Visible light-activated photocatalytic activity of TiO2–crystal violet based superhydrophobic paint against VOCs in indoor air†
Received
9th June 2022
, Accepted 27th September 2022
First published on 2nd November 2022
Abstract
With industrial growth, air pollution has also increased. Air pollution is accountable for several diseases and numerous deaths. Volatile organic compounds (VOCs) are one of the most common classes of air pollutants and are mostly caused by anthropogenic activities. These VOCs have adverse effects on human health as well as on the environment. Photocatalytic oxidation is one of the most common methods available to get rid of VOCs. Photocatalytic oxidation (PCO) is usually performed under UV light, but various photocatalysts, which can work under the visible region of light (white light), have also been reported lately. Recently, PFOTES–CV-based visible light activated-superhydrophobic TiO2 paints have been reported to have bactericidal activity according to the literature. In this study, we have used these TiO2-based paints to study their photocatalytic efficiency against VOCs. The effects of multiple parameters including the input concentration of VOCs (2–5 ppm), amount of PFOTES (1–5%), light power source (8, 24 W), and humidity (60–70%) have been studied. Substantial photocatalytic efficiency was observed against toluene, p-xylene, and acetaldehyde. ∼92% against toluene, ∼99% against p-xylene, and considerable removal efficiency against acetaldehyde were found with the paint made with 3% PFOTES. Characterization of the photocatalysts was carried out by XPS, FTIR, and DRS. The PCO reactor under visible light was used for the photocatalytic decomposition reaction and the gas analysis was performed by GC/MS.
Environmental significance
VOCs are one of the major air pollutant groups. They are harmful for human health as well as our environment and exposure to them is inevitable. Due to these reasons, VOC removal is the need of time. This article studied the photocatalytic oxidation performance of superhydrophobic-visible light-activated PFOTES–CV-based TiO2 paints against VOCs. These photocatalytic TiO2 paints are reported to have bactericidal activity under visible light in the literature. We have investigated their photocatalytic performance against toluene, p-xylene, and acetaldehyde under visible light. Various parameters such as the input concentration of toluene, amount of PFOTES, light power, and humidity were also examined. These paints successfully decomposed VOCs such as toluene, p-xylene, and acetaldehyde. These paints have high potential for commercialization.
|
Introduction
Air pollution has increased greatly since the start of the industrial age. Any physical, chemical, or biological contamination which affects the atmospheric properties is considered air pollution.1 According to WHO data, 99% of the Earth's population is exposed to the pollutants in air which are higher than the WHO guideline limit. Air pollution is responsible for numerous diseases as well as death. Ambient air pollution is accountable for almost 4.2 million deaths yearly. Additionally, 3.8 million deaths are caused by household air pollution. There are numerous pollutants present in air that come from multiple sources.
Volatile organic compounds (VOCs) are one of the major pollutant groups. The indoor concentration of VOCs can be 10 times higher than that found outdoors.2 VOCs can easily pollute the environment due to their wide use in everyday life and their ability to evaporate at room temperature.3 Exposure to VOCs is inevitable. Most of the VOCs present in air come from anthropogenic activities. The burning of fuel and the use of paints, glues, cleaning agents, aerosol sprays, etc. are all some of the most common ways for VOCs to spread as indoor air pollutants. Modern modes of transportation and chemical industries such as gas and oil fields are among the largest sources of VOCs present in ambient air.4 The effect of VOCs on human health varies substantially.2 However, some VOCs can be found as carcinogens, while others can have little to unknown effects. Headaches, dizziness, nausea, skin, and eye irritation are reported to be some of the short-term effects of VOCs, whereas organ damage (e.g. liver, nervous system, kidney), asthma, cancer, leukemia, and other deadly diseases are known as long-term effects of VOCs on human health.2,5 The presence of VOCs in the atmosphere is also dangerous to the environment. VOCs can give rise to global warming as most of them absorb the radiation in the infrared region. VOCs are also responsible for indirect global warming by the generation of tropospheric oxidants such as ozone, degradation of ozone in the stratosphere, climate change, etc.6,7 The sources and effects of VOCs studied in this article are listed in Table 1.
Table 1 Sources and effects of the studied VOCs
Name |
Boiling point (BP) °C |
Source |
Effect |
Toluene |
110.6 |
Fuel, paints |
Headache, dizziness, hearing/vision loss, problems in pregnancy |
Xylene |
138.4 |
Fuel, paints |
Dizziness, stomach problems, loss of memory, bone marrow damage |
Acetaldehyde |
20.2 |
Consumer products, building materials, automobile exhausts |
Skin/eye irritation, nausea, headache, mucous membrane, carcinogen |
Numerous methods for the removal of VOCs have been reported in previous literature.33 Photo-thermal catalytic combustion,8 plasma catalytic process,9 and catalytic oxidation10,11 are a few of the recently studied methods for the decomposition of VOCs. Several non-destructive methods have also been used for VOC removal such as absorption, adsorption,34,36 and condensation.4 Photocatalysis is one of the environmentally friendly techniques for the decomposition of various pollutants, including VOCs. Carbon dioxide and water are formed as a result of the photocatalytic decomposition of VOCs. Until now, vast research has been done on this topic, resulting in various publications including multiple review articles. Titanium oxide (TiO2) is a widely used catalyst for photocatalytic degradation of VOCs.12 The reaction mechanism of the photocatalyst has also been widely studied and reported in numerous studies.12–14 TiO2 can produce highly reactive radicals such as ˙OH, ˙O2− and hydrogen peroxide (H2O2) but only under ultraviolet (UV) light due to the high bandgap.15 The requirement of UV light hinders its wider and practical application for many reasons. Studies have mentioned that only 5% of solar light consists of light within the UV region in comparison to 45% visible light and 50% within the near-infrared region, which makes this process less efficient.14,16,17 Another drawback that was stated is the formation of various by-products due to very high-energy photons. Thus, it is important to decrease the bandgap in the TiO2 photocatalyst so it can be used under visible light. Several modifications have been reported in this regard. Doping of elements (metals and non-metals), inorganic acid treatment, constructing heterojunctions (with noble metals or other semiconductors), organic dye sensitization, generating oxygen vacancies in titanium dioxide (non-stoichiometric TiO2), and metal ion implantation are among the widely studied methods to increase the photocatalytic activity of TiO2 under the visible region of light.14,18
As mentioned previously, dye sensitization is one of the extensively studied methods in the field of visible light-activated photocatalysis. In this technique, an organic dye is adsorbed on the surface of TiO2 by weak van der Waals attraction. Subsequently, the electrons from the dye jump to the conduction band of the photocatalyst due to excitation and generate radicals under visible light.14,18,19 Additionally, silane coupling reagents can be used to protect the dye from desorption or photobleaching.20 Recently, TiO2-based white light-activated, superhydrophobic surfaces have been introduced by the addition of a water repellent silane group (1H,1H,2H,2H-perfluorooctyltriethoxysilane (PFOTES)) and organic dyes (crystal violet (CV) and toluidine blue O (BTO)) to TiO2 nanoparticles.21 Superhydrophobic materials are materials that have a water droplet contact angle higher than 150°.22 The prepared photocatalysts have shown remarkable bactericidal activity against Staphylococcus aureus (S. aureus) and Escherichia coli (E. coli). The study also hinted at the multiple applications of these paints and suggested that these paints can be applied on various surfaces such as paper, polymers, and glass. The application of these photocatalytic paints has also been reported. It was mentioned that these visible light (white light) activated materials can be used as air filters to remove various bioaerosols with ∼99.98% inactivation rate and ∼99.9% filtration efficiency,23 which is higher than conventional filter-based copper nanoparticles or copper–organic framework.35,37 Easy preparation, and simple and fast surface treatment are among the most notable advantages of these paints. Previous studies21,23 have also extensively studied the characterization of PFOTES–CV–TiO2 paints.
Therefore, our study focuses on another application of TiO2-based superhydrophobic white light-activated paints. We have studied the conversion of volatile organic compounds into environmentally friendly by-products with the use of TiO2-based paints. Toluene, p-xylene, and acetaldehyde were used as VOCs to study the photocatalytic activity of the paints. These VOCs are among the most common pollutants in indoor and outdoor air. Their decomposition study will help future researchers to understand the removal of other common VOCs of the same groups such as hydrocarbons and aldehydes. The effect of the input concentration, the amount of PFOTES, and the light power are included in the studied parameters. Differential reflectance spectroscopy (DRS), Fourier transform infrared spectroscopy (FTIR) and X-ray photoelectron spectroscopy (XPS) were used for the characterization of the photocatalysts. In the future, this study will be helpful in the development of self-cleaning paints with photocatalytic activity against chemical aerosols such as VOCs. The photocatalytic efficiency was studied by using a PCO reactor and GC/MS.
Materials and methods
Raw materials
The raw materials for the photocatalysts such as TiO2 (Degussa P25, anatase
:
rutile = 75
:
25), 1H,1H,2H,2H-perfluorooctyltriethoxysilane (PFOTES), and crystal violet (CV) were purchased from Sigma-Aldrich (USA). Toluene, p-xylene, and acetaldehyde were used as volatile organic compounds (VOCs) and were purchased from RIGAS Co. Ltd. (Korea) in the form of 50 ppm aerosol in nitrogen. Ethanol was used as the solvent for the paint preparation and was purchased from Daejung (Korea). White lights of power 8 W and 24 W were bought from Osram (Italy).
Preparation of the photocatalysts
1–5% PFOTES solutions were prepared by adding 1.0, 2.0, 3.0, 4.0, and 5.0 g of PFOTES into ethanol to make a total of 100 g of each solution, and then the solutions were sonicated for 10 min. For the preparation of the paints, 4 g of TiO2 and 40 mg of crystal violet were added to 40 mL PFOTES solution with constant stirring. Then the resulting solution was vortexed and sonicated for 5 min each. For the photocatalytic oxidation of VOCs, 1 mL of the paint solution was applied on the glass slide of 50 mm × 75 mm dimensions and dried for 3 h in the dark. Deionized water was used to wash the dried samples to remove the non-combined dye. The same preparation procedure was repeated for all the PFOTES solutions of 1–5%. The paint samples were named P-1, P-2, P-3, P-4, and P-5 in which the numbers correspond to the percentage solution of PFOTES.
Experimental details
A stainless steel photocatalytic oxidation (PCO) reactor loaded with the photocatalyst was used to study the photocatalytic activity of the paints and a Shimadzu GC/MS (QP2020) was used to analyze the input and output gas composition. Fig. 1 shows the instrumental setup used for the study. The input concentration was adjusted by the ratio between VOC and air. The total flow rate of 50 mL min−1 consisting of the VOC and air was introduced into the PCO reactor. The flow rate of the input gases was controlled by mass flow controllers (MFC) made by Kofloc, Kojima Instruments Inc. White lights of power 8 W and 24 W were used to activate the photocatalytic paint. The effect of humidity was studied between 60 and 70%. Gas peaks were identified by the National Institute of Standards and Technology (NIST) MS library whereas peak areas were calculated by using GCMS solution software. The following eqn (1) was used to calculate the photocatalytic VOC removal efficiency of the catalysts. | Conversion (%) = (1 − Aout/Ain) × 100 | (1) |
where Ain and Aout are the peak area of VOCs in the reactant gas and the product gas respectively.
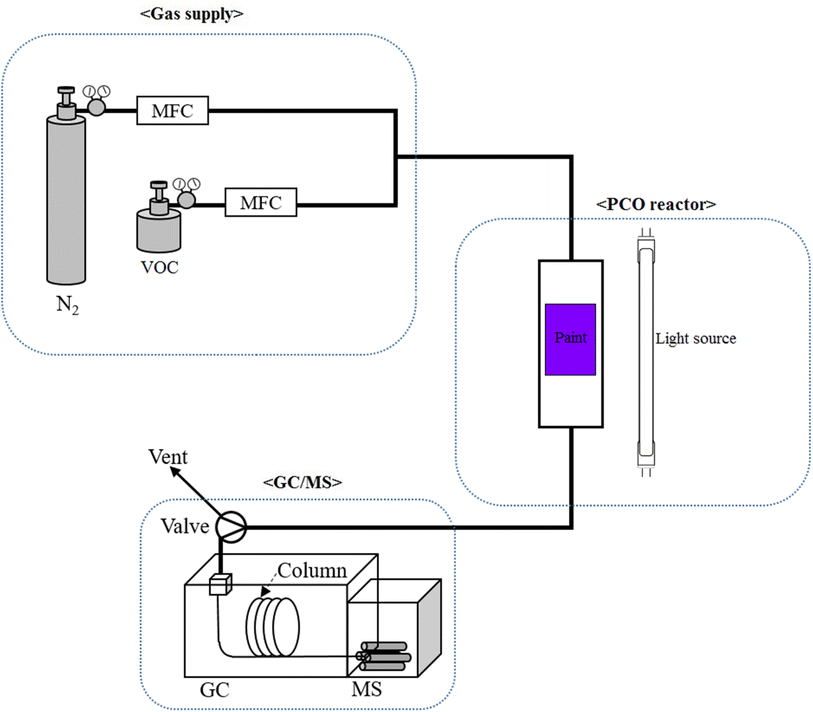 |
| Fig. 1 Schematic diagram of the photocatalytic oxidation setup. | |
Results and discussion
Input concentration of toluene
The effect of the concentration of toluene on photocatalytic decomposition is shown in Fig. 2. The photocatalytic activity of the prepared paints was studied in the visible light region by using a plate-type photocatalytic oxidation (PCO) reactor similar to a previous study.24 1 mL of the paint P-1 was spread on the glass slide to provide the surface for the reaction. The white light of 24 W power was used as a light source. The concentration of toluene was controlled by changing the input flow rate of toluene. The total flow rate (air + toluene) of the input gas was set at 50 mL min−1. Various input concentrations of toluene including 2, 3, 4, and 5 ppm were analyzed to study the photocatalytic ability of the prepared paints against VOCs. As shown in Fig. 2, it was found that the photocatalytic activity decreased with the increase in the input concentration. The highest catalytic activity was obtained with a concentration of 2 ppm at which up to 93% toluene was successfully converted to CO2via photocatalytic oxidation. The conversion gradually decreased to around 33% at a 5 ppm concentration. These results suggest that TiO2 paints made with PFOTES and CV have a photocatalytic ability to decompose VOCs to environmentally benign molecules in addition to bactericidal activity as mentioned by Hwang et al.21
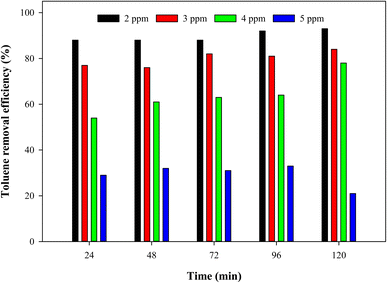 |
| Fig. 2 Photocatalytic activity of P-1 at various toluene inputs with a total flow rate of 50 mL min−1, under 24 W white light. | |
PFOTES amount
Silanes such as 1H,1H,2H,2H-perfluorooctyltriethoxysilane (PFOTES) are used to increase the hydrophobicity of materials, which can help in the self-cleaning process.25 In another study, it was suggested that PFOTES can help in CV deposition due to interstitial spaces and electrostatic attraction.23 It was revealed that PFOTES keeps the dye from leaching. The study also stated that the bond between PFOTES and TiO2 could also be covalent. Additionally, scientists mentioned that TiO2 was found to have a covalent bond with another silane group, 3-methacryloxypropyltrimethoxysilane (MPS).26 The hydrophobicity of TiO2 was increased due to Ti–O–Si bonds. In our study, PFOTES solutions of various concentrations (1–5%) were used in the preparation to study its effect on the photocatalytic activity of the paint against toluene. Fig. 3 shows the photocatalytic activity of paints P-1, P-2, P-3, P-4, and P-5. 1 mL of each paint was applied to different glass slides which were used in the PCO reactor. 3 ppm toluene concentration was introduced with the total input flow rate of 50 mL min−1 under 24 W white light. It was observed that photocatalytic activity slightly increased when the PFOTES amount was increased from 1–3%. Paint P-2 was found to have about 88% efficiency whereas paint P-3 shows the highest performance with 92% efficiency in comparison with 84% of paint P-1. This change in performance suggests that the PFOTES–TiO2 interaction increased when the concentration of PFOTES was increased (to 3%). As mentioned earlier, higher attachment of PFOTES on TiO2 will have higher deposition of CV which can increase the performance. Qi et al. also stated that a lower amount of modifier such as 1% can be insufficient and more polymer can be attached to TiO2.26 On the other hand, the performance significantly dropped in paints P-4 and P-5. The efficiency of P-4 and P-5 decreased to 45% and 23% respectively. We believe that a very high amount of PFOTES hinders the active sites which ultimately decreased the radical generation, thus decreasing the photocatalytic ability. Zhu & Wang have mentioned in their review on photocatalysis that a combination of photocatalysts with additional materials can also block the active sites and shield the light absorption.27 In another study, the authors observed similar results when the decomposition of VOCs increase up to a certain point and then decreased.28 They suggested that VOCs can also contaminate the active sites on the hydrophobic surfaces of the photocatalysts. This contamination can lead to a downward trend in photocatalytic performance.
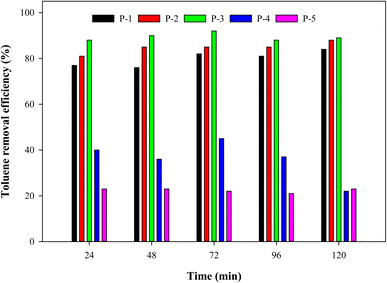 |
| Fig. 3 The photocatalytic activity of paints P-1, P-2, P-3, P-4, and P-5 against 3 ppm toluene with a total flow rate of 50 mL min−1, under 24 W white light. | |
Power source
Fig. 4 shows the photocatalytic activity of paint P-3 under the white light of power 8 W and 24 W. It was observed that the photocatalytic performance decreased with a decrease in the light power. 73% efficiency was observed with the white light of 8 W, as compared to 92% with 24 W. Other researchers also noted the change in photocatalytic performance with the change in light intensity.29,30 The authors stated that higher intensity is helpful in generating a higher number of radicals. Despite this fact, the photocatalytic performance was found to be decent under the light of lower power.
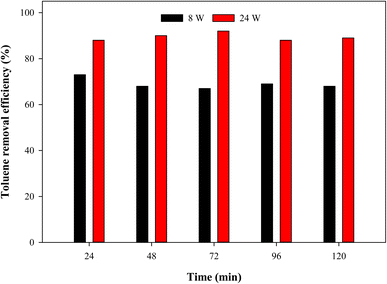 |
| Fig. 4 Photocatalytic activity of the paint P-3 under 8 W and 24 W white light against 3 ppm toluene at a total flow rate of 50 mL min−1. | |
Catalyst characterization
Fig. 5 shows the DRS spectra of the paints P-1, P-2, P-3, P-4, and P-5. The DRS was measured in the wavelength range of 240–700 nm. It was observed that the absorption increased with an increase in the amount of PFOTES to 3% and then it decreased with a further increase in PFOTES such as 4% and 5% solutions. These results are in accordance with the photocatalytic performance of the paints. As mentioned earlier in this study, the photocatalytic performance also increased to 3% PFOTES and then decreased. It strengthens the suggestion made previously that the PFOTES amount of less than 3% is insufficient whereas, more than 3% is responsible for light shielding.26,27
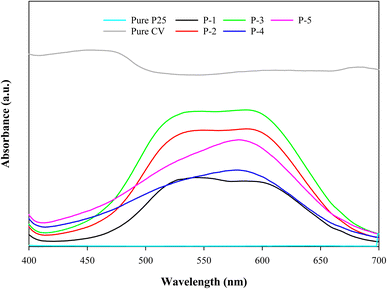 |
| Fig. 5 DRS spectra of the paints P-1, P-2, P-3, P-4, P-5, pure P25, and pure CV. | |
The converted Tauc plot of CV, P-3 and TiO2 is given in Fig. S1 in the ESI.† The bandgap values of CV, P-3 and TiO2 were calculated as 1.99 eV, 2.95 eV and 3.09 eV, respectively. The results indicate that under visible-light irradiation, photo-generated electrons can transfer from CV to TiO2, resulting in electron accumulation in TiO2. The excessive electron accumulation in TiO2 will promote electron transfer to the environment, leading to a redox reaction. We have also performed SEM to determine the particle size and surface morphology of the photocatalytic paint P-3. The results are given in Fig. S2 in the ESI.† Paint P-3 showed a spherical shape with a diameter of 33.2 ± 4.7 μm.
The FTIR spectrum of paint P-3 is compared with those of TiO2 and CV in Fig. 6. The FTIR transmittance was observed in the wavenumber range of 400–4000 cm−1. The FTIR spectra of the paint and TiO2 are found to be very similar. The broad absorption band which corresponds to the Ti–O stretching vibration was observed in the spectrum of TiO2 in the region 400–890 cm−1. The broad peaks of adsorbed water have appeared at 1636 and 3352 cm−1. The same peaks were also found in paint P-3. Although, in the spectrum of P-3, additional peaks were also observed which correspond to the presence of PFOTES. The peaks at 1047, 1146, 1212, and 1247 cm−1 were observed due to the C–F2 bands. Additionally, the peak attributed to C–H2 can be seen at 1636 cm−1. The C–F3 peak (820–890 cm−1) may have been merged with the Ti–O band. Similar spectra can also be seen in a previous study where researchers treated TiO2 with PFOTES.25
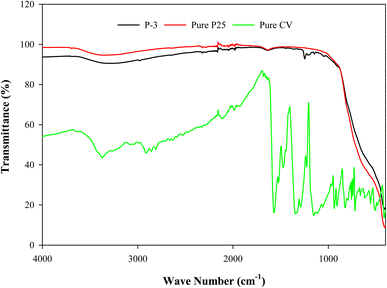 |
| Fig. 6 FTIR spectra of P-3, pure P25, and pure CV. | |
XPS data of the paint P-3 are given in Fig. 7. The XPS spectra were studied in the range of 0–1300 eV. The XPS spectra of paint P-3 confirm the coordination of TiO2, PFOTES, and CV. The peaks of Ti, O, F, C, Si, and N were observed. The Ti 2p3/2 and Ti 2p1/2 peaks of Ti4+ were observed at 458.1 eV and 463.0 eV respectively. The O 1s peak of oxygen was found at 529.3 eV. F 1s, C 1s, and Si 2p peaks were observed at 688.1, 284.7, and 101.3 eV respectively. These peaks signify the attachment of PFOTES on the TiO2 surface. The peak of N 1s was observed at 398.3 eV which represents the presence of CV. Similar XPS results have been reported in a previous study for the preparation of TiO2 paints with PFOTES and CV.23
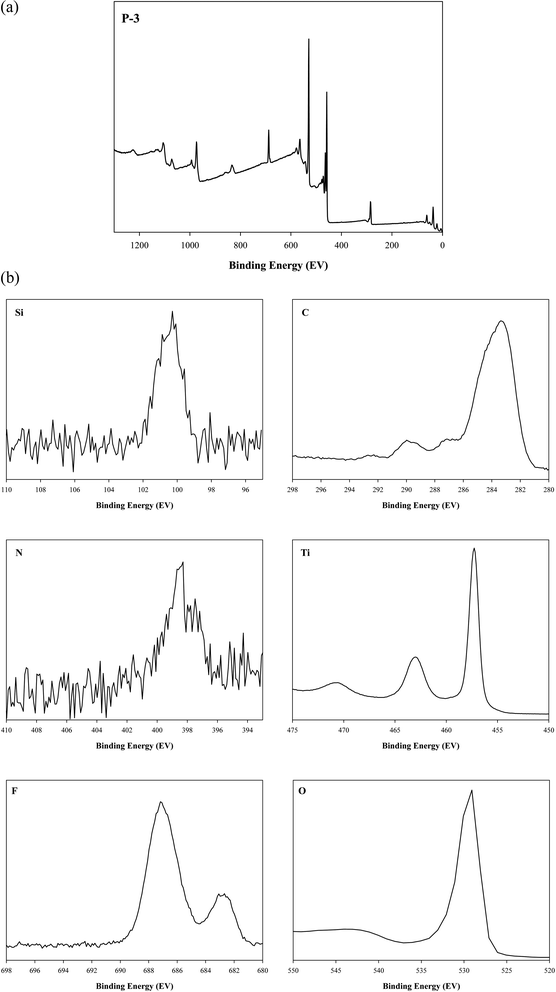 |
| Fig. 7 (a) XPS spectrum of the paint P-3 and (b) XPS peaks of the individual elements in P-3. | |
Decomposition of p-xylene and acetaldehyde
Since paint P-3 was found to have the maximum photocatalytic ability among the prepared paints against toluene, the photocatalytic activity of P-3 was also studied for p-xylene and acetaldehyde. The photocatalytic performance of paint P-3 against p-xylene is given in Fig. 8. 3 ppm concentration of p-xylene at a total flow rate of 50 mL min−1 was introduced in the reactor loaded with 1 mL of P-3 under 24 W visible light. It was observed that about 99% of photocatalytic efficiency was achieved against p-xylene. CO2 was found to be the by-product of the photocatalytic decomposition of p-xylene. Similarly, acetaldehyde also has shown substantial decomposition under the same conditions. Besides CO2, no other by-products were observed when the photocatalytic decomposition of acetaldehyde was studied. The photocatalytic performance of the superhydrophobic visible light-activated PFOTES–CV based TiO2 paint for various VOCs establishes its versatility as a futuristic paint.
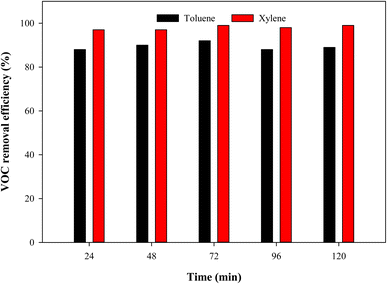 |
| Fig. 8 Photocatalytic activity of P-3 against 3 ppm toluene and 3 ppm p-xylene at a total flow rate of 50 mL min−1 under 24 W white light. | |
Effect of humidity
The photocatalytic performance of paint P-3 against toluene was also studied under humidity to simulate real atmospheric conditions. It was observed that humidity has a negative impact on the photocatalytic performance of the paint for toluene. The catalytic efficiency of P-3 against toluene decreased from 92% to 25%. It has been reported in the literature that humidity has both positive and negative effects on the photocatalytic activity of TiO2 against VOCs depending on various factors.31 The photocatalytic performance trend of TiO2 (P25) against toluene has been reported to differ considerably under humid conditions. Increase in the paint pore size can lead to a higher conversion of VOCs under humidity. An increase in the amount of P25 can increase the performance by decreasing the competition between VOCs and water.32 Hence, it is suggested that further research is required to enhance the performance of the PFOTES–CV-based TiO2 paints under humid conditions to make their use more practical.
Decomposition mechanism
TiO2 in combination with CV generates the O2˙− radical from oxygen in air under visible light.23 These radicals can react with VOCs such as toluene, p-xylene, and acetaldehyde. VOC pollutants convert into carbon dioxide and water after the reaction.15 The decomposition mechanism of VOCs is given as the following equations. | TiO2 + CV + O2 + hν → O2˙− | (2) |
| VOCs + O2˙− → CO2 + H2O | (3) |
Conclusion
The photocatalytic oxidation performance of superhydrophobic-visible light-activated PFOTES–CV-based TiO2 paints against VOCs has been studied in this article. These photocatalytic TiO2 paints are reported to have bactericidal activity under visible light in the literature and are suggested to have a wide range of applications due to easy and fast preparation and utilization. To increase the practicality of these paints, we have investigated their photocatalytic performance against toluene, p-xylene, and acetaldehyde under visible light. Moreover, various parameters such as the input concentration of toluene, amount of PFOTES, light power, and humidity were also examined. DRS, FTIR, and XPS were used for the characterization of the PFOTES–CV-based TiO2 paints, whereas the PCO reactor was used to examine the photocatalytic efficiency under white light. It was concluded that the photocatalytic efficiency decreased with the increase in the input concentration of toluene. The addition of PFOTES has a significant effect on the photocatalytic activity of superhydrophobic paints against toluene. The photocatalytic efficiency increased till the addition of a 3% PFOTES (∼92%) solution and then decreased with the addition of a higher concentration solution of PFOTES. The photocatalytic paints have shown reasonable decomposition efficiency for p-xylene (∼99%) and acetaldehyde under visible light. The decrease in the photocatalytic performance of the paints was noticed when the light intensity was decreased as well as under humidity. Due to their photobactericidal and photocatalytic efficiency against VOCs, these paints/photocatalysts have a very high potential for various applications. Even so, further study is suggested in the future to enhance their performance under humid conditions. Materials such as metal oxides (ZnO, NiO, WO3, MnO2), platinum supported materials (Pt/Al2O3–CeO2), silver-based compounds (AgBr, AgBr/WO3), and carbon based materials (CNT, graphene, graphene oxide) are some of the reported photocatalysts. It would be interesting to study their activity in photocatalytic paints.
Author contributions
Conceptualization: AA, VCTL; original draft: AA. Writing – review & editing, SK, VCTL, MS.
Conflicts of interest
The authors declare that they have no conflict of interest.
Acknowledgements
This work is financially supported by the Korea Ministry of Environment as Waste to Energy-Recycling Human Resource Development Project (YL-WE-21-001). We thank Tailer D. Cartwright for her contribution to the improvement of this manuscript.
References
-
Air Pollution, available online: https://www.who.int/health-topics/air-pollution, accessed on Feb 9, 2022 Search PubMed.
-
Volatile Organic Compounds' Impact on Indoor Air Quality, US EPA, available online: https://www.epa.gov/indoor-air-quality-iaq/volatile-organic-compounds-impact-indoor-air-quality, accessed on Feb 10, 2022 Search PubMed.
-
S. S. Anand, B. K. Philip and H. M. Mehendale, Volatile Organic Compounds, Encycl. Toxicol. Third Ed., 2014, pp. 967–970, DOI:10.1016/B978-0-12-386454-3.00358-4.
- C. Yang, G. Miao, Y. Pi, Q. Xia, J. Wu, Z. Li and J. Xiao, Abatement of various types of VOCs by adsorption/catalytic oxidation: a review, Chem. Eng. J., 2019, 370, 1128–1153, DOI:10.1016/J.CEJ.2019.03.232.
-
V. Soni, P. Singh, V. Shree and V. Goel, Effects of VOCs on Human Health, Energy, Environ. Sustain., 2018, pp. 119–142, DOI:10.1007/978-981-10-7185-0_8.
- W. Wei, S. Cheng, G. Li, G. Wang and H. Wang, Characteristics of volatile organic compounds (VOCs) emitted from a petroleum refinery in Beijing, China, Atmos. Environ., 2014, 89, 358–366, DOI:10.1016/J.ATMOSENV.2014.01.038.
- E. David and V. C. Niculescu, Volatile Organic Compounds (VOCs) as Environmental Pollutants: Occurrence and Mitigation Using Nanomaterials, Int. J. Environ. Res. Public Health, 2021, 18, 13147, DOI:10.3390/IJERPH182413147.
- Y. Qi, Z. Yang, Y. Jiang, H. Han, T. Wu, L. Wu, J. Liu, Z. Wang and F. Wang, Platinum-Copper Bimetallic Nanoparticles Supported on TiO2 as Catalysts for Photo-thermal Catalytic Toluene Combustion, ACS Appl. Nano Mater., 2022, 5(2), 1845–1854, DOI:10.1021/acsanm.1c03429.
- S. Li, X. Yu, X. Dang, P. Wang, X. Meng, Q. Wang and H. Hou, Double dielectric barrier discharge incorporated with CeO2-Co3O4/γ-Al2O3 catalyst for toluene abatement by a sequential adsorption–discharge plasma catalytic process, J. Clean. Prod., 2022, 340, 130774, DOI:10.1016/J.JCLEPRO.2022.130774.
- H. Touati, S. Valange, M. Reinholdt, C. Batiot-Dupeyrat, J.-M. Clacens and J.-M. Tatibouët, Low Temperature Catalytic Oxidation of Ethanol Using Ozone over Manganese Oxide-Based Catalysts in Powdered and Monolithic Forms, Catalysts, 2022, 12, 172, DOI:10.3390/catal12020172.
- Y. Zhang, M. Wu, Y. Wang, X. Zhao and D. Y. C. Leung, Low-cost and efficient Mn/CeO2 catalyst for photocatalytic VOCs degradation via scalable colloidal solution combustion synthesis method, J. Mater. Sci. Technol., 2022, 116, 169–179, DOI:10.1016/J.JMST.2021.11.041.
- Z. Shayegan, C.-S. Lee and F. Haghighat, TiO2 photocatalyst for removal of volatile organic compounds in gas phase – a review, Chem. Eng. J., 2018, 334, 2408–2439, DOI:10.1016/j.cej.2017.09.153.
- Y. Nosaka and A. Y. Nosaka, Generation and Detection of Reactive Oxygen Species in Photocatalysis, Chem. Rev., 2017, 117, 11302–11336, DOI:10.1021/ACS.CHEMREV.7B00161.
- V. Etacheri, C. Di Valentin, J. Schneider, D. Bahnemann and S. C. Pillai, Visible-light activation of TiO2 photocatalysts: advances in theory and experiments, J. Photochem. Photobiol., C, 2015, 25, 1–29, DOI:10.1016/J.JPHOTOCHEMREV.2015.08.003.
- J. Kim and B. K. Lee, Enhanced photocatalytic decomposition of VOCs by visible-driven photocatalyst combined Cu-TiO2 and activated carbon fiber, Process Saf. Environ. Prot., 2018, 119, 164–171, DOI:10.1016/J.PSEP.2018.07.026.
- R. Molinari, C. Lavorato and P. Argurio, Visible-Light Photocatalysts and Their Perspectives for Building Photocatalytic Membrane Reactors for Various Liquid Phase Chemical Conversions, Catalysts, 2020, 10, 1334, DOI:10.3390/catal10111334.
- V. Etacheri, M. K. Seery, S. J. Hinder and S. C. Pillai, Oxygen Rich Titania: A Dopant Free, High Temperature Stable, and Visible-Light Active Anatase Photocatalyst, Adv. Funct. Mater., 2011, 21, 3744–3752, DOI:10.1002/ADFM.201100301.
- M. Humayun, F. Raziq, A. Khan and W. Luo, Modification strategies of TiO2 for potential applications in photocatalysis: a critical review, Green Chem. Lett. Rev., 2018, 11, 86–102, DOI:10.1080/17518253.2018.1440324.
- S. Rehman, R. Ullah, A. M. Butt and N. D. Gohar, Strategies of making TiO2 and ZnO visible light active, J. Hazard. Mater., 2009, 170, 560–569, DOI:10.1016/j.jhazmat.2009.05.064.
- R. Abe, K. Hara, K. Sayama, K. Domen and H. Arakawa, Steady hydrogen evolution from water on Eosin Y-fixed TiO2 photocatalyst using a silane-coupling reagent under visible light irradiation, J. Photochem. Photobiol., A, 2000, 137, 63–69, DOI:10.1016/S1010-6030(00)00351-8.
- G. B. Hwang, A. Patir, E. Allan, S. P. Nair and I. P. Parkin, Superhydrophobic and White Light-Activated Bactericidal Surface through a Simple Coating, ACS Appl. Mater. Interfaces, 2017, 9, 29002–29009, DOI:10.1021/acsami.7b05977.
- S. Wang and L. Jiang, Definition of Superhydrophobic States, Adv. Mater., 2007, 19, 3423–3424, DOI:10.1002/ADMA.200700934.
- K. J. Heo, S. B. Jeong, J. Shin, G. B. Hwang, H. S. Ko, Y. Kim, D. Y. Choi and J. H. Jung, Water-Repellent TiO2-Organic Dye-Based Air Filters for Efficient Visible-Light-Activated Photochemical Inactivation against Bioaerosols, Nano Lett., 2021, 21, 1576–1583, DOI:10.1021/acs.nanolett.0c03173.
- R. Yang, Y.-P. Zhang and R.-Y. Zhao, An Improved Model for Analyzing the Performance of Photocatalytic Oxidation Reactors in Removing Volatile Organic Compounds and Its Application, J. Air Waste Manage. Assoc., 2004, 54, 1516–1524, DOI:10.1080/10473289.2004.10471016.
- S. Pazokifard, S. M. Mirabedini, M. Esfandeh and S. Farrokhpay, Fluoroalkylsilane treatment of TiO2 nanoparticles in difference pH values: characterization and mechanism, Adv. Powder Technol., 2012, 23, 428–436, DOI:10.1016/J.APT.2012.02.006.
- Y. Qi, B. Xiang, W. Tan and J. Zhang, Hydrophobic surface modification of TiO2 nanoparticles for production of acrylonitrile-styrene-acrylate terpolymer/TiO2 composited cool materials, Appl. Surf. Sci., 2017, 419, 213–223, DOI:10.1016/J.APSUSC.2017.04.234.
- S. Zhu and D. Wang, Photocatalysis: Basic Principles, Diverse Forms of Implementations and Emerging Scientific Opportunities, Adv. Energy Mater., 2017, 7, 1700841, DOI:10.1002/AENM.201700841.
- A. Najafidoust, E. Abbasi Asl, H. Kazemi Hakki, M. Sarani, H. Bananifard, M. Sillanpaa and M. Etemadi, Sequential impregnation and sol-gel synthesis of Fe-ZnO over hydrophobic silica aerogel as a floating photocatalyst with highly enhanced photodecomposition of BTX compounds from water, Sol. Energy, 2021, 225, 344–356, DOI:10.1016/J.SOLENER.2021.07.035.
- C. Chen, C. Wu, L. Hsieh and K. Chen, Treatment of Trichloroethylene with Photocatalyst-Coated Optical Fiber, Water, 2019, 11, 2391, DOI:10.3390/w11112391.
- J. Chu and L. Zhong, Photocatalytic Degradation of Methylene Blue with Side-glowing Optical Fiber Deliverying Visible Light, Chin. J. Chem. Eng., 2012, 20, 895–899, DOI:10.1016/S1004-9541(12)60415-7.
- A. H. Mamaghani, F. Haghighat and C. S. Lee, Photocatalytic oxidation technology for indoor environment air purification: the state-of-the-art, Appl. Catal. B Environ., 2017, 203, 247–269, DOI:10.1016/J.APCATB.2016.10.037.
- J. Jeong, K. Sekiguchi, W. Lee and K. Sakamoto, Photodegradation of gaseous volatile organic compounds (VOCs) using TiO2 photoirradiated by an ozone-producing UV lamp: decomposition characteristics, identification of by-products and water-soluble organic intermediates, J. Photochem. Photobiol., A, 2005, 169, 279–287, DOI:10.1016/J.JPHOTOCHEM.2004.07.014.
- M. Sheraz, K. A. Mir, A. Anus, V. C. T. Le, S. Kim, V. Q. Nguyen and W. R. Lee, SARS-CoV-2 airborne
transmission: a review of risk factors and possible preventative measures using air purifiers, Environ. Sci.: Processes Impacts, 2022 10.1039/d2em00333c.
- V. C. T. Le, M. Sheraz, E. Kang, H. N. Ly, H. D. Mai, A. Anus and S. Kim, Alumina beads decorated copper-based coordination polymer particle filter for commercial indoor air cleaner, Build. Environ., 2022, 217, 109012, DOI:10.1016/j.buildenv.2022.109012.
- V. C. T. Le, T. N. Thanh, E. Kang, S. Yoon, H. D. Mai, M. Sheraz, T. U. Han, J. An and S. Kim, Melamine sponge-based copper-organic framework (Cu-CPP) as a multi-functional filter for air purifiers, Korean J. Chem. Eng., 2022, 39, 954–962, DOI:10.1007/s11814-021-1000-4.
- V. C. T. Le, M. Sheraz, E. Kang, H. N. Ly, H. D. Mai, W. R. Lee, C. Kim and S. Kim, Four-in-one multifunctional air filter using copper coordination polymer particle decorated fibre for efficient pathogen removal and indoor air treatment, Process Saf. Environ. Prot., 2022, 166, 177–188, DOI:10.1016/j.psep.2022.08.007.
- V. C. T. Le, S. Yoon, E. Kang, M. Sheraz, T. U. Han, A. Anus, H. D. Mai, S. Choi and S. Kim, A capture and inactivation system against pathogens in indoor air using copper nanoparticle decorated melamine sponge hybrid air filters, Environmental Science: Advances, 2022, 1, 356, 10.1039/d2va00041e.
|
This journal is © The Royal Society of Chemistry 2023 |
Click here to see how this site uses Cookies. View our privacy policy here.