. Acad. Sci. U. S. A, 2019, 116(52), 26450–26458 CrossRef CAS PubMed.
- A. Lindström, I. J. Buerge, T. Poiger, P. A. Bergqvist, M. D. Müller and H. R. Buser, Occurrence and Environmental Behavior of the Bactericide Triclosan and Its Methyl Derivative in Surface Waters and in Wastewater, Environ. Sci. Technol., 2002, 36(11), 2322–2329 CrossRef PubMed.
- Z. Wang, X. H. Zhang, Y. Huang and H. Wang, Comprehensive evaluation of pharmaceuticals and personal care products (PPCPs) in typical highly urbanized regions across China, Environ. Pollut., 2015, 204, 223–232 CrossRef CAS PubMed.
- R. Loos, G. Locoro, S. Comero, S. Contini, D. Schwesig and F. Werres,
et al., Pan-European survey on the occurrence of selected polar organic persistent pollutants in ground water, Water Res., 2010, 44(14), 4115–4126 CrossRef CAS PubMed.
- X. Peng, W. Ou, C. Wang, Z. Wang, Q. Huang and J. Jin,
et al., Occurrence and ecological potential of pharmaceuticals and personal care products in groundwater and reservoirs in the vicinity of municipal landfills in China, Sci. Total Environ., 2014, 490, 889–898 CrossRef CAS.
- S. Montesdeoca-Esponda, L. Checchini, M. Del Bubba, Z. Sosa-Ferrera and J. J. Santana-Rodriguez, Analytical approaches for the determination of personal care products and evaluation of their occurrence in marine organisms, Sci. Total Environ., 2018, 633, 405–425 CrossRef CAS PubMed.
- F. S. Cortez, C. D. Seabra Pereira, A. R. Santos, A. Cesar, R. B. Choueri and G. de A. Martini,
et al., Biological effects of environmentally relevant concentrations of the pharmaceutical Triclosan in the marine mussel Perna perna (Linnaeus, 1758), Environ. Pollut., 2012, 168, 145–150 CrossRef CAS PubMed.
- M. Ricart, H. Guasch, M. Alberch, D. Barceló, C. Bonnineau and A. Geiszinger,
et al., Triclosan persistence through wastewater treatment plants and its potential toxic effects on river biofilms, Aquat. Toxicol., 2010, 100(4), 346–353 CrossRef CAS PubMed.
- J. L. Zhao, Q. Q. Zhang, F. Chen, L. Wang, G. G. Ying and Y. S. Liu,
et al., Evaluation of triclosan and triclocarban at river basin scale using monitoring and modeling tools: Implications for controlling of urban domestic sewage discharge, Water Res., 2013, 47(1), 395–405 CrossRef CAS PubMed.
- M. E. Balmer, H. R. Buser, M. D. Müller and T. Poiger, Occurrence of Some Organic UV Filters in Wastewater, in Surface Waters, and in Fish from Swiss Lakes, Environ. Sci. Technol., 2005, 39(4), 953–962 CrossRef CAS PubMed.
- B. D. Blair, J. P. Crago, C. J. Hedman and R. D. Klaper, Pharmaceuticals and personal care products found in the Great Lakes above concentrations of environmental concern, Chemosphere, 2013, 93(9), 2116–2123 CrossRef CAS PubMed.
- E. Kristiansson, J. Coria, L. Gunnarsson and M. Gustavsson, Does the scientific knowledge reflect the chemical diversity of environmental pollution? – A twenty-year perspective, Environ. Sci. Policy, 2021, 126, 90–98 CrossRef CAS.
- R. Altenburger, M. Scholze, W. Busch, B. I. Escher, G. Jakobs and M. Krauss,
et al., Mixture effects in samples of multiple contaminants – An inter-laboratory study with manifold bioassays, Environ. Int., 2018, 114, 95–106 CrossRef CAS PubMed.
- Z. Wang, R. Altenburger, T. Backhaus, A. Covaci, M. L. Diamond and J. O. Grimalt,
et al., We need a global science-policy body on chemicals and waste, Science, 2021, 371(6531), 774–776 CrossRef CAS PubMed.
- J. R. Gerson, N. Szponar, A. A. Zambrano, B. Bergquist, E. Broadbent and C. T. Driscoll,
et al., Amazon forests capture high levels of atmospheric mercury pollution from artisanal gold mining, Nat. Commun., 2022, 13(1), 559 CrossRef CAS PubMed.
-
UNEP, https://wesr.unepgrid.ch/?project=MX-YBJ-YYF-08R-UUR-QW6%26language=en.
-
OECD, https://www.oecd.org/env/ehs/pollutant-release-transfer-register/.
-
UBA, https://www.umweltbundesamt.de/en/topics/chemicals/iesb-specimen-banks/media-center#introducting-to-the-international-environmental-specimen-bank-group.
- K. Kümmerer, J. H. Clark and V. G. Zuin, Rethinking chemistry for a circular economy, Science, 2020, 367(6476), 369–370 CrossRef PubMed.
- K. Fenner and M. Scheringer, The Need for Chemical Simplification As a Logical Consequence of Ever-Increasing Chemical Pollution, Environ. Sci. Technol., 2021, 55(21), 14470–14472 CrossRef CAS PubMed.
-
European Commission, Chemicals Strategy for Sustainability towards a Toxic-free Environment, Brussels, 2020 Search PubMed.
- H. Chen, Y. Yao, Z. Zhao, Y. Wang, Q. Wang and C. Ren,
et al., Multimedia Distribution and Transfer of Per- and Polyfluoroalkyl Substances (PFASs) Surrounding Two Fluorochemical Manufacturing Facilities in Fuxin, China, Environ. Sci. Technol., 2018, 52(15), 8263–8271 CrossRef CAS PubMed.
- W. A. Gebbink and S. P. J. van Leeuwen, Environmental contamination and human exposure to PFASs near a fluorochemical production plant: Review of historic and current PFOA and GenX contamination in the Netherlands, Environ. Int., 2020, 137, 105583 CrossRef CAS PubMed.
- J. Tickner, K. Geiser and S. Baima, Transitioning the Chemical Industry: The Case for Addressing the Climate, Toxics, and Plastics Crises, Environ. Sci. Policy Sustain. Dev., 2021, 63(6), 4–15 CrossRef.
-
European Commission, Pathway to a Healthy Planet for All EU Action Plan: “Towards Zero Pollution for Air, Water and Soil”, 2021, Report No. COM/2021/400 final Search PubMed.
- I. T. Cousins, G. Goldenman, D. Herzke, R. Lohmann, M. Miller and C. A. Ng,
et al., The concept of essential use for determining when uses of PFASs can be phased out, Environ. Sci.: Processes Impacts, 2019, 21(11), 1803–1815 RSC.
- Z. Wang and A. Praetorius, Integrating a Chemicals Perspective into the Global Plastic Treaty, Environ. Sci. Technol. Lett., 2022 DOI:10.1021/acs.estlett.2c00763.
- J. M. Lewer, Z. R. Stickelman, J. H. Huang, J. F. Peloquin and J. Kostal, Structure-to-process design framework for developing safer pesticides, Sci. Adv., 2022, 8(13), eabn2058 CrossRef CAS PubMed.
- T. Rastogi, C. Leder and K. Kümmerer, Re-Designing of Existing Pharmaceuticals for Environmental Biodegradability: A Tiered Approach with β-Blocker Propranolol as an Example, Environ. Sci. Technol., 2015, 49(19), 11756–11763 CrossRef CAS PubMed.
- A. Haiß, A. Jordan, J. Westphal, E. Logunova, N. Gathergood and K. Kümmerer, On the way to greener ionic liquids: identification of a fully mineralizable phenylalanine-based ionic liquid, Green Chem., 2016, 18(16), 4361–4373 RSC.
- K. Kümmerer, D. D. Dionysiou, O. Olsson and D. Fatta-Kassinos, A path to clean water, Science, 2018, 361(6399), 222–224 CrossRef.
-
C. Caldeira, R. Farcal, C. Moretti, L. Mancini, K. Rasmussen and H. Rauscher, et al., Safe and Sustainable by Design Chemicals and Materials - Review of Safety and
Sustainability Dimensions, Aspects, Methods, Indicators, and Tools, Publications Office of the European Union, Luxembourg, 2022, Report No. JRC127109, https://publications.jrc.ec.europa.eu/repository/handle/JRC127109 Search PubMed.
-
UN Statistics Division, Global Indicator Framework for the Sustainable Development Goals and Targets of the 2030 Agenda for Sustainable Development, UN, https://unstats.un.org/sdgs/indicators/Global%20Indicator%20Framework%20after%202022%20refinement_Eng.pdf, 2017 Search PubMed.
- L. Melymuk, J. Blumenthal, O. Sáňka, A. Shu-Yin, V. Singla and K. Šebková,
et al., Persistent Problem: Global Challenges to Managing PCBs, Environ. Sci. Technol., 2022, 56(12), 9029–9040 CrossRef CAS PubMed.
- G. Abbasi, L. Li and K. Breivik, Global Historical Stocks and Emissions of PBDEs, Environ. Sci. Technol., 2019, 53(11), 6330–6340 CrossRef CAS PubMed.
- S. Glöser, M. Soulier and L. A. Tercero Espinoza, Dynamic Analysis of Global Copper Flows. Global Stocks, Postconsumer Material Flows, Recycling Indicators, and Uncertainty Evaluation, Environ. Sci. Technol., 2013, 47(12), 6564–6572 CrossRef PubMed.
-
OECD, Measuring Material Flows and Resource Productivity, 2008, vol. Ihttps://www.oecd.org/environment/indicators-modelling-outlooks/MFA-Guide.pdf Search PubMed.
-
Minamata Convention on Mercury, Guidance on Monitoring of Mercury and Mercury Compounds to Support Evaluation of the Effectiveness of the Minamata Convention, UNEP, 2021, https://www.mercuryconvention.org/sites/default/files/documents/information_document/4_INF12_MonitoringGuidance.English.pdf Search PubMed.
-
D. C. Evers and E. Sunderland, Technical Information Report on Mercury Monitoring in Biota: Proposed Components towards a Strategic Long-Term Plan for Monitoring Mercury in Fish and Wildlife Globally, UN Environment Programme, Chemicals and Health Branch, Geneva, Switzerland, 2019 Search PubMed.
|
This journal is © The Royal Society of Chemistry 2023 |