DOI:
10.1039/D2RA07458C
(Paper)
RSC Adv., 2023,
13, 3306-3316
Effect of germination pretreatment on the physicochemical properties and lipid concomitants of flaxseed oil
Received
23rd November 2022
, Accepted 16th January 2023
First published on 23rd January 2023
Abstract
This study investigated the effects of germination pretreatment on the physicochemical properties, lipid concomitants, and antioxidant activity of flaxseed oil in three varieties. The results indicated that the oil content of flaxseed decreased by 2.29–7.40% during the 5 days germination period. Germinated flaxseed oil showed a significantly higher acid value and lower peroxide value. The unsaturated fatty acid content was slightly increased by germination. Germination pretreatment resulted in significant increases in the α-tocopherol, stigmasterol, pigments, total phenols, and antioxidant activity. As germination time progressed to 5 days, α-tocopherol which was traditionally recognized as having the highest antioxidant activity form of vitamin E in humans increased from 3.07–6.82 mg kg−1 to 258.11–389.78 mg kg−1. Germinated oil had 1.63 to 2.05 times higher stigmasterol content than non-germinated oil. The chlorophyll and carotenoid also increased exponentially. The total phenol content of flaxseed oil increased from 64.29–75.85 mg kg−1 to 236.30–297.78 mg kg−1. Germinated flaxseed oil showed important antioxidant activity. Compared with other varieties during germination, the oil from Gansu showed a higher level of α-linolenic acid, tocopherols, and carotenoid, and a maximum increase level of tocopherols and phytosterols. The comprehensive evaluation of germination time by correlation and principal component analysis showed that when germination time exceeded 2 days, the lipid concomitants and antioxidant capacity of flaxseed oil were significantly improved.
1. Introduction
Flaxseed (Linum usitatissimum L.), also known as linseed, has been used for food and textile fibers for over 5000 years. Flaxseed is a functional food ingredient with high nutritional value and potential for exploitation. The oil content of flaxseed ranges from 40% to 50%.1 Flaxseed oil contains more unsaturated fatty acids (approximately 90%) than saturated fatty acids and contains high amounts of α-linolenic acid, ranging from 45% to 60%.2 α-Linolenic acid is an essential fatty acid that can not be synthesized in the human body and must be taken from food; it is also one of the active ingredients in flaxseed oil for its health functions, which is beneficial for infant brain development, blood lipid reduction, and cardiovascular disease.3 In addition, other trace active components in flaxseed oil, such as tocopherols, phytosterols, and phenolic compounds, have also been identified.4 When used daily, flaxseed oil promotes the health of the body due to the bioactivity and functions of its α-linolenic acid and active components.5,6
Numerous reports of the pretreatment of seeds using various methods to improve the nutritional content and quality of oils have emerged. These methods include infrared,7 microwave,8 germination,9 and superheated steam.10 Among various seed pretreatment methods, germination is considered a relatively economical and effective technology to be an important means of enhancing the nutritional value properties of plant seeds at a low cost.9 The germination of plant seeds often leads to the synthesis of bioactive phytochemicals from scratch, which protects plants from various exogenous challenges and could be considered health-promoting ingredients.11 As a result, germinated grains have attracted much attention for their greater nutritious benefits compared with seeds.12 Several studies have shown that germination pretreatment has a positive influence on the quality properties of plant seeds. Nutrients, such as vitamins, minerals, and limiting amino acids, increase during germination.13 Furthermore, a large range of plant seeds, such as sorghum,14 mustard grains,15 quinoa,16 and flaxseed,17 which release bound phenolic compounds because of the degradation of the plant cell wall after germination, had a high content of total phenolic. Furthermore, germination-treated seeds had outstanding oxidation resistance.15,18 Additionally, germination pretreatment of oilseeds before pressing could remarkably increase the content of chlorophyll and carotenoid in flaxseed oil.19 Phytosterol and tocopherol content increased significantly in germinated canola and its oil, and the total phenolic acid content decreased in germinated flaxseed oil;19,20 these results contradicted what happened in germinated sesame seeds in a previous study.21 Germination pretreatment of different oilseeds produced different effects on itself and their extracted oil. Germination had a positive effect on lignan biosynthesis and the decrease in cyanogenic glucosides in flaxseed.13,17 However, there were few studies investigating the effects of germination on lipid concomitants and oil quality properties. Whether germination can be utilized as an effective method to improve the nutritional value of flaxseed oil in the same way as plant seeds have not been established.
The present study was conducted to investigate the changes in physicochemical properties, the content of typical lipid concomitants, and the antioxidant activity of oils in three flaxseed varieties during a 5 days germination process. A comprehensive evaluation of flaxseed oil at different germination times was conducted by correlation analysis and principal component analysis to investigate the germination characteristics of flaxseed oil at different stages, thereby providing a theoretical basis for the functional and high-value processing and utilization of flaxseed oil.
2. Materials and methods
2.1 Materials and reagents
Three flaxseed varieties were collected from different areas. These areas included the Inner Mongolia Autonomous Region, Gansu Province in China, and Kazakhstan, which are important production areas of flaxseed. For simplicity, we named these flaxseed varieties as IM, GS, and KZ, respectively. Dust, impurities, and broken seeds were removed manually before the experiment.
2.2 Germination conditions
The flaxseed samples were germinated in germination buckets and kept in the dark at 25 °C and 80% relative humidity for 5 days. The process started with a uniform spray of 30% water to be absorbed by the flaxseed (about 3 h), and then the seeds were sprayed every, 6 h; the amount of water sprayed should be moderate to ensure that the flaxseed absorbs water, and the surface is not sticky. Samples were collected every day during the 5 days germination. The germinated flaxseeds were dried in a dryer at 60 °C and packed in air-tight bags for further analysis.
2.3 Preparation of flaxseed oil
The dried germinated flaxseed was roasted at 160 °C for 20 min. The temperature and time were selected on the basis of commercial processes and designed to produce samples with acceptable flavor and color. After roasting, the flaxseeds were allowed to cool to room temperature and were placed in a low-temperature press (oil extraction temperature < 60 °C) to collect oil samples. The crude oil was centrifuged at 6000 rpm to remove other solid particles. The obtained pressed oil was sealed and stored at 4 °C.
2.4 Moisture content measurement
The moisture content of flaxseed was measured in an oven at 105 °C until a constant weight was obtained.
2.5 Oil content measurement
Samples were analyzed for crude fat content by the Soxhlet method.22 All values were expressed as g/100 g flaxseed (dry basis).
2.6 Color measurement
The change of color during the germination of three flaxseed oils was determined by measuring the L*, a*, and b* color scales using a Hunter Lab colorimeter (CR-400, Konica Minolta Sensing Inc., Osaka, Japan).
2.7 Determination of chemical composition
2.7.1 Determination of acid value and peroxide value. The acid value and peroxide value were quantified by the AOCS official method Cd 3a-63 and Cd 8b-90, respectively.23,24
2.7.2 Determination of fatty acids. Gas chromatography (GC 6890 N, Agilent Technologies, Palo Alto, CA) equipped with an HP-INNOWAX capillary column (0.25 μm, 30.0 m × 0.25 mm), and a flame ionization detector was applied to measure the fatty acid composition. The heating procedure was performed in accordance with Zhang et al.25 The details were as follows: the initial temperature of the column was 60 °C, which was increased to 200 °C at a rate of 10 °C min−1, and to 240 °C at a rate of 8 °C min−1; the temperature was maintained for 15 min; injection volume was 1.0 μL, injector temperature was 250 °C, and detector temperature was 250 °C.
2.7.3 Determination of tocopherols. The method reported by Huang et al.8 was modified to determine the content of tocopherols of flaxseed oil. The samples were injected into a liquid chromatography system (Shimadzu, Japan) equipped with a PDA detector and an Athena Silica column (250 mm × 4.6 mm, 5 μm, CNW, Inc., GER). The injection volume was 10 μL. The mobile phase was hexane: isopropanol (98.5
:
1.5, v/v) with a flow rate of 1 mL min−1. Tocopherols were detected at 295 nm.
2.7.4 Determination of phytosterols. Phytosterol content was determined using a gas chromatography-mass spectrum system (Thermo Fisher, USA) equipped with an FID, as described in Jin et al. with some modifications.26 In brief, 1 μL of the prepared sample was injected into the gas chromatograph and separated by an SH-Rxi-5Sil MS capillary column (30 m × 0.25 mm × 0.25 μm, Shimadzu, Japan). The initial column temperature was set at 180 °C for 1.0 min, then increased to 300 °C at a rate of 4 °C min−1 and maintained for 15 min. The injector temperature was 290 °C. Carrier gas (helium) was injected at 0.7 mL min−1 and the split ratio was 1
:
80. In addition, the ion source temperature was 250 °C. The internal standard method is used for quantification.
2.7.5 Determination of chlorophyll and carotenoid. The chlorophyll and carotenoid content were determined in accordance with a previously described method with some modifications.19 Briefly, a 2 g sample of germinated flaxseed oil was fully dissolved in 25 mL cyclohexane. The maximum absorption at 670 and 470 nm is related to the chlorophyll fraction and carotenoid fraction, respectively.Thus, the pigment contents were calculated as follows:
Chlorophyll (mg kg−1) = (A670 × 106) / (613 × 100 × d), |
Carotenoid (mg kg−1) = (A470 × 106) / (2, 000 × 100 × d), |
where
A is the absorbance, and
d is the spectrophotometer cell thickness (1 cm).
2.7.6 Determination of total phenolic. The extracts from flaxseed oil for analyzing phenolic content were prepared in accordance with Yang et al. using the Folin–Ciocalteu colorimetric method.27 About 2 mL of Folin–Ciocalteu phenol reagent was added to 2 mL of extracts, which react in the dark for 5 min. Later, 2 mL of 7.5% sodium carbonate solutions were added. After 2 h of dark reaction, absorbance was measured at 760 nm. The results were expressed as mg of gallic acid equivalents (GAE) per kg of oil (mg GAE kg−1).
2.7.7 Determination of antioxidant activity. The antioxidant activity of flaxseed oil was evaluated by 1,1-diphenyl-2-picrylhydrazyl (DPPH) radical scavenging activity and 2,2-azino-bis (3-ethylbenz-thiazoline-6-sulfonic acid) (ABTS) radical cation scavenging activity. The DPPH radical scavenging activity was determined in accordance with the previously described method with some modifications.28 About 0.6 mL of the extracts were added to the DPPH methanol solution (3 mL). The mixture was shaken vigorously and left in the dark for 30 min. Absorbance was measured at 517 nm. All results were expressed as μmol of Trolox Equivalents (TE) per kg of oil (μmol TE kg−1).The ABTS radical cation scavenging activity was determined in accordance with the previously described method with some modifications.29 About 0.2 mL of the extracts were added to the ABTS solution (3 mL), allowing the mixture to stand in the dark at room temperature for 6 min. The absorbance reading was taken at 734 nm. All values were expressed as μmol of Trolox equivalents (TE) per kg of oil (μmol TE kg−1).
2.8 Statistical analyses
SPSS 25.0 statistical software (IBM SPSS Inc., IL, USA) was used to perform analysis of variance (ANOVA) on the obtained data. All the analyses were repeated in triplicate, and the values were expressed as mean ± standard deviation. Origin 2019 (OriginLab Corp., MA, USA) was carried out to chart.
3. Results and discussion
3.1 Moisture and oil content of flaxseed
Table 1 presents the moisture and oil content of flaxseed. The moisture and oil content of non-germinated flaxseed were 5.13–5.61% and 43.90–44.79%, respectively. Germination pretreatment significantly influenced the moisture and oil content of flaxseed (P < 0.05). The moisture content of three varieties of flaxseed increased significantly to 71.67–82.43% after 5 days germination, which was related to the continuous absorption of water by the flaxseed to achieve the development of the seed embryo growing out of the germ.13 The changes in moisture content in germinated flaxseed exhibited a quick-to-slow tendency during germination.
Table 1 Effect of germination of flaxseed on seed moisture and oil contenta,b
Flaxseed varieties |
Germination time (day) |
Moisture (%) |
Oil content (g 100 g−1) |
Results are means ± SD of triplicate determinations. Column values in the same flaxseed variety with different lowercased letters are significantly different (P < 0.05). |
IM |
0 |
5.61 ± 0.09f |
44.75 ± 0.13b |
1 |
43.48 ± 0.55e |
45.95 ± 0.10a |
2 |
55.93 ± 0.34d |
44.51 ± 0.16b |
3 |
71.51 ± 0.52c |
42.90 ± 0.02c |
4 |
77.42 ± 0.46b |
40.10 ± 0.15d |
5 |
82.43 ± 0.37a |
37.35 ± 0.40e |
GS |
0 |
5.31 ± 0.06f |
44.79 ± 0.24 ab |
1 |
44.44 ± 0.52e |
44.88 ± 0.06a |
2 |
55.94 ± 0.49d |
44.36 ± 0.28b |
3 |
63.94 ± 0.76c |
43.17 ± 0.04c |
4 |
69.12 ± 0.04b |
41.01 ± 0.49d |
5 |
71.67 ± 1.36a |
39.28 ± 0.17e |
KZ |
0 |
5.13 ± 0.06f |
43.90 ± 0.13b |
1 |
42.98 ± 1.17e |
43.67 ± 0.08b |
2 |
57.21 ± 0.55d |
44.27 ± 0.33a |
3 |
60.93 ± 1.32c |
42.92 ± 0.06c |
4 |
66.70 ± 0.37b |
42.24 ± 0.10d |
5 |
73.01 ± 0.55a |
41.61 ± 0.04e |
The oil content of flaxseed during germination showed a general trend of increasing and then decreasing. The oil content of flaxseed of IM and KZ increased significantly to the maximum on day 1 and day 2 of germination, respectively, with IM increasing by 1.2% and KZ by 0.37%. The oil content of flaxseed of GS increased slightly on day 1 but did not reach a significant difference. Other studies with pulses also found that oil content significantly increased with germination.30,31 The elevated oil content in the early stages of germination may be due to the rapid water absorption and swelling of flaxseed at this stage, with stored fat moving from the storage tissue toward the embryo and thus aggregating, and the detachment of protein-bound fat from the tissue, leading to increased oil extraction after germination.32 The oil content of flaxseed in IM, GS, and KZ decreased to 37.35%, 39.28%, and 41.61%, decreased by 7.40%, 5.51%, and 2.29%, respectively during the 5 days germination period. This result corresponded to the study of Li et al.,13 who reported that the oil content of flaxseed significantly reduced with germination. The degradation of reserve nutrients, such as oil, during germination, is to provide the energy required for protein synthesis in plant growth.19,33
3.2 Acid value, peroxide value, and color of flaxseed oil
The acid value is a crucial index to appraise the quality of oils. Our results revealed that the acid value of flaxseed oil was affected by germination pretreatment significantly (P < 0.05) (Table 2). The acid value of flaxseed oil dropped first and then rose with germination in GS and KZ, with rising in IM. The acid value in GS and KZ decreased from 1.24 and 1.59 mg KOH g−1 oil to 0.85 and 1.25 mg KOH g−1 oil on day 1 of germination, respectively. The decrease in acid value may be caused by the oxidation of the free fatty acids of flaxseed to hydroperoxides and small molecules of aldehydes and ketones during the initial germination process.34 With the deepening of germination, the increase in fat hydrolase activity, and the moisture content of flaxseed, some phospholipids, and triglyceride hydrolyzed to free fatty acids, which undoubtedly led to the increase in acid value.35,36 When germinated for 5 days, the acid value of flaxseed oil reached the maximum value (9.06, 7.07, and 20.54 mg KOH g−1 oil) in IM, GS, and KZ. Among the three varieties, the flaxseed oil in GS had the lowest acid value during germination, which may be related to low water uptake and low enzyme activity.19 Herchi et al.19 found that the acid value of flaxseed oil improved significantly during germination. The results of previous studies were slightly different from our study, which may be due to the large germination amount of flaxseed and the different varieties.
Table 2 Effect of germination of flaxseed on acid value, peroxide value, and color of flaxseed oila,b
Flaxseed varieties |
Germination time (day) |
Acid value (mg KOH g−1 oil) |
Peroxide value (meq O2 kg−1 oil) |
Color |
L* |
a* |
b* |
ΔE |
Results are means ± SD of triplicate determinations. Column values in the same flaxseed variety with different lowercased letters are significantly different (P < 0.05). |
IM |
0 |
0.6 ± 0.01e |
3.93 ± 0.24a |
80.08 ± 0.01b |
8.22 ± 0.01e |
117.10 ± 0.11b |
— |
1 |
0.7 ± 0.00e |
1.36 ± 0.18b |
82.88 ± 1.11a |
7.51 ± 1.01f |
119.84 ± 1.11a |
3.92 ± 0.02e |
2 |
1.73 ± 0.12d |
0.91 ± 0.13c |
76.29 ± 2.21c |
14.44 ± 2.03d |
117.08 ± 2.11c |
7.28 ± 0.04d |
3 |
3.08 ± 0.02c |
0.76 ± 0.08c |
70.92 ± 3.31d |
18.73 ± 3.01c |
112.61 ± 3.11d |
14.67 ± 0.07c |
4 |
4.92 ± 0.04b |
0.64 ± 0.02c |
65.70 ± 4.41e |
23.62 ± 4.02b |
107.01 ± 4.10e |
23.4 ± 0.13b |
5 |
9.06 ± 0.00a |
0.60 ± 0.03c |
61.87 ± 5.51f |
30.95 ± 5.00a |
102.76 ± 5.10f |
32.51 ± 0.07a |
GS |
0 |
1.24 ± 0.07d |
1.48 ± 0.05a |
77.22 ± 0.01c |
14.46 ± 0.01d |
122.21 ± 0.12c |
— |
1 |
0.85 ± 0.05e |
0.95 ± 0.03c |
81.09 ± 1.11a |
12.12 ± 1.01f |
126.11 ± 1.12a |
5.81 ± 0.09c |
2 |
1.28 ± 0.10d |
0.89 ± 0.03c |
79.93 ± 2.21b |
13.74 ± 2.01e |
125.34 ± 2.12b |
4.02 ± 0.17d |
3 |
2.12 ± 0.06c |
0.84 ± 0.05c |
76.33 ± 3.31d |
17.44 ± 3.01c |
121.20 ± 3.12d |
3.37 ± 0.02e |
4 |
3.00 ± 0.05b |
1.23 ± 0.06b |
74.86 ± 4.41e |
20.38 ± 4.01b |
121.00 ± 4.12e |
6.55 ± 0.04b |
5 |
7.07 ± 0.17a |
1.19 ± 0.05b |
65.26 ± 5.51f |
28.45 ± 5.01a |
108.00 ± 5.10f |
23.41 ± 0.06a |
KZ |
0 |
1.59 ± 0.06de |
2.55 ± 0.17a |
78.01 ± 0.01c |
10.17 ± 0.01e |
117.37 ± 0.11c |
— |
1 |
1.25 ± 0.01e |
1.22 ± 0.04c |
80.36 ± 1.11a |
8.84 ± 1.01f |
119.86 ± 1.11a |
3.63 ± 0.16d |
2 |
1.91 ± 0.02d |
0.87 ± 0.03d |
79.24 ± 2.21b |
11.38 ± 2.01d |
120.13 ± 2.12b |
3.20 ± 0.16e |
3 |
3.55 ± 0.13c |
1.05 ± 0.16 cd |
76.91 ± 3.31d |
14.33 ± 3.01c |
119.51 ± 3.11d |
4.78 ± 0.10c |
4 |
8.84 ± 0.17b |
1.08 ± 0.09 cd |
69.04 ± 4.41e |
22.52 ± 4.03b |
112.12 ± 4.11e |
16.16 ± 0.09b |
5 |
20.54 ± 0.40a |
1.61 ± 0.13b |
38.12 ± 5.51f |
35.82 ± 5.01a |
64.51 ± 5.64f |
71.06 ± 0.04a |
The peroxide value can reflect the formation degree of primary oxidation products of oil. As shown in Table 2, germination had pronounced effects on the peroxide value of flaxseed oil (P < 0.05). The peroxide value of germinated flaxseed oil was significantly lower than that of non-germinated oil. The peroxide value of flaxseed oil in GS and KZ showed a trend of first decreasing and then increasing during germination, and reached the minimum value on day 3 (0.84 meq O2 kg−1 oil) and day 2 (0.87 meq O2 kg−1 oil), respectively. While the peroxide value of flaxseed oil in IM showed a decreasing trend and reached the minimum value (0.60 meq O2 kg−1 oil) on day 5 of germination. The decrease in peroxide value may be caused by the complex splitting and interaction of unstable hydroperoxides to produce secondary oxidation products during germination.37
Germination significantly influenced the color of flaxseed oil (Table 2). As the time of germination progressed, the lightness (L*) values increased at the initial stage of germination and then decreased due to the release of color pigment from the plastid.38 The trend of the yellowness (b*) values is similar to the brightness values, while the trend of the red (a*) values is opposite it. After 5 days of germination, the lowest L* (38.12) and b* (64.51), and highest a* (35.82) values were observed for the flaxseed oil in KZ. In addition, ΔE increased significantly during germination, demonstrating that this process had a remarkable color-darkening effect on flaxseed oil.
3.3 Fatty acid content of flaxseed oil
The changes in the composition of fatty acids in flaxseed oil are presented in Table 3. The non-germinated flaxseed oil was rich in unsaturated fatty acids, in which the most abundant α-linolenic acid was about 52.08–56.59%, followed by oleic acid at about 18.39–21.91%, and linoleic acid at about 15.30–16.68%, with the total unsaturated fatty acid content approaching 90%. The saturated fatty acids were mainly palmitic acid and stearic acid with proportions of about 4.86–5.70% and 3.79–4.77% respectively. The obtained results resembled that of previous research.1 The α-linolenic acid content in GS was the highest (55.40%) among the three varieties. The high variability of fatty acid profiles may be explained by different genotypes and local varieties.39 Differences in fatty acid composition can be observed between the non-germinated and germinated samples. With the prolongation of germination time, the unsaturated fatty acids content of flaxseed oil in three varieties increased from 90.65%, 91.00%, and 89.64% to 90.96%, 91.31%, and 89.78%, respectively. The linoleic acid content increased by 0.44%, 0.63%, and 0.45%, respectively, and the oleic acid fluctuated. The α-linolenic acid content showed a trend of first increasing and then decreasing during germination. The α-linolenic acid content in IM, GS, and KZ increased slightly to the maximum (56.46%, 56.59%, and 53.06%) on day 1, day 2, and day 2 of germination, respectively, with IM increasing by 0.10%, GS increasing by 0.19%, and KZ by 0.06%. When germinated to 5 days, the α-linolenic acid content in IM, GS, and KZ reached the minimum, decreasing by 0.42%, 0.41%, and 0.92%, respectively. The change of α-linolenic acid showed a slow-to-quick tendency. These changes in lipid fractions may be due to lipolytic activity and the decomposition of triglycerides and polar lipids into simpler compounds during germination.40 The results were in accordance with earlier reports on lentil41 and flaxseed.13 Germination promoted a significant decrease in the proportion of palmitic acid, whereas the change of stearic acid was small. Therefore, germination pretreatment increased the proportion of unsaturated fatty acids in flaxseed oil and improved the fatty acid composition.
Table 3 Effect of germination of flaxseed on the fatty acid content of extracted oila,b
Flaxseed varieties |
Germination time (day) |
Fatty acid (%) |
Palmitic |
Stearic |
Oleic |
Linoleic |
α-Linolenic |
Σ Saturated fatty acids |
Σ Polyunsaturated fatty acids |
Σ Unsaturated fatty acids |
Results are means ± SD of triplicate determinations. Column values in the same flaxseed variety with different lowercased letters are significantly different (P < 0.05). |
IM |
0 |
5.56 ± 0.01a |
3.79 ± 0.05b |
18.97 ± 0.14 cd |
15.32 ± 0.01f |
56.36 ± 0.17 ab |
9.35 ± 0.04a |
71.68 ± 0.18bc |
90.65 ± 0.04d |
1 |
5.46 ± 0.01b |
3.81 ± 0.02b |
18.90 ± 0.03d |
15.37 ± 0.01e |
56.46 ± 0.05a |
9.27 ± 0.03b |
71.83 ± 0.05 ab |
90.73 ± 0.03c |
2 |
5.41 ± 0.00c |
3.80 ± 0.01b |
18.95 ± 0.02 cd |
15.47 ± 0.01d |
56.37 ± 0.02 ab |
9.22 ± 0.01c |
71.83 ± 0.03 ab |
90.78 ± 0.02b |
3 |
5.36 ± 0.02d |
3.83 ± 0.01 ab |
19.15 ± 0.03b |
15.57 ± 0.04c |
56.09 ± 0.07c |
9.19 ± 0.03c |
71.66 ± 0.06c |
90.81 ± 0.03b |
4 |
5.27 ± 0.03e |
3.82 ± 0.01b |
19.04 ± 0.02c |
15.64 ± 0.03b |
56.24 ± 0.02b |
9.08 ± 0.03d |
71.88 ± 0.03a |
90.92 ± 0.02a |
5 |
5.17 ± 0.02f |
3.87 ± 0.00a |
19.26 ± 0.02a |
15.76 ± 0.00a |
55.94 ± 0.04d |
9.04 ± 0.02d |
71.69 ± 0.04bc |
90.96 ± 0.02a |
GS |
0 |
5.19 ± 0.01a |
3.81 ± 0.03b |
18.55 ± 0.06 ab |
16.05 ± 0.01e |
56.40 ± 0.08a |
9.00 ± 0.03a |
72.45 ± 0.09c |
91.00 ± 0.06e |
1 |
5.16 ± 0.01b |
3.83 ± 0.01 ab |
18.49 ± 0.02bc |
16.05 ± 0.01e |
56.47 ± 0.04a |
8.99 ± 0.02a |
72.52 ± 0.04c |
91.01 ± 0.02e |
2 |
4.99 ± 0.02c |
3.85 ± 0.02a |
18.44 ± 0.12bc |
16.13 ± 0.06d |
56.59 ± 0.21a |
8.84 ± 0.04b |
72.72 ± 0.15b |
91.16 ± 0.12d |
3 |
4.92 ± 0.00d |
3.82 ± 0.00 ab |
18.39 ± 0.02c |
16.33 ± 0.00c |
56.54 ± 0.02a |
8.74 ± 0.00c |
72.87 ± 0.02a |
91.26 ± 0.02c |
4 |
4.83 ± 0.02e |
3.82 ± 0.02 ab |
18.41 ± 0.06c |
16.44 ± 0.02b |
56.50 ± 0.08a |
8.66 ± 0.01d |
72.94 ± 0.05a |
91.34 ± 0.06a |
5 |
4.86 ± 0.00e |
3.84 ± 0.01 ab |
18.63 ± 0.00a |
16.68 ± 0.01a |
55.99 ± 0.02b |
8.69 ± 0.01e |
72.67 ± 0.01b |
91.31 ± 0.00b |
KZ |
0 |
5.70 ± 0.00a |
4.65 ± 0.00b |
21.31 ± 0.02c |
15.34 ± 0.01e |
53.00 ± 0.02a |
10.36 ± 0.01a |
68.34 ± 0.02b |
89.64 ± 0.02b |
1 |
5.67 ± 0.01b |
4.74 ± 0.08a |
21.29 ± 0.10c |
15.36 ± 0.04e |
52.94 ± 0.18a |
10.41 ± 0.09a |
68.30 ± 0.17b |
89.59 ± 0.10b |
2 |
5.51 ± 0.03c |
4.72 ± 0.01a |
21.26 ± 0.04c |
15.46 ± 0.01d |
53.06 ± 0.03a |
10.23 ± 0.02b |
68.51 ± 0.02a |
89.77 ± 0.04a |
3 |
5.44 ± 0.01e |
4.75 ± 0.01a |
21.32 ± 0.01c |
15.52 ± 0.00c |
52.97 ± 0.02a |
10.19 ± 0.02b |
68.48 ± 0.02a |
89.81 ± 0.01a |
4 |
5.48 ± 0.00d |
4.73 ± 0.00a |
21.78 ± 0.00b |
15.59 ± 0.00b |
52.42 ± 0.01b |
10.20 ± 0.01b |
68.01 ± 0.01c |
89.80 ± 0.00a |
5 |
5.45 ± 0.00de |
4.77 ± 0.01a |
21.91 ± 0.02a |
15.79 ± 0.00a |
52.08 ± 0.02c |
10.22 ± 0.01b |
67.87 ± 0.03d |
89.78 ± 0.02a |
3.4 Tocopherols contents of flaxseed oil
Tocopherols are important antioxidant active substances in flaxseed oil. The effects of germination on tocopherol content in flaxseed oil of three varieties are given in Fig. 1 α-, β- and γ-tocopherol in non-germinated flaxseed oil were detected in this study, among which γ-tocopherol content was the highest, accounting for approximately 99% of the total tocopherol. The γ-tocopherol content of flaxseed oil in IM, GS, and KZ showed a decreasing trend and decreased to the minimum value (134.02, 183.12, and 149.40 mg kg−1) on day 5 of germination. The content of α-tocopherol and β-tocopherol content increased gradually during the 5 days germination period. As germination time progressed to 5 days, the maximum value of α-tocopherol (301.86, 389.78, and 258.11 mg kg−1) and β-tocopherol (39.76, 13.47, 16.17 mg kg−1) were observed in all varieties, and the total tocopherol content in IM, GS, and KZ increased from 349.10, 451.07, and 358.16 mg kg−1 to 475.64, 586.37, and 423.68 mg kg−1, respectively. Among the three varieties, GS had the highest total tocopherol content and the highest increase and increased by 135.30 mg kg−1. As the germination time reached 5 days, the tocopherol composition was changed from original γ-tocopherol dominant to α-tocopherol dominant; α-tocopherol is traditionally recognized to have the most antioxidant activity of vitamin E in humans.42 These results were consistent with the previously reported flaxseed and canola oil, which both found similar interconversion between the α- and γ-tocopherol isomers.13,20 The interconversion of the α- and γ-tocopherol isomers may be due to the activation of the enzyme γ-methyltransferase during germination; this enzyme is key to the interconversion.21 In our study, germination promoted the increase in total tocopherol content in flaxseed oil and improved the composition of tocopherol, with a significant increase in the content of the most active α-tocopherol.
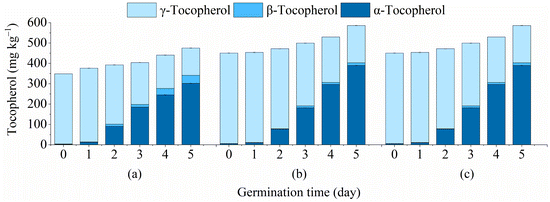 |
| Fig. 1 Effect of germination of three flaxseed samples on tocopherols content (α-, β-, γ-, and total tocopherol) of flaxseed oil: (a) IM; (b) GS; (c) KZ. | |
3.5 Phytosterol contents of flaxseed oil
Phytosterols are fat-soluble substances that are stable at high temperatures and do not affect the organoleptic properties of vegetable oils. This substance has good oxidation and thermal stability and can effectively inhibit oil rancidity.8 The common types of phytosterols found in flaxseed are β-sitosterol, campesterol, cycloartenol, stigmasterol, Δ-5-avenasterol, and 24-methylene cycloartenol, with β-sitosterol as the predominant form. As shown in Table 4, the phytosterol composition of flaxseed oil was similar between varieties, with differences in the content of each sterol monomer. The campesterol content was significantly higher in GS and KZ than in IM. Moreover, the stigmasterol content in KZ was the highest of the three varieties. The highest content of total phytosterols was in KZ, followed by GS and IM.
Table 4 Effect of germination of flaxseed on phytosterol content of extracted oila,b
Flaxseed varieties |
Germination time (day) |
Phytosterol (mg kg−1) |
Campesterol |
Stigmasterol |
β-Sitosterol |
Δ-5-Avenasterol |
Cycloartenol |
24-Methylene cycloartanol |
Total phytosterols |
Results are means ± SD of triplicate determinations. Column values in the same flaxseed variety with different lowercased letters are significantly different (P < 0.05). |
IM |
0 |
273.27 ± 11.29bc |
229.91 ± 13.22d |
499.25 ± 1.28a |
190.97 ± 1.75a |
461.61 ± 18.12a |
104.57 ± 3.90b |
1759.59 ± 9.27b |
1 |
253.55 ± 1.20c |
183.78 ± 9.48e |
448.75 ± 11.95c |
165.58 ± 0.85b |
432.71 ± 24.26 ab |
98.39 ± 5.39b |
1582.75 ± 53.14d |
2 |
256.37 ± 8.11c |
248.60 ± 8.29d |
447.36 ± 1.41c |
158.76 ± 0.75b |
428.81 ± 9.67 ab |
102.18 ± 4.07b |
1642.07 ± 32.30 cd |
3 |
281.13 ± 15.21 ab |
311.17 ± 21.03c |
447.64 ± 14.35c |
145.66 ± 6.44c |
425.14 ± 12.88b |
100.77 ± 3.52b |
1711.51 ± 73.43bc |
4 |
298.42 ± 2.86a |
361.86 ± 1.42b |
455.71 ± 5.87bc |
149.28 ± 0.06c |
411.33 ± 0.43b |
102.89 ± 0.99b |
1779.49 ± 9.53b |
5 |
295.14 ± 2.66a |
436.39 ± 15.82a |
472.14 ± 7.25b |
158.72 ± 2.23b |
424.73 ± 1.98b |
116.68 ± 1.73a |
1903.79 ± 28.21a |
GS |
0 |
429.94 ± 12.20b |
200.52 ± 0.10e |
543.21 ± 9.91 ab |
191.02 ± 1.28a |
400.39 ± 4.97a |
113.99 ± 0.89a |
1879.07 ± 29.34b |
1 |
432.63 ± 25.32b |
178.73 ± 6.75d |
606.58 ± 8.20a |
161.11 ± 0.08b |
377.48 ± 24.43 ab |
104.24 ± 2.61b |
1860.76 ± 53.73b |
2 |
307.83 ± 15.89c |
207.46 ± 3.39d |
466.32 ± 4.64b |
146.56 ± 0.41c |
380.79 ± 5.02 ab |
101.41 ± 2.74b |
1610.37 ± 0.50d |
3 |
308.85 ± 3.63c |
263.87 ± 2.92c |
473.57 ± 7.42b |
143.30 ± 2.73c |
381.88 ± 9.13 ab |
101.47 ± 2.93b |
1672.95 ± 28.76 cd |
4 |
315.56 ± 5.40c |
310.28 ± 9.54b |
512.54 ± 75.74b |
133.50 ± 2.64d |
364.68 ± 4.82bc |
101.69 ± 1.66b |
1738.24 ± 51.67c |
5 |
475.34 ± 4.34a |
411.15 ± 5.58a |
620.90 ± 2.27a |
145.51 ± 0.77c |
342.14 ± 8.18c |
113.36 ± 0.29a |
2108.41 ± 1.60a |
KZ |
0 |
435.31 ± 12.56 ab |
316.06 ± 3.04d |
583.93 ± 8.85a |
160.11 ± 1.19a |
358.95 ± 7.43a |
129.33 ± 3.59a |
1983.69 ± 21.80b |
1 |
415.11 ± 0.68bc |
291.39 ± 1.87e |
559.21 ± 4.68b |
148.73 ± 0.73b |
355.52 ± 6.04 ab |
122.79 ± 0.26 ab |
1892.73 ± 2.09 cd |
2 |
399.32 ± 12.36c |
319.42 ± 2.48d |
532.57 ± 0.19c |
139.87 ± 0.18c |
363.30 ± 13.11a |
116.02 ± 8.93 ab |
1870.50 ± 18.65d |
3 |
405.89 ± 15.43c |
359.64 ± 7.87c |
505.61 ± 9.14d |
134.69 ± 0.08d |
343.92 ± 0.05abc |
117.83 ± 0.58 ab |
1867.57 ± 31.81d |
4 |
426.33 ± 13.12abc |
442.32 ± 3.23b |
519.49 ± 14.02 cd |
134.28 ± 3.06d |
327.75 ± 12.00c |
110.72 ± 14.06b |
1960.88 ± 59.50bc |
5 |
451.94 ± 7.31a |
513.97 ± 6.03a |
527.62 ± 4.29c |
140.70 ± 2.35c |
335.77 ± 5.58bc |
121.35 ± 2.52 ab |
2091.36 ± 28.09a |
No significant changes were found in phytosterol monomer species, whereas significant changes were observed in the total phytosterol content and content of each phytosterol monomer after the germination treatment. With the extension of germination time, the content of total phytosterols and each phytosterol monomer showed a trend of first decreasing and then increasing. The total phytosterol content level varied from 1582.75 mg kg−1 to 1903.79 mg kg−1, 1610.37 mg kg−1 to 2108.41 mg kg−1, and 1867.57 mg kg−1 to 2091.36 mg kg−1 during the 5 days germination. In IM, GS, and KZ, the total phytosterol content level fell to the lowest value on day 1, day 2, and day 3 of germination, decreasing by 10.05%, 14.30%, and 5.85%, respectively. Then, it gradually increased with the extension of germination time. The total phytosterols exceeded the ungerminated oil on day 5 of germination, which increased from 1759.59, 1879.07, and 1983.69 mg kg−1 to 1903.79, 2108.41, and 2091.36 mg kg−1, respectively. The total phytosterols in GS showed the largest increase than other varieties and increased by 229.34 mg kg−1 compared with the non-germinated oil. An evident increasing trend of campesterol and stigmasterol was observed, the content of stigmasterol was higher than ungerminated oil on day 2 of germination and was approximately 1.63 to 2.05 times as high as the ungerminated oil on day 5 of germination. The result suggested that appropriate germination treatment was beneficial to the enhancement of phytosterol content; this finding was consistent with the report of Beaulieu et al.43 This result can guide the rational selection of germination times to achieve targeted access to phytosterols and phytosterol monomers.
3.6 Chlorophyll and carotenoid contents of flaxseed oil
Chlorophyll and carotenoid are the main pigments in flaxseed oil. The effect of germination pretreatment on the chlorophyll and carotenoid contents of flaxseed oil is presented in Table 5. Germination pretreatment has a significant effect on the chlorophyll and carotenoid contents of flaxseed oil (P < 0.05). The chlorophyll content increased with increasing germination time. Compared with ungerminated oil, when germinated for 5 days, the chlorophyll content increased by 134.04%, 68.04%, and 65.16%, respectively, with the largest increase in IM and significantly higher chlorophyll content in KZ than the other two varieties. Carotenoid content in flaxseed oil was greatly increased by germination. The carotenoids in IM, GS, and KZ increased from 1.56, 2.68, and 1.92 mg kg−1 to 4.81, 5.18, and 4.81 mg kg−1 during the 5 days germination period, respectively. The carotenoid content in GS was the highest (2.68 mg kg−1 oil–5.18 mg kg−1 oil) among the three varieties during germination. The flaxseed oil in IM showed the greatest increase in carotenoids, which followed a similar trend to chlorophyll, and was approximately 3.08 times greater than ungerminated oil on day 5 of germination. In a similar study, Herchi et al.19 found that germination promoted an increase in chlorophyll and carotenoid content in cold-pressed flaxseed oil, with chlorophyll content in flaxseed oil increasing from 4.45 mg kg−1 to 7.37 mg kg−1 and carotenoid content increasing from 2.23 mg kg−1 to 6.27 mg kg−1 after 4 days germination.
Table 5 Effect of germination of flaxseed on pigments, total phenolic content, and antioxidant capacity of extracted oila,b
Flaxseed varieties |
Germination time (day) |
Carotenoids (mg kg−1 oil) |
Chlorophyll (mg kg−1 oil) |
Total phenolic (mg GAE kg−1) |
DPPH radical scavenging activity (μmol TE kg−1) |
ABTS radical scavenging activity (μmol TE kg−1) |
Results are means ± SD of triplicate determinations. Column values in the same flaxseed variety with different lowercased letters are significantly different (P < 0.05). |
IM |
0 |
1.56 ± 0.00f |
0.94 ± 0.01e |
64.29 ± 1.45e |
42.67 ± 2.47f |
365.93 ± 17.28f |
1 |
1.58 ± 0.00e |
1.12 ± 0.01d |
69.48 ± 1.49e |
119.48 ± 3.84e |
458.89 ± 2.10e |
2 |
1.89 ± 0.00d |
1.48 ± 0.02c |
157.78 ± 0.03b |
486.22 ± 3.61d |
1362.77 ± 3.62d |
3 |
2.54 ± 0.01c |
1.88 ± 0.04b |
191.29 ± 1.63c |
539.50 ± 4.61c |
1496.43 ± 11.48c |
4 |
3.04 ± 0.01b |
1.90 ± 0.05b |
204.55 ± 4.52b |
564.54 ± 5.32b |
1631.58 ± 8.94b |
5 |
4.81 ± 0.00a |
2.20 ± 0.01a |
236.30 ± 0.46a |
605.62 ± 0.39a |
1864.41 ± 4.84a |
GS |
0 |
2.68 ± 0.01d |
0.97 ± 0.02d |
69.74 ± 2.03e |
54.31 ± 6.26e |
408.03 ± 20.18e |
1 |
2.42 ± 0.02e |
1.14 ± 0.01c |
74.19 ± 2.18e |
75.29 ± 8.75e |
396.50 ± 15.15e |
2 |
2.73 ± 0.04d |
1.53 ± 0.01b |
108.73 ± 0.45d |
149.92 ± 12.18d |
640.48 ± 16.32d |
3 |
2.92 ± 0.00c |
1.55 ± 0.02b |
137.20 ± 2.30c |
286.49 ± 0.44c |
987.94 ± 2.19c |
4 |
3.61 ± 0.02b |
1.53 ± 0.06b |
157.53 ± 2.41b |
312.63 ± 7.13b |
1055.18 ± 38.60b |
5 |
5.18 ± 0.01a |
1.63 ± 0.01a |
244.89 ± 4.56a |
505.65 ± 13.10a |
1599.54 ± 25.53a |
KZ |
0 |
1.92 ± 0.01e |
1.55 ± 0.00d |
75.85 ± 3.09e |
78.65 ± 3.48e |
541.48 ± 0.81e |
1 |
1.94 ± 0.02e |
1.55 ± 0.02d |
66.29 ± 0.97e |
93.16 ± 3.76e |
549.41 ± 4.91e |
2 |
2.17 ± 0.02d |
1.82 ± 0.02c |
98.84 ± 5.46d |
210.16 ± 5.08d |
816.31 ± 9.57d |
3 |
2.30 ± 0.02c |
1.86 ± 0.01c |
130.24 ± 4.64c |
262.53 ± 8.81c |
967.78 ± 17.40c |
4 |
3.24 ± 0.01b |
1.92 ± 0.02b |
201.11 ± 7.61b |
418.59 ± 5.07b |
1468.33 ± 12.76b |
5 |
4.81 ± 0.00a |
2.56 ± 0.02a |
297.78 ± 6.70a |
599.66 ± 11.27a |
2096.75 ± 19.57a |
3.7 Total phenolic content and antioxidant capacity of flaxseed oil
Table 5 illustrates the variations in the total phenolic content, DPPH radical scavenging activity, and ABTS radical scavenging activity of flaxseed oil. The total phenolic content of flaxseed oil was enhanced by germination. The total phenolic content of flaxseed oil did not change significantly at the beginning of germination, and that of the KZ decreased slightly on day 1 of germination but did not reach significance. The decrease in total phenolic content may be due to the leaching of water-soluble polyphenols or to the formation of insoluble complexes with carbohydrates and proteins.44 The initial values of the total phenolic content of the three varieties for germination had slight differences, with the initial values ranging from about 64.29 mg kg−1–75.85 mg kg−1, and increased to 236.30 mg kg−1–297.78 mg kg−1 during the 5 days germination; the total phenolic content of KZ showed the largest increase (221.93 mg GAE kg−1). These results agreed with those of Li et al.14 and Rasera et al.,15 which indicated that the moderate germination pretreatment was beneficial to the increase in total phenolic content. The increase in total phenolic content may be attributed to the fact that germination pretreatment promoted the conversion of binding phenols to free phenols, which were more readily released in oils.45
As shown in Table 5, germination pretreatment had a positive influence on the DPPH and ABTS radical scavenging activity of flaxseed oil. The DPPH and ABTS radical scavenging activity of the oils extracted from the germination–treated flaxseed were higher than the non–germinated control group (P < 0.05). Furthermore, the DPPH radical scavenging activity of flaxseed oil fluctuated between 42.67 and 605.62 μmol TE kg−1 and increased with the increase in germination time. The ABTS radical scavenging activity of flaxseed oil fluctuated between 365.93 and 2096.75 μmol TE kg−1. The trend of increase was most pronounced in the IM, with DPPH and ABTS free radical scavenging capacity increasing by 13.19 times and 3.78 times, respectively, after 5 days germination. These results were in accordance with the reported results for millet flour, which indicated that the germination pretreatment was beneficial to the increase in antioxidant activity and the total phenolic content showed a positive correlation coefficient of 0.99 with antioxidant activity.18 The enhanced antioxidant capacity of oil extracted from germination-treated flaxseed may be the result of the release of phenolic substances during germination pretreatment.15,30
3.8 Correlation analysis
The correlation analysis of the physicochemical parameters, nutritional composition, and antioxidant activity of oil extracted from germination-treated flaxseed is shown in Fig. 2. A significant positive correlation was found between germination time and numerous physicochemical and nutritional indicators of flaxseed oil, such as acid value, α-tocopherol, total tocopherol, stigmasterol, chlorophyll, carotenoids, total phenols, and DPPH and ABTS free radical scavenging activity. Additionally, a significant negative correlation was found between germination time and some indicators, such as oil content, peroxide value, γ-tocopherol, and Δ5-avenasterol. The results of the correlation analysis indicated that germination had a significant influence on the quality of flaxseed oil, among which the positive influence on nutritional indicators such as α-tocopherol, stigmasterol, chlorophyll, carotenoids, total phenols, and DPPH and ABTS radical scavenging activity of flaxseed oil was most prominent. The correlation coefficients between germination time and these nutritional indicators were 0.96, 0.80, 0.79, 0.85 0.93, 0.88, and 0.91. Based on the good correlations, these indicators can be used as good parameters for the analysis of germination time.
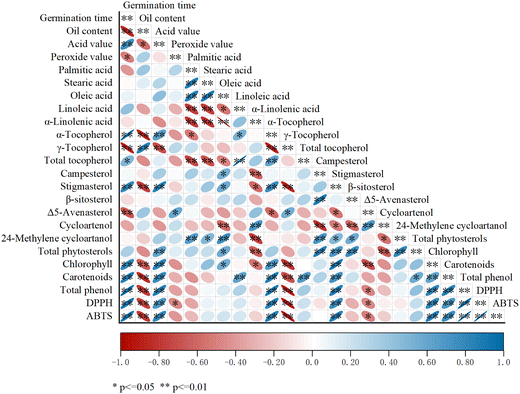 |
| Fig. 2 Correlation analysis of physicochemical parameters, nutritional composition, and antioxidant activity of germinated flaxseed oil. | |
The correlation between the fatty acids revealed a significant negative correlation between saturated fatty acids (stearic acid, palmitic acid) and polyunsaturated fatty acids (linoleic acid, α-linolenic acid), whereas a significant positive correlation existed between saturated fatty acids and monounsaturated fatty acid (oleic acid). The significant negative correlation between saturated fatty acids and polyunsaturated fatty acids may be due to germination promoting the desaturation of fatty acids in plant seeds and stimulating fatty acid desaturase activity.46 The highly significant negative correlation between α-tocopherol and γ-tocopherol (r = 0.89, P < 0.01) may be due to the activation of γ-methyltransferase during germination, which is the key enzyme for the interconversion of α-tocopherol and γ-tocopherol.21 The correlation between α-tocopherol, which has strong antioxidant activity, and phytosterols showed a positive correlation between α-tocopherol and stigmasterol, a negative correlation between α-tocopherol and Δ5-avenasterol, and no significant relationship with total phytosterols. In addition, a highly significant positive correlation was found between α-tocopherol, stigmasterol, chlorophyll, carotenoids, total phenols, and antioxidant activity. Studies have shown that chlorophyll and its degradation products have a scavenging effect on peroxyl radicals and other free radicals.47 Carotenoids could delay the oxidation of oils by reducing the activity of singlet oxygen.48 Phenolic compounds could achieve antioxidation by scavenging free radicals and chelating metal ions.49 Tocopherols, as a natural antioxidant, produce their own phenolic hydroxyl groups, which lose hydrogen easily and bind to peroxides, interrupting the chain process of oxidation reactions.50 Phytosterols also contain phenolic hydroxyl groups, whose lost hydrogen can bind to tocopherol radicals and act as synergists for the regeneration of tocopherols, which collectively improve the oxidative stability of oils.51,52 Therefore, based on the highly significant positive correlation between these nutrients and antioxidant activity, these nutritional indicators can be used as an important reference for determining the antioxidant activity of oils during germination.
3.9 Principal component analysis
The principal component analysis allows for a comprehensive characterization of sample quality based on various indicators of the oil samples. The physicochemical indicators, lipid concomitants, and antioxidant capacity of flaxseed oil at different germination times were subjected to principal component analysis, and the results are shown in Fig. 3.
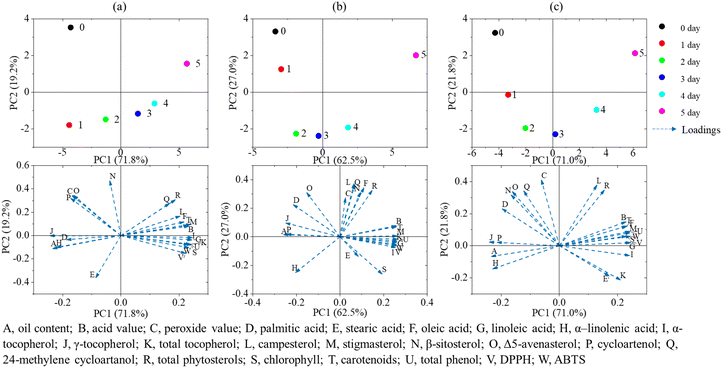 |
| Fig. 3 Principal component analysis of each indicator of flaxseed oil at different times of germination (a) IM; (b) GS; (c) KZ. | |
The physicochemical indexes, fatty acids, lipid concomitants, and antioxidant capacity of flaxseed oil of three varieties at different germination times were subjected to principal component analysis to explore the correlation between oil quality and germination. As shown in Fig. 3, the contributions of the first principal component were 71.8%, 62.5%, and 71.0% of IM, GS, and KZ, respectively, and the contributions of the second principal component were 19.2%, 27.0%, and 21.8%, with the cumulative contribution of the two components being 91.0%, 89.5%, and 92.8%, respectively, which could explain most of the information in the original data.
The principal component score plot could distinguish the germination process of flaxseed oil into three stages. In Stage 1 (day 0–1), the quality indicators of flaxseed oil at this stage did not differ much from the initial values, and the physicochemical and nutritional indicators (except peroxide value and phytosterols) were at around the initial level. In Stage 2 (day 2–3), the quality of flaxseed oil changed significantly, including an increase in acid value, a decrease in peroxide value, an increase in unsaturated fatty acids, and in the content of various nutrients, resulting in improved oil quality, oxidative stability, and antioxidant activity. In Stage 3 (day 4–5), the rate of changes of nutritional indicators, such as α–tocopherol, total phenols, chlorophylls, and carotenoids, in flaxseed oil increased rapidly, and the content of tocopherols, phytosterol, pigments, and total phenols increased significantly, with stronger DPPH and ABTS free radical scavenging ability. While the acid value at this stage was high, the target substances could be obtained by technical means as required. Through principal component analysis, flaxseed oil of different germination times can be evaluated and differentiated, revealing the dynamic changes of physicochemical properties and lipid concomitants of flaxseed oil during the germination period, which is crucial to the rational selection of germination times, quality control, and functionalized processing, and utilization of edible oils.
4. Conclusion
In this study, the effect of germination pretreatment on the quality properties of flaxseed oil was investigated. This study showed that germination could effectively improve the antioxidant capacity, α-tocopherol, stigmasterol, total phenolic, carotenoid, and chlorophyll contents of oil and reduce peroxide value. While the oil content of flaxseed decreased, the acid value increased, and the color of flaxseed oil deepened during germination. Notably, the oil from GS showed a higher level of α-linolenic acid, tocopherol, and carotenoid; a lower level of acid value; and a maximum increase level of tocopherol and phytosterol than other varieties during germination. Correlation and principal component analysis showed a significant increase in flaxseed lipid concomitants when germination time exceeded 2 days. In practice, the time of germination can be targeted in accordance with production needs. Collectively, our results can be beneficial for developing functional food with high α-tocopherol and phenolic contents, and provide a reference for the variation in the lipid concomitants in flaxseed oil during germination and the development of flaxseed oil with higher antioxidant activity.
Conflicts of interest
The authors have no conflict of interest to declare.
Acknowledgements
This work was supported by the Industrial Innovation Key Tasks List Revealed Project of Ningxia Hui Autonomous Region, China (No. 20210816).
References
- D. Zhang, X. J. Li, X. L. Duan, H. Sun and Y. P. Cao, Food Chem., 2021, 364, 130431 CrossRef CAS PubMed.
- M. A. Farag, D. M. Elimam and S. M. Afifi, Trends Food Sci. Technol., 2021, 114, 292 CrossRef CAS.
- W. X. Chen, J. M. Wang, Y. Y. Ren, H. Chen, C. L. He and Q. Wang, Algal Res., 2021, 60, 102505 CrossRef.
- J. Yang, C. T. Wen, Y. Q. Duan, Q. C. Deng, D. F. Peng, H. H. Zhang and H. L. Ma, Trends Food Sci. Technol., 2021, 118, 252 CrossRef CAS.
- K. K. Singh, D. Mridula, J. Rehal and P. Barnwal, Crit. Rev. Food Sci. Nutr., 2011, 51, 210 CrossRef CAS PubMed.
- M. Lu, L. Tan, X. G. Zhou, Z. L. Yang, Q. Zhu, J. N. Chen, H. R. Luo and G. S. Wu, Oxid. Med. Cell. Longevity, 2020, 2020, 1293935 Search PubMed.
- K. Suri, B. Singh, A. Kaur and N. Singh, J. Food Sci. Technol., 2022, 59, 366 CrossRef CAS PubMed.
- J. H. Huang, C. Y. Chen, Z. H. Song, M. Chang, L. Yao, Q. Z. Jin and X. G. Wang, Food Chem., 2022, 388, 133010 CrossRef CAS PubMed.
- Z. J. Chen, P. Wang, Y. Weng, Y. Ma, Z. X. Gu and R. Q. Yang, Food Biosci., 2017, 20, 159 CrossRef CAS.
- K. Y. Lee, M. S. Rahman, A. N. Kim, E. J. Jeong, B. G. Kim, M. H. Lee, H. J. Kim and S. G. Choi, LWT–Food Sci. Technol., 2021, 135, 110240 CrossRef CAS.
- P. Pajak, R. Socha, D. Galkowska, J. Roznowski and T. Fortuna, Food Chem., 2014, 143, 300 CrossRef CAS PubMed.
- D. Rico, E. Peñas, M. del Carmen García, C. Martínez-Villaluenga, D. K. Rai, R. I. Birsan, J. Frias and A. B. Martín-Diana, Foods, 2020, 9, 296 CrossRef CAS PubMed.
- X. Li, J. Y. Li, S. Dong, Y. F. Li, L. P. Wei, C. C. Zhao, J. Y. Li, X. B. Liu and Y. T. Wang, Int. J. Food Sci. Technol., 2019, 54, 2346 CrossRef CAS.
- R. Li, Q. Wang, G. L. Zhao, H. Peng, D. J. Zhang and Z. J. Li, Int. J. Food Sci. Technol., 2022, 57, 5175 CrossRef CAS.
- G. B. Rasera, M. H. Hilkner and R. J. S. de Castro, Food Res. Int., 2020, 133, 109115 CrossRef CAS PubMed.
- L. M. Paucar-Menacho, C. Martínez-Villaluenga, M. Dueñas, J. Frias and E. Peñas, Int. J. Food Sci. Technol., 2018, 53, 516 CrossRef CAS.
- H. Wang, J. H. Wang, X. B. Guo, C. S. Brennan, T. Li, X. Fu, G. Chen and R. H. Liu, Food Chem., 2016, 205, 170 CrossRef CAS PubMed.
- S. Sharma, R. Jan, C. S. Riar and V. Bansal, Food Chem., 2021, 361, 130073 CrossRef CAS PubMed.
- W. Herchi, S. Bahashwan, K. Sebei, H. Ben Saleh, H. Kallel and S. Boukhchina, Grasas Aceites, 2015, 66, e057 CrossRef.
- H. Y. Zhang, T. Vasanthan and M. Wettasinghe, J. Agric. Food Chem., 2007, 55, 355 CrossRef CAS PubMed.
- X. Li, S. Dong, W. L. Bai, J. Jia, R. J. Gu, C. C. Zhao, X. B. Liu and Y. T. Wang, Food Funct., 2020, 11, 9848 RSC.
- Association of Official Analytical Chemists, AOAC Official Methods, 1998, Crude fat content Search PubMed.
- American Oil Chemists' Society, AOCS Official Method Cd 3d–63, 2009, Acid value Search PubMed.
- American Oil Chemists' Society, AOCS Official Method Cd 8b-90, 2011, Peroxide value acetic acid-isooctane method Search PubMed.
- L. Y. Zhang, S. Akhymetkan, J. Chen, Y. Y. Dong, Y. Gao and X. Z. Yu, LWT-Food Sci. Technol., 2022, 155, 112947 CrossRef CAS.
- J. Jin, P. Warda, H. Y. Mu, Y. F. Zhang, L. Jie, J. H. Mao, D. Xie, J. H. Huang, Q. Z. Jin and X. G. Wang, J. Am. Oil Chem. Soc., 2016, 93, 1115 CrossRef CAS.
- M. Yang, F. H. Huang, C. S. Liu, C. Zheng, Q. Zhou and H. Wang, Food Bioprocess Technol., 2013, 6, 3206 CrossRef CAS.
- F. AL Juhaimi, M. M. Özcan, K. Ghafoor and E. E. Babiker, Food Chem., 2018, 243, 414 CrossRef CAS PubMed.
- Q. C. Deng, X. Yu, F. L. Ma, J. Q. Xu, F. H. Huang, Q. D. Huang and F. Sheng, Int. J. Food Prop., 2018, 20, S2708 CrossRef.
- H. S. Gujral, M. Angurala, P. Sharma and J. Singh, Int. J. Food Prop., 2011, 14, 1366 CrossRef CAS.
- H. L. Shi, P. K. Nam and Y. F. Ma, J. Agric. Food Chem., 2010, 58, 4970 CrossRef CAS PubMed.
- G. Waschatko, N. Billecke, S. Schwendy, H. Jaurich, M. Bonn, T. A. Vilgis and S. H. Parekh, J. R. Soc., Interface, 2016, 13, 20160677 CrossRef PubMed.
- R. Kaur and K. Prasad, J. Food Sci., 2022, 87, 2197 CrossRef CAS PubMed.
- V. M. Paradiso, A. Pasqualone, C. Summo and F. Caponio, J. Lipid Sci. Technol., 2018, 120, 1800102 CrossRef.
- R. R. Kumar, D. V. Bhargava, K. Pandit, S. Goswami, S. M. Shankar, S. P. Singh, G. K. Rai, C. T. Satyavathi and S. Praveen, Food Chem., 2021, 361, 130031 CrossRef CAS PubMed.
- A. Kawinski, M. Miklaszewska, S. Stelter, B. Glab and A. Banaś, BMC Plant Biol., 2021, 21, 50 CrossRef CAS PubMed.
- J. I. Gray, J. Am. Oil Chem. Soc., 1978, 55, 539 CrossRef CAS.
- M. Z. Islam, M. J. Shim, S. Y. Jeong and Y. T. Lee, Int. J. Food Sci. Technol., 2022, 57, 201 CrossRef CAS.
- I. A. M. Ahmed, N. Uslu, M. M. Özcan, F. A. Juhaimi, K. Ghafoor, E. E. Babiker, M. A. Osman and H. A. S. Alqah, Food Chem., 2021, 338, 128109 CrossRef PubMed.
- R. Jan, D. C. Saxena and S. Singh, Food Chem., 2018, 269, 173 CrossRef CAS PubMed.
- R. S. Pal, A. Bhartiya, P. Yadav, L. Kant, K. K. Mishra, J. P. Aditya and A. Pattanayak, J. Food Sci. Technol., 2017, 54, 909 CrossRef CAS PubMed.
- K. Szewczyk, A. Chojnacka and M. Górnicka, Int. J. Mol. Sci., 2021, 22, 6222 CrossRef CAS PubMed.
- J. C. Beaulieu, R. A. Moreau, M. J. Powell and J. M. Obando-Ulloa, Foods, 2022, 11, 220 CrossRef CAS PubMed.
- M. H. Dicko, H. Gruppen, A. S. Traore, W. J. H. Van Berkel and A. G. J. Voragen, J. Agric. Food Chem., 2005, 53, 2581 CrossRef CAS PubMed.
- L. Xu, P. Wang, B. Ali, N. Yang, Y. S. Chen, F. F. Wu and X. M. Xu, J. Sci. Food Agric., 2017, 97, 4227 CrossRef CAS PubMed.
- A. K. Choudhary and G. Mishra, Physiol. Mol. Biol. Plants, 2021, 27, 1233 CrossRef CAS PubMed.
- Y. Endo, R. Usuki and T. Kaneda, J. Am. Oil Chem. Soc., 1985, 62, 1375 CrossRef CAS.
- T. L. LI, J. M. King and D. B. Min, J. Food Biochem., 2000, 24, 477 CrossRef CAS.
- A. Zeb, J. Food Biochem., 2020, 44, e13394 CrossRef CAS PubMed.
- N. Barouh, C. Bourlieu-Lacanal, M. C. Figueroa-Espinoza, E. Durand and P. Villeneuve, Compr. Rev. Food Sci. Food Saf., 2022, 21, 642 CrossRef CAS PubMed.
- T. Wang, K. B. Hicks and R. Moreau, J. Am. Oil Chem. Soc., 2002, 79, 1201 CrossRef CAS.
- F. J. Hidalgo, M. M. León and R. Zamora, Food Res. Int., 2009, 42, 1215 CrossRef CAS.
|
This journal is © The Royal Society of Chemistry 2023 |
Click here to see how this site uses Cookies. View our privacy policy here.