DOI:
10.1039/D3RA05826C
(Paper)
RSC Adv., 2023,
13, 27167-27173
Exploring the anti-obesity bioactive compounds of Thymelaea hirsuta and Ziziphus spina-christi through integration of lipase inhibition screening and molecular docking analysis†
Received
26th August 2023
, Accepted 4th September 2023
First published on 11th September 2023
Abstract
Activity-guided fractionation of the ethanolic extracts of Thymelaea hirsuta and Ziziphus spina-christi furnished eight compounds with pancreatic lipase inhibitory activity. Six compounds were isolated from the chloroform fraction of T. hirsuta. It is worth mentioning that this is the first report for the isolation of 5,7,4′-trihydroxy-8-methoxycarbonyl flavanol (2), daphnodorin G-3′′-methyl ether (4) and daphnodorin G (5) from genus Thymelaea. Moreover, daphnoretin (1), neochamaejasmin A (3) and daphnodorin B (6) were also isolated from the chloroform fraction of the same plant. On the other hand, quercetin 3-O-α-L-rhamnopyranosyl-(1 → 2)-[α-L-rhamnopyranosyl-(1 → 6)]-β-D-galactopyranoside (7) and 3-O-[α-L-fucopyranosyl-(1 → 2)-β-D-glucopyranosyl-(1 → 3)-α-L-arabinopyranosyl] jujubogenin (christinin A) (8) were isolated from the n-butanol fraction of Z. spina-christi. Structure elucidation of the isolated compounds was carried out by detailed analysis of 1D and 2D spectral data. These compounds showed percentage inhibitions of 72% (1), 52% (2), 61.8% (3), 39% (4), 69.5% (5), 3.5% (6), 68% (7) and 75% (8) at the concentration of 250 μM and XP-G scores of lipase inhibition were 11.40 (1), 8.71 (2), 6.13 (3), 8.23 (4), 6.22 (5), 9.76 (6), 14.66 (7) and 12.00 (8). This is the first report of the isolation of lipase inhibitors from both plants T. hirsuta and Z. spina-christi. In addition to that, this might result in presenting the biscoumarin, daphnoretin, and the dammarane saponin, christinin A, as potent lipase inhibitors.
1. Introduction
Obesity is a “New World Syndrome”. It is considered as a risk factor for chronic diseases including, cardiovascular diseases, type 2 diabetes, hypertension, and stroke.1,2
Pancreatic lipase is the principal lipolytic enzyme synthesized and secreted by the pancreas which is responsible for digestion of 50–70% of dietary fats.3–5 Reduction of the fat digestion and hence, the absorption of dietary lipids in the gastrointestinal tract through the inhibition of pancreatic lipase is an important approach for the treatment of obesity.5–9
Orlistat (a semi-synthetic hydrogenated derivative of lipstatin) is considered a potent inhibitor of lipase enzyme, as it inhibits the absorption of 30% of dietary fats.10,11 It has been approved by the Food and Drug Administration as an effective treatment of human obesity.8,12 However, orlistat showed some serious side effects like steatorrhea, fecal incontinence, flatulence, risks of vitamin deficiencies and liver diseases.13–15 It is contraindicated in pregnancy, patients with malabsorption disorders and reduced gallbladder function.16 Consequently, there is a need to explore safer alternative and complementary therapies to combat obesity.17
Many researchers reported potentiation of phyto-compounds and their anti-lipase effect.18–21 This has prompted us to investigate Thymelaea hirsuta and Ziziphus spina-christi for their lipase inhibitory activities.
Thymelaea hirsuta (L.), called “Al Methnan” (Thymelaeaceae). It is a small evergreen shrub which is native to the Mediterranean region. This genus has not been investigated extensively but it is characterized by the presence of flavonoids (luteolin, trans-tiliroside, chrysoeriol), terpenes (daphnane terpenes), sterols and coumarins (daphnoretin).22–24 It is used traditionally for treatment of diabetes,25,26 dermatitis, hair-fall, constipation and vermicide. Furthermore, the aerial parts were reported to be effective inhibitors of α-glucosidase in vivo.27,28
On other hand, Ziziphus spina-christi (Rhamnaceae) is known in Egypt as ‘Nabq’ or ‘Sidr’,29 it is a tropical tree with thorny branches and producing small, orange-yellow fruits. It is used in folk medicine for the treatment of diabetes mellitus. Many studies showed improved in vitro effect of Z. spina-christi extract on the activity of α-amylase enzyme resulted in significant reduction in blood glucose level together with significant rise in serum insulin.30,31 Literature survey of Z. spina-christi species revealed the presence of different types of saponins (betulinaldehyde, betulin, christinin A),32–34 flavonoids (quercetin, quercetin 3-O-[β-xylosyl-(1 → 2)-α-rhamnoside]-4′-O-α-rhamnoside, quercetin 3-O-α-rhamnopyranosyl-(1 → 6)-α rhamnopyranosyl-(1 → 2)-β-galactopyranoside, quercetin-3-O-α-arabinosyl(1 → 2)-α-rhamnoside, gallocatechin32 and phenolics (p-hydroxybenzoic)).
Molecular docking studies are among the computational techniques which provided information about the interaction between ligand and the protein binding site; leading to fast and efficient development of potent target modulators.35 So that, in silico and in vitro studies will be conducted on isolates from the two selected Egyptian plants to evaluate their lipase inhibitory activity as well as prediction of their pharmacokinetic ADMET properties.
2. Materials and methods
2.1. General
Rotary evaporator: BUCHI Rotavapor R-200, was used for evaporation of solvents under reduced pressure. TLC analysis was conducted on silica gel plates (silica gel 60 F254 with adsorbent layer thickness 0.25 mm plates, Merck, Darmstadt, Germany). TLC spots were located using a UV lamp and by heating after spraying with acidic anisaldehyde. Silica gel (Kieselgel 60, 0.063–0.20 mm, Merck, Darmstadt, Germany) and Sephadex LH-20 (Amersham Pharmacia Biotech AB) were used for column chromatography. 1D (1H and 13C) and 2D NMR (HSQC, HMBC, NOESY, and COSY) spectra were recorded on Bruker DRX-400 MHz Ultrashield spectrometers (Bruker BioSpin, Billerica, MA, USA) in DMSO-d6 or CD3OD solution. 1H chemical shifts are referenced to the residual proton signal of the solvents (δ 2.49 or 3.30, respectively), while 13C chemical shifts are expressed in reference to the solvent signals (δ 39.5 or 49.0, respectively). RP-MPLC was carried out with a BUCHI Pure C-815 Flash chromatograph apparatus attached with packed column, silica gel 100 C18 reversed phase, 0.04–0.063 mm column (250 mm × 20 mm i.d.) monitored with a UV detector (254 nm). UV absorptions of the in vitro assay were measured on a BioTek, 800 TS Microplate spectrophotometer. RADWAG® AS 220.R2 sensitive balance was used for weighing solid materials. JENWAY 3510 PH Meter was used for adjusting the buffer PH. Incubator BT 1500 (BTC, Egypt), was used for incubation at 37 °C. Pancreatic lipase (type II, 100–500 units per mg protein), para nitrophenyl palmitate (lipase substrate) were purchased from Sigma Chemicals (USA) and orlistat (a lipase inhibitor used as positive control for the in vitro lipase inhibitory assay) was obtained from European Egyptian Pharmaceuticals (Egypt).
2.2. Plant material
Both plants Ziziphus spina christi (aerial parts) and Thymelaea hirsuta (roots) were collected from the Egyptian Siwa desserts and Ras El Hikma desserts, Matrouh, respectively, in March 2018. All plant were identified by Prof. Selim Heneidy at the Herbarium, Faculty of Science, Alexandria University, Egypt. Voucher specimens (TH004 and TH007 for Z. spina christi and T. hirsuta, respectively) were deposited in the Pharmacognosy Department, Faculty of Pharmacy, Alexandria University, Egypt.
2.3. Extraction and isolation
Aerial parts of Z. spina-christi and roots of T. hirsuta (350 g of each plant) were dried, ground, extracted by percolation at room temperature with 90% ethanol until exhaustion. The solvent was evaporated under reduced pressure yielding a solid residue. Also, dried roots of T. hirsuta (1.7 kg) were extracted with 70% ethanol, filtered and concentrated. The alcoholic extract of Z. spina christi and T. hirsuta subjected to pancreatic lipase inhibition assay. Then, each residue was suspended in ethanol
:
water 1
:
1 v/v and partitioned with organic solvents of increasing polarities. Four fractions; light petroleum, chloroform, ethyl acetate and n-butanol were subjected to pancreatic lipase inhibition assay. The most bioactive fractions were the chloroform fraction and n-butanol fraction of Z. spina-christi and roots of T. hirsute, respectively. The chloroform fraction was chromatographed using normal silica gel column and purified repeatedly with sephadex LH columns and crystallization was performed to afford six compounds (1–6) (Fig. 1). Compounds 1–6 were identified as daphnoretin (1), 5,7,4′-trihydroxy-8-methoxycarbonyl flavanol (2),36 neochamaejasmin A (3),37 daphnodorin G-3′′-methyl ether (4), daphnodorin G (5),38 daphnodorin B (6)39 by detailed analysis of NMR data. It is worth mentioning that this is the first report for the isolation of the compounds (2), (4) and (5) from genus Thymelaea. On the other hand, the butanolic fraction was subjected to further purification of subfractions using column chromatography and crystallization was performed to afford two compounds (7–8) (Fig. 1). Compounds 7–8 were identified as quercetin 3-O-α-L-rhamnopyranosyl-(1 → 2)-[α-L-rhamnopyranosyl-(1 → 6)]-β-D-galactopyranoside (7)40 and 3-O-[α-L-fucopyranosyl-(1 → 2)-β-D-glucopyranosyl-(1 → 3)-α-L-arabinopyranosyl]jujubogenin (christinin A) (8)41 by detailed analysis of NMR data and agreement of the spectral data to those reported in literature.
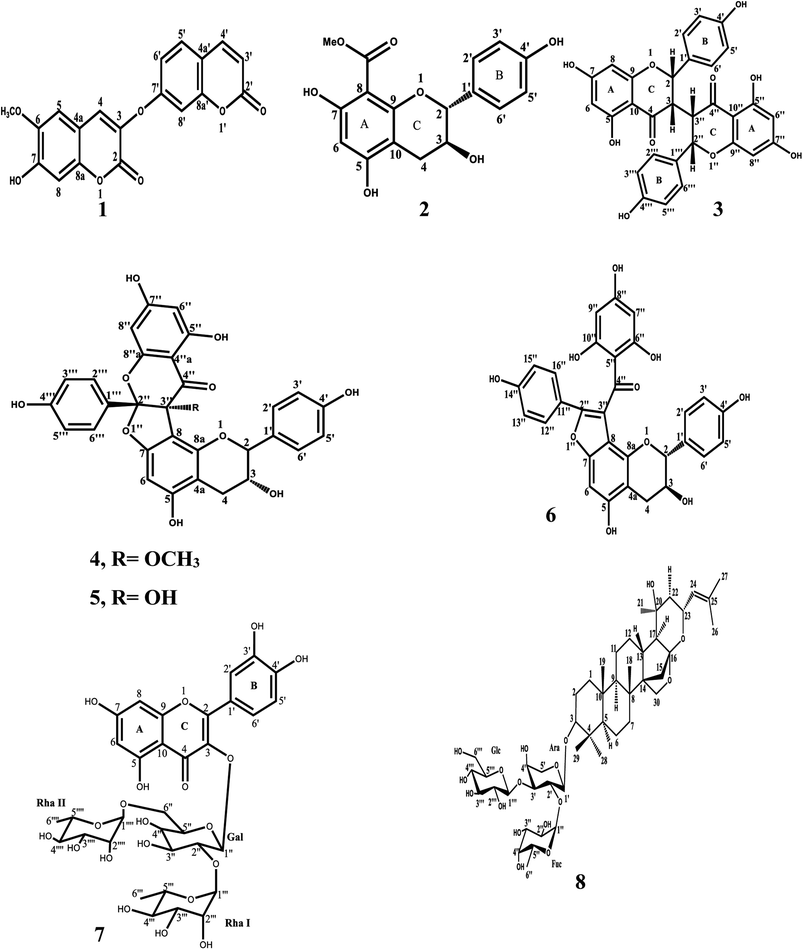 |
| Fig. 1 Structures of compounds 1–8. | |
The detailed spectral analysis and experimental procedure for lipase inhibition assay are given as ESI.†
5,7,4′-Trihydroxy-8-methoxycarbonyl flavanol (2). White crystals, m.p. °C: 221–222° (MeOH), 1H-NMR (400 MHz, DMSO-d6) δ: 4.75 (1H, d, J = 6.7 Hz, H-2), 3.88 (1H, m, H-3), 2.60 (1H, dd, J = 16.2, 5.2 Hz, H-4), 2.41(1H, dd, J = 16.2, 7.2 Hz, H-4), 6.00 (1H, s, H-6), 7.16 (2H, d, J = 8.3 Hz, H-2′), 6.74 (2H, d, J = 8.3 Hz, H-3′), 6.74 (2H, d, J = 8.3 Hz, H-5′), 7.16 (2H, d, J = 8.3 Hz, H-6′), 3.68 (3H, s, OC
3), 5.08 (1H, br. s, HO-3), 10.54 (1H, s, HO-5), 10.54 (1H, s, HO-7), 9.46 (1H, s, HO-4′); 13C-NMR (100 MHz, DMSO-d6) δ: 81.1 (C-2), 66.9 (C-3), 27.3 (C-4), 159.8 (C-5), 96.3 (C-6), 158.7 (C-7), 98.4 (C-8), 154.8 (C-9), 100.1 (C-10), 130.0 (C-1′), 128.1 (C-2′), 115.2 (C-3′), 157.1 (C-4′), 115.2 (C-5′), 128.1 (C-6′), 169.4 (C
O), 52.0 (OC
H3).
Daphnodorin G-3′′-methyl ether (4). Amorphous yellow powder, 1H-NMR (400 MHz, DMSO-d6) δ: 4.78 (1H, d, J = 6.4 Hz, H-2), 3.88 (m, H-3), 5.12 (1H, d, J = 4.6 Hz, HO-3), 2.56 (1H, dd, J = 5.1, 16.3 Hz, H-4), 2.46 (1H, dd, J = 7.0, 16.3 Hz, H-4), 6.29 (1H, s, H-6), 6.91 (2H, d, J = 8.8 Hz, H-2′, 6′), 6.59 (2H, d, J = 8.8 Hz, H-3′, 5′), 3.18 (3H, s, OC
3), 5.99 (1H, d, J = 2.2 Hz, H-6′′), 5.80 (1H, d, J = 2.2 Hz, H-8′′), 7.23 (2H, d, J = 8.8 Hz, H-2′′′, 6′′′), 6.77 (2H, d, J = 8.8 Hz, H-3′′′, 5′′′), 11.70 (1H, s, HO), 10.23(1H, s, HO), 9.80 (1H, s, HO), 9.41(1H, s, HO), 9.41(1H, s, HO); 13C-NMR (100 MHz, DMSO-d6) δ: 80.4 (C-2), 65.7 (C-3), 27.3 (C-4), 100.7 (C-4a), 159.6 (C-5), 91.8 (C-6), 160.1 (C-7), 102.5 (C-8), 153.4 (C-8a), 129.6 (C-1′), 127.5 (C-2′, 6′), 115.3 (C-3′, 5′), 157.1 (C-4′), 117.5 (C-2′′), 85.0 (C-3′′), 54.4 (O
H3), 193.1 (C-4′′), 99.0 (C-4′′a), 163.8 (C-5′′), 96.7 (C-6′′), 167.2 (C-7′′), 94.9 (C-8′′), 161.0 (C-8′′a), 124.7 (C-1′′′), 128.1 (C-2′′′, 6′′′), 115.3 (C-3′′′, 5′′′), 158.9 (C-4′′′).
Daphnodorin G (5). Amorphous yellow powder, 1H-NMR (400 MHz, DMSO-d6) δ: 4.71 (1H, d, J = 6.9 Hz, H-2), 3.76 (m, H-3), 5.07 (1H, d, J = 5.1 Hz, HO-3), 2.59 (1H, dd, J = 5.2, 16.4 Hz, H-4), 2.43 (1H, dd, J = 7.8, 16.4 Hz, H-4), 6.27 (1H, s, H-6), 6.93 (2H, d, J = 8.2 Hz, H-2′, 6′), 6.62 (2H, d, J = 8.2 Hz, H-3′, 5′), 6.22 (1H, s, 3′′-HO), 5.98 (1H, d, J = 2.2 Hz, H-6′′), 5.87 (1H, d, J = 2.2 Hz, H-8′′), 7.22 (2H, d, J = 8.5 Hz, H-2′′′, 6′′′), 6.76 (2H, d, J = 8.5 Hz, H-3′′′, 5′′′), 11.77 (1H, s, HO), 10.01 (1H, s, HO), 9.74 (1H, s, HO), 9.38 (1H, s, HO), 9.38 (1H, s, HO); 13C-NMR (100 MHz, DMSO-d6) δ: 80.4 (C-2), 66.2 (C-3), 28.02 (C-4), 102.6 (C-4a), 158.8 (C-5), 91.5 (C-6), 159.5 (C-7), 106.4 (C-8), 151.8 (C-8a), 129.8 (C-1′), 127.8 (C-2′, 6′), 115.0 (C-3′, 5′), 157.0 (C-4′), 117.8 (C-2′′), 80.9 (C-3′′), 192.9 (C-4′′), 98.8 (C-4′′a), 163.8 (C-5′′), 96.7 (C-6′′), 167.4 (C-7′′), 94.9 (C-8′′), 161.4 (C-8′′a), 124.7 (C-1′′′), 128.6 (C-2′′′, 6′′′), 115.1 (C-3′′′, 5′′′), 158.7 (C-4′′′).The detailed experimental procedure for lipase inhibition assay is given as ESI.†
2.4. In silico docking study of the lipase inhibitory activity of isolated compounds (1–8)
The 3D geometry (X-ray crystal structure) of human pancreatic lipase complexed with the ligand; diundecyl phosphatidyl choline (PLC) was obtained from the Research Collaboratory for Structural Bioinformatics Protein Data Bank (RCSB PDB) (PDB code: 1LPA, resolution; 3.04 Å)42,43 and prepared with the protein preparation wizard of Schrödinger Suite44 where water molecules were deleted, protein was typed with OPLS3 force field and minimized. Next, a receptor grid was generated using the co-crystalized ligand as ref. 45.
All ligands (the isolated compounds from T. hirsuta and Z. spina christi) were obtained from NCBI Pubchem.46 Ligands were optimized and energetically minimized through OPLS 3 force field algorithm embedded in the LigPrep module of Schrödinger Suite.47 The ionization states within pH range 7 ± 2 were generated.
In the docking experiment, Glide docking engine within the Schrödinger Suite was utilized.48,49
2.5. In silico prediction of pharmacokinetic ADMET properties
After the step of molecular docking, ADMET and drug-like properties of the isolated compounds were evaluated using Qikprop module embedded in Schrodinger® Suite software package. Qikprop was performed to assess the drug-like properties of all the isolates by calculation of the ADME (absorption, distribution, metabolism, and excretion) properties of the ligands.50 Results of the pharmacokinetics study are demonstrated in Table S1 in the ESI.†
3. Results and discussion
The in vitro spectrophotometric 96-well lipase inhibitory assay described by Slanc4,51 was used to evaluate the two selected Egyptian plants. Reaction mixtures containing 20 μl of the enzyme solution, 50 μl of the tested sample extract solution and 120 μl of Tris-base buffer solution, were incubated at 37 °C for 25 min. Then 10 μl of PNP substrate was added to the reaction mixture, followed by incubation at 37 °C for 30 min. Finally, the absorbance of released p-nitro phenol was measured at 405 nm using a microplate reader. The activity assay was performed in triplicate for each treatment and orlistat is used as a positive control. Percent inhibition values of the alcoholic plant extracts at concentration of (1 mg ml−1) were determined. It was observed that the alcoholic extracts of Z. spina christi and T. hirsuta showed significant lipase inhibitory activity with 93% and 70% inhibition values, respectively.
The results of the in vitro spectrophotometric 96-well lipase inhibitory assay of different fractions of the forementioned plants extracts were demonstrated in (Table 1). It was observed that the butanolic fraction of Z. spina christi showed the most significant anti-lipase activity (80% inhibition) followed by T. hirsuta chloroform fraction (61% inhibition). As a result, these two fractions from both plants were selected for further phytochemical investigation.
Table 1 Lipase inhibition by the fractions obtained from the Thymelaea hirsuta and Ziziphus spina-christi extracts expressed as % inhibition values
Plant name |
Fraction |
Percentage lipase inhibitory activitya (%) |
Concentration at 1 mg ml−1. |
Thymelaea hirsuta roots |
Chloroform |
61 |
Light petrol |
56 |
Ethyl acetate |
45 |
n-Butanol |
−27 |
Ziziphus spina-christi aerial parts |
n-Butanol |
80 |
Light petrol |
60 |
Chloroform |
58 |
Ethyl acetate |
−96 |
Six compounds were isolated and identified from T. hirsute, in addition to, two compounds were isolated from of Z. spina christi which were demonstrated in the schematic diagrams (Fig. S1 and S2,† respectively) present in the ESI.†
All these compounds were tested for their lipase inhibitory potencies following the Slanc method. Lipase inhibitors reduce the conversion of para nitro phenyl palmitate (PNPP) to its coloured metabolite para-nitro phenol, thus, the decrease in the absorbance produced was used as a criterion to assess lipase inhibition. The percentage inhibition was calculated for each inhibitor at the concentration of 0.25 mM.
The results (Table 2) showed that christinin A (8) and daphnoretin (1) were ranked as the most active compounds possessing the highest % inhibition values of 75 and 72%, respectively. On the contrary, daphnodorin B was considered as the least active compound with an % inhibition value of 3.5%. Results also revealed that the tested compounds daphnodorin G, quercetin 3-O-(2,6-di-O-α-rhamnopyranosyl-β-galactopyranoside), neochamaejasmin A, 5,7,4′-trihydroxy-8-methoxycarbonyl flavanol and daphnodorin G-3′′-O-methyl ether exihibited inhibition of PL activity with % inhibition values are 69.5%, 68%, 61.8%, 52% and 39%, respectively. So, these compounds could be considered as potent lipase inhibitors or drug leads for further drug development. It was worthy to mention that this is the first report of these isolated compounds from the two plants: T. hirsuta and Z. spina-christi for lipase inhibition. Furthermore, the extracts are synergistically showing better lipase inhibition and this can be useful for ethno-pharmacological use of these plant species.
Table 2 Lipase inhibition of the isolated compounds (1–8) expressed as % inhibition at concentration of 0.25 mMa
Compound name |
% Inhibition |
IC50 of orlistat (positive control) = 0.0627 mM. |
Christinin A (8) |
75 |
Daphnoretin (1) |
72 |
Daphnodorin G (5) |
69.5 |
Quercetin 3-O-(2,6-di-O-α-rhamnopyranosyl-β-galactopyranoside) (7) |
68 |
Neochamaejasmin A (3) |
61.8 |
5,7,4′-Trihydroxy-8-methoxycarbonyl flavanol (2) |
52 |
Daphnodorin G-3′′-methyl ether (4) |
39 |
Daphnodorin B (6) |
3.5 |
To investigate the mechanism of action and binding of compounds to active site of the lipase enzyme crystalline structure (1LPA), we conducted a molecular docking study using Glide docking engine within the Schrodinger molecular modeling suite35 between the target (PDB code: 1LPA), ligands (orlistat and the isolated compounds from both T. hirsuta and Z. spina-christi), aiming to obtain a deep understanding to the compounds' binding modes inside the enzyme active site and the resulting complexes were scored in terms of GLIDE scores (XPG score). A greater negative numerical value of the docking G score indicates a stronger predicted binding of the ligand–target complex. The docking protocol used flexible ligand sampling and standard precision with no docking constraints.
The molecular docking results shown in Fig. S3–S5† and Table 3, demonstrated that quercetin 3-O-(2,6-di-O-α-rhamnopyranosyl-β-galactopyranoside) (7), christinin A (8) and daphnoretin (1) had the highest binding affinity (−14.66, −12.00 and −11.40 kcal mol−1, respectively) to the 1LPA, followed by daphnodorin B (6), orlistat (positive control), 5,7,4′-trihydroxy-8-methoxycarbonyl flavanol (2) and daphnodorin G-3′′-methyl ether (4) (−9.76, −8.91, −8.71 and −8.23 kcal mol−1, respectively). On the other hand, daphnodorin G (5) and neochamaejasmin A (3) had the lowest binding energy (−6.22 and −6.13 kcal mol−1, respectively) compared to the others.
Table 3 Lipase inhibition of the tested compounds along with orlistat expressed as XP-G scores
Compound name |
Docking XPG score (kcal mol−1) |
Quercetin 3-O-(2,6-di-O-α-rhamnopyranosyl-β-galactopyranoside) (7) |
−14.66 |
Christinin A (8) |
−12.00 |
Daphnoretin (1) |
−11.40 |
Daphnodorin B (6) |
−9.76 |
Orlistat (positive control) |
−8.91 |
5,7,4′-Trihydroxy-8-methoxycarbonyl flavanol (2) |
−8.71 |
Daphnodorin G-3′′-methyl ether (4) |
−8.23 |
Daphnodorin G (5) |
−6.22 |
Neochamaejasmin A (3) |
−6.13 |
From the results of the pharmacokinetics study demonstrated in Table S1,† it was observed that all the compounds' properties lied within the acceptable range of human use. However, some of the pharmacokinetic ADMET properties – especially those depending on solubility – of both the saponin (christinin A) and the flavonoid glycoside (quercetin 3-O-(2,6-di-O-α-rhamnopyranosyl-β-galactopyranoside)) lied outside the acceptable range of human use, therefore, further in vitro pharmacokinetic experiments are recommended to verify the predicted in silico results and to afford better understanding on their ADMET profiles.
The isolated natural compounds were found to possess the best drug-like properties by Lipinski's rule of five shown in Table S1 in the ESI.† The drug molecules which have low, mol. wt. are transported, diffused and absorbed without difficulty in comparison to large molecules.52,53 Furthermore, all the isolates are showing activity against lipase and not on the nervous system.
4. Conclusion
In conclusion, the results of the present study clearly demonstrated that the two compounds; christinin A (8) and daphnoretin (1) have excellent binding interactions with lipase enzyme compared to the standard. These might corporate in presenting the biscoumarin; daphnoretin and the dammarane saponin; christinin A as potent lipase inhibitors which have not been previously reported for this activity.
Author contributions
Rokia M. Abdallah: supervision and conceptualization. Hala M. Hammoda: supervision, original draft preparation, reviewing and editing. Nahla S. El-Gazzar: methodology, writing, visualization, editing and investigation. Reham S. Ibrahim: supervision and software. Shaimaa M. Sallam: supervision and validation.
Conflicts of interest
There are no conflicts to declare.
References
- G. A. Bray, J. Nutr., 2002, 132, 3451S–3455S CrossRef CAS PubMed.
- P. G. Kopelman, Nature, 2000, 404, 635–643 CrossRef CAS PubMed.
- M. E. Lowet, J. Lipid Res., 2002, 43, 2007–2016 CrossRef PubMed.
- R. Birari, S. K. Roy, A. Singh and K. K. Bhutani, Nat. Prod. Commun., 2009, 4, 1089–1092 CrossRef CAS PubMed.
- H. Abdul Rahman, N. Saari, F. Abas, A. Ismail, M. W. Mumtaz and A. Abdul Hamid, Int. J. Food Prop., 2017, 20, 2616–2629 CrossRef CAS.
- R. Chakrabarti, Expert Opin. Ther. Targets, 2009, 13, 195–207 CrossRef CAS PubMed.
- S. Hasani-Ranjbar, Z. Jouyandeh and M. Abdollahi, J. Diabetes Metab. Disord., 2013, 12, 1–10 CrossRef PubMed.
- M. Mukherjee, J. Mol. Catal. B: Enzym., 2003, 22, 369–376 CrossRef CAS.
- C. Roh and U. Jung, Int. J. Mol. Sci., 2012, 13, 1710–1719 CrossRef CAS PubMed.
- W. Chanmee, C. Chaicharoenpong and A. Petsom, Food Nutr. Sci., 2013, 04, 554–558 CAS.
- S. Henness and C. M. Perry, Drugs, 2006, 66, 1625–1656 CrossRef CAS PubMed.
- D. Isler, C. Moeglen, N. Gains and M. K. Meier, Br. J. Nutr., 1995, 73, 851–862 CrossRef CAS PubMed.
- R. B. Birari and K. K. Bhutani, Drug Discovery Today, 2007, 12, 879–889 CrossRef CAS PubMed.
- J. G. Kang and C. Y. Park, Diabetes & Metabolism Journal, 2012, 36, 13–25 Search PubMed.
- D. S. Weigle, J. Clin. Endocrinol. Metab., 2003, 88, 2462–2469 CrossRef CAS PubMed.
- T. D. Filippatos, C. S. Derdemezis, I. F. Gazi, E. S. Nakou, D. P. Mikhailidis and M. S. Elisaf, Drug Saf., 2008, 31, 53–65 CrossRef CAS PubMed.
- R. B. Birari and K. K. Bhutani, Drug Discovery Today, 2007, 12 Search PubMed.
- H. Fei, M. Li, W. Liu, L. Sun, N. Li, L. Cao, Z. Meng, W. Huang, G. Ding, Z. Wang and W. Xiao, Pharm. Biol., 2016, 54, 2845–2850 CrossRef CAS PubMed.
- N. Jaradat, A. N. Zaid and E. Z. Zaghal, Marmara Pharm. J., 2017, 21, 828–863 CrossRef CAS.
- A. I. Martinez-Gonzalez, E. Alvarez-Parrilla, Á. G. Díaz-Sánchez, L. A. de la Rosa, J. A. Núñez-Gastélum, A. A. Vazquez-Flores and G. A. Gonzalez-Aguilar, Food Technol. Biotechnol., 2017, 55, 519–530 CAS.
- P. Worsztynowicz, M. Napierała, W. Białas, W. Grajek and M. Olkowicz, Process Biochem., 2014, 49, 1457–1463 CrossRef CAS.
- N. O. Amari, M. Bouzouina, A. Berkani and B. Lotmani, Asian Pac. J. Trop. Dis., 2014, 4, 104–109 CrossRef CAS.
- T. Mekhelfi, K. Kerbab, G. Guella, L. Zaiter, S. Benayache and F. Benayache, Pharm. Lett., 2014, 6, 152–156 CAS.
- A. M. Rizk and H. Rimpler, Phytochemistry, 1972, 11, 473–475 CrossRef CAS.
- A. Ziyyat, A. Legssyer, H. Mekhfi, A. Dassouli, M. Serhrouchni and W. Benjelloun, J. Ethnopharmacol., 1997, 58, 45–54 CrossRef CAS PubMed.
- M. Bnouham, F. Z. Merhfour, A. Legssyer, H. Mekhfi, S. Maâllem and A. Ziyyat, Pharmazie, 2007, 62, 630–632 CAS.
- S. Abid, A. Lekchiri, H. Mekhfi, A. Ziyyat, A. Legssyer, M. Aziz and M. Bnouham, J. Diabetes, 2014, 6, 351–359 CrossRef PubMed.
- M. Bnouham, W. Benalla, S. Bellahcen, Z. Hakkou, A. Ziyyat, H. Mekhfi, M. Aziz and A. Legssyer, J. Diabetes, 2012, 4, 307–313 CrossRef CAS PubMed.
- V. Täckholm, Ancient Egypt, Landscape, Flora and Agriculture, 1976 Search PubMed.
- C. G. Michel, D. I. Nesseem and M. F. Ismail, J. Ethnopharmacol., 2011, 133, 53–62 CrossRef PubMed.
- R. Avizeh, H. Najafzadeh, M. P. Borujeni and M. Mirzaee, Int. J. Appl. Res. Vet. Med., 2010, 8, 109–113 CAS.
- A. Bozicevic, M. De Mieri, A. Di Benedetto, F. Gafner and M. Hamburger, Phytochemistry, 2017, 138, 134–144 CrossRef CAS PubMed.
- N. M. I. Elnagar and B. M. Modawi, Orient. J. Chem., 2016, 32, 895–901 CrossRef CAS.
- A. Said, A. Huefner, E. A. A. Tabl and G. Fawzy, J. Biol., 2011, 70, 39–43 Search PubMed.
- J. G. Christensen, H. Y. Zou, M. E. Arango, Q. Li, J. H. Lee, S. R. McDonnell, S. Yamazaki, G. R. Alton, B. Mroczkowski and G. Los, Mol. Cancer Ther., 2007, 6, 3314–3322 CrossRef CAS PubMed.
- R. Wang, L. Tong, C. Y. Liu and C. Guo, J. Asian Nat. Prod. Res., 2019, 21, 1215–1220 CrossRef CAS PubMed.
- B. Feng, Y. Pei, H. Hua, T. Wang and Y. Zhang, Pharm. Biol., 2003, 41, 59–61 CrossRef CAS.
- M. Taniguchi and K. Baba, Phytochemistry, 1996, 42, 1447–1453 CrossRef CAS.
- P. Gürbüz and Ş. D. Doğan, Biochem. Syst. Ecol., 2017, 74, 57–59 CrossRef.
- K. Yasukawa, H. Sekine and M. Takido, Phytochemistry, 1989, 28, 2215–2216 CrossRef CAS.
- G. E. D. Hussein Mahran, K. W. Glombitza, Y. W. Mirhom, R. Hartmann and C. G. Michel, Planta Med., 1996, 62, 163–165 CrossRef PubMed.
- H. Van Tilbeurgh, M.-P. Egloff, C. Martinez, N. Rugani, R. Verger and C. Cambillau, Nature, 1993, 362, 814–820 CrossRef CAS PubMed.
- H. M. Berman, J. Westbrook, Z. Feng, G. Gilliland, T. N. Bhat, H. Weissig, I. N. Shindyalov and P. E. Bourne, Nucleic Acids Res., 2000, 28, 235–242 CrossRef CAS PubMed.
- G. Madhavi Sastry, M. Adzhigirey, T. Day, R. Annabhimoju and W. Sherman, J. Comput.-Aided Mol. Des., 2013, 27, 221–234 CrossRef CAS PubMed.
- T. A. Halgren, R. B. Murphy, R. A. Friesner, H. S. Beard, L. L. Frye, W. T. Pollard and J. L. Banks, J. Med. Chem., 2004, 47, 1750–1759 CrossRef CAS PubMed.
- Y. Wang, J. Xiao, T. O. Suzek, J. Zhang, J. Wang and S. H. Bryant, Nucleic Acids Res., 2009, 37, W623–W633 CrossRef CAS PubMed.
- D. Shivakumar, J. Williams, Y. Wu, W. Damm, J. Shelley and W. Sherman, J. Chem. Theory Comput., 2010, 6, 1509–1519 CrossRef CAS PubMed.
- S. L. Dixon, A. M. Smondyrev, E. H. Knoll, S. N. Rao, D. E. Shaw and R. A. Friesner, J. Comput.-Aided Mol. Des., 2006, 20, 647–671 CrossRef CAS PubMed.
- R. A. Friesner, R. B. Murphy, M. P. Repasky, L. L. Frye, J. R. Greenwood, T. A. Halgren, P. C. Sanschagrin and D. T. Mainz, J. Med. Chem., 2006, 49, 6177–6196 CrossRef CAS PubMed.
- G. K. Veeramachaneni, K. K. Raj, L. M. Chalasani, S. K. Annamraju, B. Js and V. Talluri, Bioinformation, 2015, 11, 535–542 CrossRef PubMed.
- P. Slanc, B. Doljak, S. Kreft, M. Lunder, D. Janeš and B. Štrukelj, Phytother. Res., 2009, 23, 874–877 CrossRef CAS PubMed.
- C. A. Lipinski, Drug Discovery Today: Technol., 2004, 1, 337–341 CrossRef CAS PubMed.
- D. F. Veber, S. R. Johnson, H. Y. Cheng, B. R. Smith, K. W. Ward and K. D. Kopple, J. Med. Chem., 2002, 45, 2615–2623 CrossRef CAS PubMed.
|
This journal is © The Royal Society of Chemistry 2023 |
Click here to see how this site uses Cookies. View our privacy policy here.