DOI:
10.1039/D3RA07183A
(Paper)
RSC Adv., 2023,
13, 35811-35815
A thiomorpholine substituted malonyl-coumarin dye for discriminative detection of hydrazine and strong acidity†
Received
21st October 2023
, Accepted 30th October 2023
First published on 8th December 2023
Abstract
Detection of toxic hydrazine and harmful strong acidity is of great importance for survival of organisms. In the present paper, a new thiomorpholine substituted malonyl-coumarin dye was synthesized for discriminative detection of hydrazine and strong acidity. At pH 7.4, the fluorescence at 560 nm decreased and that at 496 nm increased upon reaction with hydrazine, which was used for on-site detection of hydrazine vapor and endogenous hydrazine in live cells. From pH 2.0 to 1.2, the fluorescence at 563 nm increased greatly, which could be ascribed to the PET process from thiomorpholine to malonyl-coumarin. The probe was desirable for discriminative detection of toxic hydrazine and strong acidity.
Introduction
Hydrazine (N2H4) is a highly toxic chemical which has been widely used as a reductant, antioxidant, developer, fuel and raw material for pharmaceuticals.1,2 The high toxicity poses a significant threat to life and the environment.3 So the residual amount of N2H4 is strictly confined.4 Acidity is an important index in industry and the physiology of living organisms.5,6 Much industrial wastewater is strongly acidic, which could pollute the environment if discharged without effective treatment. Therefore, it is of great importance to detect N2H4 and strong acidity.
Fluorescent probes have been developed for detection of various anions, cations, small molecules and biomacromolecules.7–10 Their rapid development could be due to the advantages including good selectivity, sensitivity, low cost, easy operation and on-site monitoring, etc.11,12 To detect N2H4, fluorescent probes have been designed based on different strategies including hydroxyl/amino protection–deprotection,13–16 group transformation,17–22 cyclization,23,24 lactonic ring-opening25 and regulation of hydrogen bonds.26,27 To detect acidity, probes have been designed mainly based on the acid-base neutralization (for some probes which work in alkaline conditions, nucleophilic addition reaction of the hydroxyl ion was involved).28–32 However, most N2H4 probes work in organic solvent/water mixtures, and some suffer ultraviolet excitation, a small Stokes shift or single fluorescence channel variation. At present, most probes for detection of acidity operated at pH greater than 2.0,33,34 and few probes could operate under a strong acidic environment.35–38
Here, a new fluorescent probe was designed for discriminative detection of N2H4 and strong acidity. The dual functional probe, named CMSM, was designed based on thiomorpholine substituted malonyl-coumarin. Coumarin is a satisfactory fluorophore which has been widely used as the signal reporting moiety of fluorescent probes.39,40 Dicyanovinyl is a typical electron-deficient moiety, which served as the specific reaction site of N2H4.41,42 The thiomorpholine was introduced onto the coumarin to serve as the receptor of H+. Upon addition of N2H4, the moderate fluorescence at 560 nm decreased and the fluorescence at 496 nm increased, showing an obvious ratiometric response. The probe showed good selectivity over the rich intracellular thiols, and could be used to image N2H4 metabolized from isoniazid in live cells. Moreover, the probe could also detect N2H4 vapor on site. The detection of N2H4 featured good stability, broad pH stability, visible light excited fluorescence, detection in an almost pure aqueous solution and a ratiometric response. As for pH detection, the fluorescence at 563 nm increased greatly when pH changed from 2.0 to 1.2, and a PET process was responsible for the fluorescence changes which was verified by theoretical calculations. To sum up, the probe has the following merits: (1) detection of hydrazine both in real environmental samples and live cells; (2) detection of extremely acidic pH values by regulating the PET process; (3) the detection of hydrazine and pH could be distinguished. Comparisons have been made between CMSM and other multifunctional N2H4 probes (Table S1, Scheme S1†).
Experimental section
Synthesis
The synthesis of compound 1, 2, 3 and 4 was described in our previous literature.43 The synthesis of CMSM was as follows:
Compound 4 (0.500 g, 1.45 mmol), malononitrile (0.115 g, 1.74 mmol) and a drop of piperidine were added into absolute ethanol (50 mL). The reaction mixture was stirred at room temperature under N2 atmosphere overnight. The solvent was evaporated and the residue was subjected to column chromatography on silica gel using dichloromethane as eluent to afford CMSM as dark red solid (0.314 g, 55%). Mp: 202.2–204.7 °C. 1H NMR (CDCl3, 400 MHz) δ 7.604 (s, 1H), 7.575 (d, J = 9.6 Hz, 1H), 6.598 (d, J = 9.6 Hz, 1H), 6.454 (s, 1H), 3.849 (m 4H), 3.463 (q, J = 6.8 Hz, 4H), 2.845 (m, 4H), 1.256 (t, J = 6.8 Hz, 6H); 13C NMR (CDCl3, 100 MHz) δ 164.2, 158.1, 156.2, 153.4, 151.4, 126.9, 114.4, 112.1, 108.2, 104.0, 100.3, 96.8, 80.8, 55.1, 44.1, 27.4, 11.5. FT-IR (KBr, cm−1): 3161, 2974, 2950, 2940, 2901, 2871, 2844, 2211, 1708, 1608, 1546, 1483, 1437, 1415, 1371, 1347, 1330, 1266, 1198, 1187, 1140, 1120, 1078, 950, 739, 671. HR-MS (ESI): m/z calculated for C21H23N4O2S+ 395.1542, found 395.1534.
Spectroscopic characterization
Probe CMSM was dissolved in DMSO to get the stock solution (10−4 M). Deionized water was used to prepare the stock solutions (10−3 M) of N2H4, NH3·H2O, CH3(CH2)3NH2, (CH3)3CNH2, DGA, histamine, tyramine, tryptamine, HN(CH3)2, N(CH2CH3)3, pyridine, p-phenylenediamine, urea, thiourea, isoniazid, NaClO, H2O2, KNO3, NaNO2, NaF, NaCl, NaBr, KI, CH3COONa, NaHCO3, Na2CO3, Na2SO4, NaHSO3, Na2S2O3, Na2S, MgSO4, Al2(SO4)3, FeSO4, FeCl3, ZnSO4, CuSO4, CoCl2, CaCl2, BaCl2, NiCl2, Cys, Hcy, GSH, Arg, Lys, His, Gly, Pro, Val and Glu, respectively. For N2H4 detection, the final concentration of the probe was 1 μM, and the test solution was PBS (10 mM, pH 7.4), λex. = 400 nm and 470 nm, Slits: 10 nm/10 nm. For pH detection, λex. = 470 nm, Slits: 5 nm/5 nm.
Soil sample imaging
Two soil samples (1 and 2, 1.0 g) were obtained from Shandong University of Technology and filtered through a filter screen of 1 mm diameter. Soil 2 was sprayed with 300 μL N2H4, then with 50 μL CMSM. Soil 1 was sprayed with 50 μL CMSM only. PBS buffer (5 mL, 10 mM, pH 7.4) was added to above soil samples. After ultrasonic oscillation, the homogenate was filtered through filter paper. The visual images were recorded under a 365 nm UV lamp.
Cell imaging
HeLa cells were cultured in a 6-well plate in Dulbecco's modified Eagle's medium (DMEM) supplemented with 10% fetal bovine serum in an atmosphere of 5% CO2 and 95% air at 37 °C. CMSM was dissolved in DMSO (1 mM) and diluted to 1 μM before use. HeLa cells were incubated with CMSM for 30 min, then with N2H4 (100 μM). For endogenous N2H4 imaging, cells were incubated with isoniazid (100 μM) for 1 h, and then treated with CMSM for 2 h. Excited at 405 nm, the blue fluorescence was collected from 465 to 530 nm, and the yellow fluorescence was obtained from 540 to 600 nm by excitation at 488 nm.
Results and discussions
Design, synthesis and spectral response to N2H4
Probe CMSM was composed of a coumarin, a dicyanovinyl and a thiomorpholine moiety, and was synthesized following the route presented in Scheme 1. Electron-deficient dicyanovinyl moiety linked to coumarin at site 3 can prolong the conjugated system, and act as the reactive site for nucleophilic N2H4. A thiomorpholine moiety linked to coumarin at site 4 can act as the acceptor of H+; this moiety is also expected to hinder the electron-deficient site 4 from being attacked by nucleophiles. Therefore, CMSM was synthesized and characterized by 1H NMR, 13C NMR, FT-IR and HR-MS (Fig. S8–S11†).
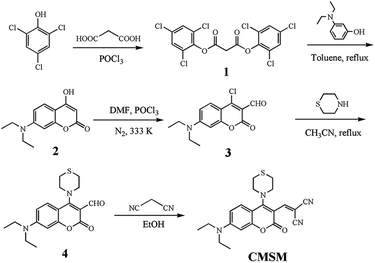 |
| Scheme 1 Synthesis of probe CMSM. | |
CMSM emitted moderate fluorescence at 560 nm when excited at 470 nm. After mixed with N2H4 (0–100 equiv.), the fluorescence decreased gradually. Meanwhile, obvious fluorescence increase at 496 nm was observed when excited at 400 nm (Fig. 1a and b). Thus, CMSM was a ratiometric fluorescent probe for detection of N2H4. The ratiometric signal was helpful to improve the accuracy and selectivity of the probe. The excitation wavelengths (400 nm or 470 nm) located in the low-energy visible light region, which was beneficial for cell imaging. Notably, the detection of N2H4 was conducted in almost pure aqueous solution (PBS, 10 mM, pH = 7.4, containing 0.15% DMSO). The dynamic detection range was from 0 to 25 equiv. of N2H4 with a good linear relationship (Fig. 1c). The detection limit was calculated to be 0.32 μM, proving satisfactory detection sensitivity.
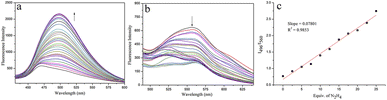 |
| Fig. 1 (a) and (b) Fluorescence titration spectra of CMSM (1 μM) in the presence of N2H4 (0–100 equiv.). (c) The linear relationship between fluorescence ratios (I496/I560) and N2H4 (0–25 equiv.). | |
Then the fluorescence spectra of CMSM were recorded over time. Fluorescence ratio I496/I560 kept stable in aqueous solution for at least 90 min, implying good stability of the probe (Fig. 2a). In the presence of 50 equiv. of N2H4, I496/I560 increased over time and kept stable after 1 h (Fig. 2a, S1†). Next, the effect of pH was investigated (Fig. 2b). CMSM was stable over a wide pH range (pH 4.0–10.0). In the presence of N2H4, not any response was detected in test solutions with pH 4.0 to 6.0; As pH of the test solution varied from 6.8 to 10.0, I496/I560 became increasingly larger. This could be due to the high nucleophilicity of N2H4 under alkaline conditions (pKa = 7.9). Finally, the selectivity and anti-interference ability of the probe was investigated (Fig. 2c). Various amines, cations, anions and amino acids brought no obvious fluorescence changes. CMSM could also react with N2H4 effectively in the presence of above species, implying good anti-interference property.
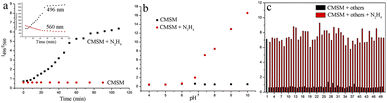 |
| Fig. 2 (a) The variations of I496/I560 as time went on. Inset: the corresponding variations of fluorescence intensity at 496 nm and 560 nm, respectively. (b) The effect of pH on the stability and reactivity of probe CMSM. (c) The selectivity and anti-interference of probe CMSM: (1) N2H4, (2) NH3·H2O, (3) CH3(CH2)3NH2, (4) (CH3)3CNH2, (5) DGA, (6) histamine, (7) tyramine, (8) tryptamine, (9) HN(CH3)2, (10) N(CH2CH3)3, (11) pyridine, (12) p-phenylenediamine, (13) urea, (14) thiourea, (15) isoniazid, (16) NaClO, (17) H2O2, (18) KNO3, (19) NaNO2, (20) NaF, (21) NaCl, (22) NaBr, (23) KI, (24) CH3COONa, (25) NaHCO3, (26) Na2CO3, (27) Na2SO4, (28) NaHSO3, (29) Na2S2O3, (30) Na2S, (31) MgSO4, (32) Al2(SO4)3, (33) FeSO4, (34) FeCl3, (35) ZnSO4, (36) CuSO4, (37) CoCl2, (38) CaCl2, (39) BaCl2, (40) NiCl2, (41) Cys, (42) Hcy, (43) GSH, (44) Arg, (45) Lys, (46) His, (47) Gly, (48) Pro, (49) Val, (50) Glu. CMSM: 1 μM; N2H4: 0 or 50 μM; others: 50 μM. | |
Mechanism of reaction between CMSM and N2H4
Firstly, the UV-vis absorption spectra of CMSM in the absence or presence of N2H4 were recorded (Fig. S2†). CMSM showed an absorption band peaked at 464 nm. After reaction with N2H4, the absorption band blue-shifted to 396 nm. This indicated that the large conjugated structure of CMSM was interrupted, whereas the conjugated structure of the coumarin backbone was unchanged.44 Thus the target site of N2H4 might be the dicyanovinyl moiety. To further clarify the reaction mechanism, HR-MS spectrum of the probe in the presence of N2H4 was recorded (Fig. S3†). The signal at m/z 361.1684 could be ascribed to [CMSM@N2H4 + H]+, implying the existence of CMSM@N2H4. Moreover, in the 1H NMR spectrum of CMSM in the presence of N2H4·H2O, proton 1 shifted from δ 7.604 to 7.831. A new band appeared at δ 5.600, which could be ascribed to protons 2 (Fig. 3). From the whole 1H NMR spectrum, the formation of CMSM@N2H4 could be clearly identified. The proposed response mechanism was shown in Scheme 2.
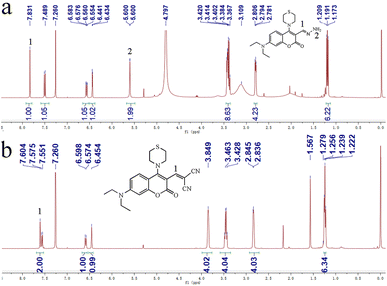 |
| Fig. 3 The 1H NMR spectra of CMSM in the presence (a) or absence (b) of N2H4. | |
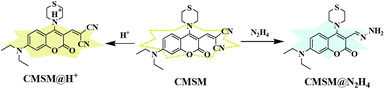 |
| Scheme 2 The proposed detection mechanisms of CMSM toward N2H4 and strong acidity. | |
Detection of N2H4 in air, soil and live cells
On TLC plate or cotton swab, probe CMSM was used to sense N2H4 vapor (Fig. 4a and b). The probe emitted light yellow fluorescence; after fumigated with N2H4 vapor, obvious blue fluorescence was observed. CMSM was further applied to sense N2H4 in soil samples (Fig. 4c). In the muddy water or filtered water of soil 1 which treated with CMSM, moderate greenish-yellow fluorescence was observed. In the muddy water or filtered water of soil 2 which treated with N2H4 and CMSM in sequence, strong greenish-blue fluorescence was observed. Therefore, CMSM could be used as a powerful tool for on-site detection of N2H4 in air and soil.
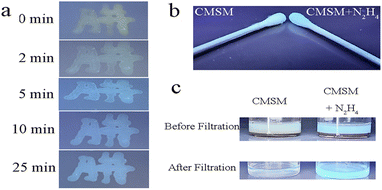 |
| Fig. 4 The visual changes of probe loaded silica TLC plate (a) or cotton swabs (b) upon fumigated by N2H4 vapor. (c) The detection of N2H4 in soil samples. All the photos were taken under a UV lamp (365 nm). | |
Probe CMSM showed low toxicity toward live HeLa cells (Fig. S4†). Then the probe was used to detect intracellular N2H4 (Fig. S5†). HeLa cells were incubated with CMSM for 30 min and images were taken, which showed moderate fluorescence in blue and yellow channel, respectively. The cells were further incubated with N2H4 and images were captured every 15 min. It could be seen that the yellow fluorescence weakened, and the blue fluorescence increased gradually as a function of time. These were consistent with the results in aqueous solution. The fluorescence intensity ratio of the blue/yellow channels changed significantly with the prolonged incubation time (Fig. S6†). As reported, N2H4 could be produced in live cells from enzymatic conversion of isoniazid.45,46 So CMSM was used to detect endogenous N2H4 in live cells (Fig. 5). Cells were incubated with isoniazid and CMSM by turn. The images were taken every 1 h. As could be seen, the fluorescence variations were significant. Therefore, probe CMSM was a desirable tool in detecting exo and endogenous N2H4.
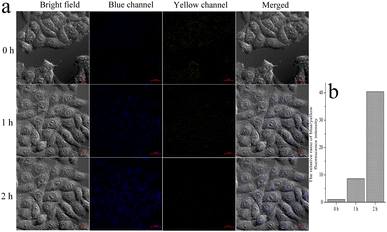 |
| Fig. 5 (a) The images of probe loaded HeLa cells incubated with isoniazid for different time. CMSM: 1 μM; isoniazid: 100 μM. (b) The relative fluorescence intensity ratios of blue and yellow channels in (a). | |
Detection of strong acidity by CMSM
Based on the fact that CMSM showed no obvious fluorescence variations from pH 4.0 to 10.0, the fluorescence spectra of CMSM in a wider pH range (1.0–11.0) were recorded. The fluorescence intensity increased greatly when pH varied from 2.0 to 1.2 (Fig. 6a, S7†). The fluorescence intensity at pH 1.2 was 59 times than that at pH 7.0, whereas the emission band peaked at 563 nm remained unchanged. The pKa value of CMSM was determined to be 1.62 by Henderson–Hasselbach equation (Fig. 6b). In addition, excellent reversibility was obtained between pH 8.0 and pH 1.2 (Fig. 6c). Potential competing anions and cations put no notable changes in the fluorescence spectra of CMSM at pH 1.2 (Fig. 6d). All above results demonstrated that CMSM was an effective tool for monitoring strong acidity in aqueous solution, with high sensitivity, good linearity, low pKa value, excellent reversibility and anti-ion interference ability.
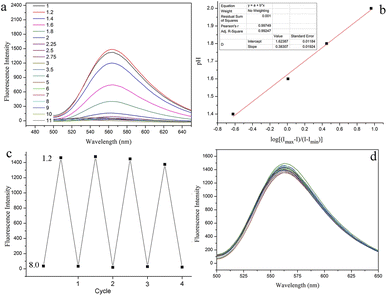 |
| Fig. 6 (a) The fluorescence spectra of CMSM (1 μM) under different pH (1.0–11.0). (b) pKa plot of pH vs. log[(Imax − I)/(I − Imin)]. (c) The reversibility curves of CMSM between pH 8.0 and pH 1.2. (d) The fluorescence spectra of probe CMSM (1 μM) in the presence of various anions and cations (Mg2+, Al3+, Fe2+, Fe3+, Zn2+, Cu2+, Co2+, Ca2+, Ba2+, Ni2+, ClO−, H2O2, NO3−, NO2−, F−, Cl, Br−, I−, OAc−, HCO3−, SO42−, HSO3−, S2O32− or S2−, 30 μM) at pH 1.2. | |
To elucidate the sensing mechanism, the optimized structure of CMSM was divided into the fluorophore (F) and thiomorpholine (T) (Fig. 7). The highest occupied molecular orbital (HOMO) and the lowest unoccupied molecular orbital (LUMO) energies of F were −5.95 eV and −2.99 eV, respectively. The HOMO energy of T was calculated to be −5.94 eV. Therefore, electron transfer could take place from T to F when CMSM was excited to induce fluorescence quenching. However, when T was protonated, the HOMO energy of T-H+ reduced to −6.84 eV. Therefore, electron transfer from T-H+ to F would be blocked. As a result, the fluorescence intensity at 563 nm enhanced remarkably due to the inhibition of PET.
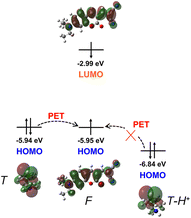 |
| Fig. 7 Energy levels and spatial distributions of the frontier molecular orbitals (HOMO and LUMO) for thiomorpholine (T), fluorophore (F) and thiomorpholine-H+ (T-H+) based on DFT-D calculations. | |
Conclusions
A new fluorescent probe was synthesized via combination of a coumarin fluorophore, a dicyanovinyl and a thiomorpholine moiety. In neutral or alkaline conditions, the probe could detect N2H4 in a ratiometric manner, which was used to detect N2H4 in air, soil samples and live cells. In addition, in strong acidic conditions, the fluorescence of probe CMSM increased significantly with the pKa to be 1.62. The protonation of the thiomorpholine was supposed to be responsible for the great fluorescence enhancement by inhibiting the PET process. We anticipated that the proposed probe here may serve as a useful detection tool and inspire the design of other simple but effective multifunctional probes.
Conflicts of interest
There are no conflicts to declare.
Acknowledgements
The work was supported by the National Natural Science Foundation of China (21907058), Natural Science Foundation of Shandong Province (ZR2022QB175) and the startup fund of Shandong University of Technology (4041/417050).
Notes and references
- G. D. Byrkit and G. A. Michalek, Ind. Eng. Chem., 1950, 42, 1862–1875 CrossRef CAS.
- A. Serov and C. Kwak, Appl. Catal., B, 2010, 98, 1–9 CrossRef CAS.
- Y. H. Kao, C. H. Chong, W. T. Ng and D. Lim, Occup. Med., 2007, 57, 535–537 CrossRef PubMed.
- F. Sun, J. Qin, Z. Wang, M. Yu, X. Wu, X. Sun and J. Qiu, Nat. Commun., 2021, 12, 4182 CrossRef CAS PubMed.
- A. Agrawal and K. K. Sahu, J. Hazard. Mater., 2009, 171, 61–75 CrossRef CAS PubMed.
- L. Zhang, L. Zhang, H. Deng, H. Li, W. Tang, L. Guan, Y. Qiu, M. J. Donovan, Z. Chen and W. Tan, Nat. Commun., 2021, 12, 2002 CrossRef CAS PubMed.
- H. Fang, Y. Chen, Z. Jiang, W. He and Z. Guo, Acc. Chem. Res., 2023, 56, 258–269 CrossRef CAS PubMed.
- S. Zhang, H. Zheng, L. Yang, Z. Li and M. Yu, Anal. Chem., 2023, 95, 5377–5383 CrossRef CAS PubMed.
- H. Niu, J. Liu, H. M. O'Connor, T. Gunnlaugsson, T. D. James and H. Zhang, Chem. Soc. Rev., 2023, 52, 2322–2357 RSC.
- C. Yan, J. Dai, Y. Yao, W. Fu, H. Tian, W.-H. Zhu and Z. Guo, Nat. Protoc., 2023, 18, 1316–1336 CrossRef CAS PubMed.
- H. Chu, L. Yang, L. Yu, J. Kim, J. Zhou, M. Li and J. S. Kim, Coord. Chem. Rev., 2021, 449, 214208 CrossRef CAS.
- Y. Li, Q. Chen, X. Pan, W. Lu and J. Zhang, Top. Curr. Chem., 2022, 380, 22 CrossRef CAS PubMed.
- Y. Ban, R. Wang, Y. Li, Z. An, M. Yu, C. Fang, L. Wei and Z. Li, New J. Chem., 2018, 42, 2030–2035 RSC.
- X.-W. Hu, M.-H. Zhang, J.-Y. Cheng, R.-J. Man and D.-D. Li, Anal. Chim. Acta, 2021, 1172, 338504 CrossRef CAS PubMed.
- Y. Chen, W. Mo, Z. Cheng, F. Kong, C. Chen, X. Li and H. Ma, Dyes Pigm., 2022, 198, 110004 CrossRef CAS.
- J. Han, X. Yue, J. Wang, Y. Zhang, B. Wang and X. Song, Chin. Chem. Lett., 2020, 31, 1508–1510 CrossRef CAS.
- Y. Jung, I. G. Ju, Y. H. Choe, Y. Kim, S. Park, Y. M. Hyun, M. S. Oh and D. Kim, ACS Sens., 2019, 4, 441–449 CrossRef CAS PubMed.
- J. Wu, J. Pan, Z. Ye, L. Zeng and D. Su, Sens. Actuators, B, 2018, 274, 274–284 CrossRef CAS.
- X.-Y. Qiu, S.-J. Liu, Y.-Q. Hao, J.-W. Sun and S. Chen, Spectrochim. Acta, Part A, 2020, 227, 117675 CrossRef CAS PubMed.
- X. Li, M. Li, Y. Chen, G. Qiao, Q. Liu, Z. Zhou, W. Liu and Q. Wang, Chem. Eng. J., 2021, 415, 128975 CrossRef CAS.
- Y. Zhou, F. Zeng, Y. Liu, M. Li, S. Xiao, J. Yan, N. Zhang and K. Zheng, Tetrahedron Lett., 2018, 59, 3253–3257 CrossRef CAS.
- X. Kong, M. Li, Y. Zhang, Y. Yin and W. Lin, Sens. Actuators, B, 2021, 329, 129232 CrossRef CAS.
- M. Luo, Q. Li, P. Shen, S. Hu, J. Wang, Z. Wu and Z. Su, Anal. Bioanal. Chem., 2021, 413, 7541–7548 CrossRef CAS PubMed.
- H. Yan, F. Huo, Y. Yue, J. Chao and C. Yin, Analyst, 2020, 145, 7380–7387 RSC.
- W. Zhang, F. Huo, T. Liu and C. Yin, J. Mater. Chem. B, 2018, 6, 8085–8089 RSC.
- A. Ghorai, J. Mondal, S. Bhattacharya and G. K. Patra, Anal. Methods, 2015, 7, 10385–10393 RSC.
- A. B. Brown, T. L. Gibson, J. C. Baum, T. Ren and T. M. Smith, Sens. Actuators, B, 2005, 110, 8–12 CrossRef CAS.
- M. Na, Y. Han, Y. Chen, S. Ma, J. Liu and X. Chen, Anal. Chem., 2021, 93, 5185–5193 CrossRef CAS PubMed.
- K. Bamnavat, V. Bhardwaj, T. Anand, S. K. A. Kumar and S. K. Sahoo, Dyes Pigm., 2021, 184, 108844 CrossRef CAS.
- Z. Wang, T. Pan, Y. Tian and J. Liao, J. Mater. Chem. B, 2022, 10, 7045–7051 RSC.
- Q. Xia, S. Feng, J. Hong and G. Feng, Sens. Actuators, B, 2021, 337, 129732 CrossRef CAS.
- L. Zhang, J. Guo, Q. You and Y. Xu, Anal. Methods, 2023, 15, 3057–3063 RSC.
- Y. Yue, F. Huo, S. Lee, C. Yin and J. Yoon, Analyst, 2017, 142, 30–41 RSC.
- X. Tian, L. C. Murfin, L. Wu, S. E. Lewis and T. D. James, Chem. Sci., 2021, 12, 3406–3426 RSC.
- J. Chao, Y. Liu, J. Sun, L. Fan, Y. Zhang, H. Tong and Z. Li, Sens. Actuators, B, 2015, 221, 427–433 CrossRef CAS.
- S. Wang, Z. Zhang, Z. Huang, X. Lei, Y. Wang, L. Li, L. Yang, H. Liu, F. Sun and L. J. Ma, J. Photochem. Photobiol., A, 2021, 418, 113438 CrossRef CAS.
- T. Liu, Z. Huang, R. Feng, Z. Ou, S. Wang, L. Yang and L. J. Ma, Dyes Pigm., 2020, 174, 108102 CrossRef CAS.
- Z. Wang, Y. Zhang, M. Li, Y. Yang, X. Xu, H. Xu, J. Liu, H. Fang and S. Wang, Tetrahedron, 2018, 74, 3030–3037 CrossRef CAS.
- D. Cao, Z. Liu, P. Verwilst, S. Koo, P. Jangjili, J. S. Kim and W. Lin, Chem. Rev., 2019, 119, 10403–10519 CrossRef CAS PubMed.
- J. Han, S. Yang, B. Wang and X. Song, Anal. Chem., 2021, 93, 5194–5200 CrossRef CAS PubMed.
- T. Liu, L. Yang, W. Feng, K. Liu, Q. Ran, W. Wang, Q. Liu, H. Peng, L. Ding and Y. Fang, ACS Appl. Mater. Interfaces, 2020, 12, 11084–11093 CrossRef CAS PubMed.
- X. Yang, Y. Liu, Y. Wu, X. Ren, D. Zhang and Y. Ye, Sens. Actuators, B, 2017, 253, 488–494 CrossRef CAS.
- D.-P. Li, F. Tang, K. Wen, Z. Yang, H. Xiao and Z. Zhou, Microchem. J., 2022, 175, 107233 CrossRef CAS.
- Z. ul Huda, A. Mansha, S. Asim and A. Shahzad, J. Fluoresc., 2022, 32, 57–66 CrossRef PubMed.
- I. G. Metushi, P. Cai, X. Zhu, T. Nakagawa and J. P. Uetrecht, Clin. Pharmacol. Ther., 2011, 89, 911–914 CrossRef CAS PubMed.
- J. Li, Y. Cui, C. Bi, S. Feng, F. Yu, E. Yuan, S. Xu, Z. Hu, Q. Sun, D. Wei and J. Yoon, Anal. Chem., 2019, 91, 7360–7365 CrossRef CAS PubMed.
|
This journal is © The Royal Society of Chemistry 2023 |
Click here to see how this site uses Cookies. View our privacy policy here.