DOI:
10.1039/D3SC02554C
(Edge Article)
Chem. Sci., 2023,
14, 9380-9388
Suitability of a diamine functionalized metal–organic framework for direct air capture†
Received
19th May 2023
, Accepted 7th August 2023
First published on 8th August 2023
Abstract
The increase in the atmospheric carbon dioxide level is a significant threat to our planet, and therefore the selective removal of CO2 from the air is a global concern. Metal–organic frameworks (MOFs) are a class of porous materials that have shown exciting potential as adsorbents for CO2 capture due to their high surface area and tunable properties. Among several implemented technologies, direct air capture (DAC) using MOFs is a promising strategy for achieving climate targets as it has the potential to actively reduce the atmospheric CO2 concentration to a safer levels. In this study, we investigate the stability and regeneration conditions of N,N′-dimethylethylenediamine (mmen) appended Mg2(dobpdc), a MOF with exceptional CO2 adsorption capacity from atmospheric air. We employed a series of systematic experiments including thermogravimetric analysis (TGA) coupled with Fourier transformed infrared (FTIR) and gas chromatography mass spectrometer (GCMS) (known as TGA-FTIR-GCMS), regeneration cycles at different conditions, control and accelerated aging experiments. We also quantified CO2 and H2O adsorption under humid CO2 using a combination of data from TGA-GCMS and coulometric Karl-Fischer titration techniques. The quantification of CO2 and H2O adsorption under humid conditions provides vital information for the design of real-world DAC systems. Our results demonstrate the stability and regeneration conditions of mmen appended Mg2(dobpdc). It is stable up to 50% relative humidity when the adsorption temperature varies from 25–40 °C and the best regeneration condition can be achieved at 120 °C under dynamic vacuum and at 150 °C under N2.
Introduction
As the carbon dioxide (CO2) emissions continue to rise, climate change pushes the Earth towards an alarmingly dire situation. Reducing emissions will no longer be sufficient to mitigate this crisis, and we must establish facilities and governmental policies that can remove billions of tons of CO2 from the air annually.1,2 Among different methods of atmospheric CO2 reduction such as flue gas capture and other point source capture strategies, direct air capture (DAC) has shown promise in several literature and environmental reports for climate change moderation.3,4 DAC aims to extract CO2 selectively from ambient air where concentrations are as low as 400 ppm. Despite having the advantage that the capture point can be anywhere and recent favorable reports and successes of DAC technologies, finding an appropriate, reversible, cyclable, sorbent material for DAC of CO2 from dilute air (400 ppm of CO2) is still a major challenge.5,6 Selective adsorption of CO2 by liquid amine solution is one of the industrially viable options, but the high cost of regeneration, decomposition of amines with time, and its severe corrosive nature are major drawbacks for practical application in a DAC setup.7,8
Amine-functionalized solid porous adsorbents are an emerging class of materials which can selectively bind CO2 through carbamate formation. The strategy was first implemented with amine-grafted mesoporous silica for natural gas purification.9,10 Among other potential solid adsorbents, metal–organic frameworks (MOFs) have received growing attention due to their high degree of tunability, selectivity, and porosity (Fig. 1).11 Amine functionality within the ligand backbone has shown the possibility of selective capture of CO2 over water.12 However, total amine loading within the MOF pore makes the overall uptake fall short under real DAC conditions.11,13 Post-synthetic amine grafting, which attaches amines within the MOF pore, to an open metal site has improved the CO2 capture performance. In 2008, diamine grafting on the coordinatively unsaturated sites (CUS) of MOFs was first introduced by Férey and Chang et al. where the unsaturated Cr(III) sites of MIL-101 are functionalized with ethylenediamine.14 Subsequently, in 2012 Jones et al. reported the first ethylenediamine (en) grafted Mg-MOF-74 (Mg2(dobdc), dobdc4− = 2,5-dioxidoterephthalate) and explore the possibility of CO2 capture. The CO2 uptake capacity of en-Mg-MOF-74 is comparable to that of polyethyleneimide (PEI)-impregnated silica and amine grafted silica which is a well-known benchmark material.15,16 Mg-MOF-74 has unsaturated magnesium (Mg) centers that can act as Lewis acid and interact with guest molecules. Upon post-synthetic functionalization various diamines can be appended to an open metal site (OMS) in κ1 fashion leaving the other amine side free to interact with carbon dioxide. Long et al. have developed diamine appended pore expanded derivative of Mg-MOF-74 labeled as Mg2(dobpdc) (dobpdc4− = 4,4′-dioxidobiphenyl-3,3′-dicarboxylate) which has an increased pore diameter (Mg-MOF-74: 11 Å vs. Mg2(dobpdc): 18.4 Å) and aids to tether more amine molecules within the channel.17 Ethylenediamine appended Mg2(dobpdc), named as en-Mg2(dobpdc) has capacity of capturing 2.83 mmol g−1 CO2 at 25 °C and 0.39 mbar or atmospheric CO2 levels, however a 6% loss of capacity was observed after first five cycles in simulated air. Long and others have tested various homo and heterodiamines to address the challenge of amine loss during regeneration to no avail.18,19 Among different homo and hetero diamines, N,N′-dimethylethylenediamine (mmen) appended Mg2(dobpdc) has been known to have high CO2 adsorption capacity at very low-pressure ranges with enhanced stability.20 A mechanistic study for CO2 adsorption in mmen-M2(dobpdc) (M = Mg, Co, Zn, Mn, Fe) has proposed that the adsorption of CO2 at ambient temperatures is possible via cooperative insertion into the mmen-M2(dobpdc) to form one dimensional carbamate chain and the uncoordinated amine of a mmen molecule acts as a strong base to remove the acidic proton from the metal-bound amine of a neighboring mmen molecule.20 Although the volatilization of coordinated diamine during the regeneration process upon dry and humid conditions remains unclear, some reports claim that diamine tethered Mg2(dobpdc) loses CO2 capture capacity due to a loss of diamine from the grafted sites.18,21
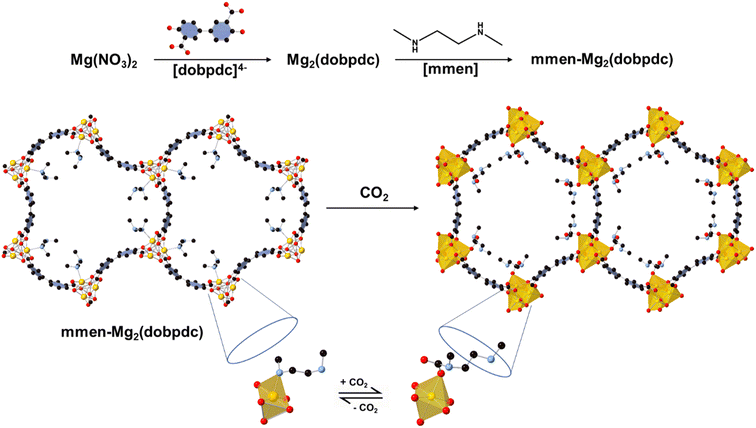 |
| Fig. 1 Schematic illustration of diamine (mmen) appended Mg2(dobpdc) synthesis and structure. The framework structure is adopted from single crystal X-ray analysis of the isostructural zinc compound DEF-1. Yellow, red, and gray spheres represent Mg, O, and C atoms respectively; H atoms are omitted for clarity. Addition of mmen to the unsaturated framework yields the amine-appended Mg2(dobpdc).17,22 | |
Therefore, it is essential to determine if the capacity fade is due to the degradation of the amine during the regeneration process or if the MOF has simply not been fully regenerated under standard conditions. Adsorption and desorption kinetics of CO2 have critical impact on the efficiency of the adsorbents, wherein the fastest kinetics allow for the highest cost effectiveness. mmen-Mg2(dobpdc) (Fig. 1) has been selected as a representative material system to understand the underlying chemical and structural changes that occur during the adsorption and desorption processes in relevant DAC conditions. Along with CO2 isotherms and cycling stability experiments, herein we identify additional metrics and experiments for evaluation of long-term performance to determine their applicability as DAC-sorbents. Real time thermogravimetric analysis (TGA) coupled with Fourier transformed infrared (FTIR) and gas chromatography mass spectrometer (GCMS) (known as TGA-FTIR-GCMS) allow us for deeper understanding of the mechanistic pathways of thermal regeneration. Since humidity is variable globally and temporally, it is important to evaluate the capture of CO2 in presence of water. Different experimental conditions were utilized to further understand the regeneration performance and suggest the desorption mechanism in dry and wet conditions. Moreover, we have performed 50 regeneration cycles under dry and humid conditions with different adsorption–desorption conditions along with long cycles test to assess their sorption performance. Finally, the capture of CO2 was quantified in presence of water by TGA-GCMS coupled instruments for determination of practical applicability of mmen-Mg2(dobpdc) globally.
Results and discussions
Synthesis, characterization, and stability of parent and diamine appended Mg2(dobpdc)
The phase purities of the mmen-Mg2(dobpdc) and diamine grafted MOF are aligned with simulated powder X-ray diffraction (PXRD) patterns. Moreover, crystallinity of the MOF is not affected after amine appendant as confirmed by PXRD measurement (Fig. S1†). The overall amine loading was confirmed by a 1H NMR study to be 50–60% amination per metal site (Fig. S2†). The pristine MOF and diamine-MOF show porosity and surface area in N2 isotherms at 77 K (Fig. 2a). Reduction in BET surface area and pore size for the amine functionalized MOF confirms the successful grafting of diamine to the metal sites of the MOFs. DFT pore size distributions and pore sizes were calculated from N2 adsorption at 77 K using the DFT pore model with cylinder pore geometries for an oxide surface. DFT pore size distributions show the amine appended structure has a smaller pore (Fig. 2b). BET surface areas for pristine MOF and mmen-MOF (60% amine per metal site) were calculated to be 2920 and 1550 m2 g−1, respectively.
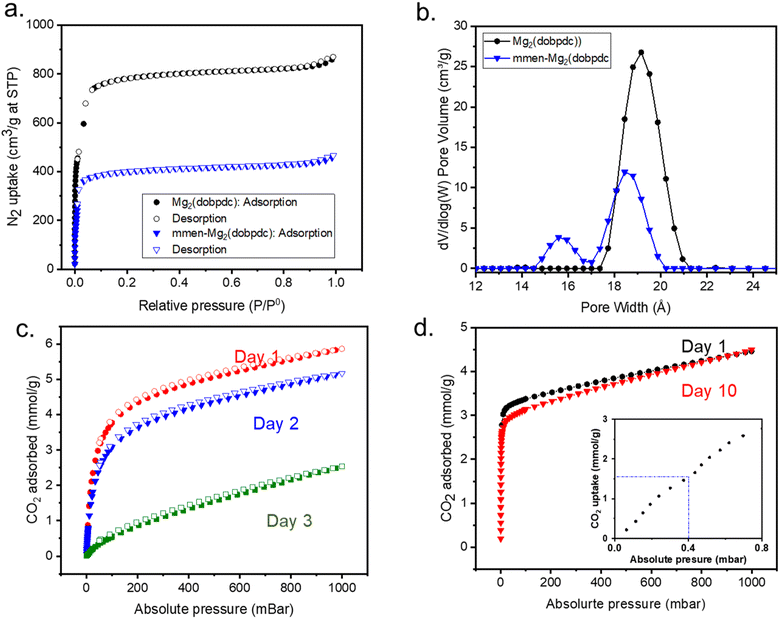 |
| Fig. 2 (a) N2 adsorption–desorption comparison isotherms of Mg2(dobpdc) (black) and mmen-Mg2(dobpdc) (blue) at 77 K. (b) Comparison of DFT pore size distribution of Mg2(dobpdc) (black) and mmen-Mg2(dobpdc) (blue). (c) CO2 uptake comparison isotherms of Mg2(dobpdc) with exposure time in air and at 25 °C and (d) CO2 uptake comparison isotherms of mmen-Mg2(dobpdc) with exposure time in air and at 25 °C (inset: low pressure CO2 uptake by mmen-Mg2(dobpdc)). | |
Determination of the stability of the parent Mg2(dobpdc) and mmen-Mg2(dobpdc), was examined by exposing the samples to ambient conditions for several days while monitoring the CO2 uptake efficiency at 25 °C (Fig. 2c and d). The results indicate that the pristine MOF, Mg2(dobpdc) loses CO2 uptake capacity after three days, while the diamine appended MOF shows comparable CO2 uptake even after 10 days. The color of the pristine Mg2(dobpdc), which is linked to the phase, changes from off white to blue within 3 days of exposure to atmosphere whereas the off white color of mmen-Mg2(dobpdc) remains same even after 1 month. This additional stability from the diamine functionalization has been reported in literature.17,23 In addition to the increased stability the diamine appended MOF also shows stronger affinity towards CO2 at low pressure indicating involvement of chemisorption (Fig. 2d: inset).
Mechanistic confirmation of carbamate formation monoamine grafted Mg2(dobpdc)
For a DAC application, adsorption capacity at 400 ppm is crucial since this mimic the concentration of CO2 under ambient conditions. Notably, the adsorption capacity of mmen-Mg2(dobpdc) at 400 ppm and 25 °C is 1.5 mmol g−1 (Fig. 2d (inset)). To validate the formation of carbamate during the capture of CO2, control experiments were performed to understand the role of diamine in the CO2 capture mechanism. Mg2(dobpdc) was functionalized with propylamine (pa) which is a monoamine following the same process used for diamine functionalization. PXRD patterns show the crystallinity is unchanged (Fig. S3†). 1H NMR studies confirmed the amine loading per metal site remains around 50–60% (Fig. S4†).
The N2 isotherm shows BET surface area is compatible with mmen-Mg2(dobpdc) (Fig. 3a). Pore size distribution reveals the presence of two different pore sizes after functionalization with pa and is comparable with the pore size of mmen appended Mg2(dobpdc) (Fig. 3b). CO2 adsorption isotherm at 25 °C illustrates that the pa-Mg2(dobpdc) is not able to capture comparable amounts of CO2 as adsorbed by mmen-Mg2(dobpdc) and chemisorption part at lower pressure is also absent (Fig. 3c). These results indicate that presence of diamine is required to capture sufficient CO2 from atmosphere through the cooperative mechanism.20
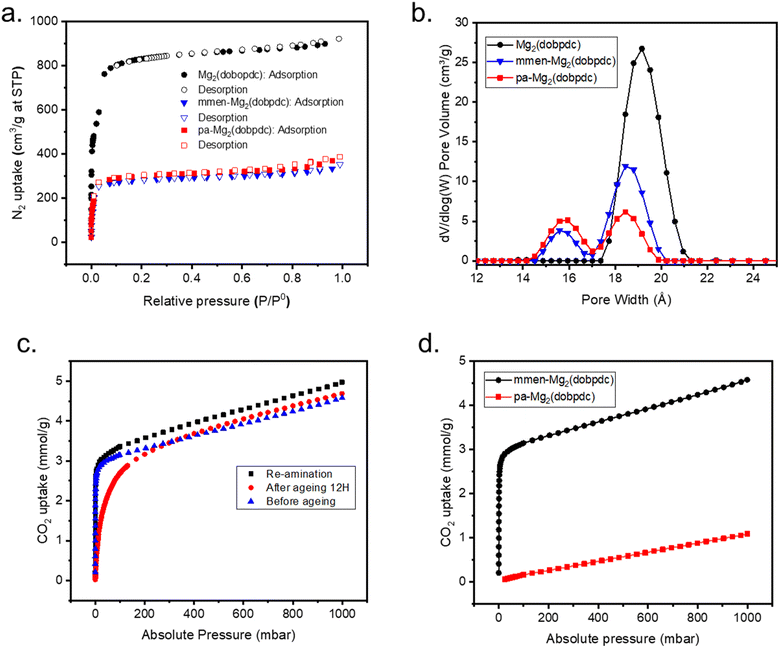 |
| Fig. 3 Control experiments (a) N2 isotherms of Mg2(dobpdc) (black), mmen-Mg2(dobpdc) (blue) and pa-Mg2(dobpdc) (red) at 77 K. (b) Pore size distributions of Mg2(dobpdc) (black), mmen-Mg2(dobpdc) (blue) and pa-Mg2(dobpdc) (red) (c) CO2 uptake of mmen-Mg2(dobpdc) (blue) and pa-Mg2(dobpdc) (red) at 25 °C; (d) CO2 uptake of mmen-Mg2(dobpdc) before ageing (purple), after ageing (orange), and after re-amination (green) at 25 °C. All the materials were used from the same batch. | |
Finally, the mmen-Mg2(dobpdc) was then aged through accelerated conditions at 80% relative humidity and 70 °C for 12 hours, 1H NMR indicates that upon exposure to such harsh conditions, amine loading was reduced from 60% to trace amount (Fig. S5†). Consequently, low-pressure (400 ppm) CO2 uptake is substantially decreased to 0.07 mmol g−1 from initial 1.5 mmol g−1 (for mmen-Mg2(dobpdc)) due to a reduction in the coordinated amines which is responsible for low pressure chemisorption (Fig. 3d). Interestingly, when the same sample was again re-aminated to 50–60% loading with the diamine the same CO2 uptake observed (Fig. 3d). This experiment confirms that the diamine is responsible for the chemisorption of CO2 and re-amination of the parent Mg2(dobpdc) is possible.
Stability of coordinated diamine during regeneration process
mmen-Mg2(dobpdc) fully regenerates when heated at 100 °C for four hours under dynamic vacuum as confirmed by PXRD and N2 isotherms. Determination of the stability of the amines in the MOFs, was evaluated with thermogravimetric analysis (TGA) coupled with FTIR and GCMS. To verify the diamine stability in DAC conditions, it is also important to evaluate its thermal stability under humid conditions when it is fully adsorbed with CO2. The thermogram under humid He does not show any significant difference than that under dry He condition (Fig. 4a). CO2 (m/z: 44) is detected in GCMS at regeneration temperature 90–100 °C (Fig. 3c) which is due to the adsorbed CO2. The lack of amine leaching under the influence of moisture at this temperature indicates that the humidity does not affect the regeneration and stability of grafted amines. The CO2 signal can also be detected with FTIR measurements (Fig. 4b) during 50 minutes of thermal regeneration where the temperature was 50–100 °C indicating desorption of adsorbed CO2 at this temperature range. Fragmentations of diamine (m/z: 58, 88) are detected after 200 °C (Fig. 4d and e). The m/z: 44 detected after 200 °C (Fig. 4c) is attributed to the CO2 released for the degradation of diamine.
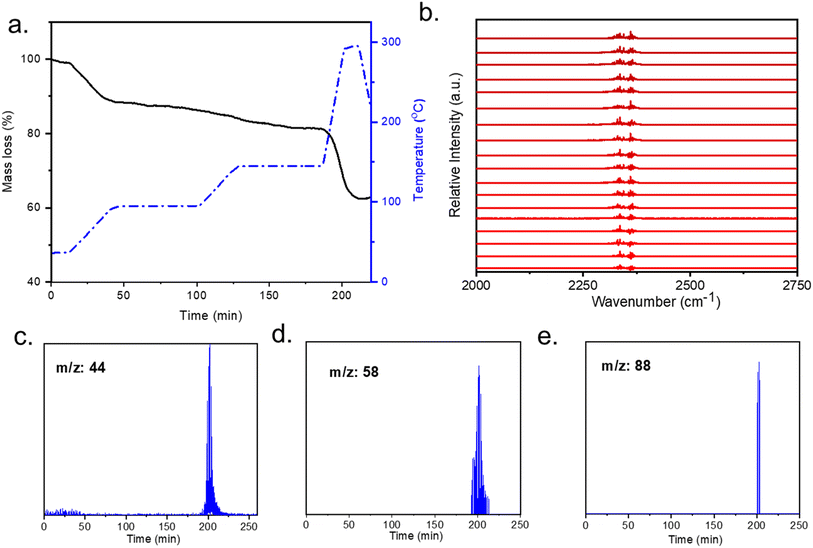 |
| Fig. 4 TGA-FTIR-GCMS experiments on mmen-Mg2(dobpdc) with adsorbed CO2: (a) thermogravimetric decomposition profile under humid He (black line); temperature ramp rate is shown in blue line; (b) FTIR spectra (0–50 min, 50–100 °C); characteristic CO2 was detected at 2360 and 2340 cm−1, each data was collected every 180 second. (c)–(e) gas chromatography mass spectra (GCMS) showing ions of released amine fragments. | |
To determine conditions for the release of adsorbed CO2 the stability of diamine was evaluated with GCMS and FTIR spectra. Both spectra for the desorbed material show that the CO2 (m/z: 44) and fragmentations of diamine (m/z: 58 and 88) molecules are released only after 200 °C. The first one indicates the stability of adsorbent without any CO2 adsorption (Fig. S6†), which shows that until 200 °C, the diamine appended MOF is stable. There is no loss of coordinated diamine at the regeneration temperature. After a CO2 capture cycle the mmen-MOF also showed no amine peak by GCMS and FTIR spectra before 200 °C which indicates no loss of grafted amines during regeneration. CO2 molecules were released between 90-100 °C and after 200 °C (Fig. S7†). The first CO2 molecules detected around 90–100 °C are due to the desorbing of the CO2 in the host structure, while the CO2 molecules released after 200 °C are due to the degradation of the amines.
Thermogravimetric CO2 adsorption–desorption under humid conditions
To establish a successful DAC experimental setup, understanding of the regeneration process of sorbents under dry and humid conditions is critical for establishing a protocol for DAC suitability. As demonstrated by TGA-FTIR GCMS no diamine loss occurs until 200–250 °C. However, the conditions for full desorption may illuminate the reason inherent capacity loss during cycling experiments.
The mmen-Mg2(dobpdc), was first regenerated and cycled at 120 °C for 30 minutes under N2 after exposing it to the ambient air. The desorption behavior under two humidity levels (25–30% RH and 40–50% RH) of the laboratory were assessed. After running 25–30% RH for 30 minutes (Fig. 5a) for 10 cycles (around 700 minutes), overall CO2 and/or H2O uptake from air was not decreased. But when the same cycle was performed after exposing to 45–50% RH (Fig. 5b), with 15% decrease in overall (CO2 + H2O) uptake was noticed.
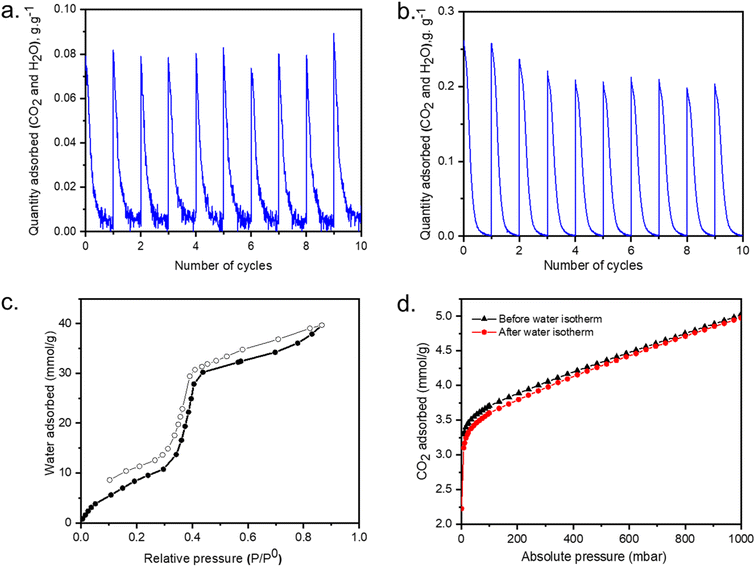 |
| Fig. 5 Regeneration performance cycles at 120 °C for mmen-Mg2(dobpdc): (a) after exposing to ambient air with 25% relative humidity; (b) after exposing to ambient air with 45% relative humidity; (c) water isotherm at 25 °C; (d) CO2 adsorption isotherms before and after measuring water isotherms at 25 °C. | |
Water isotherms were then collected at room temperature to assist in the understanding of water interactions within the structure. An S-shaped sorption isotherm was observed with the adsorbed amount of water gradually increasing until 30% RH, followed by an abrupt uptake between 40% and 50% RH, indicative of water filling into the pore system with the maximum uptake is 40 mmol g−1 at 90% RH (Fig. 5c). Water adsorption was found to be reversible with a small hysteresis over this material. Moreover, after activation at 120 °C under dynamic vacuum for 4 hours, CO2 uptake efficiency remained the same before and after water isotherm measurement (Fig. 5d).
TGA-GCMS of the mmen-Mg2(dobpdc), after exposing it to open environment (temperature: 27–32 °C, relative humidity: 30–50%) for several days, show that no amine leaching occurred at the regeneration temperature of 120 °C. However, CO2 and H2O are detected from the GCMS (Fig. S8 and S9†) indicating the diamine appended MOF adsorbs both CO2 and moisture from the open air.
Assessing the impact of regeneration conditions on cycling stability
Four different regeneration conditions (described in Fig. 6) were applied to determine if the cycling stability of the adsorbent is linked to the regeneration conditions of the sorbent (Fig. 6). It was found that the most optimal regeneration can be achieved under flowing N2 at 150 °C. In addition to these four conditions CO2 isobars with varying adsorption and desorption times as well as heating and cooling rates were also explored (Fig. S10–S13†). But it was found that time and ramp rate do not alter the total CO2 capacity of the adsorbent. For the long cycling experiments, we have maintained the adsorption and desorption time for 15 minutes for each case while the ramp rate was 10 °C min−1.
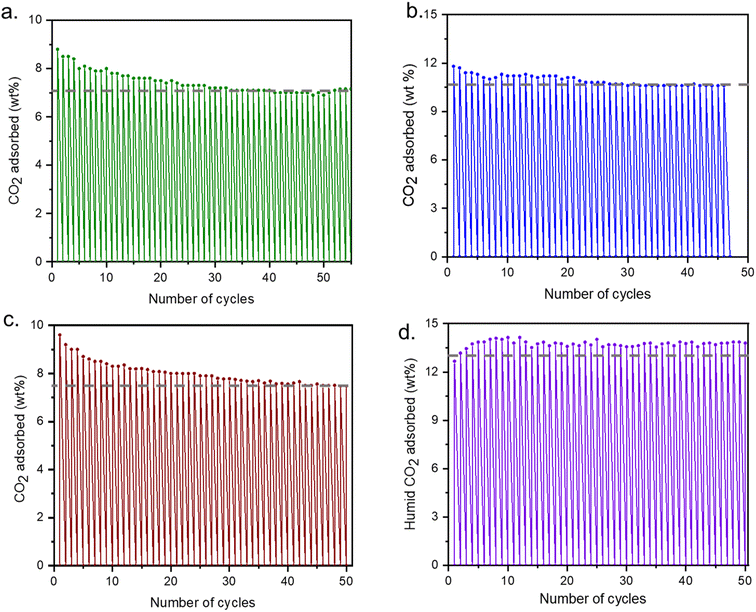 |
| Fig. 6 Adsorption–desorption cycling for mmen-Mg2(dobpdc): (a) desorption – CO2 at 150 °C (15 min) and adsorption – pure CO2 at 30 °C (15 min); (b) desorption – N2 at 150 °C (15 min) and adsorption – pure CO2 at 30 °C (15 min) (c) desorption – N2 at 150 °C (15 min) and adsorption – 15% CO2 in N2 at 30 °C (15 min) (d) desorption – N2 at 150 °C (15 min) and adsorption – humid 100% CO2 at 30 °C (15 min). The ramp rate was maintained as 10 °C for heating and cooling. The grey dashed line in each figure indicates the capacity of adsorbing dry and humid CO2 after 50 cycles. | |
When desorption is carried out under CO2 atmosphere, 7% of CO2 capturing efficiency is maintained after 50 cycles (3000 minutes), but after 20 cycles it reduces to 7.5% from 9%, which means 80% of the materials capacity is retained (Fig. 6a). When the adsorbent was regenerated under N2 at 150 °C, 90% of the total capacity was maintained and almost no change in capacity was observed after 15–20 cycles (Fig. 6b). We have also performed the cycling experiment with 15% CO2 in N2 (resemble to flue gas) and 88% of total capacity is retained (Fig. 6c). Though no such decrease was observed after 20 cycles. So, the working capacity of the adsorbent under dry CO2, remains around 85–90% after 50 cycling experiments. Finally, under the humid cycle in which dry CO2 was passed through a water bubbler at room temperature before entering the sample chamber, there is no change in overall capacity and total (CO2 + H2O) capacity is maintained throughout the cycles (Fig. 6d). However, isolation of the relative concentration of each gas is needed to further determine the CO2 capacity.
CO2 and H2O gas component quantification
Despite best efforts to make sorbents selective to CO2, most adsorbents capture both CO2 and water. As we have seen, mmen-Mg2(dobpdc) captures both CO2 and H2O from air, as detected by TGA-GCMS during the regeneration of the MOF. Herein we report a technique to quantify the amount of CO2 and H2O using the coupled TGA-GCMS technique. Integrated intensities of chromatogram for CO2 (m/z: 44) and H2O (m/z: 18) were plotted against retention time of GCMS (Fig. 7a and b). Under humid CO2 (50% RH), the mmen-Mg2(dobpdc) adsorbed 1.7 mmol g−1 of CO2 (7.5% w/w) and 3 mmol g−1 of H2O (5.7% w/w) at 120 °C. Karl-Fischer coulometric titration on the sample (after re-exposure) corroborated that the sample uptakes around 3 mmol g−1 of water. Similar CO2 uptakes with other amine appended Mg2(dobpdc) and Mg2(dotpdc) (dotpdc4− = 4,4′′-dioxido-[1,1′:4′,1′′-terphenyl]-3,3′′-dicarboxylate) have been reported by Long et al.18,24 The overall amount of adsorbed CO2+H2O is also consistent with the weight loss measured by TGA (Fig. S14†). The calibration curves for CO2 and H2O from standard calcium oxalate are shown in Fig. S15.† These values are significant to comment on the applicability of this system in real DAC plants.
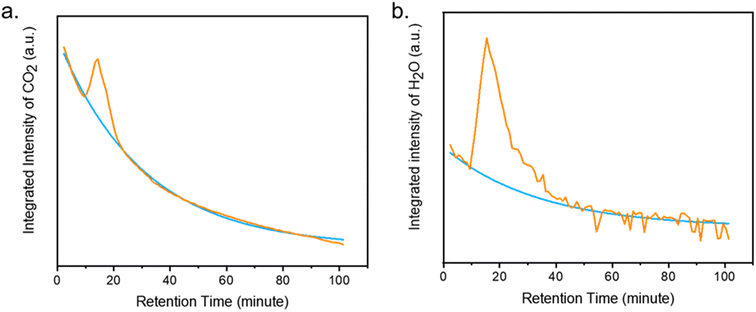 |
| Fig. 7 (a) Integrated intensity of desorbed CO2 (blue: background, orange: sample) and (b) integrated intensity of desorbed H2O (blue: background, orange: sample). Blue curve shows the background intensities of slow displacement of CO2 and H2O from the carrier gas (He), while the orange curve indicates the integrated intensity of the adsorbed CO2 and H2O from the chromatogram. | |
Conclusion
Amine appended-Mg2(dobpdc) is a well-known MOF which can capture CO2 at pressures as low as 400 ppm, making it a superior screening material for direct air capture. However, performance of these MOFs is dependent on the stability and recyclability under humid conditions. We have carried out a systematic study to assess the applicability of N,N′-dimethylethylenediamine (mmen) appended Mg2(dobpdc) MOF for direct air capture. With the help of coupled TGA-GCMS-FTIR, we have screened the performance of mmen-appended Mg2(dobpdc) under real DAC conditions. The quantification of exhaust gas revealed that this amine appended-Mg2(dobpdc) can uptake significant amount of CO2 (∼1.7 mmol g−1) in presence of 50% relative humidity. Regeneration is possible under appropriate conditions and can be enhanced through introduction of N2 gas under the regeneration conditions. These results have far-reaching implications that will afford the opportunity to engineer and design the process parameters like temperature, humidity, adsorption/regeneration conditions in similar diamine-appended MOF as future DAC sorbent.
Data availability
Information supporting this article has been uploaded as part of the ESI.† Additional data is available from the authors on reasonable request.
Author contributions
O. K. F. supervised the project. S. B., T. I., and O. K. F. conceived the project and led the investigation. S. B., D. S., T. I., and O. K. F. designed the experiments and interpreted the results, with help from K. I., H. X., and X. W. TGA-GCMS-FTIR characterization and controlled experiments were performed by S. B., C. D. M., and N. M. B. Manuscript was written by S. B., D. S., M. L. B., T. I., V. P. D., and O. F. K, and all other authors commented on and revised the manuscript.
Conflicts of interest
The authors declare the following competing financial interest(s): O. K. F. has a financial interest in the start-up company NuMat Technologies, which is seeking to commercialize metal–organic frameworks.
Acknowledgements
Authors gratefully acknowledge the support from the Department of Energy, Basic Energy Science (BES) Office through awards no. DE-SC0022332. MLB would also like to acknowledge the Ryan Fellowship for support. This work made use of the Integrated Molecular Structure Education and Research Center (IMSERC) NMR facility at Northwestern University, which has received support from NSF CHE-1048773, the Soft and Hybrid Nanotechnology Experimental (SHyNE) Resource (NSF ECCS-2025633), Int. Institute of Nanotechnology, and Northwestern University.
Notes and references
- S. Solomon, G.-K. Plattner, R. Knutti and P. Friedlingstein, Irreversible climate change due to carbon dioxide emissions, Proc. Natl. Acad. Sci. U. S. A., 2009, 106, 1704–1709 CrossRef CAS PubMed.
-
Adoption of the paris agreement, United Nations Framework Convention on Climate Change, Paris, 2015, https://unfccc.int/resource/docs/2015/cop21/eng/l09r01.pdf Search PubMed.
- D. W. Keith, M. Ha-Duong and J. K. Stolaroff, Climate Strategy with Co2 Capture from the Air, Clim. Change, 2006, 74, 17–45 CrossRef CAS.
- D. W. Keith, Why Capture CO2 from the Atmosphere?, Science, 2009, 325, 1654–1655 CrossRef CAS PubMed.
- A. Kumar, D. G. Madden, M. Lusi, K.-J. Chen, E. A. Daniels, T. Curtin, J. J. Perry Iv and M. J. Zaworotko, Direct Air Capture of CO2 by Physisorbent Materials, Angew. Chem., Int. Ed., 2015, 54, 14372–14377 CrossRef CAS PubMed.
- H. He, W. Li, M. Zhong, D. Konkolewicz, D. Wu, K. Yaccato, T. Rappold, G. Sugar, N. E. David and K. Matyjaszewski, Reversible CO2 capture with porous polymers using the humidity swing, Energy Environ. Sci., 2013, 6, 488–493 RSC.
- B. A. Oyenekan and G. T. Rochelle, Alternative stripper configurations for CO2 capture by aqueous amines, AIChE J., 2007, 53, 3144–3154 CrossRef CAS.
- M. Wang, A. Lawal, P. Stephenson, J. Sidders and C. Ramshaw, Post-combustion CO2 capture with chemical absorption: a state-of-the-art review, Chem. Eng. Res. Des., 2011, 89, 1609–1624 CrossRef CAS.
- Y. Belmabkhout, G. De Weireld and A. Sayari, Amine-Bearing Mesoporous Silica for CO2 and H2S Removal from Natural Gas and Biogas, Langmuir, 2009, 25, 13275–13278 CrossRef CAS PubMed.
- H. Y. Huang, R. T. Yang, D. Chinn and C. L. Munson, Amine-Grafted MCM-48 and Silica Xerogel as Superior Sorbents for Acidic Gas Removal from Natural Gas, Ind. Eng. Chem. Res., 2003, 42, 2427–2433 CrossRef CAS.
- C. A. Trickett, A. Helal, B. A. Al-Maythalony, Z. H. Yamani, K. E. Cordova and O. M. Yaghi, The chemistry of metal-organic frameworks for CO2 capture, regeneration and conversion, Nat. Rev. Mater., 2017, 2, 17045 CrossRef CAS.
- R. W. Flaig, T. M. Osborn Popp, A. M. Fracaroli, E. A. Kapustin, M. J. Kalmutzki, R. M. Altamimi, F. Fathieh, J. A. Reimer and O. M. Yaghi, The Chemistry of CO2 Capture in an Amine-Functionalized Metal-Organic Framework under Dry and Humid Conditions, J. Am. Chem. Soc., 2017, 139, 12125–12128 CrossRef CAS PubMed.
- A. M. Fracaroli, H. Furukawa, M. Suzuki, M. Dodd, S. Okajima, F. Gándara, J. A. Reimer and O. M. Yaghi, Metal–Organic Frameworks with Precisely Designed Interior for Carbon Dioxide Capture in the Presence of Water, J. Am. Chem. Soc., 2014, 136, 8863–8866 CrossRef CAS PubMed.
- Y. K. Hwang, D.-Y. Hong, J.-S. Chang, S. H. Jhung, Y.-K. Seo, J. Kim, A. Vimont, M. Daturi, C. Serre and G. Férey, Amine Grafting on Coordinatively Unsaturated Metal Centers of MOFs: Consequences for Catalysis and Metal Encapsulation, Angew. Chem., Int. Ed., 2008, 47, 4144–4148 CrossRef CAS PubMed.
- S. Choi, T. Watanabe, T.-H. Bae, D. S. Sholl and C. W. Jones, Modification of the Mg/DOBDC MOF with Amines to Enhance CO2 Adsorption from Ultradilute Gases, J. Phys. Chem. Lett., 2012, 3, 1136–1141 CrossRef CAS PubMed.
- E. S. Sanz-Pérez, C. R. Murdock, S. A. Didas and C. W. Jones, Direct Capture of CO2 from Ambient Air, Chem. Rev., 2016, 116, 11840–11876 CrossRef PubMed.
- T. M. McDonald, W. R. Lee, J. A. Mason, B. M. Wiers, C. S. Hong and J. R. Long, Capture of Carbon Dioxide from Air and Flue Gas in the Alkylamine-Appended Metal–Organic Framework mmen-Mg2(dobpdc), J. Am. Chem. Soc., 2012, 134, 7056–7065 CrossRef CAS PubMed.
- P. J. Milner, J. D. Martell, R. L. Siegelman, D. Gygi, S. C. Weston and J. R. Long, Overcoming double-step CO2 adsorption and minimizing water co-adsorption in bulky diamine-appended variants of Mg2(dobpdc), Chem. Sci., 2018, 9, 160–174 RSC.
- E. J. Kim, R. L. Siegelman, H. Z. H. Jiang, A. C. Forse, J.-H. Lee, J. D. Martell, P. J. Milner, J. M. Falkowski, J. B. Neaton, J. A. Reimer, S. C. Weston and J. R. Long, Cooperative carbon capture and steam regeneration with tetraamine-appended metal–organic frameworks, Science, 2020, 369, 392–396 CrossRef CAS PubMed.
- T. M. McDonald, J. A. Mason, X. Kong, E. D. Bloch, D. Gygi, A. Dani, V. Crocellà, F. Giordanino, S. O. Odoh, W. S. Drisdell, B. Vlaisavljevich, A. L. Dzubak, R. Poloni, S. K. Schnell, N. Planas, K. Lee, T. Pascal, L. F. Wan, D. Prendergast, J. B. Neaton, B. Smit, J. B. Kortright, L. Gagliardi, S. Bordiga, J. A. Reimer and J. R. Long, Cooperative insertion of CO2 in diamine-appended metal-organic frameworks, Nature, 2015, 519, 303–308 CrossRef CAS PubMed.
- E. J. Kim, R. L. Siegelman, H. Z. H. Jiang, A. C. Forse, J.-H. Lee, J. D. Martell, P. J. Milner, J. M. Falkowski, J. B. Neaton, J. A. Reimer, S. C. Weston and J. R. Long, Cooperative carbon capture and steam regeneration with tetraamine-appended metal–organic frameworks, Science, 2020, 369, 392–396 CrossRef CAS PubMed.
- R. L. Siegelman, T. M. McDonald, M. I. Gonzalez, J. D. Martell, P. J. Milner, J. A. Mason, A. H. Berger, A. S. Bhown and J. R. Long, Controlling Cooperative CO2 Adsorption in Diamine-Appended Mg2(dobpdc) Metal–Organic Frameworks, J. Am. Chem. Soc., 2017, 139, 10526–10538 CrossRef CAS PubMed.
- J. G. Vitillo and S. Bordiga, Increasing the stability of Mg2(dobpdc) metal–organic framework in air through solvent removal, Mater. Chem. Front., 2017, 1, 444–448 RSC.
- M. N. Dods, S. C. Weston and J. R. Long, Prospects for Simultaneously Capturing Carbon Dioxide and Harvesting Water from Air, Adv. Mater., 2022, 34, 2204277 CrossRef CAS PubMed.
|
This journal is © The Royal Society of Chemistry 2023 |