DOI:
10.1039/D3RA02249H
(Paper)
RSC Adv., 2023,
13, 21163-21170
The application of biosurfactant-producing bacteria immobilized in PVA/SA/bentonite bio-composite for hydrocarbon-contaminated soil bioremediation
Received
5th April 2023
, Accepted 16th June 2023
First published on 13th July 2023
Abstract
Oil spills that contaminate the environment can harm the surrounding ecosystem. The oil contains petroleum hydrocarbon which is toxic to the environment hence it needs to be removed. The use of bacteria as remediation media was modified by immobilizing into a matrix hence the bacteria can survive in harsh conditions. In this research, the ability of biosurfactant-producing bacteria (Pseudomonas aeruginosa, Bacillus subtilis, and Ralstonia pickettii) immobilized in the PVA/SA/bentonite matrix was tested in remediation on oil-contaminated soil. The immobilized beads filled with bacteria were added to the original soil sample, as well as washed soil. The beads were characterized by using FTIR and SEM. Based on FTIR analysis, the PVA/SA/bentonite@bacteria beads had similar functional groups compared to each other. SEM analysis showed that the beads had non-smooth structure, while the bacteria were spread outside and agglomerated. Furthermore, GC-MS analysis results showed that immobilized B. subtilis and R. pickettii completely degraded tetratriacontane and heneicosane, respectively. Meanwhile, after soil washing pre-treatment, immobilized bacteria could completely degrade octadecane (P. aeruginosa and R. pickettii) and tetratriacontane (P. aeruginosa and B. subtilis). Based on those results, immobilized bacteria could degrade oil compounds. The degradation result was influenced by the enzymes produced, the ability of the bacteria, the suitability of the test media, and the matrix used. Therefore, this study can be a reference for further soil remediation using eco-friendly methods.
Introduction
Crude oil is a non-renewable and irreplaceable source of energy,1 and oil is processed as a fuel and other products.2 Total Petroleum Hydrocarbon (TPH) is a term used to represent crude oil which consists of a mixture of thousands of compounds.3 Most of the scientific literature on crude oil biodegradation refers to the TPH fraction, which includes volatile and extractable crude oil hydrocarbons, such as organic gasoline (
C6–C10), organic diesel (
C11–C28), and the organic oil range (C29–C35). All of these compounds are described as toxic to the environment.4 Increasing demand for crude oil products in various industries and daily life can lead to increased oil costs, increased oil exploitation, and increased oil pollution.5 Apart from having a positive social and economic impact on society, the use of oil can also have a negative impact on the environment, especially in the case of oil spills which can pollute the environment. The waste produced by oil refineries can be in the form of liquid and solid waste. Soil contaminated with high concentrations of crude oil hydrocarbons can be a source of groundwater contamination. Most of this waste is mutagenic for humans and the surrounding environment.6 One method of eliminating this waste is using bioremediation. Bioremediation is a process mainly by using microorganisms and plant to remove pollutant in the soil and other environment.7 This method is effective and low-cost as well as can be used as a solution to removing contaminants in the environment.8
The use of bacteria culture as a bioremediation agent is more widely used because of its fast growth and ability to adapt to toxic conditions.9 Furthermore, bacteria are the most active agents in crude oil degradation because they act as the main decomposers of oil spills in the environment.10 Some bacteria were reported to be able to degrade hydrocarbons because they are capable of producing biosurfactants such as Pseudomonas aeruginosa,11 Bacillus subtilis,12 and Ralstonia pickettii.13 The synergistic effect of surfactants can occur through two mechanisms, namely changes in the bioavailability of the substrate for microorganisms and changes in the hydrophobicity of the cell surface which allows the substrate to associate more easily with bacteria cells.14
In previous research, B. subtilis could degrade crude oil in artificial seawater medium, in which 91% of a compound in crude oil was degraded.15 On the other hand, bioremediation using B. subtilis-free cells can degrade crude oil by 65% under optimal conditions.1 Bharali et al.11 reported oil contaminated soil by P. aeruginosa recovered 73.5–63.4% of residual oil from the sludge through its biosurfactant ability. In addition, the bacterium R. pickettii is also capable of degrading crude oil in the high-salinity water, which degraded about 99%.13 The biosurfactant-producing bacteria have high ability to degrade crude oil, however, it requires a long duration for the incubation process.14
Recent research showed that immobilized bacteria in the matrix could survive longer under extreme conditions.16 One technique widely used for immobilizing bacteria is entrapment where bacteria, as living cells, are encased in a polymeric matrix with a pore capacity that allows diffusion of the substrate to the bacteria. Materials that have been used successfully for cell entrapment include agar, agarose, carrageenan, collagen, alginate, chitosan, polyacrylamide, polyurethane, and cellulose.17 Nhi-Cong et al.18 used immobilization technique on wastewater polluted by oil. The result showed, within immobilized bacteria using coconut fiber matrix, it could degrade near 100% after 14 days. On the other hand, sodium alginate (SA) is also being a generally biopolymer for building an organic immobilization matrix that is non-toxic to microbes.19 Furthermore, combining SA within polyvinyl alcohol (PVA) could increase the mechanical strength of the beads matrix.20
Moreover, the addition of bentonite clay to the matrix also aims to increase the adsorption capacity of the beads.21 A previous study reported bentonite that designed into alginate matrix could enhance adsorption capacity on removal wastewater pollutant.22 Furthermore, Baigorria et al.23 used bentonite composite PVA/SA on arsenic removal.2 Hence, in this study, PVA/SA/bentonite@bacteria bio-composite beads were used to degrade hydrocarbons. This study expected that this system can degrade hydrocarbons more efficiently. Based on this hypothesis, it is necessary to conduct research on the synthesis of PVA/SA/bentonite@bacteria bio-composite and test its ability to degrade hydrocarbons.
Experimental
Materials
Bacteria P. aeruginosa NBRC 3080, B. subtilis NBRC 3009, and R. pickettii NBRC 102503 were purchased from Biological Resource Center (NBRC), Japan. Furthermore, demineralized water and 70% alcohol were obtained from SAP Chemicals, Indonesia. Nutrient Agar (NA), Luria Bertani Broth (LB), and Tween 80 were obtained from Merck, Germany. Moreover, contaminated soil sample was obtained from oil refinery wells in Bojonegoro, Indonesia.
Soil pre-treatment
Washing soil was conducted to remove impurities and decrease TPH in original soil. Approximately 100 g L−1 of contaminated soil was mixed within 0.2% Tween-80 for two times repeated cycles. The mixture was centrifuged for 20 min under 3000 rpm rotary speed, then took off the supernatant.24 The sediment of soil pre-treatment was used for the next bioremediation procedure.
Bacterial culture
Synthetic stock culture of biosurfactant-producing bacteria (BPB), namely P. aeruginosa (2.11 × 108 CFU per mL), B. subtilis (2.47 × 108 CFU per mL), and R. pickettii (2.46 × 108 CFU per mL) were inoculated on NA media and incubated at 37 °C for 24 hours.25 Subsequently, the regenerated bacteria colony was inoculated into a 500 mL Erlenmeyer with 200 mL of sterile LB media.25 The culture was incubated on an incubator shaker at 180 rpm for 24 hours for P. aeruginosa and B. subtilis25 as well as 44 hours for R. pickettii.26 Then, the bacteria were carried out for 50 mL from each species and centrifuged to obtain their biomasses. After that, they were added by 5 mL demineralized water individually.
Bacteria immobilization process
Immobilization gel was prepared by mixing 2 g PVA, 1 g SA, and 0.5 g bentonite into 45 mL demineralized water.25 The mixture was heated for an hour at 150 °C and stirred using magnetic stirrer bar.27 Then, it sterilized by autoclaving (121 °C, 15 min) to kill out other contaminants. After the hydrogel was cooling down, then 5 mL bacterium that prepared before was added. This study was not examined for consortium bacteria. Hence, one hydrogel solution was mixed within one species of bacteria.
The variable used in this process was the presence of live bacteria treatment. Beads were formed by dripping the hydrogel solution into 4% (w/v) CaCl2 solution (cross-linking agent) using a sterile disposable syringe.28 The formed beads were immersed in 4% (w/v) CaCl2 solution for 24 hours (cross-linking time). Subsequently, the beads were washed with sterile demineralized water and ready to be used.
Hydrocarbon degradation by immobilized BPB on PVA/SA/bentonite matrix
Hydrocarbon degradation by PVA/SA/bentonite matrix immobilized bacteria was carried out into 100 mL Erlenmeyer containing 5 g of soil and 10 g immobilized bacteria beads. The solution was incubated for 14 days at 37 °C under static conditions.14,15
Calculation of total petroleum hydrocarbon (TPH) and product metabolite identification
The culture was then separated from the soil media by dissolving it in 10 mL organic solvent n-hexane using a centrifuge at 2000 rpm for 10 minutes.29 The resulting oil was dried using an oven at 70 °C for 10 min to determine the mass of the bioremediated oil and obtain the TPH value by gravimetric method.30 Degradation results from oil bioremediation process were identified by analyzing oil samples. Analysis was carried out using a hydrocarbon profile test with a Gas Chromatography-Mass Spectrometry (GC-MS) instrument. The oil samples obtained were diluted 20× using n-hexane before being analyzed. GCMS was performed using an Agilent Technologies 7890 GC System linked to an Agilent Technologies 5975C VL MSD Detector with a 30 m × 50 μm × 0.25 μm Agilent 19091S-433 column. The oven temperature was programmed to start from 80 °C and held for 2 min, and then the temperature was increased to 280 °C at 5 °C min−1 and held for 15 min greater.13
Statistical analysis
The results were calculated by obtaining mean result of triplicate sample per each various bacterium. Analysis of Variance (ANOVA) was used to determine the significant difference between TPB value before and after washing soil pre-treatment bioremediation result, by each BPB. Differences between mean values at a confidence level of 5% (P < 0.05) were considered to be statistically significant.31
Results and discussion
Crude oil bioremediation and degradation product identification
Immobilized bacteria in the PVA/SA/bentonite matrix were tested for their ability to degrade crude oil contaminants in soil samples, during a 14-day incubation period. GCMS chromatograms of the degradation test results of non-pre-treatment soil are shown in Fig. 1. The chromatogram shows an increase in intensity at various points for each immobilized bacterium. The increase/decrease in intensity affects the ability of the bacteria to degrade crude oil compounds. The greater increases in intensity, the greater of percentage recovery produced or the lower of percentage degradation produced by the bacteria. Conversely, a decrease in intensity indicates a higher degradation ability of the immobilized bacteria. Fig. 2 shows the %recovery of immobilized bacteria in degrading compounds in original soil samples, where P. aeruginosa had the highest %recovery, exceeding 650%. This was due to the accumulation of compounds where the bacteria had not been able to degrade them. The accumulation of these compounds could mean the production of simple compounds resulting from the degradation of higher molecular weight compounds. This was because microorganisms are able to involve aliphatic or aromatic fractions of oil by degrading complex compounds into simpler ones.32
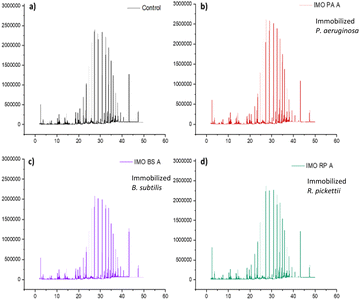 |
| Fig. 1 Chromatograms of crude oil recovery (a) control, and immobilization by bacteria (b) P. aeruginosa, (c) B. subtilis, and (d) R. pickettii after treatment 14 days. | |
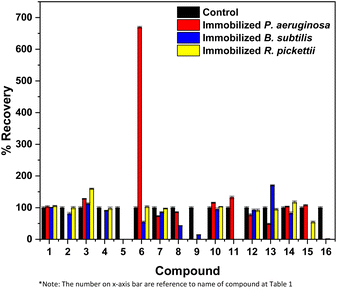 |
| Fig. 2 Crude oil recovery results by immobilized bacteria of non-pre-treatment soil bioremediation. Data showed the mean of triplicate analysis ± standard deviation (SD). | |
Generally, biosurfactant produced by bacteria enhances hydrophobicity of cell surface, causing hydrophobic substrate can interact within bacteria cell. After that, the degradation process will be taken further action. This degradation came out because of the availability of catechol 2,3-dioxygenase or ortho-cleavage dioxygenase enzymes. These enzymes have a role on making oxygen incorporation within hydrocarbon substrate and break hydrocarbon chain.14 While on degradation of aliphatic chain, terminal monooxygenase enzyme had a role on converting substrate become fatty acid and acetyl CoA.33 Among of these bacteria P. aeruginosa, B. subtilis, and R. pickettii had been reported could produce biosurfactant and secreted dioxygenase enzymes.34–36 Pseudomonas sp. had been reported as oil degraded bacteria. Study by Rodríguez-Uribe et al. (2021) reported that Pseudomonas sp. was a bacterium that had been isolated from oil contaminated soil.37 However, Bharali et al.11 also used four strains of Pseudomonas aeruginosa in oil degradation by role of rhamnolipid biosurfactant producing. Furthermore, B. subtilis also had been reported isolate from oil product.38 Zhao et al.39 showed B. subtilis produced C13-, C14- and C15-surfactin congeners and Tao et al.40 examined B. subtilis degraded crude oil within degradation ratio approximately 85%. Meanwhile study about R. pickettii was still minority. However this bacteria reported could examine crude oil under high salinity medium.13
Table 1 shows the %recovery and %degradation produced by each immobilized bacteria in the PVA/SA/bentonite matrix on native soil test media. Immobilized P. aeruginosa produced the highest %recovery of dotriacontane compound. These bacteria could completely degrade 1-heptacosanol, cyclohexane, nonadecyl-, ditetradecyl ether, hexadecane, 2,6,10,14-tetramethyl-, and tetratriacontyl heptafluorobutyrate compounds up to 100%. Meanwhile, immobilized B. subtilis, compounds that completely degraded were ditetradecyl ether and tetratriacontane. Furthermore, immobilized R. pickettii, compounds that completely degraded include tetratriacontyl heptafluorobutyrate, octacosanol, ditetradecyl ether, hexadecane, 2,6,10,14-tetramethyl-, and heneicosane. The difference in compounds degraded by bacteria was influenced by the enzymes produced by each bacterium.15
Table 1 Recovery results for metabolite compound of non-pre-treatment soil bioremediation
No. |
Name of compound |
P. aeruginosa |
B. subtilis |
R. pickettii |
Recovery (%) |
Degradation (%) |
Recovery (%) |
Degradation (%) |
Recovery (%) |
Degradation (%) |
1 |
1,4-Epoxynaphthalene-1(2H)-methanol, 4,5,7-Tris(1,1-dimethylethyl)-3,4-dihydro- |
103.217 |
0 |
100.257 |
0 |
104.968 |
0 |
2 |
1-Heptacosanol |
0 |
100 |
81.125 |
18.875 |
100.008 |
0 |
3 |
Methylbenzene |
127.943 |
0 |
112.245 |
0 |
159.571 |
0 |
4 |
Cyclohexane, nonadecyl- |
0 |
100 |
90.434 |
9.566 |
97.947 |
2.053 |
5 |
Ditetradecyl ether |
0 |
100 |
0 |
100 |
0 |
100 |
6 |
Dotriacontane |
669.518 |
0 |
54.231 |
45.769 |
102.951 |
0 |
7 |
Eicosane |
73.061 |
26.939 |
85.327 |
14.673 |
97.217 |
2.783 |
8 |
Heneicosane |
85.604 |
14.396 |
42.942 |
57.058 |
0 |
100 |
9 |
Hexadecane, 2,6,10,14-tetramethyl- |
0 |
100 |
14.137 |
85.863 |
0 |
100 |
10 |
Hexatriacontane |
115.309 |
0 |
94.723 |
5.277 |
102.925 |
0 |
11 |
Octacosanol |
132.283 |
0 |
0 |
100 |
0 |
100 |
12 |
Silane, dimethyl(docosyloxy)butoxy- |
77.438 |
22.562 |
92.315 |
7.685 |
91.630 |
8.370 |
13 |
Tetracosane |
48.644 |
51.356 |
170.223 |
0 |
94.640 |
5.360 |
14 |
Tetrapentacontane |
103.246 |
0 |
82.686 |
17.314 |
117.278 |
0 |
15 |
Tetratriacontane |
107.619 |
0 |
0 |
100 |
54.433 |
45.567 |
16 |
Tetratriacontyl heptafluorobutyrate |
0 |
100 |
1.339 |
98.661 |
0 |
100 |
Based on oil compositions, it consists of aliphatic and polyaromatic hydrogen (PAH). The degradation of oil was through enzymatic system within ring cleavage by dioxygenase14 and aliphatic chain degradation by terminal monooxygenase through production fatty acid and acetyl CoA.33 In Table 1, the oil compounds were identified by dotriacontane (C32H66), eicosane (C20H42), heneicosane (C21H44), tetracosane (C24H50), and tetratriacontane (C34H70).11 Generally, the result shows that the majority of those compounds were degrade. However immobilized P. aeruginosa did not degrade dotriacontane and tetratriacontane neither with R. pickettii, while B. subtilis degraded tetratriacontane completely, in contrast within tetracosane. Immobilized R. pickettii had completely degraded heneicosane. Moreover, the highest %recovery, which was obtained by immobilized P. aeruginosa had zero %degradation on dotriacontane. Dotriacontane is a natural product and can find in soil composition synthesized by plant or actinobacterium.41 However, this compound also could be a degraded compound from another complex compound.
Soil contaminated oil bioremediation after washing pre-treatment
Washing soil treatment was declared as a pre-treatment method for reducing TPH value. So, it was applicable on bioremediation by using microorganism.11 The highest TPH value could inhibited microorganism ability on producing oxidizing-oil enzymes.14 In this study, the degradation results after washing process soil pre-treatment are shown in Fig. 3 and 4. Based on the chromatogram in Fig. 3, there was an increase in the intensity of the compounds produced by immobilized R. picketti, which exceeded the soil sample after washing pre-treatment. Meanwhile, in immobilized P. aeruginosa and B. subtilis, there was a decrease in intensity in the chromatogram. Fig. 4 shows the graphic of %recovery analysis compounds based on degradation results. Mostly, %recovery of immobilized R. pickettii were higher than control washing soil. However, some of result were exhibited zero %recovery.
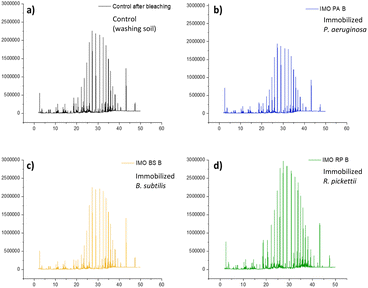 |
| Fig. 3 Chromatogram of crude oil recovery (a) control after washing pre-treatment, and immobilization by bacteria (b) P. aeruginosa, (c) B. subtilis, and (d) R. pickettii treatment 14 days. | |
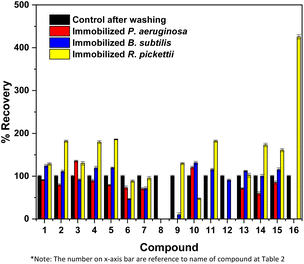 |
| Fig. 4 Crude oil recovery of washing soil by immobilized bacteria. Data showed the mean of triplicate analysis ± SD. | |
Meanwhile, Table 2 shows the %recovery and %degradation produced by immobilized bacteria in degrading oil compounds in soil samples after washing pre-treatment. Based on that table, the oil compounds were dotriacontane (C32H66), eicosane (C20H42), octadecane (C18H38), tetracosane (C24H50), and tetratriacontane (C34H70).11 R. pickettii shows high value up to 425% recovery. This was an accumulation of other compounds degraded to form tetratriacontane and it was a natural product synthesized by plant.42 Based on Table 2, P. aeruginosa can completely degrade hexacontane, hexadecane, 2,6,10,14-tetrametyl, octacosanol, octadecane, and tetratriacontane. Meanwhile, B. subtilis can only completely degrade hexacontane and tetratriacontane.
Table 2 Recovery results for metabolite compounds of washing soil treatment by immobilized bacteria
No. |
Name of compound |
P. aeruginosa |
B. subtilis |
R. pickettii |
Recovery (%) |
Degradation (%) |
Recovery (%) |
Degradation (%) |
Recovery (%) |
Degradation (%) |
1 |
1,4-Epoxynaphthalene-1(2H)-methanol, 4,5,7-Tris(1,1-dimethylethyl)-3,4-dihydro- |
90.860 |
9.140 |
124.181 |
0 |
128.479 |
0 |
2 |
1-Heptacosanol |
79.403 |
20.597 |
111.055 |
0 |
181.757 |
0 |
3 |
Benzene, methyl- |
135.260 |
0 |
91.766 |
8.234 |
130.041 |
0 |
4 |
Cyclohexane, nonadecyl- |
89.372 |
10.628 |
119.015 |
0 |
179.622 |
0 |
5 |
Cyclohexane, octadecyl- |
79.071 |
20.929 |
119.356 |
0 |
185.920 |
0 |
6 |
Dotriacontane |
73.029 |
26.971 |
46.362 |
53.638 |
88.439 |
11.561 |
7 |
Eicosane |
70.196 |
29.804 |
71.167 |
28.833 |
95.504 |
4.496 |
8 |
Hexacontane |
0 |
100 |
0 |
100 |
0 |
100 |
9 |
Hexadecane, 2,6,10,14-tetramethyl- |
0 |
100 |
10.062 |
89.938 |
129.679 |
0 |
10 |
Hexatriacontane |
120.272 |
0 |
131.175 |
0 |
47.348 |
52.652 |
11 |
Octacosanol |
0 |
100 |
115.487 |
0 |
181.951 |
0 |
12 |
Octadecane |
0 |
100 |
90.825 |
9.175 |
0 |
100 |
13 |
Silane, dimethyl (docosyloxy)butoxy- |
71.088 |
28.912 |
111.998 |
0 |
102.314 |
0 |
14 |
Tetracosane |
59.184 |
40.816 |
100.119 |
0 |
172.930 |
0 |
15 |
Tetrapentacontane |
85.086 |
14.914 |
115.267 |
0 |
160.777 |
0 |
16 |
Tetratriacontane |
0 |
100 |
0 |
100 |
425.556 |
0 |
The ability of each bacterium during the degradation process was influenced by its ability to face resistant conditions in the oil.38 This condition caused bacteria was able to die/in-active, then involved in decreasing performance in degradation. Also, the performance of bacteria was influenced by the degradative enzymes produced by each bacterium.43 Besides being influenced by enzymes, the limited ability of bacteria to penetrate immobilized beads also plays an important role in the low %degradation. This is in line with a previous study, that the immobilization method was more suitable for liquid media test samples, unlike this research that uses dry soil as test media.44 Furthermore, the mismatch of the immobilization matrix used also plays a role in this factor, where the matrix could inhibit the entry of molecules into the beads, hence bacteria were unable to completely degrade. However, based on Tables 1 and 2, all three bacteria had been proven to be capable of degrading oil compounds, but further research is needed to determine how effective these bacteria in another degradation.
Effect of washing pre-treatment on bacterial growth
By using gravimetric method, TPH values were obtained from the treatment results, which represent bacterial growth.11 From the results which are shown in the Fig. 5, it was found that there was an increasement of all bacterial species in the soil after washing process. Therefore, the soil washing process was proven to be able to eliminate impurities or high concentrations of hydrocarbon compounds, so that BPB was easier to grow and remediate oil pollutants that were contaminated in soil.
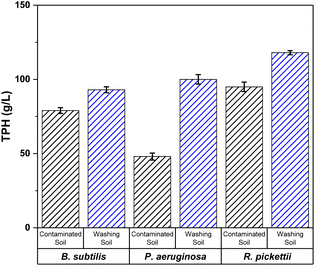 |
| Fig. 5 Involvement of soil washing on growth of BPB at PVA/SA/bentonite. This result represented mean ± SD of triplicate experiments. Statistically significant (p < 0.05) were observed among pre-treatment and each BPB result. | |
Beads synthesis and characterization
Fourier transform infrared spectroscopy (FTIR). Fourier Transform Infra-Red (FTIR) analysis on the characterization of PVA/SA/bentonite@bacteria beads were used to identify the functional groups. The FTIR results are shown in Fig. 6. Wavenumber at broad peaks around range 3500–3300 cm−1 refer to OH stretching overlapping within NH group, while peaks at around 2924 cm−1 refer to CH aliphatic stretching vibration, which identified as functional groups of bacteria, SA, and PVA components.23 Furthermore, wavenumber peaks spectra range around of 1638 cm−1 show C
C and C
O of SA and bacteria.45,46 Then, Si–O functional group of bentonites were exhibit at around 1033 cm−1.47
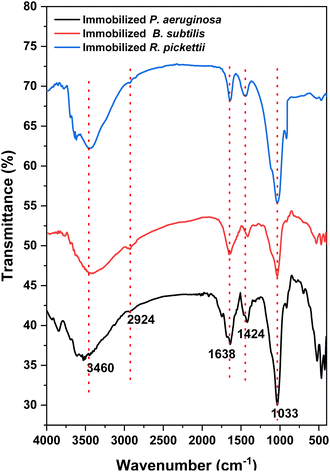 |
| Fig. 6 FTIR analysis of P. aeruginosa, B. subtilis, and R. pickettii immobilized into PVA/SA/bentonite matrix. | |
Different bacteria species additions were not shown a different significant peak. It was involved by the characteristic of bacteria which generally consist of organic biomolecule (lipid, protein, polysaccharides, etc.).46 A study reported that bacteria was arranged by OH, CH, NH, C
O and C–O functional groups.46 Hence, the FTIR spectra of synthesized beads within different bacteria addition had similarity of each peak.
Morphological analysis. Besides being characterized using FTIR, the beads were also analyzed for their topological and morphological shape using Scanning Electron Microscopy (SEM). Fig. 7a–c exhibit SEM images of PVA/SA/bentonite surface visuals each bacterium. The bacteria were stick and agglomerate within their matrix synthesized. It also showed that the bacteria were spread evenly at their matrix.
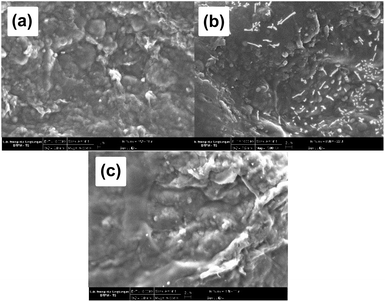 |
| Fig. 7 The SEM results of the PVA/SA/bentonite beads at 10.000× magnification (a) P. aeruginosa, (b) B. subtilis, and (c) R. pickettii immobilized bacteria. | |
Conclusion
BPB (P. aeruginosa, B. subtilis, and R. pickettii) had been analyzed for degrading TPH contaminated soil. They were successfully immobilized into PVA/SA/bentonite, which could enhance their ability to face stress level contaminant. GCMS analysis showed that immobilized bacteria completely degraded tetratriacontane by immobilized B. subtilis and heneicosane by immobilized R. pickettii. Furthermore, after washing soil pre-treatment, immobilized bacteria could completely degrade octadecane (immobilized P. aeruginosa and immobilized R. pickettii) and tetratriacontane (immobilized P. aeruginosa and immobilized B. subtilis). Therefore, this study proved that all these immobilized BPB could degrade hydrocarbon pollutant by their eco-friendly ways.
Author contributions
Adi Setyo Purnomo: conceptualization, methodology, investigation, validation, resources, writing – original draft, writing – review & editing, funding acquisition. Surya Rosa Putra: methodology, validation, supervision. Herdayanto Sulistyo Putro: supervision, validation. Afan Hamzah: supervision, validation. Nova Ainur Rohma: methodology, investigation, writing – original draft. Alya Awinatul Rohmah: revision and editing. Hamdan Dwi Rizqi: validation, formal analysis. Asranudin: visualization, writing – review & editing. Bieby Tangahu: conceptualization, methodology, resources. IDAA Warmadewanthi: conceptualization, methodology, resources. Kuniyoshi Shimizu: validation, supervision, writing – review & editing.
Conflicts of interest
There are no conflicts to declare.
Acknowledgements
The authors convey sincere thanks to the Directorate of Research and Community Service, Institut Teknologi Sepuluh Nopember (ITS), Indonesian Ministry of Education and Culture, for supporting research grant under scheme of “Scientific Research 2022”, Number: 936/PKS/ITS/2022. Part of fund was also provided by Directorate of Global Engagement ITS under scheme of “Outbound Researcher Mobility”.
Notes and references
- D. Wang, J. Lin, J. Lin, W. Wang and S. Li, Biodegradation of Petroleum Hydrocarbons by Bacillus subtilis BL-27, a Strain with Weak Hydrophobicity, Molecules, 2019, 24, 3021 CrossRef CAS PubMed.
- J. G. Speight Chapter 1 – Heavy Oil, Extra Heavy Oil, and Tar Sand Bitumen, in Heavy Oil Recovery and Upgrading, ed. Speight, J. G., Gulf Professional Publishing, 2019, pp. 3–47, DOI:10.1016/B978-0-12-813025-4.00001-5.
- K. Sayed, L. Baloo and N. K. Sharma, Bioremediation of Total Petroleum Hydrocarbons (TPH) by Bioaugmentation and Biostimulation in Water with Floating Oil Spill Containment Booms as Bioreactor Basin, Int. J. Environ. Res. Public Health, 2021, 18, 2226 CrossRef CAS PubMed.
- I. Chicca, S. Becarelli and S. Di Gregorio, Microbial Involvement in the Bioremediation of Total Petroleum Hydrocarbon Polluted Soils: Challenges and Perspectives, Environments, 2022, 9, 52 CrossRef.
- W. Kiyingi, et al., Crude oil wax: a review on formation, experimentation, prediction, and remediation techniques, Pet. Sci., 2022, 19, 2343–2357 CrossRef CAS.
- S. Bala, et al., Recent Strategies for Bioremediation of Emerging Pollutants: A Review for a Green and Sustainable Environment, Toxics, 2022, 10, 484 CrossRef CAS PubMed.
- S. Behera and S. Das, Potential and prospects of Actinobacteria in the bioremediation of environmental pollutants: cellular mechanisms and genetic regulations, Microbiol. Res., 2023, 273, 127399 CrossRef CAS PubMed.
- M. Tripathi, D. N. Singh, N. Prasad and R. Gaur Advanced Bioremediation Strategies for Mitigation of Chromium and Organics Pollution in Tannery, in Rhizobiont in Bioremediation of Hazardous Waste, ed. Kumar, V., Prasad, R. and Kumar, M., Springer Singapore, 2021, pp. 195–215, DOI:10.1007/978-981-16-0602-1_10.
- R. Rathour, J. Gupta, B. Tyagi, T. Kumari and I. S. Thakur, Biodegradation of pyrene in soil microcosm by Shewanella sp. ISTPL2, a psychrophilic, alkalophilic and halophilic bacterium, Bioresour. Technol. Rep., 2018, 4, 129–136 CrossRef.
- E. C. Omenna, K. Omage, E. Ezaka and M. A. Azeke, Tolerance, taxonomic and phylogenetic studies of some bacterial isolates involved in bioremediation of crude oil polluted soil in the southern region of Nigeria, Heliyon, 2023, 9, e15639 CrossRef CAS PubMed.
- P. Bharali, et al., Bioprospecting of indigenous biosurfactant-producing oleophilic bacteria for green remediation: an eco-sustainable approach for the management of petroleum contaminated soil, 3 Biotech, 2022, 12, 13 CrossRef PubMed.
- E. M. de Oliveira, et al., Isolation and Characterization of Biosurfactant-Producing Bacteria from Amapaense Amazon Soils, Int. J. Microbiol., 2021, 2021, e9959550 Search PubMed.
- A. S. Purnomo, et al., Biodegradation of crude oil by Ralstonia pickettii under high salinity medium, Mal. J. Fund. Appl. Sci., 2019, 15, 377–380 CrossRef.
- L. F. Chuah, K. W. Chew, A. Bokhari, M. Mubashir and P. L. Show, Biodegradation of crude oil in seawater by using a consortium of symbiotic bacteria, Environ. Res., 2022, 213, 113721 CrossRef CAS PubMed.
- A. S. Purnomo, H. D. Rizqi and L. Harmelia, Culture of Bacterium Bacillus subtilis as Degradation Agent in Attempt of Sea Water Remediation Contaminated By Petroleum, J. Indian Chem. Soc., 2020, 3, 53 Search PubMed.
- F. Liu, et al., Remediation of petroleum hydrocarbon-contaminated groundwater by biochar-based immobilized bacteria, Biochem. Eng. J., 2023, 197, 108987 CrossRef CAS.
- S. Aggarwal, A. Chakravarty and S. Ikram, A comprehensive review on incredible renewable carriers as promising platforms for enzyme immobilization & thereof strategies, Int. J. Biol. Macromol., 2021, 167, 962–986 CrossRef CAS PubMed.
- L. T. Nhi-Cong, et al., Advanced materials for immobilization of purple phototrophic bacteria in bioremediation of oil-polluted wastewater, Chemosphere, 2021, 278, 130464 CrossRef CAS PubMed.
- J. Li, et al., Use of calcium alginate/biochar microsphere immobilized bacteria Bacillus sp. for removal of phenol in water, Environ. Challenges, 2022, 9, 100599 CrossRef CAS.
- Q. Lin, et al., Simultaneous adsorption and biodegradation of polychlorinated biphenyls using resuscitated strain Streptococcus sp. SPC0 immobilized in polyvinyl alcohol-sodium alginate, Sci. Total Environ., 2023, 868, 161620 CrossRef CAS PubMed.
- Y. Zhang, et al., Immobilization of nitrifying bacteria in magnetic PVA–SA-diatomite carrier for efficient removal of NH4+-N from effluents, Environ. Technol. Innovation, 2021, 22, 101407 CrossRef CAS.
- Ravi and L. M. Pandey, Enhanced adsorption capacity of designed bentonite and alginate beads for the effective removal of methylene blue, Appl. Clay Sci., 2019, 169, 102–111 CrossRef CAS.
- E. Baigorria, L. A. Cano, L. M. Sanchez, V. A. Alvarez and R. P. Ollier, Bentonite-composite polyvinyl alcohol/alginate hydrogel beads: Preparation, characterization, and their use as arsenic removal devices, Environ. Nanotechnol., Monit. Manage., 2020, 14, 100364 Search PubMed.
- C. Liu, et al., Efficiency of diesel-contaminated soil washing with different tween 80 surfactant concentrations, pH, and bentonite ratios, Environ. Res., 2022, 214, 113830 CrossRef CAS PubMed.
- A. A. Rohmah, A. S. Purnomo and W. N. Safitri, Biodecolorization of Methylene Blue by Using Bacillus subtilis Immobilized into SA-PVA-Bentonite Matrix in Mineral Salt Medium and Non-nutritious Medium, Indones. J. Chem., 2022, 22, 1637–1650 CrossRef CAS.
- B. Nabilah, A. S. Purnomo, H. D. Rizqi, H. S. Putro and R. Nawfa, The effect of Ralstonia pickettii bacterium addition on methylene blue dye biodecolorization by brown-rot fungus Daedalea dickinsii, Heliyon, 2022, 8, e08963 CrossRef CAS PubMed.
- T. R. Alkas, et al., Immobilization of UiO-66/Brown-rot fungi (BRF) in PVA-SA matrix and its performance for methylene blue decolorization, Mater. Today Chem., 2023, 29, 101411 CrossRef.
- Asranudin, et al., Hectorite-CTAB–alginate composite beads for water treatment: kinetic, isothermal and thermodynamic studies, RSC Adv., 2023, 13, 790–801 RSC.
- H. Liu, M. Wu, H. Gao, N. Yi and X. Duan, Hydrocarbon transformation pathways and soil organic carbon stability in the biostimulation of oil-contaminated soil: implications of 13C natural abundance, Sci. Total Environ., 2021, 788, 147580 CrossRef CAS PubMed.
- M. Saeed, et al., Development of novel kinetic model based on microbiome and biochar for in-situ remediation of total petroleum hydrocarbons (TPHs) contaminated soil, Chemosphere, 2023, 324, 138311 CrossRef CAS PubMed.
- K. Tao, et al., Response of soil bacterial community to bioaugmentation with a plant residue-immobilized bacterial consortium for crude oil removal, Chemosphere, 2019, 222, 831–838 CrossRef CAS PubMed.
- H. Zhou, et al., Enhanced remediation of oil-contaminated intertidal sediment by bacterial consortium of petroleum degraders and biosurfactant producers, Chemosphere, 2023, 330, 138763 CrossRef CAS PubMed.
- A. A. Ivanova, S. A. Mullaeva, O. I. Sazonova, K. V. Petrikov and A. A. Vetrova, Current research on simultaneous oxidation of aliphatic and aromatic hydrocarbons by bacteria of genus Pseudomonas, Folia Microbiol, 2022, 67, 591–604 CrossRef CAS.
- T. Krucoń, Z. Ruszkowska, W. Pilecka, A. Szych and Ł. Drewniak, Bioprospecting of the Antarctic Bacillus subtilis strain for potential application in leaching hydrocarbons and trace elements from contaminated environments based on functional and genomic analysis, Environ. Res., 2023, 227, 115785 CrossRef PubMed.
- H. A. Hassan and A. A. Aly, Isolation and characterization of three novel catechol 2,3-dioxygenase from three novel haloalkaliphilic BTEX-degrading Pseudomonas strains, Int. J. Biol. Macromol., 2018, 106, 1107–1114 CrossRef CAS PubMed.
- D. Seo and K.-Y. Choi, Heterologous production of pyomelanin biopolymer using 4-hydroxyphenylpyruvate dioxygenase isolated from Ralstonia pickettii in Escherichia coli, Biochem. Eng. J., 2020, 157, 107548 CrossRef CAS.
- M. L. Rodríguez-Uribe, J. J. Peña-Cabriales, M. D. C. Rivera-Cruz and J. P. Délano-Frier, Native bacteria isolated from weathered petroleum oil-contaminated soils in Tabasco, Mexico, accelerate the degradation petroleum hydrocarbons in saline soil microcosms, Environ. Technol. Innovation, 2021, 23, 101781 CrossRef.
- M. I. Ja’afaru, T. Abbas, O. M. Ajunwa and K. Olaifa, Characterization and statistical optimization of biosurfactant production using Bacillus subtilis isolated from automotive oil-contaminated soil in Yola, Nigeria, Sci. Afr., 2022, 17, e01357 Search PubMed.
- F. Zhao, et al., Anaerobic production of surfactin by a new Bacillus subtilis isolate and the in situ emulsification and viscosity reduction effect towards enhanced oil recovery applications, J. Pet. Sci. Eng., 2021, 201, 108508 CrossRef CAS.
- K. Tao, et al., Biodegradation of crude oil by a defined co-culture of indigenous bacterial consortium and exogenous Bacillus subtilis, Bioresour. Technol., 2017, 224, 327–332 CrossRef CAS PubMed.
- U. Managamuri, et al., Isolation, identification, optimization, and metabolite
profiling of Streptomyces sparsus VSM-30, 3 Biotech, 2017, 7, 217 CrossRef PubMed.
- L. Ferreira, et al., Application of Fourier transform infrared spectroscopy (FTIR) techniques in the mid-IR (MIR) and near-IR (NIR) spectroscopy to determine n-alkane and long-chain alcohol contents in plant species and faecal samples, Spectrochim. Acta, Part A, 2022, 280, 121544 CrossRef CAS.
- A. S. Purnomo, H. D. Rizqi and L. Harmelia, Culture of Bacterium Bacillus subtilis as Degradation Agent in Attempt of Sea Water Remediation Contaminated By Petroleum, J. Indian Chem. Soc., 2020, 3, 53 Search PubMed.
- S. Das and H. R. Dash Microbial Bioremediation. in Microbial Biodegradation and Bioremediation, vol. 1–21, Elsevier, 2014, DOI:10.1016/B978-0-12-800021-2.00001-7.
- M.-K. Zhang, X.-H. Ling, X.-H. Zhang and G.-Z. Han, A novel alginate/PVA hydrogel-supported Fe3O4 particles for efficient heterogeneous Fenton degradation of organic dyes, Colloids Surf., A, 2022, 652, 129830 CrossRef CAS.
- M. E. Fuller, C. Andaya and K. McClay, Evaluation of ATR-FTIR for analysis of bacterial cellulose impurities, J. Microbiol. Methods, 2018, 144, 145–151 CrossRef CAS PubMed.
- B. Nabilah, A. S. Purnomo, D. Prasetyoko and A. A. Rohmah, Methylene Blue biodecolorization and biodegradation by immobilized mixed cultures of Trichoderma viride and Ralstonia pickettii into SA-PVA-Bentonite matrix, Arabian J. Chem., 2023, 16, 104940 CrossRef CAS.
|
This journal is © The Royal Society of Chemistry 2023 |
Click here to see how this site uses Cookies. View our privacy policy here.