Learning to teach chemical bonding: a framework for preservice teacher educators
Received
16th February 2022
, Accepted 13th November 2022
First published on 21st November 2022
Abstract
Chemical bonding is an important topic which is difficult to teach well, especially for novices. This study set out to support preservice teacher educators by developing a framework for understanding and addressing the complexity of teaching chemical bonding. A model of pedagogical content knowledge (PCK) in science education that has been widely endorsed by experts in the field was adopted as the theoretical lens. First, a systematic literature review was performed to articulate what recent empirical studies can tell us about the knowledge teachers require to teach chemical bonding. The review corpus consisted of 59 articles published over the past 20 years relating to four components of PCK: knowledge of how chemical bonding is embedded in curricula, knowledge of student understanding related to this topic, knowledge of instructional strategies and representations for teaching chemical bonding, and knowledge related to the assessment of chemical bonding understanding. This yielded current collective PCK for teaching chemical bonding in chemistry teacher education. Next, nine chemistry teacher educators were asked to portray their own personal knowledge for teaching chemical bonding through content representations. Analysis of the content representations revealed differences between several aspects of the collective PCK derived from the literature and the personal PCK articulated by our sample of Dutch chemistry teacher educators. Finally, findings from the literature and the teacher educator content representations were synthesized into a framework for chemistry teacher educators. Uses of the framework are discussed, and sample applications to the design of classroom activities are offered. Finally, implications of the findings and suggestions for future research are addressed.
Introduction
“Educational research is, after all, never simply research on education but always in some sense also research for education” (Biesta, 2007, p. 299, emphasis in the original). Given the goals of serving educational practice through research and vice versa, the need to understand and attend to research–practice connections has been acknowledged for decades (Kennedy, 1997; Broekkamp and van Hout-Wolters, 2007; Farley-Ripple et al., 2018). The present study demonstrates one way in which research can serve practice in relation to chemical bonding.
The important topic of chemical bonding starts early in most chemistry curricula and returns throughout many other chemistry topic areas. Yet many high school students worldwide struggle to understand it well. Teaching chemical bonding is difficult because singular, simplistic approaches yield misconceptions (Nahum et al., 2007). Rather, bonding must be addressed often, in different contexts, and with pedagogical tools that vary depending on the situation. This is particularly challenging for preservice teachers who themselves may still be learning about content and have had limited opportunity to develop a pedagogical repertoire. With the ultimate goal of supporting novice chemistry teachers in understanding the complexity of teaching chemical bonding, this study developed a framework for use in teacher education. Specifically, the framework provides an organized overview of challenges and guidelines, as well as some specific examples, when teaching chemical bonding. Discussing this framework with preservice teachers can help them to structure their knowledge on the challenges of teaching chemical bonding.
Theoretical frame: PCK
One area of scholarship which naturally involves research–practice connections and is relevant to teaching nearly any topic is that of pedagogical content knowledge (PCK). The importance of PCK was first described by Shulman (1986, 1987). He argued that both content knowledge and pedagogical knowledge are important for teachers, and that an amalgam of these two forms of knowledge is important for teaching specific topics. Since then, many aspects and sources of PCK have been described. Several models have been developed to show PCK development (Grossman, 1990; Geddis and Wood, 1997; Magnusson et al., 1999; Rollnick et al., 2008), each highlighting different social and cultural aspects of teacher knowledge. More recently, researchers have re-examined the concept of PCK (e.g., Berry et al., 2015) and developed new models (Gess-Newsome, 2015). In fact, an entire edited volume has been dedicated to new insights on PCK for teaching science (Hume et al., 2019).
Among other contributions, the aforementioned volume presents the Refined Consensus Model of PCK (Carlson et al., 2019), which “gives deep insight into the thinking of the world's leading researchers on the nature of PCK for science teaching” (Tepner and Sumfleth, 2019, p. 319). It is based on discussions held at the (2016) second PCK summit in science teacher education as well as on the findings of an extensive literature review which provided the rationale for the new model. The literature review, summit discussions, and resulting model attend to “the diverse ways in which the PCK concept is used, interpreted and investigated within the science education research community” (Hume et al., 2019, p. 4). Because the review and the new model were used to frame the present study, we describe two features of PCK which have been addressed in both the review and the model: PCK layers and PCK components.
PCK layers
Experts agree that PCK is personal (i.e. idiosyncratic) as well as shared (i.e. broadly held). To represent this variety, the Refined Consensus Model identifies three distinct realms of PCK, which can be visualized concentrically from the inside outward. At the center is enacted PCK (ePCK), which consists of specific knowledge and skills as utilized by a teacher in a particular setting to achieve particular student outcomes. Surrounding the center is personal PCK (pPCK), an individual's knowledge and expertise in a given subject area, resulting from the cumulative experiences with and contributions from students, peers and others. The third layer, referred to as collective PCK (cPCK), represents the knowledge held by a group of people and considered generalizable to some degree. For this reason, the cPCK layer is situated outside of the learning context (e.g. during class with a particular cohort of students). As teachers develop professionally, bi-directional knowledge exchanges take place between the layers in the model, reflecting individual as well as collective influences. Because it is public and sharable, cPCK is particularly well-suited to conversations among professionals, including both researchers and practitioners. Also, it is important to note that in all PCK layers three grain sizes can be recognized: discipline-, topic- and concept-specific knowledge (Mavhunga, 2019). Finally, the outermost layer of the model portrays five broader professional knowledge bases: science content knowledge, pedagogical knowledge, knowledge of students, curricular knowledge, and assessment knowledge. “Without these knowledge bases, teachers’ PCK is quite limited” (Carlson et al., 2019, p. 92).
Research has demonstrated that preservice teacher development of cPCK is informed by broader professional knowledge bases (Gess-Newsome, 2015), and that cPCK also plays a major role in the development of pPCK and ePCK (Sorge et al., 2019). This points to the importance of cPCK for beginning teachers. Moreover, understanding and supporting cPCK is crucial for teacher educators because their work strongly impacts the quality of chemistry teachers and therefore the quality of chemistry teaching and learning in schools. Further, to be better positioned to serve preservice teachers, it is likely to be useful for teacher educators to articulate their pPCK and examine its connections with cPCK.
PCK components
In their review of PCK, Chan and Hume (2019) answered several research questions, including the following: “How do the studies conceptualise individual science teachers’ PCK?” Their data revealed that, in 89% of the studies, PCK was treated as a distinct category of knowledge and conceptualized as being composed of components. The two most commonly agreed-upon and investigated PCK components were knowledge of students’ understanding and knowledge of instructional strategies and representations. About half of the studies included knowledge of assessment and knowledge of curriculum. Interestingly, they found that the affective component, teacher orientations to teaching science, which has been included in several highly-cited works (van Driel et al., 1998; Magnusson et al., 1999; Park and Oliver, 2008), was found in only about one-third of the studies in the Chan and Hume review (30 out of 88) and is not explicitly featured in the refined consensus model diagram or explanatory text. The four PCK components which, according to the model, are present across ePCK, pPCK and cPCK are described next.
Teachers need knowledge of curriculum – how things are structured, what the learning goals are, and how a specific topic (e.g. chemical bonding) fits into the envisioned learning pathways and in the overall curriculum. When teaching chemical bonding, teachers need to know which related concepts students have learned in previous years (e.g. molecules, atoms and ions), which concepts (e.g. ionic bonding, covalent bonding and metallic bonding) must be learned according to the formal curriculum, especially when assessment is a driving force, and which curriculum resources are available for learning these (e.g. textbooks, videos and demonstrations) (Magnusson et al., 1999).
It is essential that teachers have knowledge of student understanding, which includes the topics and concepts that learners experience as difficult, as well as what learners bring to class in terms of previously learned knowledge, including pre- and alternative conceptions. While preconceptions pertain to conceptions formed before learning about the scientific explanation, alternative conceptions can be described as “frameworks of understanding that are at odds with accepted knowledge” (Taber, 2001, p. 161). The term ‘misconceptions’ has a similar meaning and is also frequently used in the scientific literature (e.g.Vrabec and Prokša, 2016).
Teacher knowledge of instructional strategies (e.g. cooperative learning, problem-based learning, concept mapping and practical work) and representations (e.g. models, illustrations, simulations, examples and analogies) can help students understand concepts and the relationships between concepts. This includes what is commonly referred to in the chemistry education literature as the symbolic level. Suitable representations can help students to connect micro and macro levels, and hence influence their ability to use structure–property relationships for their understandings in chemistry (Gilbert and Treagust, 2009). Gilbert and Justi (2016) argue that the following representation types are particularly important in science education: gestural, concrete, static visual (pictures, diagrams, graphs, and mathematical and chemical equations), dynamic visual (drama, animation and simulation), oral and auditory. Teachers need to know and understand the differences in available representations for specific concepts, and be aware of their relative strengths and weaknesses, in order to determine which representation might suit a particular situation best (Magnusson et al., 1999).
Finally, knowledge of assessment refers to the understanding of what to assess and how that can be accomplished. It is essential for learning (Black and Wiliam, 1998), and to use this knowledge well, teachers require a repertoire of assessment strategies (e.g. formative and summative, formal and informal, paper and pencil test, practical tests, oral examination and portfolios). Further, they need information about and access to instruments (e.g. teacher–student dialogues in the classroom and open or multiple-choice tests) for assessing student understanding related to the topic at hand (Van der Kleij et al., 2015; Kippers et al., 2018).
Problem statement
Existing literature does describe the aforementioned four PCK components with regard to chemical bonding, but primarily in isolation. That is, individual studies are more often focused on single components rather than combinations of components or the relations between them. Similarly, outstanding resources are already available for student misconceptions, such as Kind's (2004) review covering eleven concepts or Barke et al., (2008) book entitled, “Misconceptions in Chemistry” – both of which include attention to chemical bonding. However, the evidence base for many recommendations is not always articulated. Moreover, very few sources examine all four of the aforementioned PCK components, despite the fact that experts have articulated the importance of attending to the interaction between them (Mavhunga, 2019; Park and Suh, 2019). Finally, of existing resources for (learning about) teaching chemical bonding, very few strike a productive balance between “simplifying sufficiently to suit the learners’ present purposes, but not oversimplifying to undermine their future needs” (Nahum et al., 2007, p. 585).
A comprehensive and compelling review of research findings constitutes a necessary but not sufficient pathway to understanding priorities for learning about teaching chemical bonding. If the professionals involved in research–practice connections are taken seriously, then expecting practitioners to mechanistically apply research insights would represent an impoverished view of the teaching profession. This may explain why investigation of teacher educator practices has challenged the conception of demanded professionalism (Vanassche and Kelchtermans, 2015), which asserts that teacher educators must be informed of and skilled in applying theories and principles that have been defined elsewhere. Indeed, given that crucial dimensions of teaching practice are often marginalized in the dominant research agendas of evidence-based teacher education, the field requires a focus not only on what evidence tells us but also on the beliefs and assumptions that underpin practice (Vanassche and Berry, 2020). In other words, “If PCK as a construct is to be meaningful in science teachers’ work, we would argue that it is important for concrete examples of PCK to be articulated and documented so that teachers can access and use them in shaping their own practice” (Loughran et al., 2012, p. 15).
Aim of the study
As argued above, existing sources of cPCK are available as separate publications but have rarely been synthesized in ways that also reveal their empirical justification. To the best of our knowledge, this applies to literature that can inform the teaching of chemical bonding to preservice teachers. This is unfortunate, given that published cPCK has the potential to provide a guide of canonical best-practice professional and pedagogical information (Carpendale and Hume, 2019). Further, the sensemaking process which connects cPCK to pPCK (or vice versa) inherently involves contextualized experience and judgment. It therefore seems important to examine both topic-specific cPCK and pPCK together. Thus, the following research question guided the study:
What are the characteristics of a framework about teaching chemical bonding that can support teacher education?
This study endeavors to synthesize insights from research and experience in ways that help understand and support chemistry teacher education. By combining the results of a systematic literature review and teacher educator content representations, we provide a valuable window into the key dimensions of Dutch chemistry teacher educator practical wisdom related to chemical bonding. Taken together, this yields a framework that can be used to support preservice education about teaching chemical bonding.
Methods
To answer the research question, two phases of data collection and analysis were undertaken. First, a systematic literature review, based upon the procedure by Petticrew and Roberts (2006), was conducted. This yielded a valuable inventory of insights from empirical research literature about cPCK for chemical bonding. Analysis of the literature review findings yielded eight guidelines and three overarching challenges. Second, the Dutch 10th grade chemical bonding pPCK of nine chemistry teacher educators was inventoried through content representations following the procedure reported by Loughran et al. (2012). The pPCK of the teacher educators was then compared to the guidelines distilled from the literature. Both sets of findings were then synthesized to create a framework to support teacher education on this topic.
Systematic literature review
For this study, four databases were selected: Web of Science, Scopus, ERIC and PsycINFO. The following search query was used: ((bonding) AND (attitude* OR view* OR perception* OR *conception* OR sequence OR teach* OR learn* OR “conceptual development” OR “conceptual understanding” OR transfer* OR represent* OR coherence) AND (secondary OR “high school” OR “junior high” OR “middle school”)). The category reduction “Education Educational Research” and “Education Scientific disciplines” in Web of Science and the category reduction “Social Sciences” in Scopus were applied. In ERIC and PsycINFO no category reduction was feasible. After removing duplicates, the search resulted in 582 unique entries published in the last 20 years. We chose to limit our study to the past 20 years because around 20 years ago PCK became more prominent and structured in chemistry education research.
These results were initially filtered by screening the titles, to remove unrelated papers, e.g. articles related to social bonding in schools. The following broad criteria were applied: it is about chemistry and it is about secondary or tertiary education (excluding graduate level). All titles not providing clarity were retained. Next, only articles written in the English language and published in journals that are on the “ISI master journal list” were included. These screening criteria resulted in 104 journal articles. Subsequently, the abstracts of these articles were screened applying the following criteria: the abstract or keywords are about chemical bonding, and the abstract shows that the article potentially contains one or more of the previously described PCK components. During abstract screening, exclusion of the articles turned out to be mainly based on the criterion “topic.” For instance, articles on acid and base chemistry and nuclear magnetic resonance were excluded. Only a few lacked the “potential to contain PCK.” For example, some articles described engaging activities, but nothing about student learning nor the commensurate teacher knowledge required to enact them. This abstract screening resulted in 89 articles.
Thereafter, the full texts were obtained and screened using the same screening criteria. Full-text screening mostly excluded articles that only described the execution of newly developed practicals, models, or exercises. The final step in selecting relevant studies was an appraisal of the scientific quality of the articles. The presence of a description of the research objectives and/or research questions, the context and participant sampling, the overall research approach and data collection strategies, and the data analyses were checked. This resulted in a total of 59 articles for the review corpus. Appendix A offers a schematic overview of the search and selection processes.
After quality appraisal of the full-text articles, data extraction was performed on the review corpus. First, meta-data were extracted: title, author(s), year of publication, journal title, country, education level, research method (qualitative, quantitative and mixed methods), research design (e.g. case study and quasi-experimental), research population (e.g. students, teachers and textbooks) and the size of research population. Next, relevant findings on PCK to answer our research question were extracted from the results, conclusion, discussion, or implications sections of the articles.
The data extracted from the article corpus were analyzed in three phases, each building on the previous. The first phase featured deductive analysis, in which all extracted data were classified according to the four PCK components: knowledge of curriculum, knowledge of students’ understanding, knowledge of instructional strategies and representations, and knowledge of assessment. To guard reliability, an initial codebook was established based on the literature. Next, two of the researchers extracted PCK components from seven randomly selected articles (>10% of the data); discussion of the resulting analyses yielded refinements in the codebook. Three of the researchers discussed the coded data until 100% consensus was reached. This yielded four sub-sets of data (one for each PCK component).
The second phase of analysis was undertaken within each sub-set of coded data. Here sub-codes related to each component were generated and refined through constant comparison (Glaser and Strauss, 1999) and categories of sub-codes were made through axial coding. This resulted in ‘key insights’ related to each PCK component. Across the four PCK components, a total of eight key insights were identified. Related to the key insights, eight corresponding guidelines were articulated. For example, related to the PCK component knowledge of curriculum, a key insight was that “Textbooks and teachers do not always use evidence-based teaching sequences,” and the corresponding guideline was, “Use evidence-based teaching sequences.”
This phase was undertaken by two of the researchers and the results were verified by a third researcher. Appendix B shows the number of articles containing data relevant to each PCK component, key insights within each component, and a sample quote for each key insight.
The third phase of analysis was inductive in nature. Here, two of the researchers examined the eight guidelines and identified cross-component themes. This process yielded three overarching challenges across the entire data set. The three challenges were verified by the entire research team.
Teacher educator content representations
Content representations (CoRes) can be used as a research tool to assess science teachers’ understanding of teaching a certain topic. To provide an overview of teacher educator pPCK about chemical bonding, CoRes were constructed individually by chemistry teacher educators. All chemistry teacher educators registered at the Dutch chemistry teacher educator network (n = 18) were personally invited by e-mail to participate in the research. Two teacher educators replied not being able to complete the CoRe (citing insufficient knowledge or lack of time as reasons). The CoRe was completed by nine Dutch chemistry teacher educators (3 female and 6 male) from 8 different universities, all of them having over five years of experience as teacher educators.
The CoRe structure was based on the template developed by Loughran et al. (2012), which prompts specific areas for consideration in relation to one or more big ideas. The big idea in this CoRe was: “A covalent bond is a shared electron pair.” The learning context that the teacher educators were asked to consider was a Dutch grade 10 (pre-university) chemistry class. The following eight prompts were given (translated from Dutch):
– Learning goals: What do you intend the students to learn about this concept? What are the learning goals?
– Importance: Why is it important for students to know (or be able to do) this?
– Curriculum later: What else do you know about this idea (that learners do not yet know)?
– Difficulties: What challenges or limitations do you run across when teaching this concept?
– Learner thinking: What knowledge about student thinking (including alternative conceptions) influences your teaching of this concept?
– Other factors: What other factors influence your teaching of this idea?
– Teaching procedures: Which materials do you use? How do you design your lesson (prior knowledge, content and activities)?
– Formative assessment: How do you ascertain learner understanding (e.g. diagnostic assessment, dialogue, etc.)?
To prepare the data, the teacher educators’ answers were compiled into a spreadsheet, which showed all the answers for each of the eight prompts. Next, the responses were deductively coded by two of the researchers using the eight guidelines for addressing challenges as given in the righthand column of Table 4 (e.g. B1 and M3). These were then cross-referenced for each respondent. Finally, the results from both the literature review and CoRes were synthesized into a framework for teaching chemical bonding. This was undertaken through discussion among three of the researchers until consensus was reached.
Results from the literature review
Portraying the corpus
The original studies (n = 59) were carried out in 17 different countries; most of them were carried out in Turkey (n = 13), USA (n = 9) and Sweden (n = 7). The journals in which articles most frequently appeared were Chemistry Education Research and Practice (n = 16) and International Journal of Science Education (n = 10). Appendix C shows which articles contained information relevant to each PCK component. The remainder of this section presents the results related to each component of PCK.
Knowledge of chemical bonding in the curriculum
Studies attending to teacher knowledge of chemical bonding in the curriculum focus on their prevalence in multiple subdomains and (textbook-based) sequences. One study notes that a challenge to teaching chemical bonding stems from the fact that these concepts are found in several domains of the curriculum (De Posada, 1999), and that links between them tend to be non-existent or extremely weak. Explicit attention must be paid to making chemical bonding visible in varied contexts so that students see multiple manifestations of the phenomena and still come to understand that the principle is the same. Also, learning progressions lead to better understanding. A study on a learning progression on molecular structures and properties (Cooper et al., 2012) indicated that a focus on structure–property relationships, encouraged by the curriculum materials (‘Chemistry, Life, the Universe, and Everything curriculum’), leads to better understanding of Lewis structures.
Even though teaching sequences in chemistry textbooks are not always evidence-based, these are still used by many teachers (Bergqvist and Chang Rundgren, 2017; Sibanda, 2018). In fact, six studies report the importance of an effective teaching sequence and strategies linked to students’ learning progression (Chen et al., 2017; Cheng and Gilbert, 2017). For this reason, it is important that teachers develop the ability to assess for themselves whether to adopt or adapt the sequences in their textbooks. In so doing, three guidelines emerge from this review. First, studies point to the need for a solid understanding of the particulate nature of matter as important prior knowledge (Othman et al., 2008). This not only supports insight into chemical bonding, but also prevents the development of alternative conceptions (Taber, 2003). Second, two studies yielded the suggestion to use a bottom-up approach when teaching chemical bonding, based on electrostatic interactions (Broman and Parchmann, 2014; Sibanda and Hobden, 2015). In particular, the former recommends that “chemical bonding be taught based on physical elemental principles by applying the idea of continuum of bond strengths and by avoiding the dichotomous classification of bonds as covalent/ionic and intra/intermolecular.” Third, in so doing, educators should “introduce a general notion of bonding, based on electrical interactions, before exploring specific bond types in detail; emphasize bonding as the interactions that hold structures together (rather than being related to developing full shells); emphasize that most elements are not atomic (and that reactants in chemical reactions do not tend to be atomic) and are therefore chemically bonded; emphasize that chemical bonding is present in reactants as well as products (so that metallic bonding is not tied to the metal reacting with another element)” (Taber, 2003, p. 753). Thus, it is important that teacher educators clarify to preservice teachers the need to ensure that students understand metallic bonding before explaining ionic bonding and, only after that, covalent bonding.
Knowledge of student understanding of chemical bonding
Numerous alternative conceptions are held by many students (Çalik, 2005; Acar and Tarhan, 2008; Schmidt et al., 2009; Kabapinar, 2013; Broman and Parchmann, 2014; Cokelez et al., 2014; Akkuzu and Uyulgan, 2016) as well as by (preservice) teachers (Kahveci, 2009; Kind and Kind, 2011; Uyulgan et al., 2014; Mutlu and Sesen, 2016). In fact, three studies show that preservice teachers hold alternative conceptions and that it is important to be aware of this (Toplis, 2008; Kind, 2014; Mutlu and Sesen, 2016). However, a study on South African chemistry teachers suggests that teachers know how students learn chemical bonding, but that alternative conceptions are thought to be not so important when teaching this topic (Sibanda, 2018). While it is often challenging to make sense of student conceptions (Harrison and Treagust, 2000), selected studies describe that the number of alternative conceptions decreases with the increase in education level (Birk and Kurtz, 1999; Coll and Treagust, 2001; Coll and Treagust, 2002; Coll and Treagust, 2003a). Additionally, studies show that simple, realistic mental models are preferred at all education levels (Coll and Treagust, 2001; Coll and Treagust, 2003b) and that different levels of understanding should not be seen as negative, but can be used by teachers to develop student understanding (Cheng and Oon, 2016). Table 1 provides the alternative conceptions for which sources were discussed and recommendations were given.
Table 1 Alternative conceptions in chemical bonding, their potential sources, and recommendations for teaching
|
Examples of alternative conception (reference) |
Potential source(s) |
Recommendations for teaching |
Underlying chemical bonding |
Students visualize the malleability of metals as the structural rearrangement of metal atoms/ions and an unspecific attractive force or ‘surrounding electrons.’ (Cheng and Gilbert, 2014) |
The transfer from a verbal statement to a visual representation. |
Prompt students to code their ideas both verbally and visually. Make explicit use of diagrams and visual representations. |
Students imagine energy as matter and hence chemical bonds have mass. (Kabapinar, 2013) |
Ontological mismatch. |
Have learners discuss the properties of matter, and then debate whether chemical bonds are matter by providing evidence for their arguments. Similarly, have learners compare the properties of matter and energy to decide whether energy is matter. |
Give an explanatory teleological meaning to the octet rule. (Joki and Aksela, 2018) |
Teachers who do not fully understand the concepts themselves. |
In addition to sharing knowledge (e.g. using the octet rule can cause misconceptions), teachers should consider the epistemological and historical backgrounds of the concepts they teach. |
About chemical bonding |
Molecules are present in ionic substances. (Vladušić et al., 2016) |
|
|
Bonding occurs when atoms share or transfer electrons to get a full outer shell (octet framework). (Taber, 2003) |
Student oversimplification in prior learning. |
Teachers should emphasize forces at the basis of chemical bonding and introduce the simplest type of bond first. |
Electrons are stationary. |
The dichotomous description of covalent and ionic bonding. |
Teach chemical bonding as a continuum. |
In ionic bonding electrons are first transferred and then become like ‘a sea of electrons’ in ionic compounds. (Luxford and Bretz, 2013) |
|
|
Students imagine that atoms have agency to explain the concept of chemical bonding on a macro level. (Cokelez et al., 2014) |
Misleading representations (of chemical bonding) in textbooks. |
Teachers should maintain their understanding of more advanced models, including hybrid ones, to help them assess the potential value of the models used in textbooks. Understanding the variety, purpose, and limitations of textbook models will help teachers make strategic choices about when and how to use each. |
In ionic bonding each sodium atom donates one electron and each chlorine atom accepts one electron. (Bergqvist and Chang Rundgren, 2017) |
|
|
|
(Cokelez et al., 2014, p. 486) |
|
Knowledge of instructional strategies and representations in teaching chemical bonding
Instructional strategies.
Six studies report positive effects on students’ understanding using cooperative learning (Acar and Tarhan, 2008; Frailich et al., 2009; Karacop and Doymus, 2013; Eymur and Geban, 2017) or group discussions (Waldrip and Prain, 2012; Warfa et al., 2014). Additionally, students experienced working in groups as positive, but also difficult (Tarhan et al., 2008). Besides group discussions, teacher–student discussions about appropriate models (Harrison and Treagust, 2000) are also important for student understanding and contribute to student reasoning. Table 2 shows cooperative activities that can improve student understanding according to the literature reviewed.
Table 2 Replicable cooperative activities that can increase student understanding
Example |
Characterization and goal |
Classroom enactment |
Cooperative learning based on conceptual change approach instruction (Eymur and Geban, 2017) |
Heterogeneous groups of four students were made based on academic achievement. The groups work on a worksheet together to learn about Lewis structures and the shapes of molecules. |
Discuss the Lewis structures and shapes of molecules of the following compounds with your group: H2O, NH3 and BF3 |
|
The teacher monitors the groups to make sure each group member is participating actively. |
Group discussions (
Warfa et al., 2014) |
Groups of three or four students were made. Each student was assigned a different role (e.g. the group's facilitator, spokesperson and recorder). Students discussed about exercises on dissolving salts. |
Students were given 3D magnetic models of sodium chloride and water molecules. Students then had to draw representations of sodium chloride, before and after mixing it with water. |
|
The teacher monitors the groups and asks scaffolding questions like “are they atoms or ions?” |
Teacher–student discussions (Harrison and Treagust, 2000) |
The teacher systematically discussed each metaphor, analogy, and model with the students. The teacher and students discussed different models of the atom and covalent bonding. |
A ‘new’ model/analogy of the atom is introduced. The teacher discusses the features of the model/analogy and the concept with the students. They look for similarities, but also for differences between the model/analogy and the concept. In the end, students and teacher discuss whether the model/analogy was useful or confusing. |
Furthermore, students using an enriched text strategy (Tsaparlis et al., 2018) or using the premise–reasoning–outcome structure to construct scientific explanations when learning about covalent, metallic and ionic bonding (Putra and Tang, 2016) performed much better on several chemical bonding concepts. Additionally, when 12th grade students were deployed as co-teachers, teachers had more insights into students’ difficulties, which resulted in improved teaching strategies (Schultze and Nilsson, 2018). One study showed that texts focused on conceptual change increased students’ understanding and made them aware of their existing conceptions (Özmen et al., 2009). Finally, contextualization, like case-based and problem-based learning, using real-life contexts offers a more effective learning environment and improves students’ understanding, attitude and motivation (Tarhan et al., 2008; Broman and Parchmann, 2014). For example, daily life events or problems (e.g. medical drugs and energy drinks) and learning materials based on relevant real-life contexts (e.g. alternative fuels) have been shown to help students see the relevance of chemistry in general, as well as to enhance higher order thinking, and to develop a positive attitude towards chemistry (Broman and Parchmann, 2014). Two studies described topic-specific strategies which showed that teaching chemical bonding based on electrostatic forces leads to improved student understanding (Joki et al., 2015; Cheng and Oon, 2016).
Representations.
Textbooks contain many static visual representations, and studies have shown that these are important sources of alternative conceptions (De Posada, 1999; Bergqvist et al., 2013). Teachers are influenced by representations in textbooks too and therefore need to think critically about their use (Bergqvist and Chang Rundgren, 2017). Selected studies emphasize that teachers need to be aware of the availability, strengths and weaknesses of representations (Bergqvist et al., 2016; Nimmermark et al., 2016; Patron et al., 2017; Wheeldon, 2017). This can be done by a teacher guide in which it is explained why a certain representation (e.g. ball-and-stick model, space-filling model, structural formula and chemical symbol) was chosen and explained what its limitations are. For instance, the ball-and-stick model, which is an example of a concrete representation, helps to understand the geometry of molecules, but shows the bonding electrons as static entities (sticks). Therefore, it is suggested to complement ball-and-stick models with space-filling representations (Nimmermark et al., 2016). Table 3 provides an overview of strengths and limitations of four different representations. Furthermore, the use of anthropomorphic models or language (e.g. atoms would “like” to have extra electrons) should be avoided, as this may cause alternative conceptions (Bergqvist et al., 2016).
Representation |
Strengths |
Limitations |
|
• Shows bond order |
• Does not account for the relative atomic size |
• Shows molecular geometry |
• Does not show all valence electrons |
• Easily visualizes the three-dimensionality of molecules |
• Availability of model kits |
• Students can build the model |
|
|
|
• Shows relative atomic size |
• Bond order is not visible |
• Shows molecular geometry |
• Does not show all valence electrons |
• Easily visualizes the three-dimensionality of molecules |
|
• Close to how a molecule looks like |
|
|
|
• Shows bond order |
• Does not account for the relative atomic size |
• Easy to draw |
• Does not show all valence electrons |
|
• Does not show molecular geometry |
|
|
• Shows all valence electrons |
• Does not show bond angles |
• Molecular geometry can be deduced (VSEPR) |
• Does not account for the relative atomic size |
|
• Does not visualize three-dimensionality |
Teachers need to realize that understanding representations can be challenging for students (Patron et al., 2017): they often require explicit support for the interpretation of representations. In addition, students need to be aware of the limitations and challenges associated with simplification and abstraction (Taber, 2003). This can be reached by discussing the properties of representations with students (Nimmermark et al., 2016). A teacher could, for instance, show different representations of a chemical bond to students and discuss which representation would be used best, and explain what its strengths and weaknesses are. Furthermore, students find it hard to understand what happens during experiments at the micro-level, and therefore a model showing the micro-level should be used simultaneously (Karacop and Doymus, 2013). One study suggests that becoming familiar with at least a simplified quantum model for the build-up of an atom helps secondary school students understand chemical bonding (Nimmermark et al., 2016), and another stresses the importance of reserving advanced chemical bonding models until advanced undergraduate level (Coll and Treagust, 2003).
Some studies combined digital support with specific strategies like those mentioned above. The use of dynamic visual representations like computer animations or applets (Frailich et al., 2007; Özmen et al., 2009; Lewis et al., 2012; Karacop and Doymus, 2013), computerized molecular modelling (Barnea and Dori, 1999), dynamic computer models (Toplis, 2008), and well-structured computer-assisted instructions (Özmen, 2008) have been shown to contribute to learning chemical bonding and to support student understanding of micro–macro relationships. Also, gestural representations, like embodied modelling, which involves students acting as particles (Langbeheim and Levy, 2018), as well as magnetic 3D models (Warfa et al., 2014), involved students feeling the interactions, and this increased student understanding.
Knowledge of assessment for learning chemical bonding
Formal assessment.
One promising type of formal assessment is two-tier testing, in which the students answer the multiple-choice questions in the first tier, and then reason why this answer is correct in the second. Five studies showed that two-tier testing can be used to determine alternative conceptions on chemical bonding concepts (Othman et al., 2008; Heredia et al., 2012; Uyulgan et al., 2014). The Bonding Representation Inventory (Luxford and Bretz, 2014) contained both one-tier and two-tier questions and was implemented in different schools and at different levels in the USA and Slovakia (Vrabec and Prokša, 2016). It was used as a diagnostic instrument to quickly test students’ understanding and alternative conceptions in chemical bonding. Another form of diagnostic assessment is the rule-space model, which was used to determine student learning progressions when learning about bonding energy (Chen et al., 2017). Although promising, the use of diagnostic instruments remains challenging. Written tests are not always as conclusive as anticipated (Schmidt et al., 2009).
Informal assessment.
Asking students to create their own models or concept maps, e.g. of ionic and covalent bonding, can reveal alternative conceptions (Luxford and Bretz, 2013). To make students’ mental model more explicit, let students draw molecular interactions, compare the drawings with other students, and share these in class. Although recognizing features of structures is easier than creating structures and describing the interactions, the latter is more important in assessing student understanding (Harle and Towns, 2013). Another way to characterize students’ understanding of chemical bonding is by mapping students’ conceptual knowledge in a matrix, which shows the development of clustered concepts over time. In this way, students’ strengths and weaknesses can be identified, and their developments can be monitored (Yayon et al., 2012).
Synthesis of findings
Table 4 provides an overview of the results of the systematic literature review on PCK for chemical bonding. As described previously, key insights were identified within each PCK component, and related to those, eight corresponding guidelines were articulated. By examining the eight guidelines, three overarching challenges were identified: bond type classification (B), models (M), and transfer (T). Three guidelines relate to Bond type classification: evidence-based teaching sequences, prior knowledge, and covalent–ionic dichotomy. Similarly, three guidelines were associated with Models: strength and limitations, applicability, anthropomorphic language or representations. Finally, two guidelines refer to Transfer: explicit attention to chemical bonding in other domains and micro–macro thinking.
Table 4 Key insights per PCK component, guidelines and overarching challenges
PCK component |
Key insights for chemical bonding |
Guidelines for addressing challengesa |
Alphanumeric codes are related to the overarching challenges: bond type classification (B), models (M), and transfer (T).
|
Curriculum |
Chemical bonding concepts are essential in many other domains (e.g. biochemistry and organic chemistry) and insufficient connections are made. |
Give explicit attention to transfer chemical bonding concepts to other domains. (T1) |
Textbooks and teachers do not always use evidence-based teaching sequences. |
Use evidence-based teaching sequences. (B1) |
Students need to have proper prior knowledge (e.g. particulate nature of matter). |
Activate and check student prior knowledge. (B2) |
|
Student understanding |
Consider student difficulties in chemical bonding when preparing lessons in order to prevent the formation of alternative conceptions. |
Avoid dichotomy between covalent and ionic bonds. (B3) |
Realize the presence of potential alternative conceptions and be aware of their sources. |
Be aware of the strength and limitations of models. (M1) |
|
Instructional strategies and representations |
Allow students to work on group assignments. |
n.a. |
Think critically about the choice of representations and discuss its characteristics, limitations and challenges with students. |
Explain the applicability of models and the underlying rules. (M2) |
Avoid anthropomorphic language or representations. (M3) |
Focus on thinking about structure–property relationships. |
Use micro–macro transfer while teaching. (T2) |
|
Assessment |
Diagnostic instruments (two-tier tests) can be used to test students’ understanding and alternative conceptions. |
Activate and check student prior knowledge. (B2) |
Results from the teacher educator content representations
The CoRe was completed by nine teacher educators, and most of them (n = 7) related the learning goals to the number of valence electrons and intended students to be able to draw bonds and understand which atoms can form covalent bonds. Some of the teacher educators mentioned the connection with the periodic table and the noble gas configuration. The majority of teacher educators found the concept important because it is necessary in understanding micro–macro properties. One considered the importance of the concept to be able to apply electrostatic interactions when reasoning about reactivity. Regarding ‘curriculum later,’ teacher educators mentioned polarity, Lewis structures, VSEPR, reaction mechanisms, and quantum mechanics (orbitals). The main difficulties described by the teacher educators were as follows: chemical bonding is very abstract (no context, not visible), students think that models are always true and applicable, it is difficult to relate micro to macro properties, and the idea that two (negatively charged) electrons form a pair. As to learner thinking, key answers were that it is crucial to relate to other concepts, that students find it difficult to think in models and relate micro to macro properties. Other factors that the teacher educators mentioned were the importance of prior knowledge, the difficulty to relate to students’ interest, and the availability of learning materials. About teaching procedures, most teacher educators suggested starting with activating prior knowledge (e.g. particulate nature of matter). Furthermore, several teacher educators proposed to use applets or animations, or to do an experiment. Two teacher educators chose a historical perspective to introduce the concept. As to formative assessment, teacher educators proposed to let students draw molecules, do some kind of quiz, or predict the properties of new molecules.
The CoRes were coded using the guidelines distilled from the literature review. For instance, teacher educator 3's statement “Because many different bond types exist and these have diverse consequences on micro- and therefore macro-level.” was coded as ‘micro–macro.’ Teacher educator 9's statement “It is difficult to stay away from expressions as “those atoms want to…”” was coded as ‘anthropomorphic language.’ All guidelines distilled from the literature review were found in the CoRes of at least one teacher educator. Table 5 provides an overview of the guidelines identified in the CoRes of the nine teacher educators.
Table 5 Overview of the overarching challenges and guidelines identified in the teacher educators’ CoRe about covalent bonding
Teacher educator |
1 |
2 |
3 |
4 |
5 |
6 |
7 |
8 |
9 |
Total |
x = this guideline was discovered in the CoRe. |
Bond type classification
|
B1: teaching sequence |
|
|
|
x |
|
|
x |
|
|
2 |
B2: prior knowledge |
x |
|
x |
|
x |
x |
x |
|
|
5 |
B3: covalent–ionic dichotomy |
|
|
|
|
x |
x |
|
|
x |
3 |
Models
|
M1: strengths and limitations |
|
x |
x |
|
|
x |
x |
|
x |
5 |
M2: applicability |
x |
x |
|
x |
|
|
x |
x |
|
5 |
M3: anthropomorphic language |
|
|
|
|
|
|
|
|
x |
1 |
Transfer
|
T1: other domains |
|
|
|
|
x |
|
|
|
|
1 |
T2: micro–macro |
x |
x |
x |
x |
|
|
x |
|
x |
6 |
The overview in Table 5 shows that four guidelines which were found in the literature review readily came to the majority of chemistry teacher educators’ minds: prior knowledge, strengths and limitations, applicability and micro–macro. By contrast, two guidelines were mentioned by two or three respondents: teaching sequence and covalent–ionic dichotomy. In addition, two others were mentioned by only one respondent: anthropomorphic language and other domains. It is interesting that half of the guidelines were mentioned by one third or less of the teacher educators participating. So even though these guidelines have been described in the literature in the past decades, they do not appear to readily come to teacher educators’ minds.
Conclusions
This study was set out to answer the research question: “What are the characteristics of a framework about teaching chemical bonding that can support teacher education?” The results of the systematic literature review revealed eight key insights and eight corresponding guidelines for addressing them, as well as three overarching challenges when it comes to teaching chemical bonding. The results from the teacher educator CoRes showed that, although the challenges and guidelines derived from the literature are part of the cPCK, they are not all part of the pPCK of all teacher educators in this study. Thus, the degree of consensus about cPCK for chemical bonding among teacher educators is not to be overestimated.
The framework shown in Fig. 1 constitutes a visual answer to our research question. This may be a useful discussion tool in further explorations of pPCK for chemical bonding. It can also be helpful for teacher educators to shape and prioritize chemistry teacher education coursework on how to teach bonding. Further, it can also be used by preservice teachers to help assess attention to salient considerations when evaluating teaching and learning activities. Finally, the framework can be applied during the design of classroom activities for teaching about chemical bonding. In this regard, Appendix D offers three practices for teaching chemical bonding that align with the recommendations given in the framework, namely, a curriculum sequence, two group discussion tasks, and an assessment-for-learning activity.
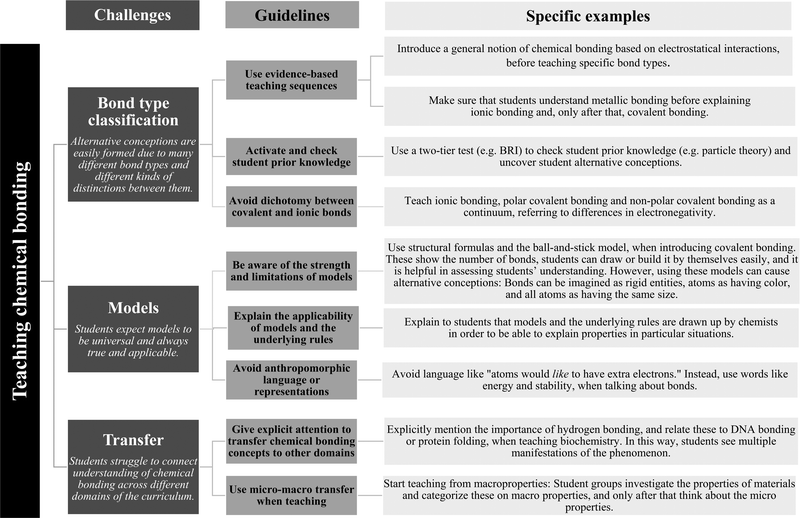 |
| Fig. 1 Framework for understanding and supporting the teaching of chemical bonding. | |
Limitations and recommendations
Literature review
The systematic literature review has several limitations, and both the limitations and insights gleaned give rise to recommendations for future research. First, our search terms were specifically for the secondary education level and the topic chemical bonding, and the search engines searched in the title, abstract and keywords. Although articles on multiple education levels (Coll and Treagust, 2003) and on general chemistry (Mutlu and Sesen, 2016) were found, we might have missed articles covering multiple education levels or chemistry topics that could contain interesting information.
Second, we limited our results by selecting only journal articles. While this was done because of our focus on empirical work, it might have excluded relevant information in books or theses. As a result, this review might be limited or reflect any publication bias in journals (mainly significant effects were found in the reviewed studies). Future research could include conference contributions reporting on empirical research to mitigate the potential effects of publication bias. For example, two book chapters provide valuable insights that relate to the framework and confirm several of the guidelines. Namely, Taber and Coll (2002) elaborate misconceptions related to chemical bonding, as well as the development of models of chemical bonding that are more authentic. Similarly, an entire chapter is devoted to the topic in Taber's (2002) volume on chemical misconceptions.
Third, in this review, the size of the student population in the studies was not considered. While the small scale, often (semi-)qualitative nature of the studies (e.g.Cheng and Gilbert, 2014) certainly offers affordances, the lack of large-scale studies could reduce the generalizability of the results. This points to a gap in the literature which could be addressed by further research.
Fourth, this study focuses only on PCK that teachers need. Of course, it is also important to consider how teachers develop (these components of) PCK. Although existing research tells us that experience (Sibanda, 2018), systematic reflection on teaching practices with colleagues (Bergqvist et al., 2016), and coteaching with senior students (Schultze and Nilsson, 2018) can be important for developing PCK, not all PCK components can be improved with each of these approaches (Aydin and Boz, 2013). Research is needed to articulate how integrated PCK development can be supported.
Finally, as we attend to developing PCK, the role of supporting materials is essential (Davis and Krajcik, 2005). Educative materials are very important because they tend to be more easily accessible to teachers and can contain educational elements that promote the learning of teachers (Schneider and Krajcik, 2002; Remillard, 2005; Penuel and Gallagher, 2009). When developing educative materials, for instance to situate learning in the context of cutting-edge chemistry research, the above-mentioned findings on PCK components for chemical bonding are relevant. Thus, studies on PCK development could also support much-needed (design) research to develop (and articulate the characteristics of effective) educative materials supporting teachers in their development of PCK regarding particular topic areas (e.g. chemical bonding).
Content representations
One limitation of the construction of the CoRes by the teacher educators was that they are not likely to be comprehensive portrayals of teacher knowledge on PCK for chemical bonding. We intentionally asked teacher educators to portray the aspects that most readily came to their minds in order to ascertain which elements are regularly part of common practice. While we maintain that this strengthens the ecological validity of the study, we do note that different results might have been obtained if we have requested comprehensive overviews. Future research could explore if and to what extent CoRes vary based on different approaches to data collection. A second limitation pertains to the fact that teacher educator background data were not collected and, as a result, could not be included in our analysis. For example, years of prior teaching experience could affect participant CoRes. Collection of such data together with CoRes could help identify issues that are particularly common, for example, among starting teacher educators and others that persist across time. Similarly, teacher orientations may influence teacher CoRes, because orientations function as filters or amplifiers for educational practice (Van Driel et al., 2007), and affect the goals and purposes with which they teach (Park and Oliver, 2008; Park, 2019). Such work may not only serve scientific understanding, but also teachers, as they can benefit from insight into their own repertoire and habits to directly support students’ meaning-making of chemistry (Patron et al., 2017). Finally, we note that the CoRes were filled in asynchronously online. It was therefore not possible to ascertain if the teacher educators had any questions while conducting the activity. While respondents were invited to ask questions if they had any, subsequent research might address this more thoroughly through synchronous data collection.
Closing considerations
As the refined consensus model authors put it, cPCK
“… is an amalgam of multiple science educators’ contributions, including the teacher's own contributions and those from the combined professional knowledge bases and varied teaching experiences within a given subject matter as understood and documented by multiple people. The resulting cPCK is a specialised knowledge base for science teaching that has been articulated and is shared among a group of professionals, which is related to teaching that particular subject matter knowledge to particular students in a particular learning context. Most importantly, this knowledge can be shared and articulated in ways that encourage conversations among researchers, teachers, and other education professionals. This cPCK is the realm of PCK that has been in the literature since Lee Shulman's presidential address to the American Education Research Association (AERA) in 1986” (Carlson et al., 2019, p. 90).
Sourced from prior research and refined through portrayals of existing practice, this study yielded a cPCK framework showing how preservice teachers might be guided to teach chemical bonding. Although the framework offers guidelines and specific examples, it does not provide concrete tools for preservice teachers to check whether a chosen element or strategy is working for their own students. This should be taken up in future research. It is also worth noting that, while the literature in our corpus included uses of technology, our data set did not address the question of why technology is important. As a result, no detailed examples of technology-based representations were included in the framework. Given the ways in which remote schooling was shown to be a necessity during the COVID-19 pandemic and the fact that many teachers have begun to explore hybrid environments since then, this seems to be an important topic for further investigation. Finally, our framework stresses the importance of attending to transfer (e.g. bonding concepts in biochemistry, biology or other science domains). To facilitate the making of such connections, research is needed to investigate if and in what ways both pre-service teachers and teacher educators are aware of bonding concepts in other domains.
Despite its limitations, this study provides a comprehensive synthesis of empirical findings related to cPCK for chemical bonding. It also identified gaps in the PCK literature for chemical bonding. While the results offer considerable guidance related to knowledge of instructional strategies and representations as well as to knowledge of student understanding, only a few articles contained information relevant to knowledge of curricula or knowledge of assessment. Especially given its prominent role in shaping teaching approaches, it seems that research is particularly needed on (formative) assessment of student learning for chemical bonding. The framework contributes to supporting teacher educators in their work with novice teachers learning to grasp the complexity of teaching chemical bonding while also providing clear guidelines and practical suggestions.
Ethics approval
This study was approved by the University of Twente BMS Ethics Committee, request nr. 201301.
Informed consent
Informed consent was secured from all participants in this study.
Conflicts of interest
The authors declare that they have no conflicts of interest.
Appendices
Appendix A: schematic overview of the article search and selection processes
Search |
WoS |
Scopus |
ERIC |
PsycINFO |
Topic and PCK and secondary school 1998 to 2018
|
|
|
|
|
((Bonding) AND (attitude* OR view* OR perception* OR *conception* OR sequence OR teach* OR learn* OR “conceptual development” OR “conceptual understanding” OR transfer* OR represent* OR coherence) AND (secondary OR “high school” OR “junior high” OR “middle school”)) |
5731 |
3981 |
218 |
243 |
Category reduction
|
|
|
|
|
– WoS: “Education Educational Research” and “Education Scientific disciplines” |
|
|
|
|
– Scopus: “Social sciences” |
156 |
186 |
n.a. |
n.a. |
Results combined, duplicates removed
|
582 |
Screening criteria applied to titles
|
|
– Secondary or tertiary education (excluding graduate level) |
158 |
– Chemistry |
|
Screening criteria applied to journals
|
|
– “ISI master journal list” |
|
– Published in the English language |
104 |
Abstract screening
|
|
– The abstract is about (one or more aspects from) chemical bonding |
89 |
– The abstract shows that the article potentially contains PCK components. |
|
Inclusion criteria on full text
|
|
– Quality appraisal |
59 |
– One or more of the 4 PCK components must be addressed |
|
Appendix B: coding overview
PCK component (deductive) |
Example of extracted data |
Corresponding key insight for chemical bonding |
Curriculum (n = 10) |
“The participating teachers introduced the different types of bonding in the same order as the textbooks they used.” (Bergqvist and Chang Rundgren, 2017, p. 229) |
Textbooks and teachers do not always use evidence-based teaching sequences. |
Student understanding (n = 26) |
“…the model of ionic bonding regarded as scientifically acceptable for inclusion in the school curriculum is not well understood by considerable numbers of students at both the secondary and tertiary levels, and even by a significant number of teachers.” (Vladušić et al., 2016, p. 693) |
Realize the presence of potential alternative conceptions and be aware of their sources. |
Instructional strategies and representations (n = 30) |
“…cooperative learning based on the conceptual change approach instruction caused a significantly better acquisition of scientific concepts related to chemical bonding and elimination of misconceptions than traditionally designed chemistry instruction.” (Eymur and Geban, 2017, p. 868) |
Allow students to work on group assignments. |
Assessment (n = 10) |
“The descriptive statistics and psychometrics suggest that the items on the BRI are generating valid and reliable data regarding student misconceptions about multiple representations of covalent and ionic bonding.” (Luxford and Bretz, 2014, p. 318) |
Diagnostic instruments (two-tier tests) can be used to test students understanding and alternative conceptions. |
Appendix C: literature relevant to each PCK component
PCK component |
Relevant sources |
Curriculum (n = 10) |
De Posada, 1999; Taber, 2003; Othman et al., 2008; Cooper et al., 2012; Broman and Parchmann, 2014; Sibanda and Hobden, 2015; Bergqvist and Chang Rundgren, 2017; Chen et al., 2017; Cheng and Gilbert, 2017; Sibanda, 2018 |
Student understanding (n = 26) |
Birk and Kurtz, 1999; Harrison and Treagust, 2000; Coll and Treagust, 2001; Coll and Treagust, 2002; Coll and Treagust, 2003; Taber, 2003; Çalik, 2005; Acar and Tarhan, 2008; Toplis, 2008; Kahveci, 2009; Schmidt et al., 2009; Kind and Kind, 2011; Kabapinar, 2013; Luxford and Bretz, 2013; Broman and Parchmann, 2014; Cheng and Gilbert, 2014; Cokelez et al., 2014; Kind, 2014; Uyulgan et al., 2014; Akkuzu and Uyulgan, 2016; Cheng and Oon, 2016; Mutlu and Sesen, 2016; Vladušić et al., 2016; Bergqvist and Chang Rundgren, 2017; Joki and Aksela, 2018; Sibanda, 2018 |
Instructional strategies and representations (n = 30) |
Barnea and Dori, 1999; De Posada, 1999; Harrison and Treagust, 2000; Coll and Treagust, 2003; Taber, 2003; Acar and Tarhan, 2008; Tarhan et al., 2008; Toplis, 2008; Özmen, 2008; Frailich et al., 2009; Özmen et al., 2009; Lewis et al., 2012; Waldrip and Prain, 2012; Bergqvist et al., 2013; Karacop and Doymus, 2013; Broman and Parchmann, 2014; Warfa et al., 2014; Joki et al., 2015; Bergqvist et al., 2016; Cheng and Oon, 2016; Nimmermark et al., 2016; Putra and Tang, 2016; Bergqvist and Chang Rundgren, 2017; Eymur and Geban, 2017; Patron et al., 2017; Wheeldon, 2017; Langbeheim and Levy, 2018; Schultze and Nilsson, 2018; Tsaparlis et al., 2018 |
Assessment (n = 10) |
Othman et al., 2008; Schmidt et al., 2009; Heredia et al., 2012; Yayon et al., 2012; Luxford and Bretz, 2013; Harle and Towns, 2013; Luxford and Bretz, 2014; Uyulgan et al., 2014; Vrabec and Prokša, 2016; Chen et al., 2017 |
Appendix D: set of practical applications based on this study
Curriculum sequence for chemical bonding
Based on the findings, we suggest starting from the macro properties in grade 8 or 9 to learn about the classification of compounds in metallic, ionic and molecular compounds. The micro properties can subsequently be specified in grades 10, 11, and/or 12 as the inter- and intramolecular bond types. This sequence is shown in Fig. 2.
Group discussion task to learn about hydrogen bonding
Fig. 3 provides a schematic overview of two related discussions tasks. First, students discuss the boiling points of several compounds, and then transfer their conclusions for application in another context (DNA binding). Based on the review findings, we recommending heterogeneous groups (comprised of students with varying levels of prior academic achievement).
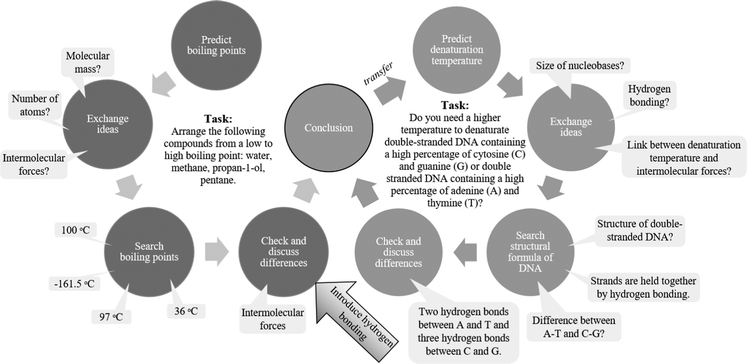 |
| Fig. 3 Schematic overview of two group discussion tasks to learn about hydrogen bonding. | |
Assessment-for-learning activity focused on the difference between intra- and intermolecular bonding
We choose this focus because the review showed that it is essential to understand the concepts of intra- and intermolecular bonding before learning the types of intra- and intermolecular bonding. This activity uses the research of Movilli et al. (2018) as a motivating context to practice the difference between intra- and intermolecular bonding. In this activity, students categorize and explain their understanding of the four steps of tumor DNA detection. Fig. 4 shows the worksheet for students. After this activity, the concept of hydrogen bonding could be taught in this context, by zooming in on the DNA detection step, since knowledge of hydrogen bonding is necessary to understand DNA binding.
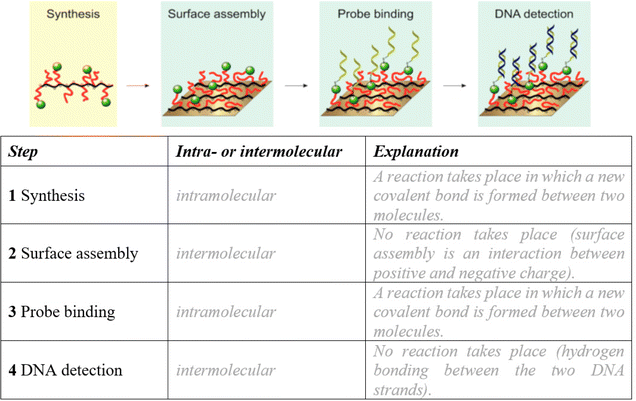 |
| Fig. 4 Student worksheet with sample student answers (grey) based on representations by Movilli et al. (2018). PLL = poly(L-lysine); OEG = oligo(ethylene glycol); Mal = maleimide; PNA = peptide nucleic acid. | |
Step 1 – synthesis.
In the first step a fraction of the NH3+ groups of PLL react with ‘OEG’ and a part reacts with Mal. The other NH3+-groups do not react.
Step 2 – surface assembly.
In this step the NH3+-groups of PLL-OEG-Mal that did not react (shown as °) bind to the negatively charged gold surface.
Step 3 – probe binding.
In this step the SH-group of the thiol-PNA (‘probe’) reacts with the maleimide group of PLL-OEG-Mal.
Step 4 – DNA detection.
In the last step the tumor DNA binds to the ‘probe’ (PNA chain).
Acknowledgements
This research received funding from the Dutch Ministry of Education, Culture and Science under the Dudoc program. The authors would like to thank the teacher educators who completed the Content Representation.
References
- Acar B. and Tarhan L., (2008), Effects of Cooperative Learning on Students' Understanding of Metallic Bonding, Res. Sci. Educ., 38, 401–420.
- Akkuzu N. and Uyulgan M. A., (2016), An epistemological inquiry into organic chemistry education: exploration of undergraduate students' conceptual understanding of functional groups, Chem. Educ. Res. Pract., 17, 36–57.
- Aydin S. and Boz Y., (2013), The Nature of Integration among PCK Components: A Case Study of Two Experienced Chemistry Teachers, Chem. Educ. Res. Pract., 14, 615–624.
- Barke H. D., Hazari A., and Yitbarek S., (2008), Misconceptions in chemistry: Addressing perceptions in chemical education, London: Springer.
- Barnea N. and Dori Y. J., (1999), High-School Chemistry Students' Performance and Gender Differences in a Computerized Molecular Modeling Learning Environment, J. Sci. Educ. Technol., 8, 257–271.
- Bergqvist A. and Chang Rundgren S.-N., (2017), The Influence of Textbooks on Teachers' Knowledge of Chemical Bonding Representations Relative to Students' Difficulties Understanding, Res. Sci. Technol. Educ., 35, 215–237.
- Bergqvist A., Drechsler M. and Chang Rundgren S.-N., (2016), Upper Secondary Teachers' Knowledge for Teaching Chemical Bonding Models, Int. J. Sci. Educ., 38, 298–318.
- Bergqvist A., Drechsler M., De Jong O. and Chang Rundgren S.-N., (2013), Representations of chemical bonding models in school textbooks – help or hindrance for understanding? Chem. Educ. Res. Pract., 14, 589–606.
- Berry A., Friedrichsen P. and Loughran J., (2015), Re-examining pedagogical content knowledge in science education, London: Routledge.
- Biesta G., (2007), Bridging the gap between educational research and educational practice: the need for critical distance, Educ. Res. Eval., 13(3), 295–301.
- Birk J. P. and Kurtz M. J., (1999), Effect of Experience on Retention and Elimination of Misconceptions about Molecular Structure and Bonding, J. Chem. Educ., 76, 124–128.
- Black P. and Wiliam D., (1998), Assessment and Classroom Learning, Assess. Educ.: Princ., Policy Pract., 5, 7–74.
- Broekkamp H., and van Hout-Wolters B., (2007), The gap between educational research and practice: a literature review, symposium, and questionnaire, Educ. Res. Eval., 13(3), 203–220.
- Broman K. and Parchmann I., (2014), Students' application of chemical concepts when solving chemistry problems in different contexts, Chem. Educ. Res. Pract., 15, 516–529.
- Çalik M., (2005), A cross-age study of different perspectives in solution chemistry from junior to senior high school, Int. J. Sci. Math. Educ., 3, 671–696.
- Carlson J. et al., (2019), The Refined Consensus Model of Pedagogical Content Knowledge in Science Education, in Hume A., Cooper R. and Borowski A. (ed.), Repositioning Pedagogical Content Knowledge in Teachers’ Knowledge for Teaching Science, Singapore: Springer, pp. 77–94.
- Carpendale J. and Hume A., (2019), Investigating Practising Science Teachers’ pPCK and ePCK Development as a Result of Collaborative CoRe Design, in Hume A., Cooper R. and Borowski A. (ed.), Repositioning Pedagogical Content Knowledge in Teachers’ Knowledge for Teaching Science, Singapore: Springer, pp. 225–252.
- Chan K. K. H. and Hume A., (2019), Towards a Consensus Model: Literature Review of How Science Teachers’ Pedagogical Content Knowledge Is Investigated in Empirical Studies, in Hume A., Cooper R. and Borowski A. (ed.), Repositioning Pedagogical Content Knowledge in Teachers’ Knowledge for Teaching Science, Singapore: Springer, pp. 77–94.
- Chen F., Zhang S. S., Guo Y. F. and Xin T., (2017), Applying the Rule Space Model to Develop a Learning Progression for Thermochemistry, Res. Sci. Educ., 47, 1357–1378.
- Cheng M. M. W. and Gilbert J. K., (2014), Students' Visualization of Metallic Bonding and the Malleability of Metals, Int. J. Sci. Educ., 36, 1373–1407.
- Cheng M. M. W. and Gilbert J. K., (2017), Modelling Students' Visualisation of Chemical Reaction, Int. J. Sci. Educ., 39, 1173–1193.
- Cheng M. M. W. and Oon P.-T., (2016), Understanding metallic bonding: structure, process and interaction by Rasch analysis, Int. J. Sci. Educ., 38, 1923–1944.
- Cokelez A., Dal B. and Harman G., (2014), Turkish middle school students’ conceptions and modelling of chemical bonds, J. Baltic Sci. Educ., 13, 483–496.
- Coll R. K. and Treagust D. F., (2001), Learners' Mental Models of Chemical Bonding, Res. Sci. Educ., 31, 357–382.
- Coll R. K. and Treagust D. F., (2002), Exploring Tertiary Students' Understanding of Covalent Bonding, Res. Sci. Technol. Educ., 20, 241–267.
- Coll R. K. and Treagust D. F., (2003a), Investigation of secondary school, undergraduate, and graduate learners' mental models of ionic bonding, J. Res. Sci. Teach., 40, 464–486.
- Coll R. K. and Treagust D. F., (2003b), Learners' Mental Models of Metallic Bonding: A Cross-Age Study, Sci. Educ., 87, 685–707.
- Cooper M. M., Underwood Sa. M., Hilley C. Z. and Klymkowsky M. W., (2012), Development and Assessment of a Molecular Structure and Properties Learning Progression, J. Chem. Educ., 89, 1351–1357.
- Davis E. A. and Krajcik J. S., (2005), Designing educative curriculum materials to promote teacher learning, Educ. Res., 34, 3–14.
- De Posada J. M., (1999), The presentation of metallic bonding in high school science textbooks during three decades: science educational reforms and substantive changes of tendencies, Sci. Educ., 83, 423–447.
- Eymur G. and Geban Ö., (2017), The Collaboration of Cooperative Learning and Conceptual Change: Enhancing the Students’ Understanding of Chemical Bonding Concepts, Int. J. Sci. Math. Educ., 15, 853–871.
- Farley-Ripple E., May H., Karpyn A., Tilley K., and McDonough K., (2018), Rethinking connections between research and practice in education: a conceptual framework, Educ. Res., 47(4), 235–245.
- Frailich M., Kesner M. and Hofstein A., (2007), The Influence of Web-Based Chemistry Learning on Students' Perceptions, Attitudes, and Achievements, Res. Sci. Technol. Educ., 25, 179–197.
- Frailich M., Kesner M. and Hofstein A., (2009), Enhancing Students' Understanding of the Concept of Chemical Bonding by Using Activities Provided on an Interactive Website, J. Res. Sci. Teach., 46, 289–310.
- Geddis A. N. and Wood E., (1997), Transforming subject matter and managing dilemmas: a case study in teacher education, Teach. Teacher Educ., 13, 611–626.
- Gess-Newsome J., (2015), A model of teacher professional knowledge and skill including PCK: results of the thinking from the PCK Summit, in Berry A., Friedrichsen, P. and John, L. (ed) Re-examining Pedagogical Content Knowledge in Science Education, London: Routledge, pp. 28–42.
- Gilbert J. K. and Justi R., (2016), The contribution of visualisation to modelling-based
teaching, in Modelling-based teaching in science education, Cham: Springer, pp. 121–148 Search PubMed.
- Gilbert J. K. and Treagust D., (2009), Multiple Representations in Chemical Education, Dordrecht: Springer Netherlands.
- Glaser B., & Strauss A., (1999), The discovery of grounded theory: strategies for qualitative research, Aldine Transaction.
- Grossman P. L., (1990), The making of a teacher: teacher knowledge and teacher education, Teachers College Press, Teachers College, Columbia University.
- Harle M. and Towns M. H., (2013), Students' Understanding of Primary and Secondary Protein Structure: Drawing Secondary Protein Structure Reveals Student Understanding Better Than Simple Recognition of Structures, Biochem. Mol. Biol. Educ., 41, 369–376.
- Harrison A. G. and Treagust D. F., (2000), Learning about atoms, molecules, and chemical bonds: a case study of multiple-model use in grade 11 chemistry, Sci. Educ., 84, 352–381.
- Heredia K., Xu X. Y. and Lewis J. E., (2012), The application and evaluation of a two-concept diagnostic instrument with students entering college general chemistry, Chem. Educ. Res. Pract., 13, 30–38.
- Hume, A., Cooper, R. and Borowski, A. (ed.), (2019), Repositioning Pedagogical Content Knowledge in Teachers' Knowledge for Teaching Science, Singapore: Springer.
- Joki J. and Aksela M., (2018), The challenges of learning and teaching chemical bonding at different school levels using electrostatic interactions instead of the octet rule as a teaching model, Chem. Educ. Res. Pract., 19, 932–953.
- Joki J., Lavonen J., Juuti K. and Aksela M., (2015), Coulombic interaction in Finnish middle school chemistry: a systemic perspective on students' conceptual structure of chemical bonding, Chem. Educ. Res. Pract., 16, 901–917.
- Kabapinar F., (2013), Secondary Students' Reference to Properties of Matter to Chemical Bonds: Is the Onus on the Ontological Mismatch Only? Hacettepe Universitesi Egitim Fakultesi Dergisi-Hacettepe University Journal of Education, 28, 235–249.
- Kahveci A., (2009), Exploring chemistry teacher candidates' profile characteristics, teaching attitudes and beliefs, and chemistry conceptions, Chem. Educ. Res. Pract., 10, 109–120.
- Karacop A. and Doymus K., (2013), Effects of Jigsaw Cooperative Learning and Animation Techniques on Students' Understanding of Chemical Bonding and Their Conceptions of the Particulate Nature of Matter, J. Sci. Educ. Technol., 22, 186–203.
- Kennedy M. M., (1997), The connection between research and practice, Educ. Res., 26(7), 4–12.
- Kind V., (2004), Beyond appearances: Students’ misconceptions about basic chemical ideas, 2nd edn, London: Royal Society of Chemistry.
- Kind V., (2014), A Degree Is Not Enough: a quantitative study of aspects of pre-service science teachers' chemistry content knowledge, Int. J. Sci. Educ., 36, 1313–1345.
- Kind V. and Kind P. M., (2011), Beginning to Teach Chemistry: How Personal and Academic Characteristics of Pre-Service Science Teachers Compare with Their Understandings of Basic Chemical Ideas, Int. J. Sci. Educ., 33, 2123–2158.
- Kippers W. B., Wolterinck C. H. D., Schildkamp K., Poortman C. L. and Visscher A. J., (2018), Teachers' views on the use of assessment for learning and data-based decision making in classroom practice, Teach. Teacher Educ., 75, 199–213.
- Langbeheim E. and Levy S. T., (2018), Feeling the Forces within Materials: Bringing Inter-Molecular Bonding to the Fore Using Embodied Modelling, Int. J. Sci. Educ., 40, 1567–1586.
- Lewis M. S., Zhao J. H. and Montclare J. K., (2012), Development and Implementation of High School Chemistry Modules Using Touch-Screen Technologies, J. Chem. Educ., 89, 1012–1018.
- Loughran J., Berry A., and Mulhall P., (2012), Understanding and developing scienceteachers’ pedagogical content knowledge, vol. 12, Springer Science & Business Media.
- Luxford C. J. and Bretz S. L., (2013), Moving beyond definitions: what student-generated models reveal about their understanding of covalent bonding and ionic bonding, Chem. Educ. Res. Pract., 14, 214–222.
- Luxford C. J. and Bretz S. L., (2014), Development of the Bonding Representations Inventory To Identify Student Misconceptions about Covalent and Ionic Bonding Representations, J. Chem. Educ., 91, 312–320.
- Magnusson S., Krajcik J. and Borko H., (1999), Nature, Sources, and Development of Pedagogical Content Knowledge for Science Teaching, in Gess-Newsome J. and Lederman, N. G. (ed.), Examining Pedagogical Content Knowledge, Dordrecht, the Netherlands: Kluwer Academic Publishers, pp. 95–132.
- Mavhunga E., (2019), Exposing Pathways for Developing Teacher Pedagogical Content Knowledge at the Topic Level in Science, in Hume A., Cooper R. and Borowski A. (ed.), Repositioning pedagogical content knowledge in teachers’ knowledge for teaching science, Springer: Singapore, pp. 131–150.
- Movilli J., Rozzi A., Ricciardi R., Corradini R., and Huskens J., (2018), Control of Probe Density at DNA Biosensor Surfaces Using Poly(L-lysine) with Appended Reactive Groups. Bioconjugate Chem., 29, 4110–4118.
- Mutlu A. and Sesen B. A., (2016), Evaluating of Pre-Service Science Teachers' Understanding of General Chemistry Concepts by Using Two Tier Diagnostic Test, J. Baltic Sci. Educ., 15, 79–96.
- Nahum T. L., Mamlok-Naaman R., Hofstein A., and Krajcik J., (2007), Developing a new teaching approach for the chemical bonding concept aligned with current scientific and pedagogical knowledge, Sci. Educ., 91(4), 579–603.
- Nahum T. L., Mamlok-Naaman R., Hofstein A., and Kronik L., (2008), A new “bottom-up” framework for teaching chemical bonding, J. Chem. Educ., 85(12), 1680.
- Nahum T. L., Mamlok-Naaman R., Hofstein A. and Taber K. S., (2010), Teaching and learning the concept of chemical bonding, Studies Sci. Educ., 46, 179–207.
- Nimmermark A., Öhrström L., Mårtensson J. and Davidowitz B., (2016), Teaching of chemical bonding: a study of Swedish and South African students' conceptions of bonding, Chem. Educ. Res. Pract., 17, 985–1005.
- Othman J., Treagust D. F. and Chandrasegaran A. L., (2008), An investigation into the relationship between students' conceptions of the particulate nature of matter and their understanding of chemical bonding, Int. J. Sci. Educ., 30, 1531–1550.
- Özmen H., (2008), The influence of computer-assisted instruction on students' conceptual understanding of chemical bonding and attitude toward chemistry: a case for Turkey, Comput. Educ., 51, 423–438.
- Özmen H., Demircioglu H. and Demircioglu G., (2009), The Effects of Conceptual Change Texts Accompanied with Animations on Overcoming 11th Grade Students' Alternative Conceptions of Chemical Bonding, Comput. Educ., 52, 681–695.
- Park S. and Oliver J. S., (2008), Revisiting the conceptualisation of pedagogical content knowledge (PCK): PCK as a conceptual tool to understand teachers as professionals, Res. Sci. Educ., 38, 261–284.
- Park S., (2019), Reconciliation
between the refined consensus model of PCK and extant PCK models for advancing PCK research in science, in Repositioning pedagogical content knowledge in teachers’ knowledge for teaching science, Springer: Singapore, pp. 119–130.
- Park S. and Suh J. K., (2019), The PCK Map Approach to Capturing the Complexity of Enacted PCK (ePCK) and Pedagogical Reasoning in Science Teaching, in Hume A., Cooper R. and Borowski A. (ed.), Repositioning pedagogical content knowledge in teachers’ knowledge for teaching science, Springer: Singapore, pp. 187–197.
- Patron E., Wikman S., Edfors I., Johansson-Cederblad B. and Linder C., (2017), Teachers' reasoning: classroom visual representational practices in the context of introductory chemical bonding, Sci. Educ., 101, 887–906.
- Penuel W. R. and Gallagher L. P., (2009), Preparing Teachers to Design Instruction for Deep Understanding in Middle School Earth Science, J. Learn. Sci., 18, 461–508.
- Petticrew M. and Roberts H., (2006), Systematic reviews in the social sciences: a practical guide, Malden, MA: Blackwell Publishing.
- Putra G. B. S. and Tang K. S., (2016), Disciplinary literacy instructions on writing scientific explanations: a case study from a chemistry classroom in an all-girls school, Chem. Educ. Res. Pract., 17, 569–579.
- Remillard J. T., (2005), Examining key concepts in research on teachers' use of mathematics curricula, Rev. Educ. Res., 75, 211–246.
- Rollnick M., Bennett J., Rhemtula M., Dharsey N. and Ndlovu T., (2008), The Place of Subject Matter Knowledge in Pedagogical Content Knowledge: a case study of South African teachers teaching the amount of substance and chemical equilibrium, Int. J. Sci. Educ., 30, 1365–1387.
- Schmidt H. J., Kaufmann B. and Treagust D. F., (2009), Students' understanding of boiling points and intermolecular forces, Chem. Educ. Res. Pract., 10, 265–272.
- Schneider R. M. and Krajcik J., (2002), Supporting science teacher learning: the role of educative curriculum materials, J. Sci. Teacher Educ., 13, 221–245.
- Schultze F. and Nilsson P., (2018), Coteaching with senior students – a way to refine teachers’ PCK for teaching chemical bonding in upper secondary school, Int. J. Sci. Educ., 1–19.
- Shulman L. S., (1986), Those who understand: knowledge growth in teaching, Educ. Res., 15, 4–14.
- Shulman L. S., (1987), Knowledge and teaching: foundations of the new reform, Harvard Educ. Rev., 57, 1–23.
- Sibanda D., (2018), What Sequence do we Follow in Teaching Concepts in Chemistry? A Study of High School Physical Science Teachers' PCK, African J. Res. Math. Sci. Technol. Educ., 22, 196–208.
- Sibanda D. and Hobden P., (2015), Planning a teaching sequence for the teaching of chemical bonding, African J. Res. Math. Sci. Technol. Educ., 19, 23–33.
- Sorge S., Stender A. and Neumann K., (2019), The Development of Science Teachers’ Professional Competence, in Hume A., Cooper R. and Borowski A. (ed.), Repositioning Pedagogical Content Knowledge in Teachers’ Knowledge for Teaching Science, Singapore: Springer, pp. 151–166.
- Taber K. S., (2001), The Mismatch between Assumed Prior Knowledge and the Learner's Conceptions: a typology of learning impediments, Educ. Studies, 27, 159–171.
- Taber K. S. and Coll R. K., (2002), Bonding, in Gilbert J., De Jong O., Justi R., Treagust D. F. and Van Driel J. (ed.), Chemical education: towards research-based practice, Dordrecht: Kluwer Academic Publishers, pp. 213–234.
- Taber K. S., (2002), Chemical Misconceptions-Prevention, Diagnosis and Cure: Classroom Resources, Vol. 2, London: Royal Society of Chemistry.
- Taber K. S., (2003), Mediating mental models of metals: acknowledging the priority of the learner's prior learning, Sci. Educ., 87, 732–758.
- Tarhan L., Ayar-Kayali H., Urek R. O. and Acar B., (2008), Problem-Based Learning in 9th Grade Chemistry Class: “Intermolecular Forces”, Res. Sci. Educ., 38, 285–300.
- Tepner O. and Sumfleth E., (2019), Postscript, in Hume A., Cooper R. and Borowski A. (ed.), Repositioning Pedagogical Content Knowledge in Teachers’ Knowledge for Teaching Science, Singapore: Springer, pp. 319.
- Toplis R., (2008), Probing student teachers' subject content knowledge in chemistry: case studies using dynamic computer models, Chem. Educ. Res. Pract., 9, 11–17.
- Tsaparlis G., Pappa E. T. and Byers B., (2018), Teaching and Learning Chemical Bonding: Research-Based Evidence for Misconceptions and Conceptual Difficulties Experienced by Students in Upper Secondary Schools and the Effect of an Enriched Text, Chem. Educ. Res. Pract., 19, 1253–1269.
- Uyulgan M. A., Akkuzu N. and Alpat S., (2014), Assessing the Student' Understanding Related to Molecular Geometry using a Two-tier Diagnostic Test, J. Baltic Sci. Educ., 13, 839–855.
- Vanassche E. and Berry A., (2020), Teacher Educator Knowledge, Practice, and S-STTEP Research, in Kitchen J. et al. (ed.), International Handbook of Self-Study of Teaching and Teacher Education Practices, Singapore, Springer, pp. 177–213.
- Vanassche E., and Kelchtermans G., (2015), The state of the art in self-study of teacher education practices: a systematic literature review, J. Curriculum Studies, 47(4), 508–528.
- Van der Kleij F. M., Vermeulen J. A., Schildkamp K. and Eggen T. J. H. M., (2015), Integrating data-based decision making, Assessment for Learning and diagnostic testing in formative assessment, Assess. Educ. Princ., Policy Pract., 22, 324–343.
- Van Driel J. H., Bulte A. M. W. and Verloop N., (2007), The relationships between teachers' general beliefs about teaching and learning and their domain specific curricular beliefs, Learn. Instruct., 17, 156–171.
- Van Driel J. H., Verloop N. and de Vos W., (1998), Developing science teachers' pedagogical content knowledge, J. Res. Sci. Teach., 35, 673–695.
- Vladušić R., Bucat R. B. and Ožić M., (2016), Understanding ionic bonding-a scan across the Croatian education system, Chem. Educ. Res. Pract., 17, 685–699.
- Vrabec M. and Prokša M., (2016), Identifying Misconceptions Related to Chemical Bonding Concepts in the Slovak School System Using the Bonding Representations Inventory as a Diagnostic Tool, J. Chem. Educ., 93, 1364–1370.
- Waldrip B. and Prain V., (2012), Developing an Understanding of Ions in Junior Secondary School Chemistry, Int. J. Sci. Math. Educ., 10, 1191–1213.
- Warfa A. R. M., Roehrig G. H., Schneider J. L. and Nyachwaya J., (2014), Collaborative discourse and the modeling of solution chemistry with magnetic 3D physical models – impact and characterization, Chem. Educ. Res. Pract., 15, 835–848.
- Wheeldon R., (2017), Improving preservice chemistry teachers' content knowledge through intervention activities, Int. J. Sci. Educ., 39, 1238–1261.
- Yayon M., Mamlok-Naaman R. and Fortus D., (2012), Characterizing and representing student's conceptual knowledge of chemical bonding, Chem. Educ. Res. Pract., 13, 248–267.
|
This journal is © The Royal Society of Chemistry 2023 |
Click here to see how this site uses Cookies. View our privacy policy here.