DOI:
10.1039/D3RA00772C
(Paper)
RSC Adv., 2023,
13, 7877-7885
Chlorination of trichlorosilane/chlorodimethylsilane using metal chlorides: experimental and mechanistic investigations
Received
5th February 2023
, Accepted 22nd February 2023
First published on 9th March 2023
Abstract
Removal of carbonaceous impurities from trichlorosilane (SiHCl3) reduces the carbon content of solar grade polysilicon produced with the improved Siemens method. The separation of chlorodimethylsilane (CH3)2SiHCl from SiHCl3 by distillation remains challenging due to the small difference in their boiling points. Herein, the chlorination of (CH3)2SiHCl/SiHCl3 with metal chlorides (WCl6, MoCl5) were studied. The aim was to convert (CH3)2SiHCl into (CH3)2SiCl2, increase the relative volatility of (CH3)2SiHCl and SiHCl3 and facilitate the distillation. The optimum reaction conditions were 60 °C, 60 min and n(WCl6 or MoCl5): n(SiHCl3 or (CH3)2SiHCl) = 0.7 at 0.8 MPa. Under these conditions, and when WCl6 and MoCl5 were used as the chlorine sources, the extents of (CH3)2SiHCl conversion were 22.7 and 18.5 times higher than those of SiHCl3, respectively. In addition, a mechanistic study showed that the difference between the reactions of SiHCl3 and (CH3)2SiHCl resulted from the different energy barriers for the reactions of the
and (CH3)2SiCl· radicals with WClx or MoClx, and the barrier for the
reaction was higher than that for the (CH3)2SiCl· reaction.
1. Introduction
Solar energy is a renewable and clean energy that will play an important role in solving the energy crisis.1–3 Therefore, polysilicon has rapidly developed as a raw material for solar photovoltaic cells. It is important to limit the impurities contained in solar-grade polysilicon to obtain higher photoelectric conversion efficiencies.
The standard for solar-grade polysilicon requires the carbon atom concentration to be less than 5 × 1018 atoms per cm3.4 Currently, the main process used in producing polysilicon is the improved Siemens method.1,5 Since the final polysilicon product is obtained by reacting SiHCl3 with H2 in a bell jar furnace,5 carbon impurities in SiHCl3 should be strictly limited. Therefore, the removal of carbonaceous impurities in SiHCl3 is a key step in the improved Siemens method.
The carbonaceous impurities in SiHCl3 were mainly methylchlorosilanes [(CH3)nSiCl4−n, n = 1–3].6 Moreover, the boiling points of CH3SiHCl2 (41.9 °C) and (CH3)2SiHCl (34.7 °C) are close to the boiling point of SiHCl3 (32.0 °C).7 Azeotropes are easily formed during SiHCl3 purification via distillation. Thus, purifying SiHCl3 by distillation is difficult. One possible method is to convert CH3SiHCl2 and (CH3)2SiHCl into methylchlorosilanes with high boiling points and high chlorine contents by chlorination. This would result in higher relative volatility and make distillation easier.
Typical chlorine sources for the chlorination reactions of CH3SiHCl2 are chlorine gas,8 chlorinated hydrocarbons6,9 and chlorosilane.4,10,11 Wan et al.8 proposed photochlorination of CH3SiHCl2 with Cl2 in a continuous microchannel reactor. The results showed that the removal rate of CH3SiHCl2 was as high as 99.67% under the optimal reaction conditions. But this method is currently in the laboratory research stage. In addition, Zhang and Huang9 reported catalytic chlorination of CH3SiHCl2 with carbon tetrachloride (CCl4) over a Pd/Al2O3 catalyst. However, the introduction of new carbon impurities cannot be avoided, and the high price of Pd limits its utilization in industry. Additionally, silicon tetrachloride, a byproduct of polysilicon production via a modified Siemens process, can also be used as a chlorine source for chlorination of CH3SiHCl2.4 However, silicon powder is easily formed, blocking the pores of the activated carbon catalyst during this process. Therefore, the catalytic performance and stability of the activated carbon catalyst are poor.
Chlorination of (CH3)2SiHCl with LiCl as the chloride source and B(C6F5)3 as the catalyst was reported to occur in a mixture of ethyl ether and toluene.12 Obviously, many researchers are studying chlorination reactions of CH3SiHCl2. However, the boiling point of (CH3)2SiHCl is closer to that of SiHCl3, which makes separation more difficult. Furthermore, SiHCl3 and (CH3)2SiHCl may be chlorinated at the same time. Therefore, it is important to study the competitive relationship between the SiHCl3 and (CH3)2SiHCl during chlorination reactions.
Metal chlorides are also used as catalysts in chlorination reactions. For instance, (a) chlorination of methylphenyldichlorosilane to chlorinated methylphenyldichlorosilanes with gaseous chlorine has been catalysed by FeCl3, SbCl5, SnCl4 and AlCl3;13 (b) chlorination of 1,3-dithiolanes and 1,3-dithianes with CH2Cl2 has been catalysed by WCl6;14 and (c) chlorination of allyl groups in terpenic olefins (β-pinene and carvone) with NaClO has been catalysed by MoCl5, AlCl3, FeCl3 and FeCl2.15,16 Metal chlorides can be used not only as catalysts but also directly as chlorine sources in reactions, such as in the photochemical chlorination of methane mediated by FeCl3.17 In addition, the boiling points of WCl6 (346.7 °C) and MoCl5 (268.0 °C) are very high and they are easily removed by distillation.
Therefore, this work will focus on the thermal chlorination reaction of SiHCl3 and (CH3)2SiHCl by using WCl6 and MoCl5 as chlorine donors. The effects of the metal chloride type, molar ratio of reactants, reaction temperature and reaction time were investigated in detail. Finally, a reaction mechanism was proposed and explored in detail with density functional theory calculations.
2. Experiments and calculations
2.1 Materials used
Analytical standard dimethylchlorosilane ((CH3)2SiHCl, 99.0%) and trichlorosilane (SiHCl3, 99.0%) were purchased from Sigma-Aldrich. Silicon tetrachloride (SiCl4, 99.0%) was kindly supplied by Xinjiang Daqo New Energy Co. Additionally, dichlorodimethylsilane ((CH3)2SiCl2, 99.0%), chloromethyldichloromethylsilane ((CH2Cl)CH3SiCl2, 99.0%), tungsten hexachloride (WCl6, 99.5%) and molybdenum pentachloride (MoCl5, 99.6%) were purchased from Adamas.
2.2 Experimental setup
The specific experimental operations were as follows: first, to prepare stock solutions, SiHCl3 or (CH3)2SiHCl was dissolved in SiCl4 at a concentration of 0.5 mol L−1. In addition, a given amount of WCl6 or MoCl5 and 10 mL of stock solution were added to a 36.5 mL batch reactor. WCl6 and MoCl5 were easily soluble in SiCl4 and formed homogeneous reaction systems, and the molar ratios of reactants [n(WCl6/MoCl5)
:
n(SiHCl3/(CH3)2SiHCl)] were 0.3, 0.7 and 1.2. Next, a nitrogen stream was introduced into the reactor three times to replace the air. To maintain a homogeneous reaction system, the N2 pressure was raised to a higher reaction pressure (0.8 MPa). Then, the reactor was heated in a water bath and cooled with an ice-salt bath (−20 °C) after the reaction. Finally, the reactor was opened after slowly relieving the pressure. The samples were then removed from the reactor and distilled to remove metal impurities.
The mole fraction of the chlorosilane solution was determined with a 9790 Plus gas chromatograph equipped with a thermal conductivity detector (TCD), and H2 was used as the carrier gas. The gas chromatography (GC) detection conditions were as follows: a 3 m 25% DC-550/Chromo packed column; an injection temperature of 150 °C; and a detector temperature of 150 °C. In addition, a programmed temperature rise was used for the oven temperature, i.e., it was first held at 60 °C for 2.5 min, then raised to 120 °C at a rate of 30 °C min−1, and finally held at 120 °C for 2.5 min. The identities of the products were determined from the retention times of standard samples.
The conversion of SiHCl3 was calculated according to eqn (1), and it was expressed as X(SiHCl3). The inlet and outlet mole fractions of SiHCl3 were expressed as x(SiHCl3)in and x(SiHCl3)out, respectively. The formula used for calculation of the (CH3)2SiHCl conversion rate was the same as that used for SiHCl3.
|
 | (1) |
To further identify the components in the reaction product, samples were dissolved in deuterated chloroform (CDCl3) and qualitatively analysed with nuclear magnetic resonance (NMR) spectroscopy using a Bruker Avance III [1H (400 MHz),13C (101 MHz)].
2.3 Theoretical method
The reactions discussed herein are free radical reactions, including chain initiation reactions, chain propagation reactions, and chain termination reactions. All the calculations were performed with the Gaussian 16 program.18 The geometric configuration of each stationary point for a reactant, transition state, or product along the reaction pathway was studied with B3LYP calculations by using the def2-TZVP basis set for the metal atoms and the 6-311G++(2d,p) basis set for the remaining atoms. In addition, frequency analyses were performed to ensure that the structure determined for a reactant or product was at a local minimum (all frequencies were positive) or in a transition state (only one negative frequency). The intrinsic reaction coordinates (IRCs) were used to evaluate whether the structures of the transition states were correctly connected to the products and reactants.19 The energy (E) in the entire reaction process was taken from the Gibbs free energy in the output file, that is, EE + thermal free energy correction (T = 298.15 K). The energy barrier (EB), the energy change (ΔE) and the dissociation energy (DE) were calculated with eqn (2), (3), and (4). |
EB = E(transition state) − E(reactant)
| (2) |
|
ΔE = E(product) − E(reactant)
| (3) |
|
DE = ∑E(free radicals) − E(molecular)
| (4) |
3. Results and discussion
3.1 Experimental research on chlorination of SiHCl3/(CH3)2SiHCl with WCl6
3.1.1 Effect of reaction temperature. The influence of reaction temperature on the conversion rate for the reaction of WCl6 and SiHCl3/(CH3)2SiHCl was investigated over the temperature range of 40–80 °C with a reaction pressure of 0.8 MPa and n(WCl6)
:
n[SiHCl3/(CH3)2SiHCl] = 0.3. The SiHCl3 or (CH3)2SiHCl conversion rate as a function of temperature is shown in Fig. 1. These results showed that the conversion rates for (CH3)2SiHCl and SiHCl3 both increased with increasing temperature and time. The conversion rate of SiHCl3 was extremely low, between 0.8% and 6.3% at 40 °C to 80 °C for 10 min to 120 min. The (CH3)2SiHCl conversion was also low, between 2.0% and 9.5%, at 40 °C. However, when the reaction temperature was 60 °C, the conversion of (CH3)2SiHCl increased substantially from 3.3% to 59.9% with an increase in the reaction time from 10 min to 30 min. By prolonging the reaction time to 60 min, the conversion of (CH3)2SiHCl gradually increased to 75.2%. And the conversion of (CH3)2SiHCl increased slightly with increasing temperature and time. Therefore, the optimum reaction conditions were 60 °C for 60 min.
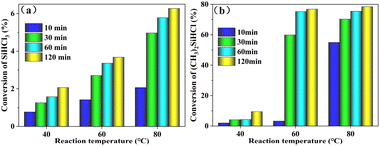 |
| Fig. 1 Conversions of (a) SiHCl3/(b) (CH3)2SiHCl in chlorination reactions run with WCl6 at different reaction temperatures. | |
In addition, a solid precipitated from the reaction products obtained with conversion rates greater than 50%. The metal chloride is highly moisture-sensitive, and it was difficult to analyse it further. Since the high-valent tungsten chloride was highly soluble in silicon tetrachloride, (CH3)2SiHCl is thought to react with WCl6 to form a low-valent tungsten chloride or elemental tungsten.
3.1.2 Effect of reactant ratio. The conversions of SiHCl3 or (CH3)2SiHCl observed for chlorination reactions run with WCl6 at different reactant ratios are shown in Fig. 2. Herein, the experimental conditions included a reaction pressure of 0.8 MPa, a reaction temperature of 60 °C and a reaction time of 60 min. When WCl6 and (CH3)2SiHCl were reacted, with increases in the molar ratio of WCl6 to (CH3)2SiHCl from 0.3 to 0.7 and 1.2, the conversion of (CH3)2SiHCl increased from 75.2% to 100.0% and 100.0%. The conversion rate of SiHCl3 was far lower than that of (CH3)2SiHCl. Obviously, the optimum molar ratio of WCl6 to (CH3)2SiHCl/SiHCl3 was 0.7. The ratio of the two conversion rates was 22.7.
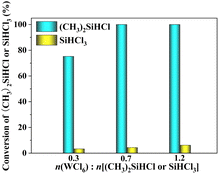 |
| Fig. 2 Conversions of SiHCl3/(CH3)2SiHCl during chlorination of SiHCl3/(CH3)2SiHCl with WCl6 at different reactant ratios. | |
The product from the 100% conversion reaction was analysed by GC. It contained a large amount of (CH3)2SiCl2 and a small amount of (CH2Cl)CH3SiCl2. To further confirm the composition of the sample, the product was qualitatively analysed by 1H NMR and 13C NMR, as shown in Fig. 3. The main product (CH3)2SiCl2 (1H NMR: 0.81 ppm; 13C NMR: 6.83 ppm) was identified from the NMR spectrum. Therefore, the main product of the reaction between (CH3)2SiHCl and WCl6 was (CH3)2SiCl2, and the byproduct was (CH2Cl)CH3SiCl2.
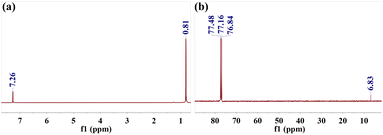 |
| Fig. 3 (a) 1H NMR and (b) 13C NMR spectra for the product of (CH3)2SiHCl chlorination by WCl6. | |
3.2 Experimental research on chlorination of SiHCl3/(CH3)2SiHCl with MoCl5
3.2.1 Effect of reaction temperature. The conversion rates for SiHCl3/(CH3)2SiHCl in chlorination reactions run with MoCl5 at different temperatures are shown in Fig. 4. Here, the reaction conditions were the same as those used for SiHCl3/(CH3)2SiHCl and WCl6. Similarly, the conversion rates for (CH3)2SiHCl and SiHCl3 both increased with increases in temperature and time. The conversion rate of SiHCl3 was very low. (CH3)2SiHCl hardly reacted at 40 °C but reacted rapidly at 60 °C and 80 °C. Apparently, the conversion levels for the MoCl5 reaction with (CH3)2SiHCl were much higher than those with SiHCl3 at 60 °C and 80 °C. In addition, the conversion of (CH3)2SiHCl was as high as 64.0% at 60 °C for 60 min. Therefore, we will continue to explore the effect of the reactant ratio on the conversion of SiHCl3/(CH3)2SiHCl in the chlorination reactions with MoCl5 under these reaction conditions. Additionally, when the products were formed with conversion rates greater than 40%, a solid deposit formed during the reaction of MoCl5 with (CH3)2SiHCl. The reaction was also presumed to yield a low-valent molybdenum chloride or elemental molybdenum.
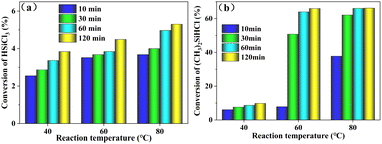 |
| Fig. 4 Conversions of (a) SiHCl3/(b) (CH3)2SiHCl in chlorination reactions run with MoCl5 at different reaction temperatures. | |
3.2.2 Effect of reactant ratio. Fig. 5 shows the conversion of SiHCl3/(CH3)2SiHCl during chlorination with MoCl5 at different reactant ratios. Herein, the experiment was also carried out at 0.8 MPa, 60 °C and 60 min. Fig. 5 shows that high molar ratios of MoCl5 to SiHCl3/(CH3)2SiHCl favoured conversion of SiHCl3 or (CH3)2SiHCl. As expected, the conversion rate of (CH3)2SiHCl was still much higher than that of SiHCl3. When the molar ratio of MoCl5 to SiHCl3/(CH3)2SiHCl was 0.7, the ratio of the two conversion levels was the largest at 18.5. Therefore, the optimum conditions were 60 °C, 60 min and n(MoCl5)
:
n(SiHCl3 or (CH3)2SiHCl) = 0.7 for chlorination of SiHCl3/(CH3)2SiHCl with MoCl5 at 0.8 MPa. Furthermore, the product formed with 100% conversion was also analysed by GC, 1H NMR and 13C NMR, which showed that the main product of the reaction between (CH3)2SiHCl and MoCl5 was (CH3)2SiCl2 and that the byproduct was (CH2Cl)CH3SiCl2.
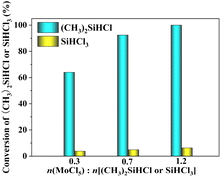 |
| Fig. 5 Conversions of SiHCl3 or (CH3)2SiHCl in chlorination reactions run with MoCl5 at different reactant ratios. | |
3.3 Mechanism calculation of chlorination of SiHCl3/(CH3)2SiHCl with WCl6
To verify the results of the calculations, the main geometric parameters of SiHCl3, (CH3)2SiHCl, WCl6 and MoCl5 were compared with the reported experimental results. It can be seen from the data in Table 1 that the calculated geometric parameters have small errors compared with the experimental values.
Table 1 Calculated and experimental geometric parameters of SiHCl3, (CH3)2SiHCl, WCl6 and MoCl5 (bond lengths/Å and bond angles/deg)
Compound |
Parameter |
Value |
Calculated |
Experimental20–22 |
Equatorial plane. Axial direction. |
SiHCl3 |
Si–H |
1.462 |
1.464 |
|
Si–Cl |
2.052 |
2.020 |
|
Cl–Si–Cl |
109.582 |
109.4 |
|
Cl–Si–H |
109.360 |
109.5 |
(CH3)2SiHCl |
Cl–Si |
2.101 |
2.0604 |
|
C–Si |
1.868 |
1.8542 |
|
Cl–Si–C |
108.37 |
108.43 |
|
C–Si–C |
112.83 |
112.32 |
WCl6 |
W–Cl |
2.316 |
2.26 ± 0.02 |
MoCl5 |
Mo–Cl |
2.247a, 2.324b |
2.27 ± 0.02 |
3.3.1 Chain initiation reaction. The dissociation energies for the bonds in WClx (x indicates the number of chlorine atoms in the metal chloride, and 1 ≤ x ≤ 6), SiHCl3 and (CH3)2SiHCl are shown in Table 2 and 3. By comparing the dissociation energies, it was found that the bond energy of the W–Cl bonds is the smallest in SiHCl3 and WClx (4 ≤ x ≤ 6), with values of 155.6 kJ mol−1, 202.0 kJ mol−1 and 314.2 kJ mol−1 respectively. Moreover, the Si–H bond, with an energy of 333.3 kJ mol−1, is the weakest bond in SiHCl3, WCl3, WCl2, and WCl. Therefore, chain initiation reaction can be divided into cleavage of the Si–H bond and cleavage of the W–Cl bond. Chain initiation involves a decomposition reaction in which Cl· is released from WClx (eqn (5)) for the SiHCl3 reaction with WCl6, WCl5, and WCl4. In contrast, in the reactions between SiHCl3 and WCl3, WCl2, and WCl, the chain initiation reaction is cleavage of the Si–H bond of SiHCl3 (eqn (6)). |
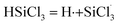 | (6) |
Table 2 Dissociation energies of the W–Cl bonds in WClx
Compound |
Parameter |
DE/kJ mol−1 |
WCl6 |
W–Cl |
155.6 |
WCl5 |
|
202.0 |
WCl4 |
|
314.2 |
WCl3 |
|
374.4 |
WCl2 |
|
389.5 |
WCl |
|
368.6 |
Table 3 Dissociation energies for SiHCl3 and (CH3)2SiHCl
Compound |
Parameter |
DE/kJ mol−1 |
SiHCl3 |
H–Si |
333.3 |
|
Si–Cl |
379.6 |
(CH3)2SiHCl |
H–Si |
343.5 |
|
H–C |
378.4 |
|
Si–Cl |
411.9 |
Correspondingly, in reactions of (CH3)2SiHCl with WCl6, WCl5 and WCl4, the chain initiation reaction is cleavage of the W–Cl bond in WClx (eqn (5)). In the (CH3)2SiHCl reactions with WCl3, WCl2 and WCl, the chain initiation reaction involves breakage of the Si–H bond in (CH3)2SiHCl (eqn (7)).
|
(CH3)2SiHCl = H· + (CH3)2SiCl·
| (7) |
3.3.2 Chain propagation reaction. In the SiHCl3 reactions with WCl6, WCl5 and WCl4, the chlorine atoms from WClx continue to react with SiHCl3. Then, chain growth could occur via two reactions: ① in the substitution reaction, Cl· attacks the silicon atom in SiHCl3 to generate H· and SiCl4, and the energy barrier for this step is 144.8 kJ mol−1. The structure of transition state TS1 involved in this reaction is shown in Fig. 6. ② In the hydrogen abstraction reaction, Cl· abstracts the hydrogen atom on silicon to generate HCl and
, and the energy barrier is so small that it can be treated as no energy barrier. DeSain et al. also considered the reaction to be a barrierless hydrogen abstraction reaction.23
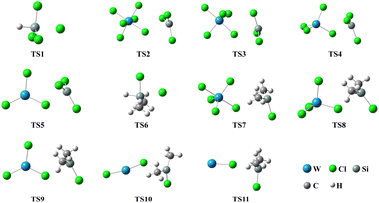 |
| Fig. 6 Structures of transition states formed during the reactions of SiHCl3/(CH3)2SiHCl with WCl6. | |
Therefore, when WCl6, WCl5 and WCl4 react with SiHCl3, the chain growth reaction is hydrogen transfer between SiHCl3 and Cl· (eqn (8)). The generated
continues to react with WCl6, WCl5 and WCl4 (eqn (9)). In the SiHCl3 reactions with WCl3, WCl2, and WCl,
from SiHCl3 continues to react with WClx (eqn (9)). The relative energies for the reactions of SiHCl3 with WCl6, WCl5, WCl4 and WCl3 are shown in Fig. 7. The structures of the transition states involved in each reaction are shown in Fig. 6.
|
 | (8) |
|
 | (9) |
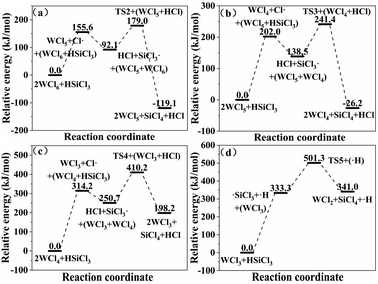 |
| Fig. 7 (a–d) Relative energies for the reactions of SiHCl3 with WClx. | |
In the (CH3)2SiHCl reactions with WCl6, WCl5 and WCl4, the Cl· from WClx decomposition continues to react with (CH3)2SiHCl. There are also two possible chain growth reactions. ① Substitution reaction: Cl· directly attacks the silicon atom in (CH3)2SiHCl, passes through transition state TS6, and generates H· and (CH3)2SiCl2, and the reaction energy barrier is 85.6 kJ mol−1. TS6 is shown in Fig. 6. ② Hydrogen abstraction reaction: Cl· attacks the hydrogen atom on silicon to generate (CH3)2SiCl· and HCl. This reaction step is also regarded as having no energy barrier.
Therefore, the pathway followed in the reaction of Cl· with (CH3)2SiHCl is hydrogen transfer from silicon to the chlorine atom (eqn (10)). The generated (CH3)2SiCl· continues to react with WCl6, WCl5, and WCl4 to form (CH3)2SiCl2 (eqn (11)). In the (CH3)2SiHCl reactions with WCl3, WCl2, and WCl, (CH3)2SiCl· and WClx react to form (CH3)2SiCl2 (eqn (11)). The relative energies for the (CH3)2SiHCl reaction with WCl6, WCl5, WCl4, WCl3, WCl2 and WCl are shown in Fig. 8. The structures of the transition states involved in each reaction are shown in Fig. 6.
|
(CH3)2SiHCl + Cl· = HCl + (CH3)2SiCl·
| (10) |
|
WClx + (CH3)2SiCl· = (CH3)2SiCl2 + WClx−1
| (11) |
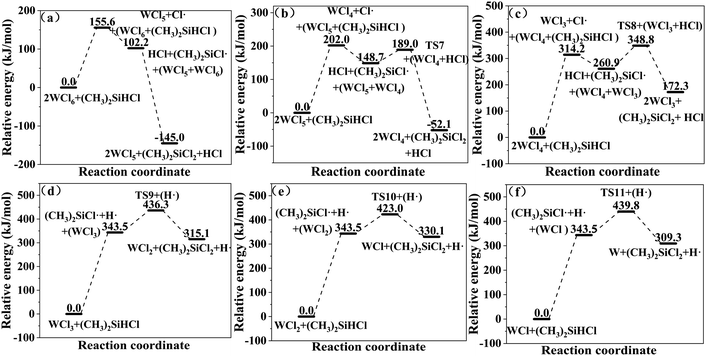 |
| Fig. 8 (a–f) Relative energies for the reactions of (CH3)2SiHCl with WClx. | |
Fig. 7 and 8 show that the difference in the reactions of SiHCl3 and (CH3)2SiHCl with WClx lies in the energy barriers of the
and (CH3)2SiCl· reactions with WClx. The energy barriers and Gibbs free energy changes of the reactions of WClx with
and (CH3)2SiCl· are shown in Table 4. The data show that the energy barriers for the reactions of WCl6, WCl5, WCl4 and WCl3 with
are higher than those for the (CH3)2SiCl· reactions, with differences of 86.9 kJ mol−1, 62.6 kJ mol−1, 71.6 kJ mol−1 and 75.2 kJ mol−1. These results also show that ΔG > 0 for the reactions of WCl3, WCl2, and WCl with
and that ΔG < 0 for the reactions of WCl3, WCl2, and WCl with (CH3)2SiCl·. Obviously, WClx reacts more readily with (CH3)2SiHCl. These results are consistent with the experimental data, which demonstrates the practicality of the calculations.
Table 4 Energy barriers and Gibbs free energy changes for the reactions of WClx with
and (CH3)2SiCl·
Reactant |
EB (kJ mol−1) |
ΔGa (kJ mol−1) |
The formula used for calculation of ΔG was the same as that for ΔE (eqn (3)). |
WCl6 |
·SiCl3 |
86.9 |
−211.2 |
|
·(CH3)2SiCl |
0 |
−247.2 |
WCl5 |
·SiCl3 |
102.9 |
−164.7 |
|
·(CH3)2SiCl |
40.3 |
−200.8 |
WCl4 |
·SiCl3 |
159.5 |
−52.5 |
|
·(CH3)2SiCl |
87.9 |
−88.6 |
WCl3 |
·SiCl3 |
168.0 |
7.7 |
|
·(CH3)2SiCl |
92.8 |
−28.4 |
WCl2 |
·SiCl3 |
— |
22.7 |
|
·(CH3)2SiCl |
79.5 |
−13.4 |
WCl |
·SiCl3 |
— |
1.8 |
|
·(CH3)2SiCl |
96.3 |
−34.2 |
3.3.3 Chain termination reaction. At the chain termination stage, free radicals combine with each other to form the bond with the largest bond energy. According to a comparative analysis using the data in Tables 5 and 6, in the reactions of SiHCl3 and (CH3)2SiHCl with WCl6, WCl5, and WCl4, chain termination involves reactions of both
and (CH3)2SiCl· with Cl· (eqn (12) and (13)). The calculated dissociation energy for H2 is 436.9 kJ mol−1. Hence, in the reactions of SiHCl3 and (CH3)2SiHCl with WCl3, WCl2, and WCl, the chain termination reaction involves the combination of two H· (eqn (14)). |
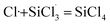 | (12) |
|
(CH3)2SiCl· + Cl· = (CH3)2SiCl2
| (13) |
Table 5 Free radicals and dissociation energies in the chlorination reaction of SiHCl3
DE/kJ mol−1 |
·Cl |
·SiCl3 |
·Cl |
193.6 |
366.8 |
·SiCl3 |
— |
216.4 |
Table 6 Free radicals and dissociation energies in the chlorination reaction of (CH3)2SiHCl
DE/kJ mol−1 |
·Cl |
·(CH3)2SiCl |
·CH2CH3SiCl2 |
·Cl |
193.6 |
402.9 |
264.0 |
·(CH3)2SiCl |
— |
234.6 |
275.4 |
·CH2CH3SiCl2 |
— |
— |
250.9 |
In summary, the overall process for SiHCl3 and (CH3)2SiHCl reactions with WCl6, WCl5 and WCl4 is shown in eqn (15) and (16), and the overall process for reactions with WCl3, WCl2 and WCl is shown in eqn (17) and (18).
|
HSiCl3 + 2WClx = SiCl4 + 2WClx−1 + HCl
| (15) |
|
(CH3)2SiHCl + 2WClx = (CH3)2SiCl2 + 2WClx−1 + HCl
| (16) |
|
2HSiCl3 + 2WClx = 2SiCl4 + 2WClx−1 + H2
| (17) |
|
2(CH3)2SiHCl + 2WClx = 2(CH3)2SiCl2 + 2WClx−1 + H2
| (18) |
3.4 Mechanism calculation of chlorination of SiHCl3/(CH3)2SiHCl with MoCl5
3.4.1 Chain initiation reaction. The calculation process was the same as that in the previous section. By comparing the bond energies of Si–H bonds and Mo–Cl bonds in Tables 3 and 7, we can also divide the chain reaction into two reaction paths. In the processes involving MoCl5 and MoCl4 reactions with SiHCl3 and MoCl5, MoCl4, MoCl3 and MoCl reactions with (CH3)2SiHCl, Mo–Cl bond cleavage serves as the chain initiation reaction (eqn (19)). In the processes involving MoCl3, MoCl2, and MoCl reactions with SiHCl3 and MoCl2 reaction with (CH3)2SiHCl, chain initiation involves cleavage of the Si–H bonds (eqn (6) and (7)). |
MoClx = MoClx−1 + Cl·
| (19) |
Table 7 Dissociation energies of Mo–Cl in MoClx
Compound |
Parameter |
DE/kJ mol−1 |
MoCl5 |
Mo–Cl |
126.5 |
MoCl4 |
|
281.5 |
MoCl3 |
|
336.5 |
MoCl2 |
|
347.2 |
MoCl |
|
341.3 |
3.4.2 Chain propagation reaction. When the chain initiation reaction involves the release of Cl· from molybdenum chloride, chlorine atoms capture the hydrogen on the silicon to generate
or (CH3)2SiCl· (eqn (8) and (10)). When the chain initiation reaction involves cleavage of Si–H bonds,
or (CH3)2SiCl· is formed. The
or (CH3)2SiCl· generated in the two reactions reacts with MoClx (eqn (20) and (21)). The relative energies for the reactions of SiHCl3 and (CH3)2SiHCl with MoClx are shown in Fig. 9 and 10. The structures of the transition states involved in these reactions are shown in Fig. 11. |
 | (20) |
|
MoClx + (CH3)2SiCl· = (CH3)2SiCl2 + MoClx−1
| (21) |
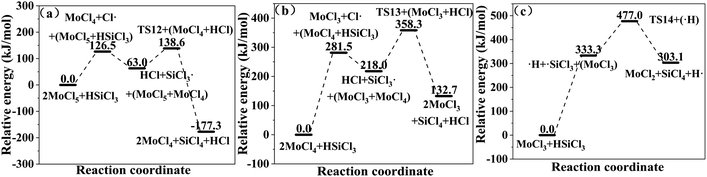 |
| Fig. 9 (a–c) Relative energies for the reactions of SiHCl3 with MoClx. | |
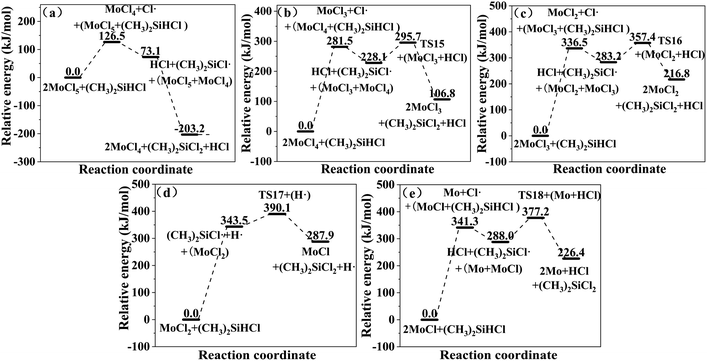 |
| Fig. 10 (a–e) Relative energies for the reactions of (CH3)2SiHCl with MoClx. | |
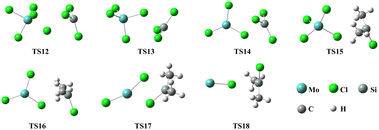 |
| Fig. 11 Structures of transition states during the reactions of SiHCl3/(CH3)2SiHCl with MoCl5. | |
The main difference between the reactions of MoClx with SiHCl3 and (CH3)2SiHCl lies in the energy barriers for the reactions of ·SiCl3 and ·(CH3)2SiCl with MoClx. Fig. 9 and 10 show the energy barriers and Gibbs free energy changes for MoClx reactions with
and (CH3)2SiCl·, and these are summarized in Table 8. The energy barriers for the reactions of MoCl5, MoCl4, and MoCl3 with
are higher than those for the (CH3)2SiCl· reactions, and the differences are 75.6 kJ mol−1, 72.7 kJ mol−1, and 69.3 kJ mol−1, respectively. The ΔG values for the MoCl5, MoCl4, MoCl3, MoCl2 and MoCl reactions with
are all larger than the ΔG values for the corresponding (CH3)2SiCl· reactions. The above results reveal that MoClx reacts more easily with (CH3)2SiHCl. In addition, the energy barrier difference between the reactions of MoCl5 with SiHCl3 and (CH3)2SiHCl and the reactions of WCl6 can be explained by the presence of only five Cl atoms in MoCl5. WCl6 is more conducive the chlorination of (CH3)2SiHCl in SiHCl3. Obviously, the calculated results are in good agreement with the experimental results.
Table 8 Energy barriers and Gibbs free energy changes for MoClx reactions with
and (CH3)2SiCl·
Reactant |
EB (kJ mol−1) |
ΔG (kJ mol−1) |
MoCl5 |
·SiCl3 |
75.6 |
−240.3 |
|
·(CH3)2SiCl |
0 |
−276.3 |
MoCl4 |
·SiCl3 |
140.3 |
−85.3 |
|
·(CH3)2SiCl |
67.6 |
−121.3 |
MoCl3 |
·SiCl3 |
143.6 |
−30.3 |
|
·(CH3)2SiCl |
74.3 |
−66.4 |
MoCl2 |
·SiCl3 |
— |
−19.6 |
|
·(CH3)2SiCl |
46.6 |
−55.6 |
MoCl |
·SiCl3 |
— |
−25.5 |
|
·(CH3)2SiCl |
89.2 |
−61.6 |
3.4.3 Chain termination reaction. In the same way, in the SiHCl3 reactions with MoCl5 and MoCl4 and the (CH3)2SiHCl reactions with MoCl5, MoCl4, MoCl3 and MoCl, chain termination occurs via a combination of
and (CH3)2SiCl· reactions with Cl· (eqn (12) and (13)). In the processes for SiHCl3 reactions with MoCl3, MoCl2 and MoCl and (CH3)2SiHCl reaction with MoCl2, chain termination occurs via the combination of two hydrogen atoms (eqn (14)).Therefore, the overall equation for the reactions of SiHCl3 with MoCl5 and MoCl4 is shown as eqn (22), and the overall equation for the reactions with MoCl3, MoCl2 and MoCl is shown as eqn (23). The overall equation of the reactions of (CH3)2SiHCl with MoCl5, MoCl4, MoCl3 and MoCl is shown as eqn (24), and the overall equation of the reaction with MoCl2 is shown as eqn (25).
|
HSiCl3 + 2MoClx = SiCl4 + 2MoClx−1 + HCl
| (22) |
|
2HSiCl3 + 2MoClx = 2SiCl4 + 2MoClx−1 + H2
| (23) |
|
(CH3)2SiHCl + 2MoClx = (CH3)2SiCl2 + 2MoClx−1 + HCl
| (24) |
|
2(CH3)2SiHCl + 2MoClx = 2(CH3)2SiCl2 + 2MoClx−1 + H2
| (25) |
4. Conclusions
Chlorination reactions of SiHCl3/(CH3)2SiHCl were carried out with two metal chlorides (WCl6 and MoCl5) as the chlorine sources. The conversion rates for (CH3)2SiHCl and SiHCl3 both increased with increases in the reactant ratio, temperature and time. The conversions for the reactions of (CH3)2SiHCl with WCl6/MoCl5 were much higher than those of SiHCl3. Furthermore, the use of WCl6 as the chlorine source showed higher conversion of the (CH3)2SiHCl than MoCl5. The optimum conditions for the reaction of WCl6 with (CH3)2SiHCl were as follows: a reaction pressure of 0.8 MPa, a reaction temperature of 60 °C, a reaction time of 60 min and n(WCl6)
:
n(SiHCl3 or (CH3)2SiHCl) = 0.7. The conversion rate for (CH3)2SiHCl was 22.7 times that for SiHCl3 in the reactions of SiHCl3/(CH3)2SiHCl with WCl6.
The mechanisms for the reactions of SiHCl3/(CH3)2SiHCl with WCl6/MoCl5 were explored in detail with density functional theory calculations. The differences in the reactions of SiHCl3 and (CH3)2SiHCl with WCl6 or MoCl5 were found to lie in the energy barriers of the
and (CH3)2SiCl· reactions with WClx/MoClx. The energy barriers for the reactions of WClx (3 ≤ x ≤ 6) with
were higher than those for the (CH3)2SiCl· reaction. The same is true of MoClx (3 ≤ x ≤ 5). On the whole, the energy barrier differences for WCl6 reactions with SiHCl3 and (CH3)2SiHCl were higher than those for MoCl5 reactions with SiHCl3 and (CH3)2SiHCl. The experimental results were in good agreement with the calculation results. (CH3)2SiHCl is converted to (CH3)2SiCl2 in a chlorination reaction, which is conducive to the removal of carbonaceous impurities from SiHCl3 by distillation in the improved Siemens method.
Conflicts of interest
There are no conflicts to declare.
Acknowledgements
This work was financially supported by the Major Science and Technology Project of Xinjiang Bingtuan (No. 2017AA007, 2020AA004) and the Major Science and Technology Project of Shihezi (No. 2020ZD02).
References
- F. Chigondo, From Metallurgical-Grade to Solar-Grade Silicon: An Overview, Silicon, 2017, 10, 789–798 CrossRef.
- J. Gong, C. Li and M. R. Wasielewski, Advances in solar energy conversion, Chem. Soc. Rev., 2019, 48, 1862–1864 RSC.
- N. Zhang, Q. Zheng, Y. Wang, D. Wang, W. Peng, J. Zhang, J. Zhang and J. Liu, Theoretical study on the reaction mechanism of Si2Cl6 and HCl catalyzed by amine catalysts, New J. Chem., 2022, 46, 17977–17984 RSC.
- Q. Geng and G. Huang, Catalytic conversion of carbon-containing impurity methyldichlorosilane to purify raw material trichlorosilane of polysilicon production, React. Chem. Eng., 2022, 2022, 1544–1554 RSC.
- S. Ranjan, S. Balaji, R. A. Panella and B. E. Ydstie, Silicon solar cell production, Comput. Chem. Eng., 2011, 35, 1439–1453 CrossRef CAS.
- G. Huang and M. Zhang, Reactive distillation purification method and device for removing carbon-containing impurities from chlorosilane, CN Pat., CN110980742A, 2020.
- H. Wang, J. Chen, C. Hua and Y. Liu, Device and method for preparing high-purity trichlorosilane by adsorption of methyl chlorosilane impurities, CN Pat., CN109205627A, 2018.
- Y. Wan, J. Xiao, D. Yan and J. Liu, Removal of methyldichlorosilane from trichlorosilane via photochemical chlorination, Jingxi Huagong, 2020, 37, 201–206 Search PubMed.
- M. Zhang and G. Huang, Conversion reaction process of methyldichlorosilane in chlorosilane, Jingxi Huagong, 2020, 37, 758–764 Search PubMed.
- M. Ishida, S. Hiroshi and H. Masyuki, Method for producing trichlorosilane, JP Pat., JP2014152093A, 2013.
- H. Wang, J. Chen, C. Hua, Y. Liu and F. Li, Device and method for removing methyldichlorosilane from trichlorosilane by means of reactive distillation, WIPO Pat., WO2020103799A1, 2020.
- K. Chulsky and R. Dobrovetsky, B(C6F5)3-Catalyzed Selective Chlorination of Hydrosilanes, Angew. Chem., Int. Ed., 2017, 56, 4744–4748 CrossRef CAS PubMed.
- Y. Fu, Y. Jiang, H. Yin, L. Shen, Y. Feng and A. Wang, Catalytic chlorination of methylphenyldichlorosilane with gaseous chlorine to chlorinated methylphenyldichlorosilanes over Lewis acids, J. Ind. Eng. Chem., 2014, 20, 1022–1029 CrossRef CAS.
- H. Firouzabadi, N. Iranpoor and B. Karimi, Tungsten Hexachloride (WCl6) in the Presence of Dimethylsulfoxide Promoted Facile and Efficient One-Pot Ring Expansion-Chlorination Reactions of 1,3-Dithiolanes and 1,3-Dithianes, Synlett, 1999, 1999, 413–414 CrossRef.
- B. Boualy, L. E. Firdoussi, M. A. Ali and A. Karim, Allylic chlorination of terpenic olefins using a combination of MoCl5 and NaOCl, J. Braz. Chem. Soc., 2011, 22, 1259–1262 CrossRef CAS.
- A. A. Mekkaoui, M. Laayati, H. Orfi, L. El Firdoussi and S. El Houssame, Catalytic Allylic Chlorination of Natural Terpenic Olefins Using Supported and Nonsupported Lewis Acid Catalysts, J. Chem., 2020, 2020 Search PubMed.
- S. Huo, H. Chen and W. Zuo, Selective Chlorination of Methane Photochemically Mediated by Ferric Chloride at Ambient Temperature, Chin. J. Org. Chem., 2021, 41, 1683–1690 CrossRef CAS.
- M. Frisch, G. Trucks, H. Schlegel, G. Scuseria, et al., Gaussian 16 Revision C. 01, Gaussian, Inc., Wallingford CT, 2016 Search PubMed.
- C. Gonzalez and H. B. Schlegel, Reaction path following in mass-weighted internal coordinates, J. Phys. Chem., 1990, 94, 5523–5527 CrossRef CAS.
- R. V. G. Ewens and M. W. Lister, The structures of molybdenum pentachloride and tungsten hexachloride, Trans. Faraday Soc., 1938, 34, 1358–1362 RSC.
- Y. Kawashima, The rotational spectrum of chlorodimethylsilane using Fourier transform microwave spectroscopy, J. Mol. Struct., 2001, 563–564, 227–230 CrossRef CAS.
- H. Takeo and C. Matsumura, The Microwave Spectra, Molecular Structures, and Quadrupole Coupling Constants of Methyltrichlorosilane and Trichlorosilane, Bull. Chem. Soc. Jpn., 1977, 50, 1633–1634 CrossRef CAS.
- J. D. DeSain, L. Valachovic, L. E. Jusinski and C. A. Taatjes, Reaction of chlorine atom with trichlorosilane from 296 to 473 K, J. Chem. Phys., 2006, 125, 224308 CrossRef PubMed.
|
This journal is © The Royal Society of Chemistry 2023 |
Click here to see how this site uses Cookies. View our privacy policy here.