DOI:
10.1039/D3RA00251A
(Paper)
RSC Adv., 2023,
13, 4754-4756
Synthesis of warfarin analogs: conjugate addition reactions of alkenyl-substituted N-heterocycles with 4-hydroxycoumarin and related substrates†
Received
13th January 2023
, Accepted 20th January 2023
First published on 6th February 2023
Abstract
We have developed a procedure for the Michael addition of 4-hydroxycoumarins to vinyl-substituted N-heterocycles. The chemistry is also suitable for thiocoumarins and quinolinones. A mechanism is proposed involving nucleophilic attack at the vinyl-group of the protonated N-heterocycle.
Introduction
Warfarin (1) is a clinically important anticoagulant drug.1 It was first approved for use in the mid-1950s and warfarin is currently listed on the World Health Organization's List of Essential Medicines.2 The substance is commonly prepared using a base-catalyzed reaction of 4-hydroxycoumarin (2) with benzalacetone (eqn (1)).3 Enantioselective addition reactions have also been developed.4 Our group recently described the Michael addition reactions of 1,3-dicarbonyl compounds with vinyl-substituted N-heterocycles (eqn (2)).5 Based on this chemistry, we hypothesized that 4-hydroxycoumarins would exhibit similar nucleophilic reactivity with vinyl-substituted N-heterocycles. In the following Communication, we describe a convenient method for the synthesis of heterocycle-containing analogs of warfarin. |
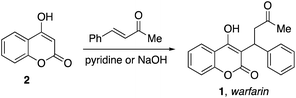 | (1) |
|
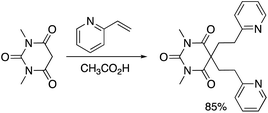 | (2) |
Results and discussion
Using our previous methodology,5 4-hydroxycoumarin was reacted with 2-vinylpyridine and acetic acid in acetonitrile and the addition product 3 was isolated in 69% yield (Table 1). The product was exceptionally difficult to purify using chromatography, so a methodology was developed with crystalizing the product directly from the crude product mixture. Similar addition products (3–8) were prepared from substituted 4-hydroxycoumarins, including halogen, alkyl, and the methoxy-substituted systems. A modest yield of product 9 was obtained from 5-nitro-2-vinylpyridine and 4-hydroxycoumarin. The conversions were also accomplished with 4-vinylpyridine, providing compounds 10–14 in fair to good yields. The lower yields seem be associated with systems that did not crystalize well from the crude product mixtures. For example, 4-hydroxycoumarin reacted with di-(4-pyridyl)ethylene but inefficient crystallization provided only a 39% isolated yield of compound 15. Additionally, we found some systems slowly formed products from double addition reactions. These minor biproducts were identified from mass spectral analysis and NMR analysis of crude product mixtures. The data suggests C- and O-alkylation products (i.e. 16). To suppress formation of these biproducts, some of the conversions were best conducted with equimolar ratios of the vinylpyridine and 4-hydroxycoumarin.
Table 1 Products and yields from the reactions of 4-hydroxycoumarins with vinylpyridines
Over the past 50 years, several types of vinyl-substituted heterocycles have been shown to be reactive as Michael acceptors.6 We have found that 4-hydroxycoumarin also reacts with other types of olefinic heterocycles. When 4-hydroxycoumarin is reacted with a vinyl-substituted 1,2,4-oxadiazole, product 17 is obtained, albeit in low isolated yield (Scheme 1, eqn (3)). Likewise, vinylpyrazine gives the adduct 18 in 39% yield from a reaction with 4-hydroxycuomarin (eqn (4)). The chemistry is also compatible with closely related nucleophiles. Thus, 4-hydroxyquinolin-2(1H)-one reacts with 2- and 4-vinylpyridine to give products 19–20 in fair yields (eqn (5) and (6)). Similarly, the brominated 4-hydroxyquinolin-2(1H)-one gives compound 21 from 2-vinylpyridine (eqn (7)). The adduct (22) from 4-hydroxy-2H-thiochromen-2-one is also formed in fair yield from 2-vinylpyrdine (eqn (8)).
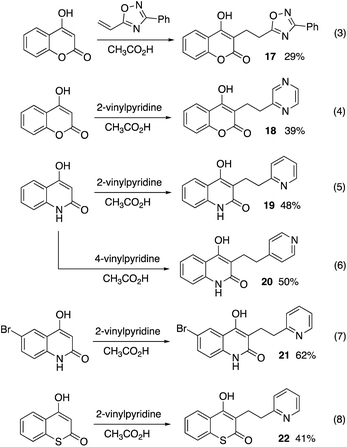 |
| Scheme 1 Addition reactions with varied heterocycles. | |
As acid-promoted addition reactions, it is suggested that the acid protonates the N-heterocycle and enhances the electrophilic reactivity of the vinyl group (Scheme 2). We propose a mechanism involving nucleophilic attack of the enol group at the electrophilic vinyl group. As the enol transfers electron density into the vinyl group, negative charge accumulates at the α-carbon. This leads to a simultaneous proton transfer to the α-carbon – giving intermediate 23 which rapidly isomerizes to the pyridinium salt of the observed product. Working from the proposed mechanism, we sought to determine if other electrophiles or groups could be transferred to the α-carbon, besides a simple proton. Compounds 24 were prepared, but unfortunately neither the acetyl or allyl groups were observed to migrate and give products 25.
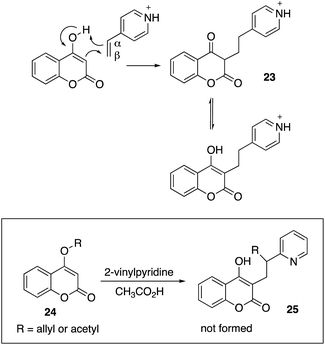 |
| Scheme 2 Proposed mechanism for the addition reaction and an unsuccessful application of the chemistry. | |
Conclusions
In summary, we have found that 4-hydroxycuomarins react with vinyl-substituted N-heterocycles using an acid promoter. The enol groups of 4-hydroxycuomarins are sufficiently nucleophilic to undergo Michael additions to vinyl-substituted pyridines, pyrazine, and 1,2,4-oxadiazole. Similar reactivity has been demonstrated with 4-hydroxyquinolin-2(1H)-one and 4-hydroxy-2H-thiochromen-2-one. This work and other recent studies further demonstrates the utility of Michael addition as a useful route to functionalized heterocycles.7
Author contributions
The experimental work was carried out by B. G. and the conceptual work was done by D. A. K.
Conflicts of interest
There are no conflicts to declare.
Acknowledgements
The support of the NIGMS-NIH (1R15GM126498-01) is gratefully acknowledged. We also acknowledge the generous support from the NSF MRI program (award no. CHE-1726931) for the purchase of a high-resolution mass spectrometer and an NMR instrument (award no. CHE-2117776). We thank Northern Illinois University for the support of the Molecular Analysis Core Facility.
Notes and references
- S. S. Garg, J. Gupta, S. Sharma and D. Sahu, Eur. J. Pharm. Sci., 2020, 152, 105424 CrossRef CAS PubMed.
- D. Wardrop and D. Keeling, Br. J. Haematol., 2008, 141, 757 CrossRef PubMed; World Health Organization Model List of Essential Medicines, 22nd List, 2021, p. 34, https://www.who.int/publications/i/item/WHO-MHP-HPS-EML-2021.02 Search PubMed.
- A. M. Rayar, N. Lagarde, F. Martin, F. Blanchard, B. Liagre, C. Ferroud, J.-F. Zagury, M. Montes and M. Sylla-Iyarreta Veitia, Eur. J. Med. Chem., 2018, 146, 577 CrossRef CAS PubMed.
- N. Halland, T. Hansen and K. A. Jorgensen, Angew. Chem., Int. Ed., 2003, 42, 4955 CrossRef CAS PubMed; J. Dong and D.-M. Du, Org. Biomol. Chem., 2012, 10, 8125 RSC; N. M. Moreira, L. S. R. Martelli and A. G. Correa Beil, J. Org. Chem., 2021, 17, 1952 Search PubMed.
- J. C. Hood, Y. Tshikaya, A. R. Manz, M. C. LaPorte and D. A. Klumpp, J. Org. Chem., 2022, 87, 4908 CrossRef CAS PubMed.
- D. A. Klumpp, Synlett, 2012, 23, 1590 CrossRef CAS.
- B. Mao, Z.-W. Chen, J.-F. Wang, C.-H. Zhang, Z.-Q. Du and C.-M. Yu, Org. Lett., 2022, 24, 6588 CrossRef CAS PubMed; W.-D. Chu, Y.-T. Wang, T.-T. Liang, T. Long, J.-Y. Zuo, Z. Shao, B. Chen, C.-Y. He and Q.-Z. Liu, Org. Lett., 2022, 24, 3965 CrossRef PubMed; K. Jung, S. Kim, S. Choi, I. Kim and G. Han, J. Org. Chem., 2021, 86, 16785 CrossRef PubMed; J. Han, J. Kim, J. Lee, Y. Kim and S. Y. Lee, J. Org. Chem., 2020, 85, 15476 CrossRef PubMed; M. Stentzel and D. A. Klumpp, J. Org. Chem., 2020, 85, 12740 CrossRef PubMed; Z. Zhang, S. Dai, L. Li, C. Jia, Y. Zhang and H. Li, Tetrahedron Lett., 2020, 61, 151926 CrossRef; Y.-L. Zeng, B. Chen, Y. T. Wang, C.-Y. He, Z.-Y. Mu, J.-Y. Du, L. He, W.-D. Chu and Q.-Z. Liu, Chem. Commun., 2020, 56, 1693 RSC; F. Buttard, C. Bertonneau, M.-A. Hiebel, J.-F. Briere and F. Suzenet, J. Org. Chem., 2019, 84, 3702 CrossRef PubMed; R. P. Jumde, F. Lanza, T. Pellegrini and S. R. Harutyunyan, Nat. Commun., 2017, 8, 1 CrossRef PubMed; S. H. Kennedy and D. A. Klumpp, J. Org. Chem., 2017, 82, 10219 CrossRef PubMed; R. P. Jumde, F. Lanza, M. J. Veenstra and S. R. Harutyunyan, Science, 2016, 352, 433 CrossRef; Y.-Y. Wang, K. Kanomata, T. Korenaga and M. Terada, Angew. Chem., Int. Ed. Engl., 2016, 55, 927 CrossRef PubMed; S. Wang, X. Li, H. Liu, L. Xu, J. Zhuang, J. Li, H. Li and W. Wang, J. Am. Chem. Soc., 2015, 137, 2303 CrossRef.
|
This journal is © The Royal Society of Chemistry 2023 |
Click here to see how this site uses Cookies. View our privacy policy here.