DOI:
10.1039/D3SU90043F
(Essay)
RSC Sustain., 2023,
1, 1604-1607
The excellence of chemical science in achieving a sustainable world
First published on 5th October 2023
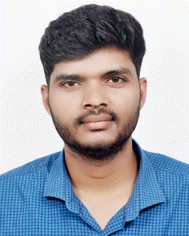 Selvakumar Selvarasu | Selvakumar Selvarasu originates from and lives in India. He studied MSc in chemistry in D. G. Vaishnav College, affiliated to University of Madras, India. He has won prizes in intercollege competitions in ChemTalk, ChemTunes, and ChemQuiz topics. After MSc., he was qualified for UGC fellowship. He is now studying Ph. D. in Indian Institute of Science, Bangalore, India. His work focusses on activation of small molecules by rhodium and iridium pincer complexes. He has received Carl Storm International Diversity Fellowship. His ambition is to contribute his research skills in converting CO2 and CH4 into commodity chemicals and sustainable chemistry. |
1. Introduction
Abnormal changes like global warming, ocean acidification, melting glaciers, extreme weather conditions, and biodiversity loss in the Earth's climate and environment are growing concerns for scientists, policymakers, and the public worldwide. These changes are mainly due to human activities. Chemical science plays a vital role in addressing the issues. Utilizing Earth's elemental energy resources with chemical science's aid is crucial. This can lead to development of green energy systems, reduce greenhouse gas emissions, improve waste management processes, and create sustainable materials and methods, like biodegradable plastics and green chemistry techniques.
However, more than science and technological solutions, social and political solutions are needed to address the complex environmental changes. We also need to adopt sustainable practices and policies globally, like reducing our reliance on fossil fuels and promoting renewable energy and circular economy principles. Working to preserve and protect our natural resources, like forests, oceans, and wildlife, is essential for maintaining biodiversity and mitigating the impact of climate change. This essay will mainly focus on the excellence of chemical science and technology in addressing the issues and briefly discuss policies.
2. Chemical science for a sustainable world
The abnormal changes in the Earth's climate and environment are mainly due to the continuous increase in CO2 concentration.1 Prior to 1911, the atmospheric CO2 concentration remained below 300 parts per million (ppm). Subsequently, it experienced a pronounced and accelerating increase, primarily driven by the growth in human population, the proliferation of automobiles, and the expansion of industrial activities. As of this year, the concentration has surged to 420 ppm.1 The annual CO2 emission from burning fossil fuels and industrial processes was 36.2 gigatons in 2019, increasing with an average rate of around 1.4% yearly.2 There are two important ways to decrease CO2 concentration with the aid of chemical science. Firstly, capturing CO2 from industrial processes and the atmosphere directly and storing it, and secondly, converting CO2 into value-added chemicals by chemical reactions. They are mainly done by carbon capture utilization (CCU) and storage (CCS) processes. Despite 400 million tons of CO2 being captured annually, this is a small fraction of the global emissions,1 and the initiation and continuous development of efforts to increase the amount of CO2 being captured are desired. In capturing processes, various chemical solvents, adsorbents, and membranes are used.3 In utilizing processes (Fig. 1), catalytic and electrochemical reactions are carried out to convert CO2 into chemicals like methanol and ethanol.4
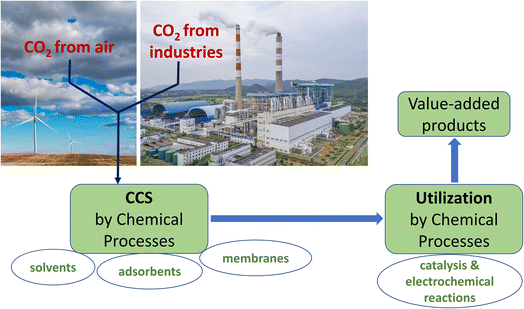 |
| Fig. 1 Carbon capture storage (CCS) and utilization by chemical processes. | |
Research is ongoing to develop new types of sorbents and membranes that can capture CO2 more effectively with lower energy requirements. Some materials are based on metal–organic frameworks (MOFs) that can selectively adsorb CO2. Other research is aiming to develop new types of catalysts that can convert CO2 into valuable chemicals selectively and efficiently. In addition, researchers are investigating the use of renewable energy resources (RER) like solar and wind power to power the CCU and CCS processes instead of using fossil fuels to reduce CO2 emissions further.5
Plastic waste is another significant concern. Plastic pollution adversely affects the environment and health, mainly marine life, land pollution, and ecosystem disruption. Around 300 million tons of plastic waste are generated annually.6 14% of plastic packaging is collected for recycling, and just 2% is effectively recycled into new materials.7 Plastic waste is recycled by mechanical, chemical, and feedstock recycling processes depending on the type of plastic and desired products.
Chemical recycling often involves pyrolysis, gasification, and depolymerization.8 The processes obtain value-added products like bio-oil, syngas, monomers, and oligomers (Fig. 2). One of the research areas is on improving the yield of the products. Another area is exploring new catalytic reactions and their reaction conditions to increase the efficiency and selectivity in pyrolysis processes. Improvements in plasma-based gasification methods for the conversion of plastic waste into hydrogen and other valuable products are also in progress.9
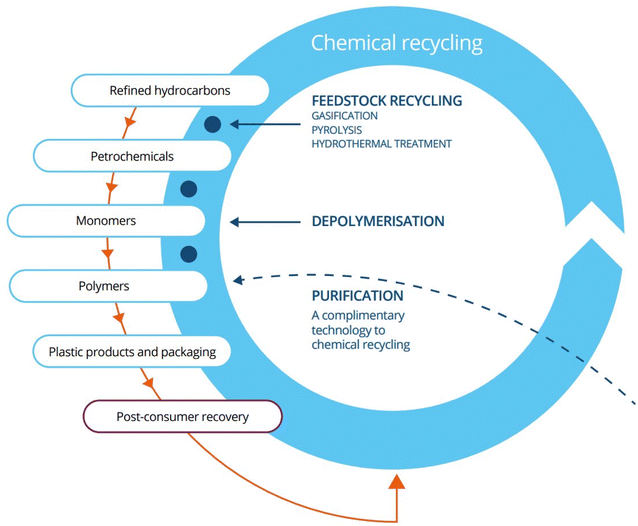 |
| Fig. 2 Chemical recycling of plastic waste.10 | |
Traditional energy resources (TER), coal, oil, and natural gas, are the major contributors to around 75% of global greenhouse emissions.11 Hence, the reliance on them must be replaced as much as possible with RER, such as solar, wind, and geothermal energies, biomass, and hydropower. The development of RER heavily relies on the contribution of chemical science. In this field, chemical science contributes in various ways, like material and energy device developments, improving catalytic processes, and process optimization.12
Scientists are developing efficient catalysts for hydrogen production and biomass conversion into fuels and chemicals in catalysis. Hydrogen is produced by the electrolysis of water, thermochemical processes, steam methane reforming, coal gasification, and a concentrated solar power process.13 Biomass is converted into fuels and chemicals by transesterification, fermentation, and hydrolysis processes.14 In materials and energy storage developments, new materials and methods have been developed for efficient solar cells (perovskite and organic materials), batteries (lithium–sulfur and sodium-ion batteries), and supercapacitors (graphene and carbon nanotubes, MOFs, and 2D materials).15 In the optimization process, scientists optimize the production processes for renewable energy technologies like photovoltaic and hydrogen fuel cells to reduce costs and improve scalability.16,17 In photovoltaic cells, silicon semiconductors convert sunlight into electricity. In hydrogen fuel cells, hydrogen and oxygen are used to produce electricity, with water as the only byproduct.
Green chemistry aims to design and develop chemical products and processes that reduce or eliminate the use and generation of hazardous substances like toxic chemicals, radioactive materials, greenhouse gases, and ozone-depleting substances.18 Some important principles, atom economy, solvent selection, biocatalysts, process intensification, and life cycle assessment, are involved in this field.19 This approach has a significant impact on addressing climate change and promoting the development of RER. It helps reduce the environmental impact of chemical manufacturing, which can reduce greenhouse gas emissions and other pollutants. It contributes to the development of new sustainable materials, such as bioplastics and biodegradable materials.
Another attractive and growing field is the hydrogen economy. It involves hydrogen production using RER as a fuel in transportation, power generation, and other industrial processes. One of the significant advantages of the hydrogen economy is zero emissions, with only water as the byproduct. This field has four stages: hydrogen production, storage, transport, and usage. In all of them, many chemical processes and materials are involved.20,21 Some of the processes for hydrogen production have been discussed above in the part on catalysis. In hydrogen storage and transport, solid materials such as metal hydrides, carbon nanotubes, and graphene are used. Ammonia and formic acids are used as chemical hydrogen storage. Liquid organic hydrogen carriers (LOHCs) can be used for transport over long distances. Scientists are working in the field to overcome the challenges of improving processes and materials' efficiency.
3. Policies
Along with the developments in science and technology to solve the issues, updating the existing policies as well as mandatory implementation and following them in society must also be done globally. In addition to the globally existing approaches, the following guidelines can be considered. Worldwide conferences on reducing CO2 concentration, recycling plastic waste, and developing sustainable systems must be conducted frequently to share knowledge among scientists and bring innovative ideas and proposals. All the countries must collectively work to attach carbon recycling processes like CCS and CCU to all the existing industries that emit CO2 and to increase the number of plants worldwide. Permission to start new industries must be denied if their processes emit more CO2. Many appreciable policies for reducing and recycling plastic waste exist globally. However, they are not followed strictly in all countries.
People must be taught to learn all the problems and solutions from childhood itself. To gain attention and awareness enormously, all the countries must often promote it through famous people like sports players, cinema celebrities, etc. The number of electric vehicles in transportation must be increased to reduce the usage of cars that burn hydrocarbons. The use of RER in all the fields of energy production and storage instead of TER must be raised as quickly as possible. All countries must increase the number of educational and industrial research and development technologies for a sustainable world. Research and development for improving the Earth's climate and environment must be prioritized over other purposes.
4. Conclusions
CO2 emission, plastic waste, and other industrial waste are the primary reasons for the abnormal changes. Reducing them by recycling and replacing the usage of TER with RER are effective solutions for the problems. Chemical science and technology deliver immense efforts to address the issues in this goal. Research is growing in this field in many countries, especially in increasing the usage of RER, achieving the principles of green chemistry, and exploring the hydrogen economy. Periodic updating of existing policies, adding new approaches according to the current situations, and international negotiations for sustainable practices globally are equally essential, along with the development of science and technology to address the issues. Let us work together for a sustainable world.
Acknowledgements
I would like to acknowledge Indian Institute of Science, Bangalore, India for financial support and facility. Prof Balaji R Jagirdar (research supervisor) for financial support and encouragement, and UGC, India for fellowship.
References
-
Global Climate Change: Carbon dioxide, NASA, March 2023, available at: https://climate.nasa.gov/vital-signs/carbon-dioxide/ Search PubMed.
-
Global Carbon Atlas, 2023, available at: http://www.globalcarbonatlas.org/en/content/welcome-carbon-atlas Search PubMed.
- M. Bui,
et al, Carbon capture and storage (CCS): the way forward, Energy Environ. Sci., 2018, 11, 1062–1176, 10.1039/C7EE02342A.
- A. Goeppert, M. Czaun, J. P. Jones, G. K. S. Prakash and G. A. Olah, Recycling of carbon dioxide to methanol and derived products – closing the loop, Chem. Soc. Rev., 2014, 43, 7995–8048, 10.1039/C4CS00122B.
- S. Sun, H. Sun, P. T. Williams and C. Wu, Recent advances in integrated CO2 capture and utilization: a review, Sustainable Energy & Fuels, 2021, 5, 4546, 10.1039/D1SE00797A.
- R. Geyer, J. R. Jambeck and K. L. Law, Production, use, and fate of all plastics ever made, Sci. Adv., 2017, 3, e1700782, DOI:10.1126/sciadv.1700782.
-
S. Kaza, L. C. Yao, P. Bhada-Tata and F. Van Woerden, What a Waste 2.0; what a Waste: A Global Snapshot of Solid Waste Management to 2050, Urban Development; World Bank, Washington, DC, 2018, available at: https://openknowledge.worldbank.org/entities/publication/d3f9d45e-115f-559b-b14f-28552410e90a Search PubMed.
- A. Rahimi and J. M. García, Chemical recycling of waste plastics for new materials production, Nat. Rev. Chem., 2017, 1, 0046 CrossRef.
- E. Sanjaya and A. Abbas, Plasma gasification as an alternative energy-from-waste (EFW) technology for the circular economy: An environmental review, Resour., Conserv. Recycl., 2023, 189, 106730, DOI:10.1016/j.resconrec.2022.106730.
-
Chemical Recycling 101, British Plastics Federation, 2023, available at: https://www.bpf.co.uk/plastipedia/chemical-recycling-101.aspx Search PubMed.
-
IPCC Fifth Assessment Report: Climate Change 2014, available at: https://www.ipcc.ch/report/ar5/syr/ Search PubMed.
- R. Schlögl, The role of chemistry in the Energy Challenge, ChemSusChem, 2010, 3, 209–222, DOI:10.1002/cssc.200900183.
-
Hydrogen production, ScienceDirect, Springer, 2023, available at https://www.sciencedirect.com/topics/earth-and-planetary-sciences/hydrogen-production Search PubMed.
- R. Calvo-Serrano, M. Guo, C. Pozo, Á. Galán-Martín and G. Guillén-Gosálbez, Biomass Conversion into Fuels, Chemicals, or Electricity? A network-based life cycle optimization approach applied to the European Union, ACS Sustainable Chem. Eng., 2019, 7, 10570–10582, DOI:10.1021/acssuschemeng.9b01115.
- L. Fagiolari, M. Sampó, A. Lamberti, J. Amici, C. Francia, S. Bodoardo and F. Bella, Integrated energy conversion and storage devices: Interfacing solar cells, batteries and supercapacitors, Energy Storage Mater., 2022, 51, 400–434, DOI:10.1016/j.ensm.2022.06.051.
-
Pcells, ScienceDirect, Springer, 2023, available at: https://www.sciencedirect.com/topics/earth-and-planetary-sciences/photovoltaic-cells Search PubMed.
- L. Fan, Z. Tu and S. H. Chan, Recent development for hydrogen and fuel cell technologies: A review, Energy Rep., 2021, 7, 8421–8446, DOI:10.1016/j.egyr.2021.08.003.
-
P. T. Anastas and J. C. Warner, Green Chemistry: Theory and Practice, Oxford University Press, Oxford, 1998 Search PubMed.
-
12 Principles of Green Chemistry, American Chemical Society, Washington DC, available at: https://www.acs.org/greenchemistry/principles/12-principles-of-green-chemistry.html Search PubMed.
- M. van der Spek, C. Banet, C. Bauer, P. Gabrielli, W. Goldthorpe, M. Mazzotti, S. T. Munkejord, N. A. Røkke, N. Shah and N. Sunny,
et al., Perspective on the hydrogen economy as a pathway to reach net-zero CO2 emissions in Europe, Energy Environ. Sci., 2022, 15, 1034–1077, 10.1039/D1EE02118D.
-
H2 at Scale: Deeply decarbonizing our energy system. HTAC Presentation, US Department of Energy, Washington DC, 6 April 2016, available at: http://www.hydrogen.energy.gov/pdfs/htac_apr16_10_pivovar.pdf Search PubMed.
|
This journal is © The Royal Society of Chemistry 2023 |