DOI:
10.1039/D2SC05377B
(Edge Article)
Chem. Sci., 2023,
14, 950-954
A bioinspired, one-step total synthesis of peshawaraquinone†
Received
27th September 2022
, Accepted 20th December 2022
First published on 21st December 2022
Abstract
A concise synthesis of a stereochemically complex meroterpenoid, peshawaraquinone, via the unsymmetrical dimerization of its achiral precursor, dehydro-α-lapachone, is reported. Enabled by reversible oxa-6π-electrocyclizations of 2H-pyran intermediates, the base-catalyzed dimerization sets up an intramolecular (3 + 2) cycloaddition, with the formation of six stereocenters during the cascade. Combining the generation and in situ dimerization of dehydro-α-lapachone allows a one-step total synthesis of peshawaraquinone from lawsone and prenal.
Introduction
Dimerization occurs frequently in the biosynthesis of complex natural products, and this has often been exploited in the field of biomimetic total synthesis.1 However, unsymmetrical dimerizations of achiral intermediates that form stereochemically complex natural products (i.e. those with multiple stereocenters) are rare.2 A famous example is Chapman's total synthesis of carpanone via an unsymmetrical, oxidative dimerization of a simple phenol that constructs five stereocenters and two rings in a single step (Fig. 1).3
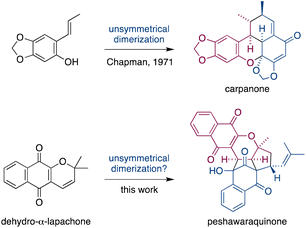 |
| Fig. 1 Unsymmetrical dimerizations of achiral intermediates in Chapman's biomimetic synthesis of carpanone, and in our proposed synthesis of peshawaraquinone. | |
Herein, we propose that a complex meroterpenoid, peshawaraquinone, is biosynthesized via an unsymmetrical dimerization of dehydro-α-lapachone, a naturally occurring naphthoquinone. Peshawaraquinone was isolated from the heartwood of Fernandoa adenophylla,4 a flowering tree widely used in traditional medicine, alongside the more common dehydro-α-lapachone.5 Its elaborate, polycyclic structure featuring six stereocenters and seven rings was elucidated by NMR and X-ray crystallographic studies. A detailed mechanistic proposal for the biosynthesis of peshawaraquinone is outlined in Scheme 1. First, dehydro-α-lapachone is formed by oxidative cyclization of lapachol,6 a historically significant meroterpenoid first isolated by Paternò in 1882 and later revised in structure by Hooker,7 who also used lapachol as the original substrate for his eponymous oxidation.8 Next, retro-oxa-6π-electrocyclization of dehydro-α-lapachone gives a reactive 1-oxatriene intermediate 1. Similar electrocyclic ring opening of 2H-pyrans are known to be thermodynamically accessible,9 and dehydro-α-lapachone itself has been shown to isomerize via Lewis acid catalyzed retro-6π-electrocyclization.10 Deprotonation of 1-oxatriene 1 to give an extended enolate 2 then sets up the key unsymmetrical dimerization via an intermolecular bisvinylogous Michael reaction to form the first dimeric intermediate 3,11 while regenerating a 1-oxatriene motif that undergoes an oxa-6π-electrocyclization to give 2H-pyran 4. We predict that the oxa-6π-electrocyclization of 3 is unlikely to be highly diastereoselective (i.e. torqueselective), so that 4 should be formed as a mixture of diastereomers that differ in their relative configurations at C-13 and C-11′. Next, a concerted but asynchronous, intramolecular (3 + 2) cycloaddition between the hydroxynaphthoquinone and the 2H-pyran of 4 gives peshawaraquinone.12 Our biosynthetic proposal therefore also suggests that the diastereomer 11′-epi-peshawaraquinone could be a previously unrecognized natural product.13 While mechanistically related intramolecular (3 + 2) cycloadditions of hydroxynaphthoquinones are synthetically known, the functionalization of a 2,2-dimethyl-2H-pyran via a retro-oxa-6π-electrocyclization, deprotonation, alkylation and oxa-6π-electrocyclization is unprecedented. We further speculated that the unsymmetrical dimerization of dehydro-α-lapachone is unlikely to be enzyme catalyzed in nature, and that 3 and all subsequent chiral compounds in the pathway are therefore formed as racemates.14 No optical rotation data for natural peshawaraquinone was reported, but an image of the structure in a biological activity paper4b indicates that it crystallized in the centrosymmetric monoclinic space group C2/c and it is therefore racemic.15
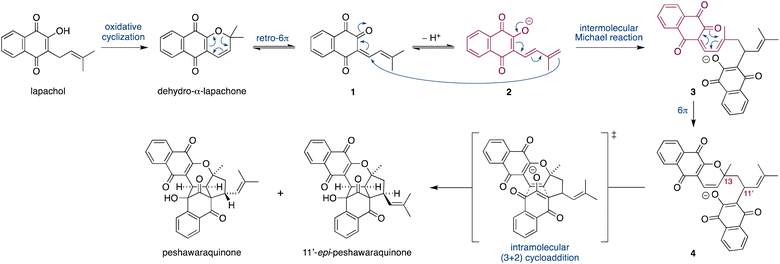 |
| Scheme 1 Proposed biosynthesis of peshawaraquinone via the dimerization of dehydro-α-lapachone. | |
Results and discussion
To investigate the chemical feasibility of our biosynthetic hypothesis, we conducted a biomimetic synthesis of peshawaraquinone (Scheme 2). First, dehydro-α-lapachone was synthesized by Knoevenagel condensation of lawsone with prenal and subsequent oxa-6π-electrocyclization. This reaction was conveniently carried out on a 10 g scale using Lee's “on-water” procedure.16 After extensive screening of acid/base catalysts and thermal/photochemical conditions for the dimerization of dehydro-α-lapachone, we found that tertiary amine bases in PhMe at high temperature mediated efficient conversion to a mixture of peshawaraquinone and 11′-epi-peshawaraquinone.17 For example, heating dehydro-α-lapachone in PhMe at reflux in the presence of one equivalent of N,N-diisopropylethylamine (DIPEA) gave peshawaraquinone and its C-11′-epimer in 54% combined yield (1
:
2.2 d.r. in favor of the epimer) after flash column chromatography with neat CH2Cl2 as the eluent. Alternatively, use of 4-dimethylaminopyridine (DMAP) as the base gave a 2.2
:
1 d.r. in favor of the natural product, in 46% combined yield. The nature of the ammonium cation therefore appears to subtly influence the diastereoselectivity of the oxa-6π-electrocyclization of the dimeric intermediate 3. The dimerization did not proceed to full conversion, with dehydro-α-lapachone starting material typically recovered in 10–15% yield. NMR spectra of the crude reaction products are clean, showing product formation and unreacted starting material as the only compounds present in significant amounts.18 Both reactions were conducted on multi-gram scale. Analytical samples of peshawaraquinone and the epimer were obtained by preparative thin layer chromatography or by repeated flash column chromatography with hexane-CH2Cl2. From the DMAP-catalyzed dimerization, pure peshawaraquinone was obtained in 20% isolated yield. NMR spectra for synthetic peshawaraquinone fully matched the isolation data, while the structure of 11′-epi-peshawaraquinone was proven by single crystal X-ray crystallography.19 A trace of 11′-epi-peshawaraquinone can be observed in the NMR spectrum of isolated peshawaraquinone,4b which supports our hypothesis that both compounds are natural products derived from dimerization of dehydro-α-lapachone. Although the synthesis of peshawaraquinone was conducted in PhMe at reflux to maximize conversion, some product formation was observed at room temperature (e.g. 3% formation of the natural product on treating dehydro-α-lapachone with DMAP in PhMe at rt for 24 h).
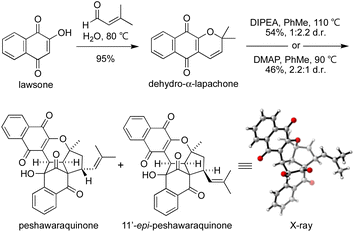 |
| Scheme 2 Dimerization of dehydro-α-lapachone. | |
Given the success of our two-step approach, we also investigated a one-step total synthesis of peshawaraquinone (Scheme 3). Using DMAP in PhMe at 110 °C, lawsone and a slight excess of prenal were converted into a 2.6
:
1 mixture of peshawaraquinone and its C-11′ epimer in 17% combined yield, with an 8% yield of the pure natural product obtained by repeated flash column chromatography. Although low yielding, this reaction meets several requirements of an “ideal synthesis” as stated by Wender,20 with a one-step total synthesis of a complex target from inexpensive, readily available starting materials under simple conditions. The four-component cascade reaction generates six bonds and six stereocenters in one step, enabled by the conversion of six trigonal planar carbon atoms in the reactants to six tetrahedral centers in the product.
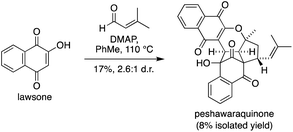 |
| Scheme 3 One-step total synthesis of peshawaraquinone. | |
To gain further insight into the mechanism of the synthesis and biosynthesis of peshawaraquinone, molecular geometries and energies of proposed intermediates were calculated by density functional theory (DFT) using the ORCA quantum chemistry software package (version 5.0.3).21 Geometries were optimized in the gas phase using the ωB97X-D3 functional22 and def2-SVP basis set.23 Transition states were obtained using a combination of the climbing image nudged elastic band method and an eigenvector-following optimization of the climbing image.24 Single-point energy calculations of the optimized geometries were also carried out using the same functional with the def2-TZVPD basis set25 and the SMD continuum solvent model26 with toluene as the solvent. Firstly, our calculations show that retro-oxa-6π-electrocyclization of dehydro-α-lapachone is thermally accessible but with ΔG‡ = 102.3 kJ mol−1 that is probably rate-limiting for the overall cascade.27 Bond rotation required to convert the initially formed s-cis conformation of 1-oxatriene 1 to the more stable s-trans conformation is much faster than the retro-oxa-6π-electrocyclization, so any subsequent dimerization reactions probably involve 1 (s-trans) (Scheme 4).
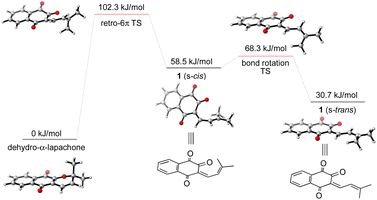 |
| Scheme 4 Computational analysis of the thermal retro-oxa-6π-electrocyclization of dehydro-α-lapachone. | |
Given the number of possible alkene configurations and the conformational flexibility of both 1-oxatriene 1 and extended enolate 2, the precise mechanism of the dimerization was not modelled. Instead, we calculated a complex series of bond rotations that convert the favored s-trans conformation of the unsymmetrical dimer 3 to the sterically disfavored s-cis conformation required to set up the exergonic oxa-6π-electrocyclization leading to 4, with an overall ΔG‡ = 91.4 kJ mol−1.28 Finally, the intramolecular (3 + 2) cycloaddition of 4 was calculated to be a concerted but asynchronous process, to give the alkoxide anion of peshawaraquinone which is then favorably protonated to complete the cascade (Scheme 5). Although one bond is more fully formed, there is only a single transition state in the conversion of 4 into the alkoxide anion of peshawaraquinone, so the mechanism does not appear to be a stepwise sequence of intramolecular Michael and aldol reactions. The calculated reaction profile leading to 11′-epi-peshawaraquinone via a stereodivergent oxa-6π-electrocyclization of 3 is very similar in energy, thus rationalizing the formation of both diastereomers in our biomimetic synthesis.
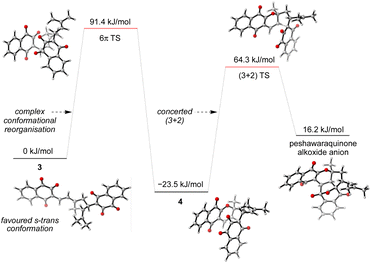 |
| Scheme 5 Computational analysis of the oxa-6π-electrocyclization and concerted (3 + 2) cycloaddition steps. | |
Conclusions
In summary, we have discovered a remarkably simple synthesis of a complex meroterpenoid, peshawaraquinone, via the unsymmetrical dimerization of a 2H-pyran monomer. Our work highlights the power of biomimetic synthesis to interrogate biosynthetic pathways, while also assisting in the discovery of new natural products. In addition to the rapid generation of stereochemical complexity, this biomimetic cascade features a novel alkylation of seemingly inert methyl group of the 2,2-dimethyl-2H-pyran of dehydro-α-lapachone that is facilitated by reversible oxa-6π-electrocyclic reactions.29
Data availability
All experimental procedures, spectral data and computational calculations are available in the ESI.†
Author contributions
The manuscript was written through contributions of all authors. All authors have given approval to the final version of the manuscript.
Conflicts of interest
There are no conflicts to declare.
Acknowledgements
We thank the Australian Research Council (DP200102964 and LE210100163), the University of Adelaide and the University of Edinburgh for funding this work. This research was undertaken with the assistance of resources and services from the National Computational Infrastructure (NCI), which is supported by the Australian Government.
Notes and references
- J. Sun, H. Yang and W. Tang, Chem. Soc. Rev., 2021, 50, 2320 RSC.
- For selected examples of unsymmetrical dimerizations of achiral intermediates in biomimetic synthesis, see:
(a) J.-P. Lumb and D. Trauner, J. Am. Chem. Soc., 2005, 127, 2870 CrossRef CAS PubMed;
(b) D. Ma, Y. Liu and Z. Wang, Angew. Chem., Int. Ed., 2017, 56, 7886 CrossRef CAS PubMed;
(c) J. Huang, Y. Gu, K. Guo, L. Zhu, Y. Lan, J. Gong and Z. Yang, Angew. Chem., Int. Ed., 2017, 56, 7890 CrossRef CAS PubMed;
(d) J. Feng, X. Lei, Z. Guo and Y. Tang, Angew. Chem., Int. Ed., 2017, 56, 7895 CrossRef CAS PubMed;
(e) X. Long, Y. Huang, Y. Long and J. Deng, Org. Chem. Front., 2018, 5, 1152 RSC;
(f) I. De Silvestro, S. L. Drew, G. S. Nichol, F. Duarte and A. L. Lawrence, Angew. Chem., Int. Ed., 2017, 56, 6813 CrossRef CAS PubMed;
(g) J. S. Bestwick, D. J. Jones, H. E. Jones, P. G. Kalomenopoulos, R. Szabla and A. L. Lawrence, Angew. Chem., Int. Ed., 2022, 61, e202207004 CrossRef CAS PubMed.
- O. L. Chapman, M. R. Engel, J. P. Springer and J. C. Clardy, J. Am. Chem. Soc., 1971, 93, 6696 CrossRef CAS.
-
(a) Z. A. Shah and M. R. Khan, Rec. Nat. Prod., 2015, 9, 169 Search PubMed;
(b) F. A. Alhumaydhi, A. S. M. Aljohani, U. Rashid, Z. A. Shah, A. Rauf, N. Muhammad, Y. S. Al-Awthan and O. S. Bahattab, ACS Omega, 2021, 6, 996 CrossRef CAS PubMed.
- S. C. Hooker, J. Am. Chem. Soc., 1936, 58, 1190 CrossRef CAS.
- E. Paterno, Gazz. Chim. Ital., 1882, 12, 337 Search PubMed.
- S. C. Hooker, J. Chem. Soc., Trans., 1896, 69, 1355 RSC.
- S. C. Hooker, J. Am. Chem. Soc., 1936, 58, 1168 CrossRef CAS.
- For reviews of oxa-6π-electrocyclizations in natural product synthesis, see:
(a) C. M. Beaudry, J. P. Malerich and D. Trauner, Chem. Rev., 2005, 105, 4757 CrossRef CAS PubMed;
(b) S. P. Roche, Organics, 2021, 2, 376 CrossRef CAS. For selected examples of retro-oxa-6π-electrocyclizations in biomimetic cascade reactions, see:
(c) M. J. Begley, L. Crombie, D. A. Slack and D. A. Whiting, J. Chem. Soc., Perkin Trans. 1, 1977, 2402 RSC;
(d) J. P. Malerich, T. J. Maimone, G. I. Elliott and D. Trauner, J. Am. Chem. Soc., 2005, 127, 6276 CrossRef CAS PubMed;
(e) C. Qi, Y. Xiong, V. Eschenbrenner-Lux, H. Cong and J. A. Porco Jr, J. Am. Chem. Soc., 2016, 138, 798 CrossRef CAS PubMed;
(f) L. A. M. Murray, T. Fallon, C. J. Sumby and J. H. George, Org. Lett., 2019, 21, 8312 CrossRef CAS PubMed;
(g) A. J. Day, C. J. Sumby and J. H. George, J. Org. Chem., 2020, 85, 2103 CrossRef CAS PubMed;
(h) B. Lockett-Walters, S. Thuillier, E. Baudouin and B. Nay, Org. Lett., 2022, 24, 4029 CrossRef CAS PubMed.
- R. Inagaki, M. Ninomiya, K. Tanaka and M. Koketsu, ChemMedChem, 2015, 10, 1413 CrossRef CAS PubMed.
- C. Curti, L. Battistini, A. Sartori and F. Zanardi, Chem. Rev., 2020, 120, 2448 CrossRef CAS PubMed.
- For some related organocatalytic, formal (3 + 2) cycloadditions of lawsone derivatives, see:
(a) D. B. Ramachary, M. A. Pasha and G. Thirupathi, Angew. Chem., Int. Ed., 2017, 56, 12930 CrossRef CAS PubMed;
(b) S. Peraka, M. A. Pasha, G. Thirupathi and D. B. Ramachary, Chem.–Eur. J., 2019, 25, 14036 CrossRef CAS PubMed;
(c) M. A. Pasha, S. Peraka and D. B. Ramachary, Chem.–Eur. J., 2021, 27, 10563 CrossRef PubMed. For selected examples of (4 + 2) cycloadditions of 2H-pyrans in biomimetic synthesis, see:
(d) C. Li, E. Lobkovsky and J. A. Porco Jr, J. Am. Chem. Soc., 2000, 122, 10484 CrossRef CAS;
(e) M. Shoji, J. Yamaguchi, H. Kakeya, H. Osada and Y. Hayashi, Angew. Chem., Int. Ed., 2002, 41, 3192 CrossRef CAS;
(f) G. Mehta and S. C. Pan, Org. Lett., 2004, 6, 3985 CrossRef CAS PubMed.
- B. E. Hetzler, D. Trauner and A. L. Lawrence, Nat. Rev. Chem., 2022, 6, 170 CrossRef.
- A. J. E. Novak and D. Trauner, Trends Chem., 2020, 2, 1052 CrossRef CAS.
- T. Rekis, A. Berzins, L. Orola, T. Holczbauer, A. Actins, A. Seidel-Morgenstern and H. Lorenz, Cryst. Growth Des., 2017, 17, 1411 CrossRef CAS.
- E. J. Jung, B. H. Park and Y. R. Lee, Green Chem., 2010, 12, 2003 RSC.
- See the ESI† for full details of reaction screening and optimization of the dimerization.
- See the ESI† for a representative 1H NMR spectrum of the crude reaction mixture.
- CCDC 2179170 contains the ESI† crystallographic data for this paper.
- P. A. Wender, Nat. Prod. Rep., 2014, 31, 433 RSC.
-
(a) F. Neese, WIREs Computational Molecular Science, 2012, 2, 73 CrossRef CAS;
(b) F. Neese, F. Wennmohs, U. Becker and C. Riplinger, J. Chem. Phys., 2020, 152, 224108 CrossRef CAS PubMed.
- Y.-S. Lin, G.-D. Li, S.-P. Mao and J.-D. Chai, J. Chem. Theory Comput., 2013, 9, 263 CrossRef CAS PubMed.
- F. Weigand and R. Ahlrichs, Phys. Chem. Chem. Phys., 2005, 7, 3297 RSC.
- V. Ásgeirsson, B. O. Birgisson, R. Bjornsson, U. Becker, F. Neese, C. Riplinger and H. Jónsson, J. Chem. Theory Comput., 2021, 17, 4929 CrossRef PubMed.
- D. Rappaport and F. Furche, J. Chem. Phys., 2010, 133, 134105 CrossRef PubMed.
- A. V. Marenich, C. J. Cramer and D. G. Truhlar, J. Phys. Chem. B, 2009, 113, 6378 CrossRef CAS PubMed.
- This value correlates with previous calculations of a thermal retro-oxa-6π-electrocyclization: L. M. Bishop, M. Winkler, K. N. Houk, R. G. Bergman and D. Trauner, Chem.–Eur. J., 2008, 14, 5405 CrossRef CAS PubMed.
- See the ESI† for a stepwise analysis of this conformational reorganization, alongside full details of all our computational modelling.
- While this manuscript was in preparation, the Hao group reported a more stepwise, bioinspired approach to peshawaraquinone:
(a)
H. Guo, L. Ren, D. Yuanhao, R. Zhang, T. Li, A. Rauf, W. Zhou and H.-D. Hao, ChemRxiv, 2022, preprint, DOI:10.26434/chemrxiv-2022-drjln. For our earlier preprint, see:
(b)
T. Vieira de Castro, D. M. Huang, C. J. Sumby, A. L. Lawrence and J. H. George, ChemRxiv, 2022, preprint, DOI:10.26434/chemrxiv-2022-tlczl.
|
This journal is © The Royal Society of Chemistry 2023 |
Click here to see how this site uses Cookies. View our privacy policy here.