DOI:
10.1039/D2SU00143H
(Communication)
RSC Sustain., 2023,
1, 439-445
Acrolein-free synthesis of polyacrolein derivatives via the chemo-selective reduction of polyacrylates†
Received
21st December 2022
, Accepted 7th February 2023
First published on 7th February 2023
Abstract
In this research, chemo-selective partial reduction of polyacrylates was feasible by using the state-of-the-art reducing agent, lithium diisobutyl-t-butoxyaluminum hydride (LDBBA), to afford polymers featuring acrolein segments without the direct use of the acrolein monomer. Furthermore, the obtained polymers featuring acrolein segments were subjected to Passerini-3CR post-polymerization modification to demonstrate the synthetic benefits.
Sustainability spotlight
The acrolein monomer is one of the most important vinyl monomers due to the intrinsic reactivity owing to aldehyde moieties, thereby enabling a feasible post-polymerization modification of polymers featuring acrolein units. Despite its importance, the use of acrolein would face severe problems in modern chemistry due to its high toxicity and high volatility. Furthermore, we have installed bio-derived cinnamic acid via by-product free Passerini three component reaction. This work is aligned with the UN's Sustainable Development Goal 12 regarding the sustainable consumption and production of chemical products.
|
Introduction
As illuminated by the importance of functionalized polymers, polymers featuring high reactivity have been spotlighted. In this context, the integration of click reactions has accelerated the production of functionalized polymers based on the state-of-the-art chemical tools.1–6 For this purpose, the key lies in the employment of highly reactive functional groups that would allow the chemical conversion of polymers. Among reactive functional units, aldehydes should be one of the most important functionalities due to their high reactivity for a wide range of organic transformation reactions. Considering that the aldehyde group is the smallest carbonyl unit, a vinyl-conjugated aldehyde, namely acrolein, would be a promising building block in polymer science because acrolein can be polymerized under standard vinyl polymerization conditions. As such, acrolein-containing polymers have found their way in a range of material applications due to the inherent reactivity of the aldehyde moieties.7–9 Despite the potential importance of acrolein in modern polymer chemistry, the use of acrolein would not be straightforward due to its chemical nature.10 First, acrolein shows high toxicity and thus its commercial access and lab use have been limited to avoid unnecessary contact. Second, acrolein shows multiple reactive sites owing to its conjugated structure, which suppresses the direct use of acrolein in a modern chemical conversion. Third, polymers featuring acrolein units often suffer from insolubility in common organic solvents, thereby increasing the difficulty in handling and chemical synthesis.10 Unfortunately, the protection–deprotection strategy would not be helpful in the case of acrolein because standard aldehyde protection, such as acetalization, would inevitably result in the loss of vinyl polymerizability by diminishing the efficient conjugation between vinyl and carbonyl groups. These considerations encouraged us to propose a novel synthetic strategy that would allow the installation of acrolein units into polymer structures.
Considering the above-mentioned background, the employment of the post-polymerization modification protocol should provide a promising alternative synthetic pathway to polymers featuring acrolein units. In cases where conventional reducing agents are used for the poly(meth)acrylate reductions, polymers featuring ester units are reduced directly to alcohol derivatives since the aldehyde intermediates are in situ reduced with the same reducing agents.11,12 Furthermore, polymers featuring esters are converted into alkyl units via the decarboxylation pathway in recent contributions.13–15 However, the direct and selective chemical conversion of polymers featuring esters into those with aldehydes has been scarcely developed. In this context, we have turned our attention to the recent developments in chemo-selective partial reduction of esters.16–19 Provided that esters can be used as a precursor, polyacrylates can be considered as a starting polymer for acrolein-featuring polymers. This would be crucially important because polyacrylates are commercially available and numerous living/controlled polymerization techniques are available for polyacrylate synthesis.20–22 Though the partial reduction of esters is difficult with conventional reducing agents, An and co-workers have reported lithium diisobutyl-t-butoxyaluminum hydride (LDBBA) as a new class of chemo-selective reducing agent and LDBBA was discovered to promote the reduction of ester compounds into the corresponding aldehydes without noticeable over-reduction of aldehydes into alcohols.19 To the best of our knowledge, no study has focused on the preparation of polymers featuring acrolein units via the partial reduction of common polyacrylates with LDBBA being the reducing agent.
All in all, in this study, we provide an insight into the post-polymerization modification reaction of polyacrylates with LDBBA, thereby affording the corresponding polymers featuring acrolein units (Scheme 1).
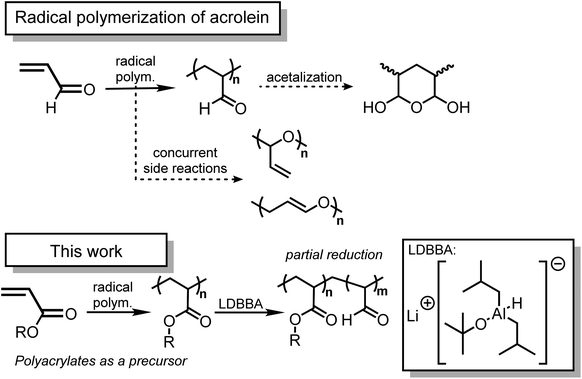 |
| Scheme 1 Schematic overview of this work. | |
Results and discussion
As discussed in the introduction, we design and validate a streamlined synthetic protocol for polymers featuring acrolein segments via the partial reduction of precursor polyacrylates with LDBBA being the reducing agent. To verify the process, the employed polymers and the corresponding product polymer featuring acrolein units need to be well-solubilized in a standard solvent. In particular, the product polymer might suffer from limited solubility in standard organic solvents because acrolein units are well-known to largely decrease the polymer solubility. Accordingly, we selected radically prepared poly[poly(ethylene glycol) monomethyl ether acrylate] (poly-mPEGA) as a precursor polymer because the poly(ethylene glycol) units are well-known to increase the solubility in most common solvents. Therefore, we performed the post-polymerization modification reaction of poly-mPEGA with LDBBA being the reducing agent. The LDBBA partial reduction of poly-mPEGA was carried out in THF at 0 °C according to the report by An and co-workers and the initial [mPEGA]0/[LDBBA]0 ratio was adjusted to be 1/2, where [mPEGA]0 refers to the concentration of the monomeric units. After the reaction was terminated, the reaction mixture became turbid, indicating that polymer solubility was decreased by the feasible construction of acrolein segments in the poly-mPEGA via the partial reduction. The [mPEGA]0/[LDBBA]0 ratio was reduced to 1/1 and 1/0.75, which still provided turbid products. Therefore, in order to secure the polymer solubility, the LDBBA partial reduction of poly-mPEGA was carried out with a [mPEGA]0/[LDBBA]0 ratio of 1/0.5. The progress of the partial reduction reaction was provided by the ATR-mode FT-IR measurements of the polymers before and after the partial reduction as depicted in Fig. S1 (ESI†). In Fig. S1,† the measured IR spectra of the polymers before and after the reduction are depicted, showing that C
O peaks were observed for both samples in the range of 1710–1750 cm−1. Unfortunately, the ester peak and the aldehyde one were severely overlapped and thus a precise discussion over the aldehyde development was very difficult. Therefore, the spectrum was deconvoluted to afford two series of peaks, namely a peak owing to the esters at 1740 cm−1 and one due to aldehyde at 1730 cm−1. In order to provide clear evidence of aldehyde generation, 1H NMR measurements were carried out. According to the NMR result of the obtained polymer depicted in Fig. 1, the post-polymerization partial reduction with LDBBA proceeded in the presence of excess LDBBA, and the ester conversion reached 9.1%, as evidenced by the obvious development of aldehyde protons at 9.3–9.7 ppm. Since the post-polymerization modification with LDBBA proceeded in the standard reaction manner, the acrolein monomer was thus expected to be randomly distributed along the polymer chains. In addition to the 1H NMR measurements, SEC traces of poly-mPEGA before and after the post-polymerization partial reduction of esters supported that the partial ester reduction is free from side reactions (Fig. 2). All in all, the combined results of FT-IR, 1H NMR, and SEC measurements showed that the target co-polymer poly(mPEGA-co-acrolein) was obtained via the feasible partial reduction of polyacrylates within the measurement sensitivity, thereby providing a facile synthetic approach that would allow the synthesis of polymers featuring acrolein segments without the use of acrolein and structural defects in the target polymer backbones. Of note here again, radical polymerization of acrolein usually involves concurrent side reactions and the obtained polymer structures are usually heterogeneous.
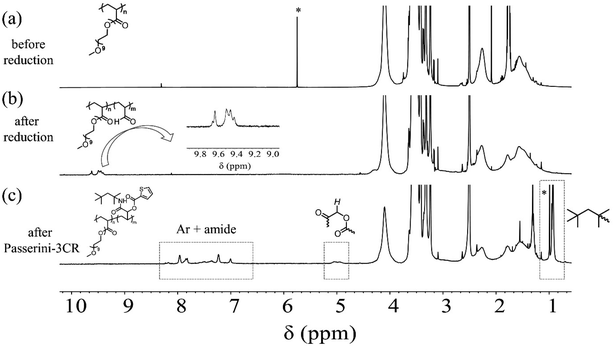 |
| Fig. 1
1H NMR spectra measured in DMSO-d6 of poly-mPEGA (a), the obtained polymer after the post-polymerization partial reduction with LDBBA (b), and the obtained polymer after the Passerini-3CR with 2-thiophenecarboxylic acid and 1,1,3,3-tetramethyl butyl isocyanide (c) (the symbol * refers to peaks owing to grease at 1.0 ppm and CH2Cl2 at 5.7 ppm). | |
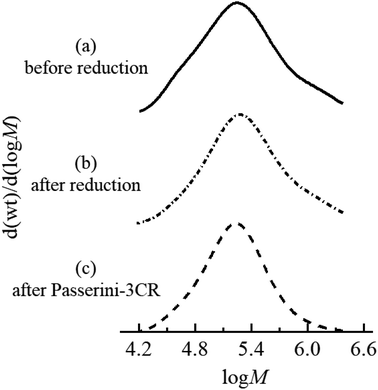 |
| Fig. 2 Size-exclusion chromatography traces of poly-mPEGA (a), the obtained polymer after the post-polymerization partial reduction with LDBBA (b), and the obtained polymer after the Passerini-3CR with 2-thiophenecarboxylic acid and 1,1,3,3-tetramethyl butyl isocyanide (c). | |
As demonstrated, the post-polymerization partial reduction with LDBBA proceeded to afford poly(mPEGA-co-acrolein). Though the aldehyde concentration could be further reduced by using less amount of LDBBA (∼3% aldehyde generation for the [mPEGA]0/[LDBBA]0 = 1/0.3), the above-obtained polymer was used in the following discussions due to the measurement simplicity. In order to showcase the synthetic benefits of the obtained copolymer, we have turned our attention to the recent developments in polymer chemistry based on multi-component reactions (MCRs) because most MCRs employ aldehydes as a reactant partner.23,24 For this, poly(mPEGA-co-acrolein) was subjected to Passerini three component reaction (Passerini-3CR) in CHCl3 in the presence of excess amounts of isocyanide and carboxylic acid.25,26 Specifically, we selected 2-thiophenecarboxylic acid and 1,1,3,3-tetramethyl butyl isocyanide as model reactants and set the following reaction conditions: 50 °C temperature, 8 h reaction time. According to the NMR result depicted in Fig. 1, the Passerini-3CR post-modification proceeded swiftly, and the aldehyde conversion reached practically quantitative aldehyde conversions. In the 1H NMR spectra of poly(mPEGA-co-acrolein) before and after the Passerini-3CR post-modification as depicted in Fig. 1, the presence of 1,1,3,3-tetramethyl butyl isocyanide and the 2-thiophenecarboxylic acid residue was evidenced by the appearance of a new alkyl peak at 1.0 ppm attributable to the former and a set of peaks at 5.8–6.2 ppm due to the latter, whereas the aldehyde peak at 9.3–9.7 ppm practically disappeared. These experimental data proved that the employed Passerini-3CR post-modification proceeded with >95% reaction conversion. To be precise, the integration ratio of the peaks at 1.0 ppm, that at 5.0 ppm, and those ranging from 7.0 to 8.0 ppm was calculated to be 10.4/1/4.3, providing a good agreement with the target structures within the measurement sensitivities. In addition to the 1H NMR measurements, SEC traces of poly(mPEGA-co-acrolein) before and after the Passerini-3CR post-modification supported that the Passerini post-modification is free from side reactions (Fig. 2). As demonstrated above, the LDBBA-based post-polymerization modification paved a facile way to polymers featuring acrolein units that showed high compatibility with Passerini-3CR. In order to demonstrate the synthetic benefits arising from the combination of LDBBA partial reduction and the subsequent Passerini-3CR post-polymerization modification reaction, a direct installation of vinyl compounds was designed because vinyl compounds are not compatible with radical polymerization due to the intrinsic reactivity of the carbon–carbon double bonds. For this, cinnamic acid was selected as the reaction partner for the Passerini-3CR due to the photo-responsive property arising from the [2 + 2] cycloaddition reaction.27–32 As such, poly(mPEGA-co-acrolein) was subjected to the Passerini-3CR with cinnamic acid and 1,1,3,3-tetramethyl butyl isocyanide, thereby affording polymers poly-mPEGA featuring cinnamate units in their pendants (poly-mPEGA-CA). The obtained poly-mPEGA-CA was thus subjected to photo-crosslinking based on the photo-induced [2 + 2] cycloaddition reaction of cinnamate carbon–carbon double bonds. For this, poly-mPEGA-CA was placed on a glass matrix and exposed to 50 mW cm−2 UV irradiation at 300–600 nm. Building on the polymer design, the long pendant oligoethyleneglycol segments provided the starting poly-mPEGA-CA with viscous nature and high solubility in common organic solvents. Upon the photo irradiation, the viscous starting poly-mPEGA-CA gradually transformed into the solid state and concurrently lost its solubility in organic solvents such as DMF and DMSO. The photo-induced cycloaddition reaction was monitored by the FT-IR measurements, where the peak at around 1640 cm−1 due to the vinyl group disappeared after the photo-irradiation process, thereby giving a good agreement with the mechanical changes of the polymer (Fig. 3).
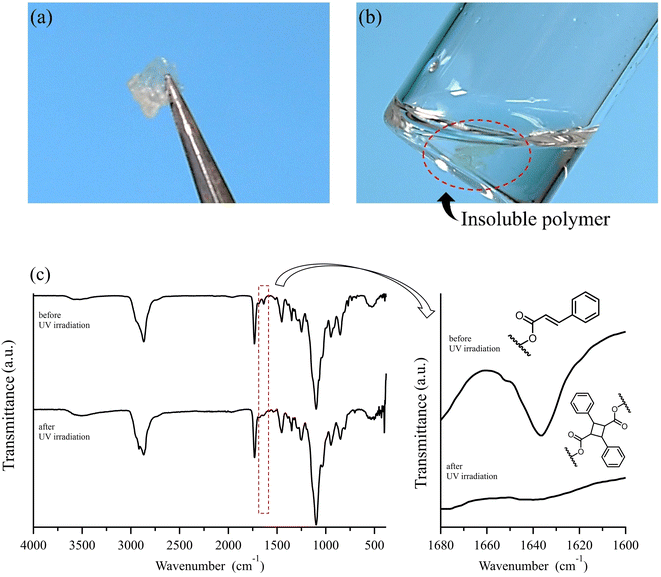 |
| Fig. 3 (a) Photographs of cross-linked poly-mPEGA-CA by UV irradiation; (b) dispersion in DMSO; (c) FT-IR spectra of polymers obtained by the Passerini-3CR of poly-mPEGA-CA before and after the photo-triggered cross-linking at 300–600 nm. | |
Conclusions
In this research, polyacrylates were found be a feasible precursor for polymers featuring acrolein segments via the chemo-selective partial reduction of esters mediated by LDBBA. In addition, polymers featuring acrolein units were feasibly subjected to the Passerini-3CR, thereby taking advantage of the aldehyde groups embedded in polymer structures. The thus generated aldehyde side groups were confirmed to be reactive sites, which was visually proved by emerging photo-crosslinked gels from the viscous polymer with cinnamoyl pendants attached to acrolein units. All in all, the present research paved a new synthetic pathway to polymers featuring acrolein units without the direct use of acrolein as a monomer.
Experimental
Materials
Lithium diisobutyl-tert-butoxyaluminum hydride solution (LDBBA, 0.25 M in THF/hexane) was purchased from Sigma-Aldrich Co. LLC. The mPEGA (polyethylene glycol monomethyl ether acrylate (repeating unit = ∼9)) was purchased from Tokyo Chemical Industry Co., Ltd. and passed through basic aluminum oxide to remove the inhibitors. AIBN was purchased from FUJIFILM Wako Pure Chemical Co., Ltd. and used after recrystallization using MeOH. trans-Cinnamic acid was purchased from Tokyo Chemical Industry Co. For the dialysis purifications, Spectra/Por 7 (MWCO 1000) was used as received. The starting poly(mPEGA) was prepared via standard free-radical polymerization. The procedure is briefly documented here. A 1,4-dioxane (2.1 mL) solution of mPEGA (3.0 g, 6.2 mmol) and AIBN (10.2 mg, 0.06 mmol) was degassed under an argon atmosphere for 30 min, and then stirred at 70 °C for 24 hours. After the reaction completion, the crude product was purified by dialysis in MeOH to afford the target polymer poly(mPEGA) as a viscous transparent product in 82.8% yield. Mn (SEC, DMF): 122 kg mol−1; Đ: 2.6. All other chemicals were commercially available and used without further purification unless otherwise stated.
Instruments
All NMR spectra in deuterated solvents were recorded on a Bruker 500 MHz FT-NMR spectrometer, and the chemical shifts (δ) are given in ppm with either the solvent peak or tetramethylsilane as the internal standard. The SEC measurements were performed at 40 °C and a flow rate of 1.0 mL min−1 in dimethylformamide (DMF) containing 10 mmol per L LiCl on a Waters gel permeation chromatography e2695 system embedded with two columns (7.8 × 300 mm Styragel HR 4E and 5E columns). The Mn and Đ values of the polymers were calculated with polystyrene calibration. The standard IR spectra were recorded using an Agilent Cary 630 spectrometer equipped with an ATR unit. The photoreaction of the obtained polymers was performed by UV irradiation at λ = 300–600 nm. The polymer samples which were cast on a glass plate were irradiated with a focused UV beam from a xenon lamp (MAX-303, Asahi Spector Co., Ltd.).
Syntheses
Typical procedure for the partial reduction reaction of poly(mPEGA).
To a dry THF solution (4.5 mL) of poly(mPEGA) (547.7 mg, 1.1 mmol), LDBBA solution (2.3 mL, 0.25 M in THF/hexane) was then added under an argon atmosphere at 0 °C. After 3 h, the reaction mixture was hydrolyzed with 4.5 mL of 1 N HCl (aq) and the product was extracted with dichloromethane. The obtained organic layer was dried over anhydrous magnesium sulfate. The crude product was purified by dialysis in acetone to afford the target polymer poly(mPEGA-co-CHO) as a viscous pale-yellow product.
Yield: 350 mg (64.0%); ester conversion (aldehyde generation ratio): 9.1 mol%; Mn (SEC, DMF): 141 kg mol−1; Đ: 2.5.
Typical procedure for the Passerini-3CR of poly(mPEGA-co-CHO).
Under an open condition, a CHCl3 (1.0 mL) solution of poly(mPEGA-co-CHO) (120 mg), 2-thiophenecarboxylic acid (32.0 mg, 0.25 mmol), and 1,1,3,3-tetramethyl butyl isocyanide (44.0 μL, 0.25 mmol) was stirred at 50 °C for 8 hours. After 8 h, the solution was purified by dialysis in acetone to afford the target as a viscous pale-yellow product.
Yield: 102 mg (84.8%); Mn (SEC, DMF): 121 kg mol−1; Đ: 1.9.
Typical procedure for the photo-induced cross-linking of poly-mPEGA-CA.
Poly-mPEGA-CA was directly cast onto a glass plate and irradiated with UV light for 24 hours from upside. The thickness of the resulting film was approximately 250 μm. For photo-reactions, photo-irradiation was performed with a focused UV beam from a xenon lamp (MAX-303, Asahi Spector Co., Ltd.; distance from sample, 5 cm; illuminance at 350 nm, 50 mW cm−2).
Author contributions
The manuscript was written through contributions of all authors. All authors have given approval to the final version of the manuscript.
Conflicts of interest
There are no conflicts to declare.
Acknowledgements
R. K. gratefully acknowledges the Leading Initiative for Excellent Young Researchers (LEADER) and Grant-in-Aid for Scientific Research (C) (Grant number: 19K05578) for financial support. R. K. also acknowledges the S-Membrane Project and the F-Materials Project at Gunma University for the financial support.
References
- C. Barner-Kowollik, F. E. Du Prez, P. Espeel, C. J. Hawker, T. Junkers, H. Schlaad and W. Van Camp, “Clicking” Polymers or Just Efficient Linking: What Is the Difference?, Angew. Chem., Int. Ed., 2011, 50(1), 60–62, DOI:10.1002/anie.201003707
.
- C. R. Becer, R. Hoogenboom and U. S. Schubert, Click Chemistry beyond Metal-Catalyzed Cycloaddition, Angew. Chem., Int. Ed., 2009, 48(27), 4900–4908, DOI:10.1002/anie.200900755
.
- R. K. Iha, K. L. Wooley, A. M. Nyström, D. J. Burke, M. J. Kade and C. J. Hawker, Applications of Orthogonal Click Chemistries in the Synthesis of Functional Soft Materials, Chem. Rev., 2009, 109(11), 5620–5686, DOI:10.1021/cr900138t
.
- D. Fournier, R. Hoogenboom and U. S. Schubert, Clicking polymers: a straightforward approach to novel macromolecular architectures, Chem. Soc. Rev., 2007, 36(8), 1369–1380 RSC
.
- P. L. Golas and K. Matyjaszewski, Marrying click chemistry with polymerization: expanding the scope of polymeric materials, Chem. Soc. Rev., 2010, 39(4), 1338–1354 RSC
.
- C. E. Hoyle, A. B. Lowe and C. N. Bowman, Thiol-click chemistry: a multifaceted toolbox for small molecule and polymer synthesis, Chem. Soc. Rev., 2010, 39(4), 1355–1387 RSC
.
- M. d. F. V. Marques, P. R. Pinto, C. T. de Andrade and R. C. Michel, Synthesis and characterization of low-molecular-weight polyacrolein, J. Appl. Polym. Sci., 2009, 112(3), 1771–1779, DOI:10.1002/app.29533
.
- Z.-P. Xiao, K.-M. Yang, H. Liang and J. Lu, Synthesis of magnetic, reactive, and thermoresponsive Fe3O4nanoparticles via surface-initiated RAFT copolymerization ofN-isopropylacrylamide and acrolein, J. Polym. Sci., Part A: Polym. Chem., 2010, 48(3), 542–550, DOI:10.1002/pola.23752
.
- Y. Li, T. Li, J. Tang, Y. Liu, P. Huang and Y. F. Zhang, The Synthesis and Characterization of Polyacrolein through Radical Polymerization, Macromol. React. Eng., 2020, 15(1), 2000046 CrossRef
.
-
O. Nuyken, Poly(vinyl aldehyde)s, Poly(vinyl ketone)s, and Phosphorus-Containing Vinyl Polymers, in Handbook of Polymer Synthesis, ed. H. R. Kricheldorf, O. Nuyken and G. Swift, CRC Press, 2004 Search PubMed
.
- M. B. Larsen, S. J. Wang and M. A. Hillmyer, Poly(allyl alcohol) Homo- and Block Polymers by Postpolymerization Reduction of an Activated Polyacrylamide, J. Am. Chem. Soc., 2018, 140(38), 11911–11915, DOI:10.1021/jacs.8b07542
.
- W. Zhang, M. Huang, S. a. Abdullatif, M. Chen, Y. Shao-Horn and J. A. Johnson, Reduction of (Meth)acrylate-Based Block Copolymers Provides Access to Self-Assembled Materials with Ultrasmall Domains, Macromolecules, 2018, 51(17), 6757–6763, DOI:10.1021/acs.macromol.8b01588
.
- S. Frech and P. Theato, Synthesizing Polyethylene from Polyacrylates: A Decarboxylation Approach, ACS Macro Lett., 2022, 11(2), 161–165, DOI:10.1021/acsmacrolett.1c00723
.
- C. Jeon, D. W. Kim, S. Chang, J. G. Kim and M. Seo, Synthesis of Polypropylene via Catalytic Deoxygenation of Poly(methyl acrylate), ACS Macro Lett., 2019, 8(9), 1172–1178, DOI:10.1021/acsmacrolett.9b00522
.
- J. B. Garrison, R. W. Hughes, J. B. Young and B. S. Sumerlin, Photoinduced SET to access olefin-acrylate copolymers, Polym. Chem., 2022, 13(7), 982–988, 10.1039/D1PY01643A
.
- J. S. An, W. K. Shin and D. K. An, Quantitative Synthesis of Aldehydes from Weinreb Amides Using Lithium Diisobutyl-t-Butoxyaluminum Hydride (LDBBA), Bull. Korean Chem. Soc., 2015, 36(12), 2928–2931, DOI:10.1002/bkcs.10571
.
- S.-J. Choi, K.-J. Lee, G.-B. Lee and D. K. An, Reducing Characteristics of Metal Diisobutyl-t-butoxyaluminum Hydrides for Tertiary Amides, Bull. Korean Chem. Soc., 2008, 29(7), 1407–1408, DOI:10.5012/BKCS.2008.29.7.1407
.
- J. Y. Kim, W. K. Shin, A. K. Jaladi and D. K. An, Chemoselective reduction of aldehydes and ketones by potassium diisobutyl-t-butoxy aluminum hydride (PDBBA), Tetrahedron, 2018, 74(31), 4236–4241, DOI:10.1016/j.tet.2018.06.048
.
- M. S. Kim, Y. M. Choi and D. K. An, Lithium diisobutyl-t-butoxyaluminum hydride, a new and efficient reducing agent for the conversion of esters to aldehydes, Tetrahedron Lett., 2007, 48(29), 5061–5064, DOI:10.1016/j.tetlet.2007.05.091
.
- G. Moad, E. Rizzardo and S. H. Thang, Living Radical Polymerization by the RAFT Process, Aust. J. Chem., 2005, 58(6), 379–410, DOI:10.1071/CH05072
.
- R. B. Grubbs and R. H. Grubbs, 50th Anniversary Perspective: Living Polymerization—Emphasizing the Molecule in Macromolecules, Macromolecules, 2017, 50(18), 6979–6997, DOI:10.1021/acs.macromol.7b01440
.
- M. Ouchi and M. Sawamoto, 50th Anniversary Perspective: Metal-Catalyzed Living Radical Polymerization: Discovery and Perspective, Macromolecules, 2017, 50(7), 2603–2614, DOI:10.1021/acs.macromol.6b02711
.
- R. Kakuchi, Multicomponent Reactions in Polymer Synthesis, Angew. Chem., Int. Ed., 2014, 53(1), 46–48, DOI:10.1002/anie.201305538
.
- R. Kakuchi, The Dawn of Polymer Chemistry Based on Multicomponent Reactions, Polym. J., 2019, 51(10), 945–953, DOI:10.1038/s41428-019-0209-0
.
- R. Kakuchi, R. Tsuji, K. Fukasawa, S. Yamashita, M. Omichi and N. Seko, Polymers of lignin-sourced components as a facile chemical integrant for the Passerini three-component reaction, Polym. J., 2021, 53(4), 523–531, DOI:10.1038/s41428-020-00448-w
.
- R. Kakuchi and Y. Okura, The Passerini three-component reaction of aldehyde end-functionalized polymers via RAFT polymerization using chain transfer agents featuring aldehyde, Polym. J., 2020, 52(9), 1057–1066, DOI:10.1038/s41428-020-0368-z
.
- N. Kawatsuki, R. Tsutsumi, A. Hiraiwa, H. Takatsuka and T. Sakai, Thermally enhanced photoinduced in-plane reorientation in photo-cross-linkable polymer liquid crystalline films and its application to linear polarizer, J. Polym. Sci., Part A: Polym. Chem., 2008, 46(14), 4712–4718, DOI:10.1002/pola.22803
.
- N. Kawatsuki, T. Kawanishi and E. Uchida, Photoinduced Cooperative Reorientation in Photoreactive Hydrogen-Bonded Copolymer Films and LC Alignment Using the Resultant Films, Macromolecules, 2008, 41(13), 4642–4650, DOI:10.1021/ma800505c
.
- E. Uchida and N. Kawatsuki, Photoinduced Orientation in Photoreactive Hydrogen-Bonding Liquid Crystalline Polymers and Liquid Crystal Alignment on the Resultant Films, Macromolecules, 2006, 39(26), 9357–9364, DOI:10.1021/ma061381p
.
- A. Lendlein, H. Jiang, O. Jünger and R. Langer, Light-induced shape-memory polymers, Nature, 2005, 434(7035), 879–882, DOI:10.1038/nature03496
.
- N. Kawatsuki and Y. Koezuka, Influence of alkylene spacer length on photoinduced molecular reorientation and LC alignment behavior in photo-cross-linkable liquid crystalline polymeric films with H-bonded cinnamic acid side groups, Polymer, 2009, 50(11), 2349–2356, DOI:10.1016/j.polymer.2009.03.033
.
- N. Kawatsuki, A. Yamashita, M. Kondo, T. Matsumoto, T. Shioda, A. Emoto and H. Ono, Photoinduced reorientation and polarization holography in photo-cross-linkable liquid crystalline polymer films with large birefringence, Polymer, 2010, 51(13), 2849–2856, DOI:10.1016/j.polymer.2010.04.043
.
Footnote |
† Electronic supplementary information (ESI) available: ATR-mode FT-IR spectra of polymers, 1H NMR spectrum and SEC chart of the poly-mPEGA-CA. See DOI: https://doi.org/10.1039/d2su00143h |
|
This journal is © The Royal Society of Chemistry 2023 |