DOI:
10.1039/D3SC04457B
(Edge Article)
Chem. Sci., 2023,
14, 13060-13066
Photochemical α-selective radical ring-opening reactions of 1,3-disubstituted acyl bicyclobutanes with alkyl halides: modular access to functionalized cyclobutenes†
Received
24th August 2023
, Accepted 25th October 2023
First published on 27th October 2023
Abstract
Although ring-opening reactions of bicyclobutanes bearing electron-withdrawing groups, typically with β-selectivity, have evolved as a powerful platform for synthesis of cyclobutanes, their application in the synthesis of cyclobutenes remains underdeveloped. Here, a novel visible light induced α-selective radical ring-opening reaction of 1,3-disubstituted acyl bicyclobutanes with alkyl radical precursors for the synthesis of functionalized cyclobutenes is described. In particular, primary, secondary, and tertiary alkyl halides are all suitable substrates for this photocatalytic transformation, providing ready access to cyclobutenes with a single all-carbon quaternary center, or with two contiguous centers under mild reaction conditions.
Introduction
Four-membered rings such as cyclobutanes and cyclobutenes are important frameworks found in an array of natural products and pharmaceuticals with diverse biological and medicinal properties (Scheme 1a).1 Meanwhile, they also serve as key intermediates in the synthesis of structurally complex targets.2 Therefore, the development of new methods for their efficient synthesis has been intensively pursued by the synthetic community. Despite the significant advances made in the area for the synthesis of four-membered rings, modular strategies for the synthesis of these structures remain relatively few in number, particularly in comparison to the available methodologies to synthesize five- and six-membered rings.3 Specifically, synthesis of multisubstituted functionalized cyclobutanes and cyclobutenes featuring quaternary carbon stereocenters remains challenging.4
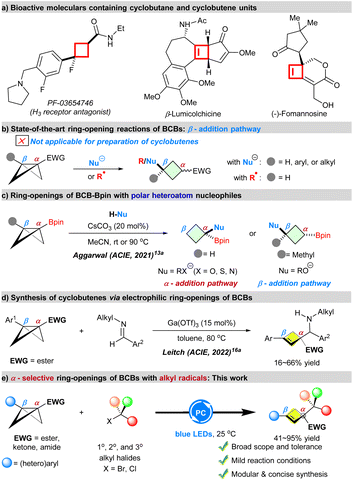 |
| Scheme 1 Ring-opening reactions of BCBs for synthesis of four-membered rings and their scientific context. | |
As the smallest of bicyclic hydrocarbons, bicyclo[1.1.0]butanes (BCBs) are ‘spring-loaded’ yet bench-stable molecules.5 The potential energy stored in the bridging bond (66.3 kcal mol−1 (calculated))6 has been harnessed for applications in the design or discovery of new reactions,7 materials science,8 medicinal chemistry9 and analytical chemistry.10 Among them, BCBs carrying an electron withdrawing group at one end of the bridging bond are often used as substrates in ring-opening reactions with polar carbon11 and heteroatom-based nucleophiles.12 In most cases, these reactions occur exclusively at the β-position of the bridging bond, leading to the synthesis of multisubstituted cyclobutanes, as shown in the elegant studies by Gaoni,11a,12a Fox,11b,c Baran,12c,d Wipf,12e Aggarwal,13 Malins,12f Biju,11e and others (Scheme 1b).11,12 Besides these, BCBs have been used as a C4 building block for the synthesis of cyclobutanes via β-selective ring-opening reactions with radicals.14 For example, Ernouf and Cintrat presented the application of photoredox-generated nucleophilic secondary (2°) alkyl radicals in reaction with BCBs in 2020. A computational study was performed to compare BCBs with commonly utilized Michael acceptors for the radical addition process, which indicated that the radical addition to the BCB is less favored than the corresponding olefin.14a Subsequently, the cyclobutylation reactions of primary (1°) alkyl, tertiary (3°) alkyl and silyl radical precursors with monosubstituted BCBs via strain release have been described by Jui,14b Lin14c & Xia,14d and Studer14e respectively. Furthermore, the Aggarwal group demonstrated that in situ generated BCB boronate complexes can be efficiently attacked by electrophilic trifluoromethyl and α-carbonyl radicals which could be generated by photolysis of C–I bonds.14f Of note, a mechanistically distinct homolytic addition reaction of BCBs via light-driven cobalt catalysis was demonstrated by Gryko and co-workers.15 Despite significant progress, the heterolytic- and homolytic strain-release cyclobutylation reaction continues to face several challenges such as (a) previous reports on the radical ring-openings of BCBs were limited to monosubstituted BCBs. Again, these reactions occur exclusively at the β-position of the bridging bond of BCBs (Scheme 1b);14 (b) although α-selective ring-opening reactions of monosubstituted bicyclo[1.1.0]butyl boronic ester (BCB-Bpin) with polar heteroatom nucleophiles were reported by Aggarwal and co-workers in 2021, a β-addition pathway was observed when β-methyl-substituted BCB-Bpin was used as the substrate (Scheme 1c).13a To the best of our knowledge, there is no report on the α-selective ring-opening reactions of BCBs with radical nucleophiles. (c) So far, most of the transformations have focused on the conjugate addition-type reactivity of BCBs for synthesis of cyclobutanes,11–14 while less work has been reported on the selective construction of cyclobutenes from BCBs via ring-opening reactions.16 As a rare example, the Leitch group reported Lewis acid catalyzed polar electrophilic ring-opening reactions of BCBs with N-alkylimines to generate cyclobutenyl methanamine products with poor to moderate yields (Scheme 1d).16a Therefore, discovery of new catalytic systems for efficient synthesis of valuable cyclobutene products is highly desirable.
As part of our ongoing interest in small ring chemistry17 and in order to expand the library of known BCB reactivity, we became interested in whether 1,3-disubstituted BCBs could be employed as radical acceptors in radical ring-opening reactions. Along this line, other intriguing questions arise: what type of radical substrate is suitable for the reaction? How to control the regioselectivity? Can this reaction lead to cyclobutenes? Here, we describe a modular strategy for the synthesis of functionalized cyclobutenes via α-selective ring-opening reactions of acyl BCBs with alkyl radicals, which could be generated from the commercially available 1°, 2°, and 3° alkyl bromides by photolysis of C–Br and C–Cl bonds (Scheme 1e).18
Results and discussion
Initial experiments on a representative α-bromo ketone 2a18a and 1,3-disubstituted BCB ester1a under the irradiation of blue LEDs revealed that the commonly used metal-based photocatalysts (PCs) (Ru(bpy)3Cl2, Ru(bpy)3(PF6)2, and Ir[dF(CF3)ppy]2[dtbbpy]PF6) and organic-based PCs (Eosin Y, [Acr+-Mes]ClO4, and 4-CzlPN) were lowly effective, affording the desired product 3aa with ≤7% NMR yield (see Table S1, in the ESI†). To our delight, fac-Ir(ppy)3 gave a moderate yield of the desired cyclobutene 3aa along with a 5% yield of 4a and cyclobutane 5aa (12% yield) in MeCN (Table 1, entry 1). Importantly, no aimed product 3aa was observed only with either the fac-Ir(ppy)3 catalyst or light, which indicated that photoredox catalysis is essential to this ring-opening process (entries 2 and 6). Subsequent investigations demonstrated the importance of solvent (entries 3–5). The use of DMF as the solvent dramatically improved the yield of 3aa from 30% to 58% (entry 5 versus 1). The base also had a substantial effect on the product distribution but no improvement over K2HPO4 was seen (entries 7–10). Further improvement of the yield was achieved with dimethylacetamide (DMA) instead of DMF as the solvent (entry 11). Notably, the ring-opening reaction can tolerate H2O (entry 12). Only a 3-h reaction time was needed to reach >99% conversion (entry 13). In the absence of a base, the reaction yield was reduced from 71 to 31% (entry 14). Rather than alkyl bromide 2a, α-chloroacetophenone was also competent to afford the product, albeit with a lower yield (entry 15).
Table 1 Optimization of reaction conditionsa
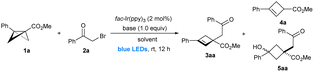
|
Entry |
Base |
Solvent |
Yieldb [%] |
3aa
|
4a
|
5aa
|
Conditions: 1a (0.2 mmol), 2a (0.3 mmol), fac-Ir(ppy)3 (2 mol%) and base (1.0 equiv.) in solvent (2.0 mL) at 25 °C under the irradiation of 12 W blue LEDs for 12 h.
NMR yield with CH2Br2 as an internal standard. Isolated yields are indicated in parentheses.
Without fac-Ir(ppy)3.
Reaction in the absence of light irradiation.
H2O (0.5 equiv.) was added.
Reaction time: 3 h.
Without base.
α-Chloroacetophenone instead of 2a.
|
1 |
K3PO4 |
MeCN |
30 |
5 |
12 |
2c |
K3PO4 |
MeCN |
0 |
5 |
0 |
3 |
K3PO4 |
THF |
43 |
9 |
6 |
4 |
K3PO4 |
DCE |
0 |
36 |
<5 |
5 |
K3PO4 |
DMF |
58 |
<5 |
6 |
6d |
K3PO4 |
DMF |
0 |
<5 |
0 |
7 |
KH2PO4 |
DMF |
30 |
0 |
5 |
8 |
K2HPO4 |
DMF |
62 |
<5 |
11 |
9 |
Na2CO3 |
DMF |
35 |
<5 |
<5 |
10 |
Et3N |
DMF |
11 |
5 |
0 |
11 |
K2HPO4 |
DMA |
71 |
<5 |
5 |
12e |
K2HPO4 |
DMA |
71 |
<5 |
7 |
13f |
K2HPO4 |
DMA |
71(67) |
<5 |
6 |
14f |
—g |
DMA |
31 |
19 |
11 |
15h |
K2HPO4 |
DMA |
55 |
<5 |
0 |
With optimized reaction conditions established, we tested a range of different alkyl bromides (Scheme 2A). The reaction of bromoacetophenones with different substituents on the aryl ring, including electron-donating (4-OMe as in 3ab) and electron-withdrawing groups (4-CF3 as in 3ae; 4-CN as in 3af), at either the para, meta, or ortho position, also proceeded with good efficiency (3aa–3ai). The naphthyl-containing α-bromo ketone 2j furnished 3aj in 91% yield. Additionally, heterocyclic α-bromo ketone 2k underwent the reaction smoothly to deliver the products in moderate yield (3ak). Aside from aromatic ketones, alkyl ketone 2l containing a cyclopropyl group, can be smoothly transformed into the corresponding product 3al. α-Carbonyl alkyl bromides bearing cyano (2m),19 amide (2n) and ester (2o) groups are also suitable substrates. Besides primary alkyl bromides, secondary alkyl bromides are still maintaining decent yields (3ap–3aq). Photoredox catalysis has recently demonstrated potential for the construction of congested all-carbon quaternary centers via highly reactive radicals.18 However, intermolecular construction of two contiguous all-carbon quaternary centers by visible light-induced photoredox catalysis remains underdeveloped.18p To our delight, tertiary-α-bromoalkyl esters (2r–2t) successfully afforded the corresponding congested cyclobutenes (3ar–3at) in 49–95% yields with exclusive α-selectivity. Importantly, ethyl bromodifluoroacetate also had high reactivity and delivered product 3au bearing a gem-difluoromethylene (CF2) group, which is popular in drugs and in agrochemicals. Furthermore, tertiary-alkyl bromide 2v with a nitro group smoothly reacted with 1a and gave the product 3av in reasonable yield. Besides α-carbonyl alkyl bromides, less-reactive benzyl bromide derivative 2w was also a suitable substrate in the presence of fac-Ir(ppy)3 as the photocatalyst under reoptimized condition B.
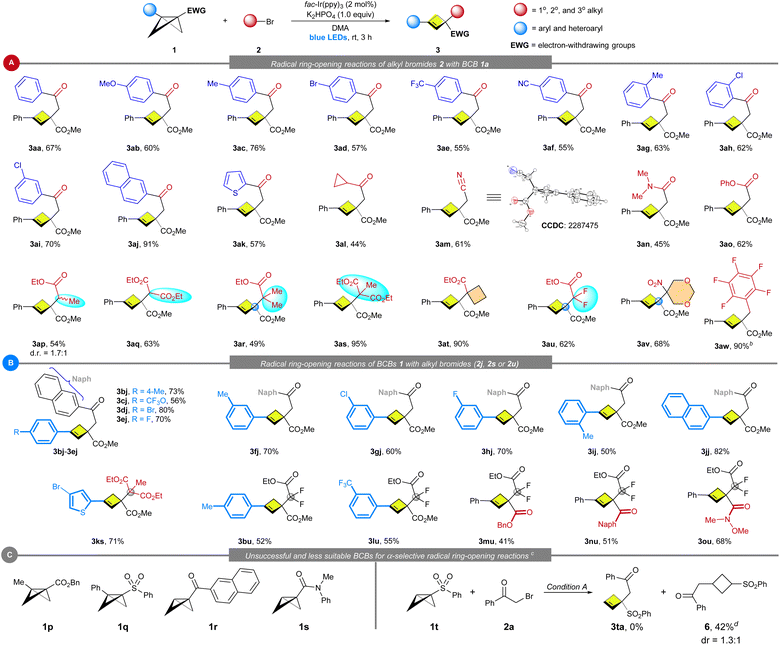 |
| Scheme 2 Substrate scope investigation.a a Unless otherwise noted, reactions were performed with 1 (0.2 mmol), 2 (0.30 mmol), fac-Ir(ppy)3 (2 mol%), K2HPO4 (1.0 equiv.), and DMA (2.0 mL), at room temperature under irradiation with 12 W blue LEDs for 3 h (condition A). Isolated yields are indicated. b Condition B: 1 (0.2 mmol)), 2 (0.30 mmol), fac-Ir(ppy)3 (2 mol%), Na2CO3 (1.0 equiv.), Et3N (0.1 equiv.), and DMF (2.0 mL), at room temperature under irradiation with 12 W blue LEDs for 12 h. c The ring-opening reactions of BCBs (1p–1t) with α-bromo ketone 2a were conducted using condition A. d Combined isolated yield of the diastereomers. | |
The scope and generality of this α-selective ring-opening in terms of 1,3-disubstituted BCBs with representative alkyl bromides (2j, 2s and 2u) is summarized in Scheme 2B. This method is amenable to a series of BCBs bearing different aromatic substituents, including alkyl (1b, 1f & 1i), CF3O (1c), halogen (1d, 1e, 1g & 1h) and CF3 (1l) groups at the para-, meta- or ortho-position of aryl rings, and led to the corresponding cyclobutenes in moderate to excellent yields (50–80%). Both naphthyl (1j) and thienyl (1k) substituted BCBs reacted smoothly under condition A. Apart from BCB esters (1b–1m), both BCB ketone 1n and Weinreb amide derived BCB 1o were compatible, giving the corresponding ring-opening products bearing two contiguous quaternary centers and CF2 groups in synthetically useful yield (1n + 2u → 3nu; 1o + 2u → 3ou). The reaction is not limited to aryl-substituted BCBs. Alkyl-substituted BCB 1p was also found to be compatible, albeit with a low yield (5% NMR yield) under conditions A (for more details, see ESI Scheme S1†). 1,3-Disubstituted sulfonyl BCB 1q did not furnish the desired cyclobutene product. Additionally, we investigated the influence of the substituent at the β-position of BCB in this site-selective reaction. Although the ring-opening reaction of monosubstituted BCB ketone 1r (or BCB amide 1s) results in a complex mixture, the reaction of monosubstituted BCB sulfone 1t with 2a produces the cyclobutane product 6 through β-addition (Scheme 2C).
To explore synthetic transformations of these functionalized cyclobutenes, a scale-up synthesis of 3aj (1.0 mmol) was performed without any loss in efficiency and selectivity (Scheme 3). The double bond in 3aj was hydrogenated over Pd/C to produce trisubstituted cyclobutane 7 in 90% yield. The ketone group in 3aj was converted into an alkene in 8. Hydrolysis of the ester group of 3aj afforded the free carboxylic acid 9. Moreover, reduction of the ester group in 3am using NaBH4 provided the primary alcohol 10 in 86% yield. Also, functionalized cyclobutene 11 was synthesized from 10 by hydrolysis of the nitrile under basic conditions. Of note, reduction of the Weinreb amide 3ou with LiAlH4 followed by oxidation allowed for the construction of spirolactone 12 containing a 4-membered ring.
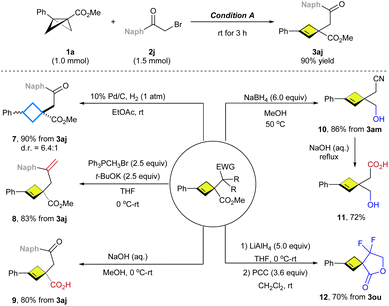 |
| Scheme 3 Scale-up synthesis and synthetic transformations. | |
To rationalize the reaction pathway, some experiments were designed and performed. First, the reaction was completely shut down in the presence of TEMPO. In the meantime, the radical trapping product 13 was obtained in 82% yield (Scheme 4A). Second, the reaction of 2-bromoacetonitrile with 1a and 4-methoxystyrene was attempted under condition A, and the cyclobutene 3am together with 14 and 15 was detected by 1H NMR and HRMS, indicating that the reaction proceeds via a radical pathway with α-selectivity (Scheme 4B). Subsequently, the treatment of 4a with 2a under the standard reaction conditions did not furnish the aimed 3aa (Scheme 4C). This result demonstrated that the reaction was not proceeding via the cyclobutene intermediate 4a. In addition, as shown in Scheme 4D, when 5aa was treated with the photocatalyst under optimized conditions, no desired product was observed.
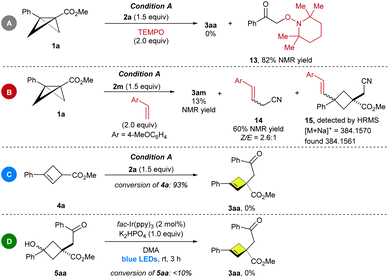 |
| Scheme 4 Control experiments. | |
Moreover, the light–dark experiment was performed using alternative intervals of light and dark (Scheme 5). It has been demonstrated that this photochemical transformation necessitates continuous exposure to visible light, indicating that chain propagation may not be the primary reaction pathway. A Stern–Volmer plot showed strong quenching of fac-Ir(ppy)3 (EIV/III* = −1.84 V vs. SCE) by alkyl bromide 2a (Eox > 1.6 V vs. SCE, Ered = −1.2 V vs. SCE),18q favoring an oxidative quenching cycle (Scheme 6 and Fig. S2–S4, in the ESI†).
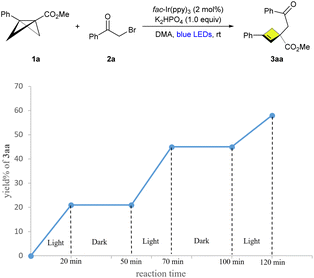 |
| Scheme 5 Light on/off experiment. | |
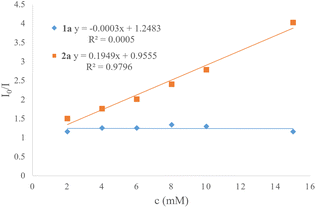 |
| Scheme 6 Stern–Volmer plots of fac-Ir(ppy)3 with different quenchers (1a or 2a). | |
Based on these preliminary results, a reasonable mechanistic rationale for our photoredox transformation was formulated, as shown in Scheme 7. Upon photoexcitation, the photocatalyst fac-Ir(ppy)3 is converted into the strongly reducing excited state Ir(III)*, which is capable of reducing an alkyl halide 2 to its corresponding alkyl radical INT-A and generating Ir(IV). Upon α-selective radical addition of the electrophilic species INT-A to BCB 2, the key C–C bond formation step occurred, thus forming the more stable tertiary radical INT-B. Then, INT-B undergoes a single-electron oxidation with Ir(IV) to form the carbocation INT-C with the regeneration of the photocatalyst. Finally, the cyclobutene 3 can be formed through an elimination reaction with the assistance of a base.
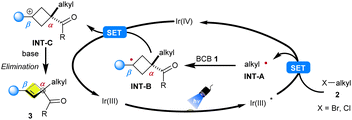 |
| Scheme 7 Proposed mechanism. | |
Conclusion
In summary, we report the first use of ring-opening reactions of 1,3-disubstituted bicyclobutanes with alkyl radical precursors to construct cyclobutenes via visible light-induced photoredox catalysis. This study provides a concise and modular method for the synthesis of highly valuable alkylated cyclobutenes. A broad range of alkyl halides including 1°, 2°, and 3° alkyl bromides can be coupled under mild reaction conditions to afford 1,1,3-trisubstituted cyclobutenes with a single all-carbon quaternary center, or with two contiguous (all-carbon quaternary) centers. The synthetic utility of the reaction was showcased by several transformations. Notably, unlike the β-selectivity disclosed in classical polar and radical strain-release reactions of BCBs, an exclusive α-selectivity in current radical ring-openings of acyl-BCBs with carbon-centered radicals is obtained, which is complementary to Aggarwal's α-selective ring-opening reactions of monosubstituted BCB-Bpin with heteroatom-centred polar nucleophiles.13a
Data availability
All detailed procedures, characterization data and NMR spectra are available in the ESI.†
Author contributions
Y. X., T.-T. X., J.-L. Z., F. W., L. T., and R.-Y. L. performed the experiments and conducted the analytical characterization. W.-B. W. and J.-J. F. wrote the manuscript. J.-J. F. conceived the catalytic system.
Conflicts of interest
There are no conflicts to declare.
Acknowledgements
We are grateful to the Fundamental Research Funds for the Central Universities.
References
-
(a) C. Hui, Y. Liu, M. Jiang and P. Wu, Trends Chem., 2022, 4, 677–681 CrossRef CAS;
(b) M. R. van der Kolk, M. A. C. H. Janssen, F. P. J. T. Rutjes and D. Blanco-Ania, ChemMedChem, 2022, 17, e202200020 CrossRef CAS PubMed;
(c) V. M. Dembitsky, J. Nat. Med., 2008, 62, 1–33 CAS.
- For reviews, see:
(a) J. Chen, Q. Zhu, H. Fang and P. Lu, Chin. J. Chem., 2022, 40, 1346–1358 CrossRef CAS;
(b) J. Li, K. Gao, M. Bian and H. Ding, Org. Chem. Front., 2020, 7, 136–154 RSC;
(c) M. Wang and P. Lu, Org. Chem. Front., 2018, 5, 254–259 RSC;
(d) T. Seiser, T. Saget, D. N. Tran and N. Cramer, Angew. Chem., Int. Ed., 2011, 50, 7740–7752 CrossRef CAS PubMed;
(e) E. Lee-Ruff and G. Mladenova, Chem. Rev., 2003, 103, 1449–1483 CrossRef CAS PubMed;
(f) J. C. Namyslo and D. E. Kaufmann, Chem. Rev., 2003, 103, 1485–1537 CrossRef CAS PubMed;
(g) R. Guo and M. K. Brown, Acc. Chem. Res., 2023, 56, 2253–2264 CrossRef CAS PubMed.
-
(a) D. Didier and F. Reiners, Chem. Rec., 2021, 21, 1144–1160 CrossRef CAS PubMed;
(b) Y. Xu, M. L. Conner and M. K. Brown, Angew. Chem., Int. Ed., 2015, 54, 11918–11928 CrossRef CAS PubMed;
(c) N. Gauvry, C. Lescop and F. Huet, Eur. J. Org Chem., 2006, 5207–5218 CrossRef CAS.
- For reviews, see:
(a) K.-G. Wen, Y.-Y. Peng and X.-P. Zeng, Org. Chem. Front., 2020, 7, 2576–2597 RSC;
(b) E. N. Hancock, J. M. Wahl and M. K. Brown, Nat. Prod. Rep., 2019, 36, 1383–1393 RSC ; For representative examples on synthesis of quaternary carbon containing cyclobutenes, see:;
(c) H. Xu, W. Zhang, D. Shu, J. B. Werness and W. Tang, Angew. Chem., Int. Ed., 2008, 47, 8933–8936 CrossRef CAS PubMed;
(d) A. Masarwa, A. Fürstner and I. Marek, Chem. Commun., 2009, 5760–5762 RSC;
(e) Y. Odabachian and F. Gagosz, Adv. Synth. Catal., 2009, 351, 379–386 CrossRef CAS;
(f) V. López-Carillo and A. M. Echavarren, J. Am. Chem. Soc., 2010, 132, 9292–9294 CrossRef PubMed;
(g) F. Frébault, M. Luparia, M. T. Oliveira, R. Goddard and N. Maulide, Angew. Chem., Int. Ed., 2010, 49, 5672–5676 CrossRef PubMed;
(h) C. Schotes and A. Mezzetti, Angew. Chem., Int. Ed., 2011, 50, 3072–3074 CrossRef CAS PubMed;
(i) S. Niyomchon, D. Audisio, M. Lupariaa and N. Maulide, Org. Lett., 2013, 15, 2318–2321 CrossRef CAS PubMed;
(j) M. M. Maturi and T. Bach, Angew. Chem., Int. Ed., 2014, 53, 7661–7664 CrossRef CAS PubMed;
(k) K. Enomoto, H. Oyama and M. Nakada, Chem.–Eur. J., 2015, 21, 2798–2802 CrossRef CAS PubMed;
(l) T. Kang, S. Ge, L. Lin, Y. Lu, X. Liu and X. Feng, Angew. Chem., Int. Ed., 2016, 55, 5541–5544 CrossRef CAS PubMed;
(m) C. García-Morales, B. Ranieri, I. Escofet, L. López-Suarez, C. Obradors, A. I. Konovalov and A. M. Echavarren, J. Am. Chem. Soc., 2017, 139, 13628–13631 CrossRef PubMed;
(n) F.-G. Zhang and I. Marek, J. Am. Chem. Soc., 2017, 139, 8364–8370 CrossRef CAS PubMed;
(o) E. N. Hancock, E. L. Kuker, D. Tantillo and M. K. Brown, Angew. Chem., Int. Ed., 2020, 59, 436–441 CrossRef CAS PubMed;
(p) C. Zhong, Y. Huang, H. Zhang, Q. Zhou, Y. Liu and P. Lu, Angew. Chem., Int. Ed., 2020, 59, 2750–2754 CrossRef CAS PubMed;
(q) F. W. Goetzke, A. M. L. Hell, L. van Dijk and S. P. Fletcher, Nat. Chem., 2021, 13, 880–886 CrossRef CAS PubMed;
(r) M. Yan, Q. Zhou and P. Lu, Angew. Chem., Int. Ed., 2023, 62, e202218008 CrossRef CAS PubMed.
-
(a) M. Golfmann and J. C. L. Walker, Commun. Chem., 2023, 6, 9 CrossRef CAS PubMed;
(b) C. B. Kelly, J. A. Milligan, L. J. Tilley and T. M. Sodano, Chem. Sci., 2022, 13, 11721–11737 RSC;
(c) A. Fawcett, Pure Appl. Chem., 2020, 92, 751–765 CrossRef CAS;
(d) J. Turkowska, J. Durka and D. Gryko, Chem. Commun., 2020, 56, 5718–5734 RSC;
(e) M. A. A. Walczak, T. Krainz and P. Wipf, Acc. Chem. Res., 2015, 48, 1149–1158 CrossRef CAS PubMed.
- K. B. Wiberg, Angew. Chem., Int. Ed., 1986, 25, 312–322 CrossRef.
-
(a) A. Cairncross and E. P. Blanchard Jr, J. Am. Chem. Soc., 1966, 88, 496–504 CrossRef CAS;
(b) P. Wipf and M. A. A. Walczak, Angew. Chem., Int. Ed., 2006, 45, 4172–4175 CrossRef CAS PubMed;
(c) R. Kleinmans, T. Pinkert, S. Dutta, T. O. Paulisch, H. Keum, C. G. Daniliuc and F. Glorius, Nature, 2022, 605, 477–482 CrossRef CAS PubMed;
(d) R. Guo, Y.-C. Chang, L. Herter, C. Salome, S. E. Braley, T. C. Fessard and M. K. Brown, J. Am. Chem. Soc., 2022, 144, 7988–7994 CrossRef CAS PubMed;
(e) M. Xu, Z. Wang, Z. Sun, Y. Ouyang, Z. Ding, T. Yu, L. Xu and P. Li, Angew. Chem., Int. Ed., 2022, 61, e202214507 CrossRef CAS PubMed;
(f) Y. Liu, S. Lin, Y. Li, J.-H. Xue, Q. Li and H. Wang, ACS Catal., 2023, 13, 5096–5103 CrossRef CAS;
(g) N. Radhoff, C. G. Daniliuc and A. Studer, Angew. Chem., Int. Ed., 2023, e202304771 CAS;
(h) R. Kleinmans, S. Dutta, K. Ozols, H. Shao, F. Schäfer, R. E. Thielemann, H. T. Chan, C. G. Daniliuc, K. N. Houk and F. Glorius, J. Am. Chem. Soc., 2023, 145, 12324–12332 CrossRef CAS PubMed;
(i) S. Agasti, F. Beltran, E. Pye, N. Kaltsoyannis, G. Crisenza and D. J. Procter, Nat. Chem., 2023, 15, 535–541 CrossRef CAS PubMed;
(j) M. Wang, Y. Huang, C. Li and P. Lu, Org. Chem. Front., 2022, 9, 2149–2153 RSC;
(k) X. Ma, D. L. Sloman, Y. Han and D. J. Bennett, Org. Lett., 2019, 21, 7199–7203 CrossRef CAS PubMed;
(l) Y. Liang, R. Kleinmans, C. G. Daniliuc and F. Glorius, J. Am. Chem. Soc., 2022, 144, 20207–20213 CrossRef CAS PubMed;
(m) Y. Liang, F. Paulus, C. G. Daniliuc and F. Glorius, Angew. Chem., Int. Ed., 2023, e202305043 CAS;
(n) Y. Zheng, W. Huang, R. K. Dhungana, A. Granados, S. Keess, M. Makvandi and G. A. Molander, J. Am. Chem. Soc., 2022, 144, 23685–23690 CrossRef CAS PubMed;
(o)
T. V. T. Nguyen, A. Bossonnet and J. Waser, ChemRxiv, 2023, preprint, DOI:10.26434/chemrxiv-2023-s8j30;
(p) T. Yu, J. Yang, Z. Wang, Z. Ding, M. Xu, J. Wen, L. Xu and P. Li, J. Am. Chem. Soc., 2023, 145, 4304–4310 CrossRef CAS PubMed;
(q) R. Bychek and P. K. Mykhailiuk, Angew. Chem., Int. Ed., 2022, e202205103 CAS;
(r) H. Wang, H. Shao, A. Das, S. Dutta, H. T. Chan, C. Daniliuc, K. N. Houk and F. Glorius, Science, 2023, 381, 75 CrossRef CAS PubMed.
- H. K. Hall Jr and A. B. Padias, J. Polym. Sci., Part A: Polym. Chem., 2003, 41, 625–635 CrossRef.
-
(a) K. Tokunaga, M. Sato, K. Kuwata, C. Miura, H. Fuchida, N. Matsunaga, S. Koyanagi, S. Ohdo, N. Shindo and A. Ojida, J. Am. Chem. Soc., 2020, 142, 18522–18531 CrossRef CAS PubMed;
(b) P. Zhang, R. Zhuang, X. Wang, H. Liu, J. Li, X. Su, X. Chen and X. Zhang, Bioconjugate Chem., 2018, 29, 467–472 CrossRef CAS PubMed;
(c) B. D. Schwartz, A. P. Smyth, P. E. Nashar, M. G. Gardiner and L. R. Malins, Org. Lett., 2022, 24, 1268–1273 CrossRef CAS PubMed.
- A. Kaur, W. Lin, V. Dovhalyuk, L. Driutti, M. L. D. Martino, M. Vujasinovic, J.-M. Löhr, M. E. Sellin and D. Globisch, Chem. Sci., 2023, 14, 5291–5301 RSC.
-
(a) Y. Gaoni, Tetrahedron Lett., 1982, 23, 5215–5218 CrossRef CAS;
(b) R. Panish, S. R. Chintala, D. T. Boruta, Y. Fang, M. T. Taylor and J. M. Fox, J. Am. Chem. Soc., 2013, 135, 9283–9286 CrossRef CAS PubMed;
(c) R. A. Panish, S. R. Chintala and J. M. Fox, Angew. Chem., Int. Ed., 2016, 55, 4983–4987 CrossRef CAS PubMed;
(d) M. J. Kerner and P. Wipf, Org. Lett., 2021, 23, 3615–3619 CrossRef CAS PubMed;
(e) A. Guin, S. Bhattacharjee, M. S. Harariya and A. T. Biju, Chem. Sci., 2023, 14, 6585–6591 RSC.
-
(a) Y. Gaoni, Tetrahedron Lett., 1988, 29, 1591–1594 CrossRef CAS;
(b) S. Hoz, C. Azran and A. Sella, J. Am. Chem. Soc., 1996, 118, 5456–5461 CrossRef CAS;
(c) R. Gianatassio, J. M. Lopchuk, J. Wang, C.-M. Pan, L. R. Malins, L. Prieto, T. A. Brandt, M. R. Collins, G. M. Gallego, N. W. Sach, J. E. Spangler, H. Zhu, J. Zhu and P. S. Baran, Science, 2016, 351, 241–246 CrossRef CAS PubMed;
(d) J. M. Lopchuk, K. Fjelbye, Y. Kawamata, L. R. Malins, C.-M. Pan, R. Gianatassio, J. Wang, L. Prieto, J. Bradow, T. A. Brandt, M. R. Collins, J. Elleraas, J. Ewanicki, W. Farrell, O. O. Fadeyi, G. M. Gallego, J. J. Mousseau, R. Oliver, N. W. Sach, J. K. Smith, J. E. Spangler, H. Zhu, J. Zhu and P. S. Baran, J. Am. Chem. Soc., 2017, 139, 3209–3226 CrossRef CAS PubMed;
(e) J. A. Milligan, C. A. Busacca, C. H. Senanayake and P. Wipf, Org. Lett., 2016, 18, 4300–4303 CrossRef CAS PubMed;
(f) B. D. Schwartz, M. Y. Zhang, R. H. Attard, M. G. Gardiner and L. R. Malins, Chem.–Eur. J., 2020, 26, 2808–2812 CrossRef CAS PubMed;
(g) M. Takatsuki, H. Aoyama, K. Murai, M. Arisawa and M. Sako, Chem. Commun., 2023, 59, 7467–7470 RSC;
(h) Z. Zhang and V. Gevorgyan, J. Am. Chem. Soc., 2022, 144, 20875–20883 CrossRef CAS PubMed.
-
(a) L. Guo, A. Noble and V. K. Aggarwal, Angew. Chem., Int. Ed., 2021, 60, 212–216 CrossRef CAS PubMed;
(b) S. H. Bennett, A. Fawcett, E. H. Denton, T. Biberger, V. Fasano, N. Winter and V. K. Aggarwal, J. Am. Chem. Soc., 2020, 142, 16766–16775 CrossRef CAS PubMed;
(c) B. Wölfl, N. Winter, J. Li, A. Noble and V. K. Aggarwal, Angew. Chem., Int. Ed., 2023, e202217064 Search PubMed;
(d) H.-C. Shen, M. V. Popescu, Z.-S. Wang, L. de Lescure, A. Noble, R. S. Paton and V. K. Aggarwal, J. Am. Chem. Soc., 2023, 145, 16508–16516 CrossRef CAS PubMed;
(e) A. Fawcett, T. Biberger and V. K. Aggarwal, Nat. Chem., 2019, 11, 117–122 CrossRef CAS PubMed.
-
(a) G. Ernouf, E. Chirkin, L. Rhyman, P. Ramasami and J.-C. Cintrat, Angew. Chem., Int. Ed., 2020, 59, 2618–2622 CrossRef CAS PubMed;
(b) C. J. Pratt, R. A. Aycock, M. D. King and N. T. Jui, Synlett, 2020, 31, 51–54 CrossRef CAS PubMed;
(c) X. Wu, W. Hao, K.-Y. Ye, B. Jiang, G. Pombar, Z. Song and S. Lin, J. Am. Chem. Soc., 2018, 140, 14836–14843 CrossRef CAS PubMed;
(d) H. Gao, L. Guo, C. Shi, Y. Zhu, C. Yang and W. Xia, Adv. Synth. Catal., 2022, 364, 2140–2145 CrossRef CAS;
(e) X. Yu, M. Lübbesmeyer and A. Studer, Angew. Chem., Int. Ed., 2021, 60, 675–679 CrossRef CAS PubMed;
(f) M. Silvi and V. K. Aggarwal, J. Am. Chem. Soc., 2019, 141, 9511–9515 CrossRef CAS PubMed.
- M. Ociepa, A. J. Wierzba, J. Turkowska and D. Gryko, J. Am. Chem. Soc., 2020, 142, 5355–5361 CrossRef CAS PubMed.
-
(a) K. Dhake, K. J. Woelk, J. Becica, A. Un, S. E. Jenny and D. C. Leitch, Angew. Chem., Int. Ed., 2022, 61, e202204719 CrossRef CAS PubMed;
(b) M. Pomerantz, R. N. Wilke, G. W. Gruber and U. Roy, J. Am. Chem. Soc., 1972, 94, 2752–2758 CrossRef CAS;
(c) E. P. Blanchard Jr and A. Cairncross, J. Am. Chem. Soc., 1966, 88, 487–495 CrossRef.
-
(a) C.-Z. Zhu, J.-J. Feng and J. Zhang, Angew. Chem., Int. Ed., 2017, 56, 1351–1355 CrossRef CAS PubMed;
(b) J.-J. Feng and J. Zhang, ACS Catal., 2017, 7, 1533–1542 CrossRef CAS;
(c) T.-Y. Lin, H.-H. Wu, J.-J. Feng and J. Zhang, ACS Catal., 2017, 7, 4047–4052 CrossRef CAS;
(d) L. Tang, Q.-N. Huang, F. Wu, Y. Xiao, J.-L. Zhou, T.-T. Xu, W.-B. Wu, S. Qu and J.-J. Feng, Chem. Sci., 2023, 14, 9696–9703 RSC;
(e) L. Tang, Y. Xiao, F. Wu, J.-L. Zhou, T.-T. Xu and J.-J. Feng, Angew. Chem., Int. Ed., 2023, e202310066 Search PubMed.
- For reviews, see:
(a) X.-H. Ouyang, R.-J. Song and J.-H. Li, Chem.–Asian J., 2018, 13, 2316–2332 CrossRef CAS PubMed;
(b) M. M. D. Pramanik, H. Qian, W.-J. Xiao and J.-R. Chen, Org. Chem. Front., 2020, 7, 2531–2537 RSC;
(c) A. L. G. Kanegusuku and J. L. Roizen, Angew. Chem., Int. Ed., 2021, 60, 21116–21149 CrossRef PubMed;
(d) A. Lipp, S. O. Badir and G. A. Molander, Angew. Chem., Int. Ed., 2021, 60, 1714–1726 CrossRef CAS PubMed;
(e) S. Poplata, A. Tröster, Y.-Q. Zou and T. Bach, Chem. Rev., 2016, 116, 9748–9815 CrossRef CAS PubMed;
(f) D. Staveness, I. Bosque and C. R. J. Stephenson, Acc. Chem. Res., 2016, 49, 2295–2306 CrossRef CAS PubMed;
(g) D. M. Schultz and T. P. Yoon, Science, 2014, 343, 1239176 CrossRef PubMed;
(h) D. P. Hari and B. König, Angew. Chem., Int. Ed., 2013, 52, 4734–4743 CrossRef CAS PubMed;
(i) C. K. Prier, D. A. Rankic and D. W. C. MacMillan, Chem. Rev., 2013, 113, 5322–5363 CrossRef CAS PubMed;
(j) J. Xuan and W.-J. Xiao, Angew. Chem., Int. Ed., 2012, 51, 6828–6838 CrossRef CAS PubMed ; For selected references, see: ;
(k) D. A. Nicewicz and D. W. C. MacMillan, Science, 2008, 322, 77–80 CrossRef CAS PubMed;
(l) J. D. Nguyen, J. W. Tucker, M. D. Konieczynska and C. R. J. Stephenson, J. Am. Chem. Soc., 2011, 133, 4160–4163 CrossRef CAS PubMed;
(m) C.-J. Wallentin, J. D. Nguyen, P. Finkbeinera and C. R. J. Stephenson, J. Am. Chem. Soc., 2012, 134, 8875–8884 CrossRef CAS PubMed;
(n) Q. Liu, H. Yi, J. Liu, Y. Yang, X. Zhang, Z. Zeng and A. Lei, Chem.–Eur. J., 2013, 19, 5120–5126 CrossRef CAS PubMed;
(o) Y. Zhu, L. Zhang and S. Luo, J. Am. Chem. Soc., 2014, 136, 14642–14645 CrossRef CAS PubMed;
(p) W. Chen, Z. Liu, J. Tian, J. Li, J. Ma, X. Cheng and G. Li, J. Am. Chem. Soc., 2016, 138, 12312–12315 CrossRef CAS PubMed;
(q) T. Lei, W.-Q. Liu, J. Li, M.-Y. Huang, B. Yang, Q.-Y. Meng, B. Chen, C.-H. Tung and L.-Z. Wu, Org. Lett., 2016, 18, 2479–2482 CrossRef CAS PubMed;
(r) M. Silvi, C. Sandford and V. K. Aggarwal, J. Am. Chem. Soc., 2017, 139, 5736–5739 CrossRef CAS PubMed;
(s) D. F. J. Caputo, C. Arroniz, A. B. Dürr, J. J. Mousseau, A. F. Stepan, S. J. Mansfield and E. A. Anderson, Chem. Sci., 2018, 9, 5295–5300 RSC;
(t) J. Li, M. Kong, B. Qiao, R. Lee, X. Zhao and Z. Jiang, Nat. Commun., 2018, 9, 2445 CrossRef PubMed;
(u) B. Schweitzer-Chaput, M. A. Horwitz, E. de P. Beato and P. Melchiorre, Nat. Chem., 2019, 11, 129–135 CrossRef CAS PubMed;
(v) X. Zhang, R. T. Smith, C. Le, S. J. McCarver, B. T. Shireman, N. I. Carruthers and D. W. C. MacMillan, Nature, 2020, 580, 220–226 CrossRef CAS PubMed.
- CCDC 2287475 (3am).†.
Footnotes |
† Electronic supplementary information (ESI) available: Experimental details, characterization, and spectroscopic data. CCDC 2287475. For ESI and crystallographic data in CIF or other electronic format see DOI: https://doi.org/10.1039/d3sc04457b |
‡ These authors contributed equally. |
|
This journal is © The Royal Society of Chemistry 2023 |