DOI:
10.1039/D3RA02515B
(Paper)
RSC Adv., 2023,
13, 17812-17816
POCl3 mediated one-pot deoxygenative aromatization and electrophilic chlorination of dihydroxy-2-methyl-4-oxo-indeno[1,2-b]pyrroles†
Received
15th April 2023
, Accepted 7th June 2023
First published on 13th June 2023
Abstract
A class of indenopyrroles is presented by the treatment of known dihydroxy-2-methyl-4-oxoindeno[1,2-b]pyrroles with phosphorus oxychloride (POCl3). The elimination of vicinal hydroxyl groups at the 3a and 8b positions, formation of a π bond, and electrophilic chlorination of the methyl group attached to C2 resulted in the fused aromatic pyrrole structures. Benzylic substitution of various nucleophiles such as H2O, EtOH, and NaN3 with a chlorine atom gave diverse 4-oxoindeno[1,2-b]pyrrole derivatives in 58 to 93% yields. The reaction was investigated in different aprotic solvents, and the highest reaction yield was obtained in DMF. The structures of the products were confirmed by spectroscopic methods, elemental analysis, and X-ray crystallography.
Introduction
Deoxygenation–chlorination of oxygen-containing organic compounds is essential in synthesizing a variety of organic and biological materials.1 Phosphorus oxychloride (POCl3), owing to its outstanding position among the phosphorus-based reagents, plays a prominent role in these transformations. Simultaneous chlorination during deoxygenation using POCl3 could occur by electrophilic or nucleophilic substitution. N-oxide and S-oxide derivatives of nitrogen or sulfur-bearing heterocycles are typical examples of materials used in these reactions.2 Furthermore, the great attention paid to POCl3 stems from its diverse applications in chlorination,3 dehydration,4 Bischler–Napieralski cyclization,5 and Vilsmeier–Haack formylation.6 Dihydroxy-4-oxo-indeno[1,2-b]pyrroles possessing two vicinal hydroxyl groups have been deoxygenated by reagents such as triphenylphosphine (route A in Scheme 1),7 thionyl chloride,8 and tetraalkylthionylamides ((NR2)2SO) (route B in Scheme 1).8,9
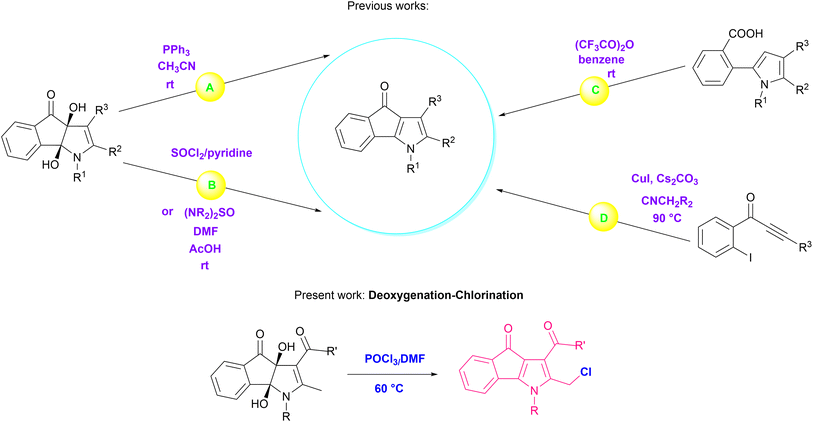 |
| Scheme 1 Known synthesis methods of 4-oxo-indeno[1,2-b]pyrroles and our novel synthesis strategy. | |
The aromatic 4-oxo-indeno[1,2-b]pyrroles have been produced due to the elimination of vicinal hydroxyl groups and the formation of π bond during these reactions. It is noteworthy that other methods have also synthesized 4-oxo-indeno[1,2-b]pyrroles as potent human protein kinase CK2 inhibitors;9 for example, intramolecular cyclization of 5-(o-carboxyphenyl)pyrroles via trifluoroacetic anhydride (route C in Scheme 1),10 and copper-catalyzed coupling reaction of 1-(2-iodoaryl)-2-yn-1-ones with isocyanides (route D in Scheme 1).11
POCl3 can form phosphate ester through the reaction with alcohols, phenols, and epoxides based on the inherent affinity of phosphorus for oxygen.12 Meanwhile, vicinal diols can construct cyclic phosphate ester, which is stable as long as there is no stimulating factor to open the ring.13
Regard to our recent interest in the study of indenopyrrole structures14 due to their biological activities,15 herein, we report an efficient POCl3-mediated deoxygenation–chlorination of dihydroxy-2-methyl-4-oxo-indeno[1,2-b]pyrroles via a cyclic chlorophosphate ester intermediate to achieve novel derivatives of 2-(chloromethyl)-4-oxo-indeno[1,2-b]pyrrole. Nucleophilic substitution of several nucleophiles, such as H2O, EtOH, and NaN3, with the chlorine atom, resulted in the related products.
Results and discussion
At the outset, we aimed to formylate the ortho position of the hydroxyl group of the phenyl ring in compound 1f with Vilsmeier–Haack reagent (POCl3/DMF) at 60 °C. Surprisingly, product 3e was isolated as a major product after hydrolysis instead of formylated product f* (Scheme 2). It seems that 3e with a hydroxymethyl group (OH-product) is a result of the hydrolysis of a chloromethyl derivative (Cl-product) which is primarily formed under the action of POCl3 reagent.
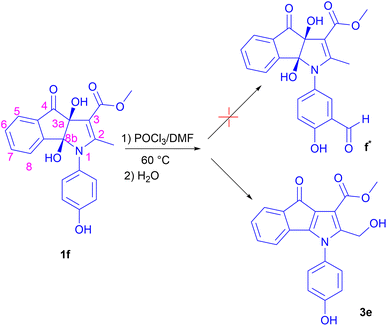 |
| Scheme 2 Formation of product 3e under Vilsmeier–Haack reaction instead of product f*. | |
Based on the above results, we proposed a mechanism that has been shown as Scheme 3. First, nucleophilic attack of the two vicinal hydroxyl groups of compound 1 on the phosphorus atom of POCl3 and loss of two moles of HCl leads to the formation of intermediate Im1 as a cyclic chlorophosphate ester. Enol tautomer of Im1 shows the nucleophilicity of the methyl group attached to C2 that can take electrophilic chlorine linked to the chlorophosphate ester ring. Chlorination of the benzylic methyl group is accompanied by opening the phosphate ester ring, deoxygenation at 3a and 8b positions and the formation of π bond in these positions. Cl-product 2 and metaphosphoric acid (HPO3) are finally produced (Scheme 3). At last, adding water to the reaction mixture hydrolyses 2, resulting in the OH-product 3. Whereas, applying alkaline hydrolysis to accelerate the nucleophilic substitution and neutralize the acidic medium of the reaction caused the decomposition of the product 3.
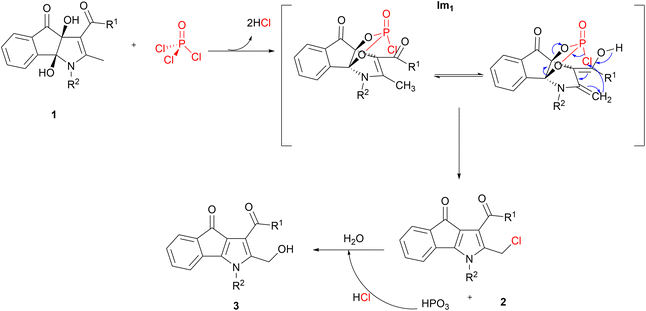 |
| Scheme 3 A plausible reaction mechanism. | |
To explore the generality of the reaction on substrate scope, a variety of known dihydroxy-2-methyl-4-oxo-indeno[1,2-b]pyrroles (1a–i,14 Table 1) were employed in these reactions to the synthesis of diverse 4-oxo-indeno[1,2-b]pyrrole derivatives. The related products were obtained with moderate to excellent yields (entries 1–15). We separated the Cl-products in some of the derivatives, which were a precipitate in the reaction mixture (2a–c, entries 1–3). Cl-product 2d was also separated as a major product after the addition of water at room temperature and fast filtration of the formed precipitate (entry 4). Whereas, adding water and stirring the mixture at 60 °C afforded the OH-product 3d (entry 9). Treatment of 1e with POCl3 in DMF led to the Cl-product 2e, which was isolated as the only reaction product after adding water and extraction with chloroform. This indicates that compound 2e does not tend to hydrolyze (entry 5). To confirm the formation of the primary Cl-products 2, their nucleophilic substitutions with H2O, EtOH, and NaN3 were also examined, and hydroxymethyl (3a, 3b and 3d–h), ethoxymethyl (3c and 3i), and azidomethyl (3j) derivatives were obtained respectively (entries 6–15). Furthermore, the positivity of the NaI in acetone test16 on all the isolated chlorinated derivatives (2a–e) proved the formation of the primary Cl-products. Among the substrates 1, those containing electron-donating groups attached to the phenyl ring of the N1 position represented higher yield and rate reaction compared to substrates bearing the electron-withdrawing groups, especially the nitro group. It seems that electronic resonance of the phenyl ring containing the electron-donating groups with electrons of the pyrrole ring towards the acetyl or ester group attached to C3 causes more acidity of the methyl hydrogens linked to C2, which itself is a driving factor for the fast opening of the phosphate ester ring. The reaction time presented in Table 1 is related to the disappearance of substrate 1. Moreover, to confirm the assigned structure, a single crystal of 3f was determined using X-ray crystallography (Fig. 1). Orange crystals of 3f were formed through slow diffusion of n-hexane over a saturated chloroform solution. The compound 3f crystallizes in the monoclinic crystal system and P21/c space group. The X-ray crystallographic study unambiguously elucidated the coplanar indanone–pyrrole–phenyl network. The hydroxyl group bonded to methylene attached to C2 was also verified.
Table 1 Substrate scope of dihydroxy-2-methyl-4-oxo-indeno[1,2-b]pyrrolesa

|
Entry |
R′ |
R |
Substrate |
Nucleophile |
Time (h) |
Product |
Yieldb % |
Reactions were carried out using 1.0 mmol of substrates and 1.3 mmol of POCl3 in DMF (2 mL) at 60 °C (2a–e and 3a–j). Isolated yield. |
1 |
OMe |
C6H5 |
1a |
— |
3.5 |
2a |
74 |
2 |
OEt |
4-ClC6H4 |
1b |
— |
3 |
2b |
67 |
3 |
OMe |
4-O2NC6H4 |
1c |
— |
7 |
2c |
53 |
4 |
OtBu |
n-Butyl |
1d |
— |
0.25 |
2d |
68 |
5 |
Me |
3-(N-Morpholino)propyl |
1e |
— |
1.5 |
2e |
65 |
6 |
OMe |
C6H5 |
1a |
H2O |
3.5 |
3a |
81 |
7 |
OEt |
4-ClC6H4 |
1b |
H2O |
3 |
3b |
81 |
8 |
OMe |
4-O2NC6H4 |
1c |
EtOH |
24 |
3c |
58 |
9 |
OtBu |
n-Butyl |
1d |
H2O |
0.25 |
3d |
76 |
10 |
OMe |
4-OHC6H4 |
1f |
H2O |
3 |
3e |
93 |
11 |
OtBu |
4-MeOC6H4 |
1g |
H2O |
3 |
3f |
87 |
12 |
OtBu |
4-MeC6H4 |
1h |
H2O |
3 |
3g |
83 |
13 |
OMe |
2-OMeC6H4 |
1i |
H2O |
1.5 |
3h |
80 |
14 |
OEt |
4-ClC6H4 |
1b |
EtOH |
24 |
3i |
75 |
15 |
OEt |
4-ClC6H4 |
1b |
NaN3 |
3 |
3j |
70 |
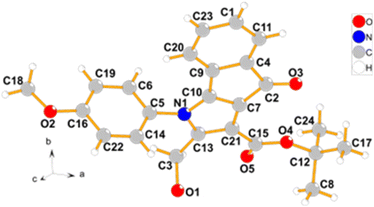 |
| Fig. 1 Molecule of 3f determined by X-ray crystallographic analysis. Labels of hydrogen atoms were omitted for clarity. | |
Finally, to investigate the effect of solvent, substrate 1b was treated with POCl3 in different dry aprotic solvents such as THF, DMF, CHCl3, and CH3CN (Table 2). TLC from the reaction mixtures showed the formation of both Cl-product 2b and OH-product 3b. Although there was no water in the reaction medium, observation of the OH-product 3b could be due to water formation during the reaction. According to the mechanism presented in Scheme 2, the generated H2O in the reaction probably originates from the reaction of HPO3 and HCl. Eventually, the highest yield and reaction rate was acquired in DMF.
Table 2 Investigation of the solvent effect
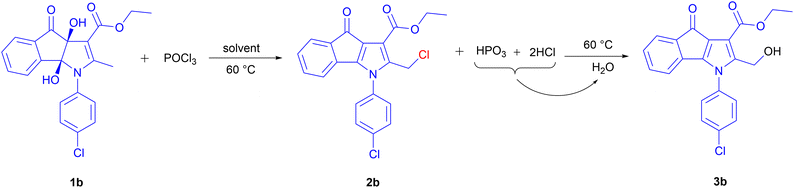
|
Entry |
Solvent (dry) |
Time (h) |
Yield% (3b) |
Entry |
Solvent (dry) |
Time (h) |
Yield% (3b) |
1 |
CHCl3 |
5 |
10 |
3 |
DMF |
3 |
81 |
2 |
CH3CN |
5 |
15 |
4 |
THF |
3 |
70 |
Conclusions
In summary, we have disclosed an exciting deoxygenation–chlorination transformation of known dihydroxy-2-methyl-4-oxo-indeno[1,2-b]pyrroles via POCl3 reagent in DMF. This reaction caused electrophilic chlorination of the benzylic methyl group attached to C2 accompanied by dehydroxyalation at 3a and 8b positions and the formation of π bond. The cyclic chlorophosphate ester served as a key intermediate in this reaction to achieve 2-(chloromethyl)-4-oxo-indeno[1,2-b]pyrroles. Moreover, several nucleophiles such as H2O, EtOH, and NaN3 participated in benzylic substitution with a chlorine atom, leading to a variety of 4-oxoindeno[1,2-b]pyrroles in moderate to excellent yields. Furthermore, the evaluation of the solvent effect on the yield and reaction rate revealed that the highest reactivity and efficiency were acquired in DMF. We synthesized 15 novel compounds (2a–e and 3a–j) in this work.
Conflicts of interest
There are no conflicts to declare.
Acknowledgements
Authors gratefully acknowledge research grant support from the University of Tabriz. Crystallographic experiments were performed in MGML (https://mgml.eu), supported within the Czech Research Infrastructures program (project no. LM2018096).
Notes and references
-
(a) R. S. Malykhina and A. Y. Sukhorukov, Adv. Synth. Catal., 2021, 363, 3170–3188 CrossRef;
(b) J. V. Jun, E. J. Petersson and D. M. Chenoweth, J. Am. Chem. Soc., 2018, 140, 9486–9493 CrossRef CAS PubMed;
(c) M. Bollenbach, C. Lugnier, M. Kremer, E. Salvat, S. Megat, F. Bihel, J. J. Bourguignon, M. Barrot and M. Schmitt, Eur. J. Med. Chem., 2019, 177, 269–290 CrossRef CAS PubMed;
(d) R. Alvarez, L. Aramburu, C. Gajate, A. V. Blazquez, F. Mollinedo, M. Medarde and R. Pelaez, Bioorg. Chem., 2020, 98, 103755 CrossRef CAS PubMed;
(e) S. Hu, Z. Zhao and H. Yan, Bioorg. Chem., 2019, 92, 103232 CrossRef PubMed.
-
(a) J. H. Come, P. N. Collier, J. A. Henderson, A. C. Pierce, R. J. Davies, A. Le Tiran, H. O'Dowd, U. K. Bandarage, J. Cao, D. Deininger, R. Grey, E. B. Krueger, D. B. Lowe, J. Liang, Y. Liao, D. Messersmith, S. Nanthakumar, E. Sizensky, J. Wang, J. Xu, E. Y. Chin, V. Damagnez, J. D. Doran, W. Dworakowski, J. P. Griffith, M. D. Jacobs, S. Khare-Pandit, S. Mahajan, C. S. Moody and A. M. Aronov, J. Med. Chem., 2018, 61, 5245–5256 CrossRef CAS PubMed;
(b) M. I. El-Gamal and C.-H. Oh, J. Enzyme Inhib. Med. Chem., 2018, 33, 1160–1166 CrossRef CAS PubMed;
(c) G. T. Lee, X. Jiang, T. R. Vedananda, K. Prasad and O. Repic, Adv. Synth. Catal., 2004, 346, 1461–1464 CrossRef CAS;
(d) Sh. Youssif, Arkivoc, 2001, 2001, 242–268 Search PubMed;
(e) C. W. Bird, J. Chem. Soc. C, 1968, 1230–1232 RSC.
-
(a) D. W. Sun, X. Jiang, M. Jiang, Y. Lin and J. T. Liu, Eur. J. Org. Chem., 2018, 2018, 2078–2081 CrossRef CAS;
(b) M. A. A. Radwan, M. A. Alshubramy, M. Abdel-Motaal, B. A. Hemdan and D. S. El-Kady, Bioorg. Chem., 2020, 96, 103516 CrossRef CAS;
(c) A. Abdelhameed, X. Liao, C. A. McElroy, A. C. Joice, L. Rakotondraibe, J. Li, C. Slebodnick, P. Guo, W. D. Wilson and K. A. Werbovetz, Bioorg. Med. Chem. Lett., 2020, 30, 126725 CrossRef CAS PubMed.
-
(a) L. Chen, H. Duan, X. Zhang, Q. Zhang, H. Huang, J. Zhao, B. Chen, C. Hua and X. Gou, J. Heterocycl. Chem., 2018, 55, 1978–1985 CrossRef CAS;
(b) L. M. Heckman, Z. He and T. F. Jamison, Org. Lett., 2018, 20, 3263–3267 CrossRef CAS PubMed;
(c) A. Nocentini, F. Carta, M. Tanc, S. Selleri, C. T. Supuran, C. Bazzicalupi and P. Gratter, Chem.–Eur. J., 2018, 24, 7840–7844 CrossRef CAS;
(d) W. Zeinyeh, Y. J. Esvan, B. Josselin, B. Baratte, S. Bach, L. Nauton, V. Théry, S. Ruchaud, F. Anizon, F. Giraud and P. Moreau, Bioorg. Med. Chem., 2019, 27, 2083–2089 CrossRef CAS PubMed.
- M. Li, Y. Yuan and Y. Chen, Chin. J. Chem., 2021, 39, 3101–3115 CrossRef CAS.
- T. Mohammed, A. Abdullatif Khan, S. M. S. Iqubal and B. A. Alyami, Chem. Pap., 2022, 76, 2615–2621 CrossRef CAS.
- H. Karami, Z. Hossaini, M. Sabbaghan and F. Rostami-Charati, Chem. Heterocycl. Compd., 2018, 54, 1040–1044 CrossRef CAS.
- H.-J. Hemmerling and G. Reiss, Synthesis, 2009, 2009, 985–999 CrossRef.
-
(a) G. J. Gozzi, Z. Bouaziz, E. Winter, N. Daflon-Yunes, M. Honorat, N. Giragosian, Ch. Marminon, G. Valdameri, A. Bollacke, J. Guillon, N. Pinaud, M. Marchivie, S. M. Cadena, J. Jose, M. L. Borgne and A. D. Pietro, Drug Des., Dev. Ther., 2015, 9, 3481–3495 CAS;
(b) G. J. Gozzi, Z. Bouaziz, E. Winter, N. Daflon-Yunes, D. Aichele, A. Nacereddine, C. Marminon, G. Valdameri, W. Zeinyeh, A. Bollacke, J. Guillon, A. Lacoudre, N. Pinaud, S. M. Cadena, J. Jose, M. L. Borgne and A. D. Pietro, J. Med. Chem., 2015, 58, 265–277 CrossRef PubMed;
(c) C. Hundsdörfer, H. J. Hemmerling, C. Götz, F. Totzke, P. Bednarski, M. L. Borgne and J. Jose, Bioorg. Med. Chem., 2012, 20, 2282–2289 CrossRef PubMed;
(d) F. Alchab, E. Sibille, L. Ettouati, E. Bana, Z. Bouaziz, A. Mularoni, E. Monniot, D. Bagrel, J. Jose, M. L. Borgne and P. Chaimbault, J. Enzyme Inhib. Med. Chem., 2016, 31, 25–32 CrossRef CAS PubMed;
(e) F. Alchab, L. Ettouati, Z. Bouazizi, A. Bollacke, J. G. Delcros, C. G. W. Gertzen, H. Gohlke, N. Pinaud, M. Marchivie, J. Guillon, B. Fenet, J. Jose and M. L. Borgne, Pharmaceuticals, 2015, 8, 279–302 CrossRef CAS PubMed.
- A. N. Grinev, I. N. Nesterova and M. V. Mezentseva, Chem. Heterocycl. Compd., 1987, 23, 1067–1069 CrossRef.
- Q. Cai, F. Zhou, T. Xu, L. Fu and K. Ding, Org. Lett., 2011, 13, 340–343 CrossRef CAS PubMed.
- D. Meziane, A. Elias and E. Guénin, J. Chem., 2016, 2016, 1–7 CrossRef.
-
(a) F. Wang, S. Pan, P. Zhang, H. Fan, Y. Chen and J. Yan, Fibers Polym., 2018, 19, 1057–1063 CrossRef CAS;
(b) P. M. Cullis, M. J. P. Harger and M. Lee, Angew. Chem., 2000, 112, 4415–4417 CrossRef.
- F. Nasuhipur, Z. Ghasemi, A. Shahrisa and N. Arsalani, J. Heterocycl. Chem., 2022, 60, 106–115 CrossRef.
-
(a) N. Chen, X. Meng, F. Zhu, J. Cheng, X. Shao and Zh. Li, J. Agric. Food Chem., 2015, 63, 1360–1369 CrossRef CAS PubMed;
(b) S. ElKalyoubi and E. Fayed, J. Chem. Res., 2016, 40, 771–777 CrossRef;
(c) K. Mal, B. Naskar, A. Mondal, S. Goswami, C. Prodhan, K. Chaudhuri and C. Mukhopadhyay, Org. Biomol. Chem., 2018, 16, 5920–5931 RSC.
- R. L. Shriner, C. K. Hermann, T. C. Morrill, D. Y. Curtin and R. C. Fuson, The Systematic Identification of Organic Compounds, John Wiley & Sons, 8th edn, 2004, ch. 9 Search PubMed.
|
This journal is © The Royal Society of Chemistry 2023 |
Click here to see how this site uses Cookies. View our privacy policy here.