DOI:
10.1039/D3RA06526J
(Paper)
RSC Adv., 2023,
13, 33459-33462
Complexation study of a 1,3-phenylene-bridged cyclic hexa-naphthalene with fullerenes C60 and C70 in solution and 1D-alignment of fullerenes in the crystals†
Received
25th September 2023
, Accepted 7th November 2023
First published on 14th November 2023
Abstract
To investigate the host ability of a simple macrocycle, 1,3-phenylene-bridged naphthalene hexamer N6, we evaluated the complexation of N6 with fullerenes in toluene and in the crystals. The complexes in the solid-state demonstrate the one-dimensional alignment of fullerenes. The single-crystals of the C60@N6 composite have semiconductive properties revealed by photoconductivity measurements.
Introduction
Macrocyclic arenes constitute an important class of shape-persistent host molecules.1 This system features structural rigidness, interesting optoelectronic properties, self-assembling behavior, and host–guest chemistry.2 In many cases, they form a large cavity inside to be used for fullerene recognition.3,4 Naphthalene is one of the simplest polycyclic aromatic hydrocarbons (PAHs) with a rigid π-plane, which has been incorporated into cycloarylenes.5–9 We reported a macrocyclic arene, 1,3-phenylene-bridged cyclic naphthalene hexamer N6, that could be synthesized by a straightforward one-pot Suzuki–Miyaura reaction (Fig. 1).10 The crystal structure showed that the N6 had a symmetric hexagonal structure and a large cavity (d = ca. 15 Å). This unique structural characteristic allows N6 to bind the spherical molecule C60 by forming a one-dimensional (1D)-alignment in the solid-state.10
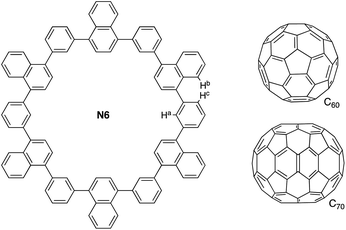 |
| Fig. 1 Structures of a cyclic naphthalene hexamer N6, and fullerenes C60 and C70. | |
Here, to examine the effects of a variation in the size and shape of fullerenes,11 we investigated the host–guest interactions between N6 and spherical C60 and ellipsoidal C70 using 1H NMR spectroscopy in solution and single-crystal X-ray diffraction analysis in the solid state. Especially, we could determine the association constants and stoichiometry of the complexation fullerene@N6 in solutions, for the first time, based on the statistical methodology.
Results and discussion
Complexation analysis in solution
In our previous report,10 we could not observe the formation of the complexes of N6 with C60 in chloroform due to the low solubility of C60. In the present study, we analyzed complexation of N6 in toluene as a better solvent for fullerenes. Recently, it is noticed that Job's plot is inappropriate for estimating stoichiometries in the presence of more than one complex.11 Therefore, we attempted titration experiments with three different host–guest association models; 1
:
1, 1
:
2 and 2
:
1 complexation.12 Solutions of N6 (0.4 mM) and C60 (0.4 mM) in toluene-d8 were mixed in different ratios from 10
:
0 to 1
:
9 to prepare 10 samples. As an example, the chemical shift of the proton Ha in Fig. 1 was illustrated: the chemical shift originally observed at 7.64 ppm was shifted to down-field at 7.88 ppm due to the host–guest interactions (Fig. 2a). The spectral features are analogous to those of other naphthalene-C60 supramolecular systems.13 This guest-binding profiles (the initial data points: 7.64, 7.53 and 7.40 ppm, 10 samples, total data points N = 30) were analyzed with the curve-fitting for 1
:
1, 1
:
2 and 2
:
1 binding systems (Fig. S2 and Table S1†).12 All the fitted curves agreed with the observed chemical shift changes. It is difficult to compare the fitted curves of these models quantitatively so further analysis was made by investigation of the goodness-of-fit (GOF). To evaluate the GOF of these models, Akaike's information criterion (AIC) statistics were applied according to the recent exercise to a similar binding system.14
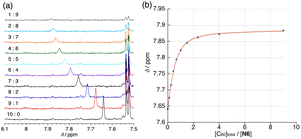 |
| Fig. 2 (a) The chemical shift changes of inside proton resonance observed in 1H NMR titration of N6 with C60 in toluene-d8. ([N6] : [C60] = 10 : 0 to 1 : 9, the total concentration was 0.4 mM, 600 MHz, 298 K) (b) curve-fitting obtained by using the 2 : 1 binding model. | |
The AIC values are summarized in Table S1.† Based on the quantitative GOF analysis given by AIC calculation, the 2
:
1 additive model is the most preferred (Fig. 2b).15 We obtained K11 = 1.69 (±0.06) × 104 M−1 and K21 = 4.54 (±0.42) × 102 M−1 for the first and second complexation, respectively. The smaller K21 value than K11 indicates that 1
:
1 complex is dominant in solution and 2
:
1 complex becomes coexistent when the concentration of the host is high (Fig. S3†).15
Previously we obtained the crystal structure of the complex of N6 and C60, which allowed us to precisely determine the 1
:
1 stoichiometry of this complex in the solid state (CCDC 1838834 for N6, 1838835 for C60@N6).10 The crystallographic analysis confirmed the π-stacking between N6 and encapsulated C60. The dihedral angles of the facing naphthalene moieties vary to maximize the interactions between the N6 and C60: upon the complexation, the dihedral angles became slightly wider from the energy minimized structure. The closest distance between the C60 and naphthalene is 3.32 Å in the range of π-stacking.
Then, we performed the titration between N6 and C70. The formation of the N6–C70 complex in toluene-d8 was clearly suggested using the 1H NMR (Fig. 3a). A curve-fitting simulation of guest-binding profile also supported a 2
:
1 model (Fig. S5 and Table S2†). We obtained K11 = 2.52 (±0.22) × 104 M−1 and K21 = 2.12 (±0.80) × 102 M−1 for the complexation (Fig. 3b). The estimated K11 binding constant was 1.5 times larger than that with C60, inevitably due to the ellipsoidal shape of C70.
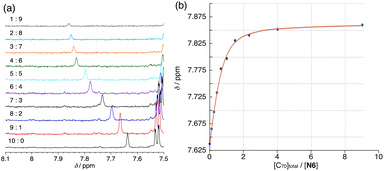 |
| Fig. 3 (a) The chemical shift changes of inside proton resonance observed in 1H NMR titration of N6 with C70 in toluene-d8. ([N6] : [C70] = 10 : 0 to 1 : 9, the total concentration was 0.4 mM, 600 MHz, 298 K) (b) curve-fitting obtained by using the 2 : 1 binding model. | |
Interestingly, the peak of N6 at 8.38 ppm assigned to Hb exhibited down-field shift upon the addition of C60, while it showed up-field shift upon the addition of C70 (Fig. S7†). The peak at 7.53 ppm assigned to Hc similarly exhibited opposite peak shifts due to the addition of C60 and C70. These results illustrate that the angles between naphthalene and phenylene respond to the C60 and C70 encapsulation with smaller and larger sizes, respectively.
Single-crystal X-ray analysis
Fortunately, we obtained the composite structure of N6–C70 by single-crystal X-ray diffraction analysis despite low resolution (>1.0 Å) due to very weak diffraction at the high θ angle (Fig. 4).|| Single-crystals of N6–C70 composite were obtained by vapor diffusion of MeOH into a chlorobenzene solution. C70 in the crystal is nicely captured within the cavity made by N6 with intermolecular distances in the range of 3.2–3.4 Å. Closer inspection of the crystal structure revealed that N6 keeps a 1
:
1 complex with C70 similar to N6–C60 with the dihedral angles of naphthalene toward phenylene (51° and 72°). The fullerene moiety occupied at the special position refined by applying appropriate instructions. As shown in Fig. 4c, the complex also consequently forms the directly-contacting one-dimensional C70 array along the crystallographic a-axis. The long-axis of the C70 is tilted to the alignment direction by 33°, which is expected to increase the contact area between two fullerenes and thus to increase the interaction strengths. The closest C–C distance between fullerenes in the array is 4.1 Å, suggesting the larger electronic interaction between fullerenes than that in the N6–C60 composite (4.4 Å). In addition, the C–H⋯π interactions between the hydrogen atoms of the naphthalene units and the C70 also contribute to the stabilization of the N6–C70 assembly.
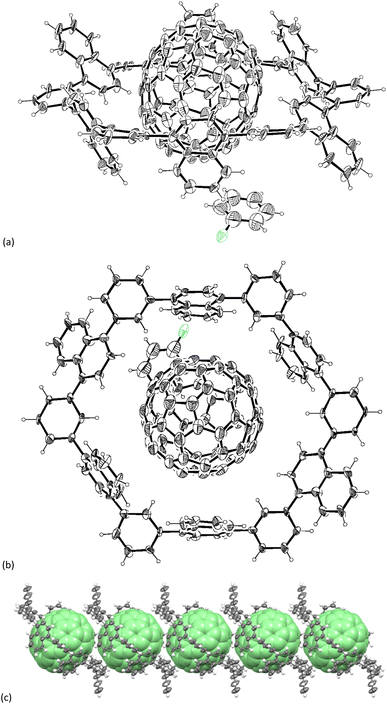 |
| Fig. 4 X-ray structure of the N6–C70 1 : 1 complex, ORTEP drawing from (a) the side view and (b) top view with thermal ellipsoids scaled at 25% probability. Disordered molecules are omitted for clarity. (c) A columnar array of N6–C70 along the a-axis. For clarity, C70 is shown as a space-filling model. | |
Photoconductivity measurements
The structures of N6–C60 and N6–C70 are expected to have large intermolecular orbital couplings. To discuss charge transport property in detail, the charge transfer integrals of the HOMOs (Vhole) and LUMOs (Velectron) between the neighbouring C60 and C70 pairs were calculated based on the crystal structures using ADF program16 (Fig. S9†). Along the crystallographic a-axis, the Vhole and Velectron values for C60 pairs were calculated to be 3.1 and 3.0 meV, respectively. On the other hand, the Vhole and Velectron values for C70 pairs were 10.4 and 15.8 meV, respectively, higher than those of C60. With these expecting charge–transport properties in mind, we conducted flash-photolysis time-resolved microwave conductivity (FP-TRMC) measurements of C60@N6. This electrodeless method allows for evaluating short-range (∼10 nm) transient conductivities of materials.17 With a 355 nm laser pulse at 25 °C, the pseudo-conductivity (ϕΣμmax in cm2 V−1 s−1 in which ϕ is the quantum efficiency of charge generation and Σμmax is the sum of hole and electron mobilities) of N6–C60 exhibited ϕΣμmax = 1.3 × 10−4 cm2 V−1 s−1 (Fig. 5). This value is comparable to PC61BM18 and other conjugated molecules.19 Unfortunately, for C70@N6 which was expected to exhibit better charge mobilities than N6–C60, we were unable to prepare single crystals of good enough quantity to measure the FP-TRMC.
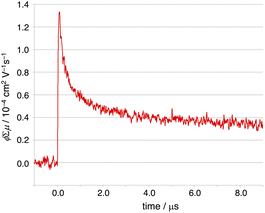 |
| Fig. 5 FP-TRMC profile of the single-crystals (C60@N6·PhCl) recorded at an excitation wavelength of 355 nm with a photon density of 9.1 ×1015 photons per cm−2. | |
Conclusions
In summary, we present the molecular host N6 can bind the fullerenes C60 and C70 in solution and in the solid-state. The NMR titration experiments and curve-fitting suggest that the binding profile between N6 and fullerenes analyzed by 2
:
1 model was most likelihood with binding constants of K11 = 1.69 (±0.06) × 104 M−1 and K21 = 4.54 (±0.42) × 102 M−1 for C60@N6 and K11 = 2.52 (±0.22) × 104 M−1 and K21 = 2.12 (±0.80) × 102 M−1 for C70@N6. In the solid-state, on the other hand, both N6–C60 and N6–C70 composites show 1
:
1 complex and make the 1D arrays of fullerenes with the aid of the N6 agent as confirmed by the single-crystal X-ray analysis. Among these, N6–C60 exhibited the moderate ϕΣμmax = 1.3 × 10−4 cm2 V−1 s−1 by FP-TRMC. We are currently investigating the host–guest chemistry of N6 with larger fullerenes, expecting it to exhibit different selectivity and affinity.
Author contributions
The manuscript was written through contributions of all authors. All authors have given approval to the final version of the manuscript.
Conflicts of interest
There are no conflicts to declare.
Acknowledgements
This work was partly supported by Grants-in-Aid for Scientific Research (No. JP20H02816 (HH), JP20H00379 (HY), JP20H05833 (HY), JP22K19067, and JP23H01787 (NA), JP22K05255 (KM), and JP20H05836 (AS), and JST, PRESTO (No. JPMJPR21AC (HH)). HM thanks University Fellowships for the Creation of Innovation in Science and Technology. We acknowledge Dr Mitsuaki Yamauchi (Kyoto University) for helpful discussions. This work was partially supported by ISHIZUE 2023 of Kyoto University.
Notes and references
- S. E. Lewis, Chem. Soc. Rev., 2015, 44, 2221–2304 RSC; Y. Segawa, H. Ito and K. Itami, Nat. Rev. Mater., 2016, 1, 15002 CrossRef CAS; M. A. Majewski and M. Stępień, Angew. Chem., Int. Ed., 2019, 58, 86–116 CrossRef PubMed.
- K. Yazaki, L. Catti and M. Yoshizawa, Chem. Commun., 2018, 54, 3195–3206 RSC; D. Lorbach, A. Keerthi, T. M. Figueira-Duarte, M. Baumgarten, M. Wagner and K. Müllen, Angew. Chem., Int. Ed., 2016, 55, 418–421 CrossRef CAS PubMed; S. Toyota and E. Tsurumaki, Chem.–Eur. J., 2019, 25, 6878–6890 CrossRef PubMed; M. Iyoda and H. Shimizu, Chem. Soc. Rev., 2015, 44, 6411–6424 RSC; X. Lu, S. Lee, Y. Hong, H. Phan, T. Y. Gopalakrishna, T. S. Herng, T. Tanaka, M. E. Sandoval-Salinas, W. Zeng, J. Ding, D. Casanova, A. Osuka, D. Kim and J. Wu, J. Am. Chem. Soc., 2017, 139, 13173–13183 CrossRef PubMed; Y. Yamamoto, K. Wakamatsu, T. Iwanaga, H. Sato and S. Toyota, Chem.–Asian J., 2016, 11, 1370–1375 CrossRef PubMed.
- D. Canevet, E. M. Pérez and N. Martín, Angew. Chem., Int. Ed., 2011, 50, 9248–9259 CrossRef CAS; Y. Yamamoto, E. Tsurumaki, K. Wakamatsu and S. Toyota, Angew. Chem., Int. Ed., 2018, 57, 8199–8202 CrossRef; H. Chen, Z. Xia and Q. Miao, Chem. Sci., 2022, 13, 2280–2285 RSC.
- T. Iwamoto, Y. Watanabe, T. Sadahiro, T. Haino and S. Yamago, Angew. Chem., Int. Ed., 2011, 50, 8342–8344 CrossRef CAS PubMed; H. Ueno, T. Nishihara, Y. Segawa and K. Itami, Angew. Chem., Int. Ed., 2015, 54, 3707–3711 CrossRef PubMed.
- V. Hensel, K. Lützow, J. Jakob, K. Gessler, W. Saenger and A. D. Schlüter, Angew. Chem., Int. Ed. Engl., 1997, 36, 2654–2656 CrossRef CAS; V. Hensel and A. D. Schlüter, Chem.–Eur. J., 1999, 5, 421–429 CrossRef.
- Z. Sun, P. Sarkar, T. Suenaga, S. Sato and H. Isobe, Angew. Chem., Int. Ed., 2015, 127, 12991–12995 CrossRef CAS; S. E. Lewis, Chem. Soc. Rev., 2015, 44, 2221–2304 RSC.
- H. A. Staab and F. Binnig, Tetrahedron Lett., 1964, 5, 319–321 CrossRef.
- J. Y. Xue, K. Ikemoto, N. Takahashi, T. Izumi, H. Taka, H. Kita, S. Sato and H. Isobe, J. Org. Chem., 2014, 79, 9735–9739 CrossRef CAS PubMed and the references are therein..
- H.-W. Jiang, S. Ham, N. Aratani, D. Kim and A. Osuka, Chem.–Eur. J., 2013, 19, 13328–13336 CrossRef CAS PubMed; Y. Nakamura, N. Aratani and A. Osuka, Chem. Soc. Rev., 2007, 36, 831–845 RSC.
- P. Mei, A. Matsumoto, H. Hayashi, M. Suzuki, N. Aratani and H. Yamada, RSC Adv., 2018, 8, 20872–20876 RSC.
- P. Thordarson, Chem. Soc. Rev., 2011, 40, 1305–1323 RSC; D. B. Hibbert and P. Thordarson, Chem. Commun., 2016, 52, 12792 RSC; F. Ulatowski, K. Dabrowa, T. Bałakier and J. Jurczak, J. Org. Chem., 2016, 81, 1746 CrossRef CAS PubMed.
- Bindfit, http://supramolecular.org Search PubMed.
- S. Mizyed, M. Ashram, D. O. Miller and P. E. Georghiou, J. Chem. Soc., Perkin Trans. 2, 2001, 10, 1916–1919 Search PubMed; X.-W. Chen, K.-Sh. Chu, R.-J. Wei, Z.-L. Qiu, C. Tang and Y.-Z. Tan, Chem. Sci., 2022, 13, 1636–1640 RSC.
- K. Ikemoto, K. Takahashi, T. Ozawa and H. Isobe, Angew. Chem., Int. Ed., 2023, 62, e202219059 CrossRef CAS PubMed.
- Even for a simple cycloparaphenylene (CPP) macrocycle, 1:1 and 2:1 complexes of [10]CPP and C60/C70 were detected in solution. M. Freiberger, M. B. Minameyer, I. Solymosi, S. Frühwald, M. Krug, Y. Xu, A. Hirsch, T. Clark, D. M. Guldi, M. von Delius, K. Amsharov, A. Görling, M. E. Pérez-Ojeda and T. Drewello, Chem.–Eur. J., 2023, 29, e202203734 CrossRef CAS PubMed.
- ADF2021, Scientific Computing & Modeling (SCM), Theoreti-cal Chemistry, Vrije Universiteit, Amsterdam, The Nether-lands, http://www.scm.com Search PubMed.
- A. Saeki, Y. Koizumi, T. Aida and S. Seki, Acc. Chem. Res., 2012, 45, 1193–1202 CrossRef CAS PubMed.
- A. Baumann, T. J. Savenije, D. H. K. Murthy, M. Heeney, V. Dyakonov and C. Deibel, Adv. Funct. Mater., 2011, 21, 1687–1692 CrossRef CAS.
- H. Yokoi, Y. Hiraoka, S. Hiroto, D. Sakamaki, S. Seki and H. Shinokubo, Nat. Commun., 2015, 6, 8215 CrossRef CAS PubMed; T. Okamoto, K. Nakahara, A. Saeki, S. Seki, J. H. Oh, H. B. Akkerman, Z. Bao and Y. Matsuo, Chem. Mater., 2011, 23, 1646–1649 CrossRef; J. Terao, Y. Tanaka, S. Tsuda, N. Kambe, M. Taniguchi, T. Kawai, A. Saeki and S. Seki, J. Am. Chem. Soc., 2009, 131, 18046–18047 CrossRef PubMed.
Footnotes |
† Electronic supplementary information (ESI) available: Detailed experimental procedures and additional spectroscopic data. CCDC: 2296635. For ESI and crystallographic data in CIF or other electronic format see DOI: https://doi.org/10.1039/d3ra06526j |
‡ . Current address: College of Chemistry and Molecular Engineering, Peking University, Beijing 100871, People's Republic of China. |
§ . Current address: Institute for Chemical Research, Kyoto University, Gokasho, Uji, Kyoto 611-0011, Japan. |
¶ . Current address: Center for Basic Research on Materials, National Institute for Materials Science (NIMS), 1-2-1 Sengen, Tsukuba, Ibaraki 305-0047, Japan. |
|| Crystallographic data for C70@N6: C96H60 C70 C6H5Cl, Mw = 2166.69, triclinic, space group P (#2), a = 11.75(4), b = 14.98(5), c = 15.88(5) Å α = 106.46(3), β = 101.62(3), γ = 98.45(3)°, V = 2563(14) Å3, T = 103(2) K, Z = 1, reflections measured 5549, 4553 unique. The final R1 was 0.1092 (I > 2σ(I)), and the final wR on F2 was 0.4205 (all data), GOF = 1.084. CCDC: 2296635 |
|
This journal is © The Royal Society of Chemistry 2023 |
Click here to see how this site uses Cookies. View our privacy policy here.