DOI:
10.1039/D2RA06395F
(Paper)
RSC Adv., 2023,
13, 1066-1077
Synthesis and immunopharmacological evaluation of novel TLR7 agonistic triazole tethered imidazoquinolines†
Received
11th October 2022
, Accepted 10th November 2022
First published on 4th January 2023
Abstract
Toll-like receptors-7 and -8 are expressed abundantly on antigen-presenting cells, and their agonists make potential adjuvant candidates for the development of new efficacious vaccines. In view of the importance of new efficacious imidazoquinoline based adjuvants, herein we have synthesized a focused library of a new class of imidazoquinolines retaining the N-isobutyl substitution of an imidazole moiety as in imiquimod and introduced a 1,2,3-triazolyl moiety upon alkyl substitution at the imidazolemethyne carbon employing triazolyl click chemistry. All the novel analogues were characterized using various spectroscopic techniques and the target specificity of these molecules was determined using HEK TLR7/8 transfected cell lines. TLR7/8 activity and also the molecular docking results correlated primarily to the position of the substituent for aromatic groups and also to the chain length in alkyl substitutions. The immunomodulatory properties of these analogues were evaluated using murine DC activation and also with hPBMC activation markers, cytokines which revealed that these analogues after modification were able to target the TLR7 receptors and also had a pro-inflammatory immune response.
Introduction
Small molecule Toll-like receptor-7/8 (TLR) agonists exhibit potential vaccine adjuvant activity with improved humoral and T-cell mediated immunity, through their ability to activate the antigen presenting cells (APCs). Even though ssRNAs are the natural ligands for TLR7/TLR8, the synthetic small molecule imidazoquinolines such as imiquimod and resiquimod1 have been used in the majority of vaccine studies conducted so far. Imidazoquinoline based imiquimod and resiquimod have been evaluated clinically with regard to their ability to potentially activate an innate immune response against chronic viral infections and cancer with type I interferon production. Stimulation of TLR7/8 with these molecules enhances DC activation as well as cellular immunity. Thus, the ability of TLR7/8 agonists to act as vaccine adjuvants has been unraveled majorly using these imidazoquinolines2 as they activate APCs directly through their interaction with the co-stimulatory molecules and cytokines to evoke a powerful adaptive immune response. The ability of small molecule TLR7/8 agonists to activate specific innate immune cells results in the modulation of humoral and T-cell mediated immunity, and thus these agonists have found application as adjuvant candidates for inactivated viral antigens apart from various subunit and recombinant antigens, that are poorly immunogenic. In order to be effective and safe, it is crucial that TLR7/8 agonists should evoke immune activation at the site of injection without any systemic activation. Imiquimod is approved for clinical use as topical, cream containing imiquimod (Aldara® imiquimod 5%), for external genital warts, actinic keratosis and superficial basal cell carcinoma. However, no real vaccine application of TLR7 and/or TLR8 agonists could happen except early-phase clinical studies till 2020.3 SAR studies revealed unique structural features in various active chemotypes that activate TLR7 and/or TLR8.4 Subtle variation in the structure of these chemotypes resulted in modulation of TLR7/8 activation. It has been revealed from SAR studies that substitution on C2 position with various alkyl groups enhanced the activity through TLR7 activation where as unsaturated hydrocarbon group substitution reduced the activity. Any substitution at C4–NH2 was proven to be detrimental, for TLR7 activity.5 IL-12-dependent, enhancement of tetanus-specific IgG response in human peripheral blood monocytes (hPBMCs) and potential activation of NK cells was induced by TLR7 agonistic Loxoribine. Similarly, 3M-052, a dual TLR7/8 agonist, was reported to enhance immunogenicity of influenza vaccine.6 Similarly, an oil-in-water emulsion formulation comprising HBsAg and 3M 052, exhibited improved Th1 immunity in terms of serum IgG2a as well as IFN-γ derived from splenocytes. TLR7 agonists are more potent than their TLR8 counterparts at inducing IFN-regulated chemokines viz., IFN-inducible T cell α chemoattractant and protein.1,7 A synthetic dendrimer based adjuvant consisting of six TLR7/8 agonists reported by Shukla et al., has been reported to prevent rapid clearance of the adjuvant from the antigenic site.8 Even though TLR8 activity was not detected in the multimeric adjuvant, the TLR7 agonistic effects were retained by the dendrimeric adjuvant making it a better adjuvant compared to the imidazoquinoline monomer in inducing elevated titers of high-affinity antibodies against α-lactalbumin of bovis in rabbits. Recently TLR7 agonistic imidazoquinoline compound adsorbed on alum has been successfully employed clinically as adjuvant in COVAXIN, an inactivated SARS CoV-2 vaccine developed by Bharat Biotech during the Covid pandemic as emergency use vaccine.9 This clearly reveals, the great demand for new imidazoquinoline based TLR7/8 adjuvants to improve viral vaccine efficacy. In this endeavour, we set out to design a focused library of new class of imidazoquinoline retaining N-isobutyl substitution of imidazole moiety as in imiquimod and introduce a 1,2,3-triazolyl moiety on the alkyl substitution at the imidazolemethyne carbon employing triazolyl click chemistry. This further offers opportunity to create diversity across triazolyl moiety. Furthermore, 1,2,3-triazoles are capable of establishing hydrogen bonds to enhance their solubility of imdiazoquinoline moiety, thus improving the bioavailability and their potential to interact with biomolecular targets.10 We could successfully synthesize target molecules in eight steps with good yields and purity (>99% purity). The new compounds thus synthesized were subjected to adjuvant activity and target specific assay in HEK blue TLR7/8 cells co-transfected with SEAP gene, to assess their TLR7/8 agonistic activity.
Materials and methods
Chemical synthetic procedures
All reagents were purchased from Sigma-Aldrich, TCI, and then used without further purification. Chloroform, dichloromethane (DCM), and tetrahydrofuran (THF) were dried and freshly distilled before use wherever necessary, by using standard procedures. Moisture- or air-sensitive reactions were conducted under nitrogen atmosphere conditions. All the chemical transformations were monitored using Thin Layer Chromatography (TLC) carried out on silica gel CCM pre coated aluminum sheets and TLC was detected under UV, staining was developed with iodine or by charring TLC in p-anisaldehyde or 10% phosphomolybdic acid solution in MeOH and then heated on a hot plate. The solvents were removed under reduced pressure using rotary evaporators. Compound purification done by column chromatography using silica gel (60–120 and 100–200 mesh) and also carried out using grace column chromatography (Reveleris® X2) with technical grade chloroform and methanol as eluent. All melting points were determined on a melting point apparatus and are uncorrected. 1H and 13C NMR spectra were recorded on Bruker instruments (300, 400 or 500 MHz for 1H NMR, 101 or 126 MHz for 13C NMR). Chemical shifts were quoted in parts per million (ppm) referenced to the appropriate solvent peak or 0.0 ppm for tetramethylsilane. The following abbreviations were used to describe peak splitting patterns when appropriate: s = singlet, d = doublet, t = triplet, q = quartet, dd = doublet of doublet, td = triplet of doublet, ddd = doublet of doublet of doublet, and m = multiplet. Coupling constants, J, were reported in hertz (Hz). ESI-MS, m/z values are reported in atomic mass units and recorded in Shimadzu instrument.
Synthesis of compound 2: 4-chloro-3-nitroquinoline. 3-Nitroquinolin-4-ol was dissolved (2.0 g, 10.5 mmol) in N,N-dimethylformamide (30 mL) and phosphoryl chloride (3.2 g, 21 mmol) was added dropwise to the solution. The internal temperature was maintained at 32 °C, during the addition.11,12 The reaction mixture was then stirred for an hour at 50 °C, and then was cooled to room temperature and poured into ice/water (50 mL), and the product precipitated was filtered, washed thoroughly with water, and dried to obtain the compound 2 (1.9 g, 86%). 1H NMR (400 MHz, CDCl3) δ 9.27 (s, 1H), 8.45 (dd, J = 8.5, 0.9 Hz, 1H), 8.23 (d, J = 8.4 Hz, 1H), 7.96 (ddd, J = 8.4, 7.0, 1.4 Hz, 1H), 7.83 (ddd, J = 8.3, 7.0, 1.1 Hz, 1H). MS (ESI) calcd for C9H5ClN2O2, m/z 208.004, found 208.90 [M + H]+.
Synthesis of compound 3: 4-isobutylamino-3-nitroquinoline. 4-Chloro-3-nitroquinoline (1.2 g, 5.8 mmol) in was solubilized in 30 mL of dry CH2Cl2 and was cooled to 0 °C, and a solution of isobutylamine (0.6 g, 7.5 mmol) and triethylamine (0.9 g, 8.7 mmol) were added to the reaction mixture with stirring and the reaction mixture was refluxed at 45 °C for 2 hour. After refluxing the dichloromethane was evaporated under vacuum. Water was added to it and the residue is allowed to form over a period of 3 hours.13 Filtered the compound and dried to obtain the compound 3 (1.2 g, 85%). 1H NMR (400 MHz, CDCl3) δ 9.81 (s, 1H), 9.37 (s, 1H), 8.31 (dd, J = 8.5, 0.9 Hz, 1H), 7.99 (dd, J = 8.3, 1.1 Hz, 1H), 7.76 (ddd, J = 8.3, 7.0, 1.3 Hz, 1H), 7.48 (ddd, J = 8.4, 7.0, 1.4 Hz, 1H), 3.80 (dd, J = 6.5, 4.9 Hz, 2H), 2.12–2.02 (m, 1H), 1.10 (d, J = 6.7 Hz, 6H). 13C NMR (101 MHz, CDCl3) δ 151.33, 150.60, 147.62, 132.72, 130.39, 127.28, 125.70, 125.37, 119.46, 57.19, 30.17, 20.16. MS (ESI) calcd for C13H15N3O2, m/z 245.116, found 245.95 [M + H]+.
Synthesis of compound 4: 3-amino-4-isobutylaminoquinoline. A suspension of 4-isobutylamino-3-nitroquinoline (2.5 g, 10.2 mmol), 5% palladium on carbon (50 mg), and sodium sulfate (1.5 g) in ethyl acetate (50 mL) was hydrogenated at 55 psi hydrogen pressure for 6 hour. TLC was taken at regular intervals to check the completion of reaction and after completion, the reaction mixture was then filtered through celite, and the filtrate was concentrated in vacuo. The compound was purified using column chromatography (7% MeOH/CH2Cl2), to obtain pure compound 4 (1.5 g, 68%). 1H NMR (400 MHz, CDCl3) δ 8.47 (s, 1H), 7.99–7.95 (m, 1H), 7.84–7.80 (m, 1H), 7.50–7.41 (m, 2H), 3.08 (d, J = 6.7 Hz, 2H), 1.94–1.84 (m, 1H), 1.05 (d, J = 6.7 Hz, 6H). 13C NMR (126 MHz, CDCl3) δ 144.41, 143.53, 136.70, 130.48, 129.89, 125.97, 125.92, 123.55, 120.18, 54.75, 30.01, 20.53. MS (ESI) calcd for C13H17N3, m/z 215.142, found 216.00 [M + H]+.
Synthesis of compound 5: 2-(but-3-yn-1-yl)-1-isobutyl-1H-imidazo[4,5-c]quinolone. To a solution of pentynoic acid (495 mg, 5.0 mmol) in anhydrous DMF and was added with HBTU (1.9 g, 5.0 mmol), trimethylamine (642 mg, 6.3 mmol), a catalytic amount of DMAP, and 3-amino-4-isobutylaminoquinoline (900 mg, 4.2 mmol). The reaction mixture was then heated to 90 °C for 1 h. The solvent was then removed under vacuum. The residue was dissolved in EtOAc, washed with water, dried over Na2SO4, and then concentrated.8 This was purified using column chromatography (6% MeOH/CH2Cl2) to obtain the compound 5 (459 mg, 72%). 1H NMR (400 MHz, CDCl3) δ 9.30 (s, 1H), 8.30–8.26 (m, 1H), 8.13–8.09 (m, 1H), 7.69–7.61 (m, 2H), 4.36 (d, J = 7.6 Hz, 2H), 3.23–3.18 (m, 2H), 2.96–2.90 (m, 2H), 2.45–2.34 (m, 1H), 2.03 (t, J = 2.7 Hz, 1H), 1.04 (d, J = 6.7 Hz, 6H). 13C NMR (101 MHz, CDCl3) δ 153.60, 145.21, 144.90, 136.72, 133.81, 131.26, 126.88, 126.50, 120.08, 118.06, 82.92, 69.66, 53.00, 29.36, 27.27, 19.95, 17.09. MS (ESI) calcd for C18H19N3, m/z 277.157, found 277.95 [M + H]+.
Synthesis of compound 6: 2-(but-3-yn-1-yl)-1-isobutyl-1H-imidazo[4,5-c]quinoline 5-oxide. Compound 5 (500 mg, 1.8 mmol) was dissolved in MeOH
:
dichloromethane
:
chloroform (0.1
:
1
:
1) containing peracetic acid (32% active oxygen, 776 mg, 4.5 mmol). The solution was heated at 45–50 °C (internal temperature) for 2 h, and the reaction mixture was concentrated using rotary evaporator14 and the residue was purified using column chromatography (8% MeOH/CH2Cl2), to obtain the compound 6 (438 mg, 83%).8 1H NMR (400 MHz, CDCl3) δ 9.07–9.02 (m, 2H), 8.12–8.07 (m, 1H), 7.78–7.72 (m, 2H), 4.33 (d, J = 7.6 Hz, 2H), 3.18 (t, J = 7.5 Hz, 2H), 2.90 (ddd, J = 8.4, 6.9, 2.6 Hz, 2H), 2.41–4.31 (m, 1H), 2.03 (t, J = 2.6 Hz, 1H), 1.05 (d, J = 6.7 Hz, 6H). 13C NMR (101 MHz, CDCl3) δ 155.52, 138.65, 136.11, 130.87, 128.95, 127.87, 127.34, 122.31, 120.43, 118.38, 82.61, 69.87, 53.08, 29.27, 27.20, 19.93, 17.05. MS (ESI) calcd for C18H19N3O, m/z 293.152, found 294.00 [M + H]+.
Synthesis of compound 7: N-(2-(but-3-yn-1-yl)-1-isobutyl-1H-imidazo[4,5-c]quinolin-4-yl)benzamide. To a solution of 6 (1 g, 3.4 mmol) in anhydrous dichloromethane (100 mL), followed by the dropwise addition of benzoyl isocyanate (750 mg, 5.1 mmol), with continuously stirring and refluxed at 45 °C for 30 min after the completion of the reaction, the solvent was removed under vacuum and the residue was purified using column chromatography (8% MeOH/CH2Cl2), to obtain the compound 7 (985 mg, 73%).11,15 1H NMR (400 MHz, CDCl3) δ 8.20 (s, 2H), 8.08 (s, 1H), 7.98 (d, J = 8.2 Hz, 1H), 7.64–7.47 (m, 5H), 4.33 (d, J = 7.3 Hz, 2H), 3.19 (t, J = 7.6 Hz, 2H), 2.97–2.90 (m, 2H), 2.43–2.31 (m, 1H), 2.04 (t, J = 2.6 Hz, 1H), 1.04 (d, J = 6.6 Hz, 6H). 13C NMR (101 MHz, CDCl3) δ 152.96, 134.48, 132.05, 128.62, 128.37, 127.67, 125.04, 119.92, 82.95, 69.71, 53.05, 29.38, 27.17, 19.87, 17.23. MS (ESI) calcd for C25H24N4O, m/z 396.490, found 397.30 [M + H]+.
Synthesis of compound 8: 2-(but-3-yn-1-yl)-1-isobutyl-1H-imidazo[4,5-c]quinolin-4-amine. The compound 7 (1 g, 2.5 mmol) was dissolved in anhydrous MeOH, followed by the addition of excess sodium methoxide.15 The reaction mixture was then heated at 65 °C for 1 h. The solvent was removed under vacuum, and the residue was purified using column chromatography (10% MeOH/CH2Cl2) to obtain pure compound 8 (475 mg, 65%). 1H NMR (400 MHz, CDCl3) δ 7.88 (dd, J = 8.3, 1.1 Hz, 1H), 7.82 (dd, J = 8.4, 1.1 Hz, 1H), 7.50 (ddd, J = 8.4, 7.0, 1.4 Hz, 1H), 7.32 (ddd, J = 8.3, 7.1, 1.3 Hz, 1H), 5.52 (s, 2H), 4.27 (d, J = 7.6 Hz, 2H), 3.17–3.11 (m, 2H), 2.90–2.84 (m, 2H), 2.42–2.31 (m, 1H), 2.03 (t, J = 2.7 Hz, 1H), 1.02 (d, J = 6.7 Hz, 6H). 13C NMR (101 MHz, CDCl3) δ 151.70, 151.27, 144.76, 133.67, 127.30, 127.16, 127.05, 122.37, 119.92, 115.69, 83.07, 69.58, 52.87, 29.36, 27.16, 19.91, 17.31. HRMS: calcd for C18H20N4, m/z 292.390, found 293.176 [M + H]+.
General procedure for synthesis of 1,2,3-triazolyl imidazoquinoline derivatives (9a–m). To a solution of compound 8 (100 mg, 0.342 mmol, 1.0 equiv.) and azides (0.410 mmol, 1.2 equiv.) in DMF/H2O (2
:
1), was added CuSO4 (171 mg, 0.684 mmol, 2 equiv.), sodium ascorbate (195 mg, 1.02 mmol, 3 equiv.), and the resulting heterogeneous mixture was allowed to stir vigorously for 8–12 h at room temperature. After completion of the reaction, the cold water was added to the reaction mass and extracted with EtOAc (3 × 20 ml). The organic layer was washed with brine, dried over MgSO4, filtered and concentrated under reduced pressure. The crude product was purified by flash chromatography (MeOH/CH2Cl2) to afford the desired compound.16–18
Synthesis of compound 9a: 1-isobutyl-2-(2-(1-phenyl-1H-1,2,3-triazol-4-yl)ethyl)-1H-imidazo[4,5-c]quinolin-4-amine. Compound 8 (100 mg, 0.342 mmol) and azidobenzene (49 mg, 0.410 mmol) were reacted together according to the above general procedure to afford the product in good yield (124 mg, 88%) as white solid; melting point: 232–234 °C; 1H NMR (400 MHz, CDCl3) δ 7.87 (t, J = 7.8 Hz, 2H), 7.79 (s, 1H), 7.68–7.63 (m, 2H), 7.55–7.45 (m, 3H), 7.43–7.33 (m, 2H), 6.28 (s, 2H), 4.27 (d, J = 7.6 Hz, 2H), 3.49 (dd, J = 11.0, 4.3 Hz, 2H), 3.40 (dd, J = 11.2, 4.5 Hz, 2H), 2.38–2.27 (m, 1H), 1.00 (d, J = 6.7 Hz, 6H). 13C NMR (101 MHz, CDCl3) δ 153.21, 150.90, 147.04, 137.16, 134.02, 129.86, 128.79, 127.64, 126.64, 125.96, 122.98, 120.58, 120.11, 119.96, 115.23, 52.77, 29.37, 27.40, 23.68, 19.85. HRMS: calc. for C24H25N7, m/z 411.217, found 412.224 [M + H]+.
Synthesis of compound 9b: 2-(2-(1-(4-bromophenyl)-1H-1,2,3-triazol-4-yl)ethyl)-1-isobutyl-1H-imidazo[4,5-c]quinolin-4-amine. Compound 8 (100 mg, 0.342 mmol) and 1-azido-4-bromobenzene (81 mg, 0.410 mmol) reacted according to the above general procedure to afford the product in good yield (157 mg, 94%) as light yellow solid; melting point: 192–194 °C; 1H NMR (400 MHz, CDCl3 + CD3OD) δ 8.09 (d, J = 5.3 Hz, 1H), 7.91 (d, J = 6.8 Hz, 1H), 7.75 (d, J = 6.1 Hz, 1H), 7.63 (d, J = 4.1 Hz, 3H), 7.53–7.45 (m, 2H), 7.39–7.31 (m, 1H), 4.30 (d, J = 6.1 Hz, 2H), 3.41 (dd, J = 9.3, 4.8 Hz, 4H), 2.36–2.27 (m, 1H), 0.99 (d, J = 6.0 Hz, 6H). 13C NMR (101 MHz, CDCl3 + CD3OD) δ 153.74, 151.37, 147.50, 136.44, 134.73, 133.36, 131.17, 128.40, 124.97, 123.58, 122.88, 122.35, 121.08, 120.82, 53.07, 29.69, 27.60, 23.50, 19.82, HRMS: calc. for C24H24BrN7, m/z 489.127, found 490.134 [M + H]+.
Synthesis of compound 9c: 1-isobutyl-2-(2-(1-(4-isopropylphenyl)-1H-1,2,3-triazol-4-yl)ethyl)-1H-imidazo[4,5-c]quinolin-4-amine. Compound 8 (70 mg, 0.240 mmol) and 1-azido-4-isopropylbenzene (39 mg, 0.288 mmol) were reacted together following the above general procedure to give the product in good yield (105 mg, 97%) as white solid; melting point: 224–226 °C; 1H NMR (400 MHz, CDCl3) δ 7.88 (dd, J = 8.3, 1.0 Hz, 1H), 7.82 (dd, J = 8.4, 1.1 Hz, 1H), 7.71 (s, 1H), 7.57–7.53 (m, 2H), 7.50 (ddd, J = 8.4, 7.1, 1.3 Hz, 1H), 7.35–7.29 (m, 3H), 5.56 (s, 2H), 4.24 (d, J = 7.6 Hz, 2H), 3.48 (dd, J = 11.1, 4.6 Hz, 2H), 3.41–3.36 (m, 2H), 3.01–2.90 (m, 1H), 2.39–2.29 (m, 1H), 1.26 (d, J = 6.9 Hz, 6H), 0.99 (d, J = 6.7 Hz, 6H). 13C NMR (101 MHz, CDCl3) δ 153.01, 151.04, 149.86, 146.89, 143.54, 135.07, 133.88, 127.79, 127.43, 126.77, 126.53, 122.74, 120.67, 120.06, 119.99, 115.43, 52.74, 33.95, 29.36, 27.46, 24.02, 23.78, 19.84, HRMS: calc. for C27H31N7, m/z 453.264, found 454.269 [M + H]+.
Synthesis of compound 9d: 2-(2-(1-(4-(tert-butyl)phenyl)-1H-1,2,3-triazol-4-yl)ethyl)-1-isobutyl-1H-imidazo[4,5-c]quinolin-4-amine. Compound 8 (70 mg, 0.240 mmol) and 1-azido-4-(tert-butyl)benzene (51 mg, 0.288 mmol) were reacted according to the above general procedure to afford the product in good yield (107 mg, 96%) as white solid; melting point: 220–221 °C; 1H NMR (400 MHz, CDCl3) δ 7.85 (ddd, J = 12.7, 8.3, 0.9 Hz, 2H), 7.73 (s, 1H), 7.58–7.53 (m, 2H), 7.53–7.45 (m, 3H), 7.32 (ddd, J = 8.2, 7.1, 1.2 Hz, 1H), 5.95 (s, 2H), 4.24 (d, J = 7.6 Hz, 2H), 3.48 (dd, J = 11.0, 4.4 Hz, 2H), 3.38 (dd, J = 11.2, 4.6 Hz, 2H), 2.38–2.27 (m, 1H), 1.33 (s, 9H), 0.98 (d, J = 6.7 Hz, 6H). 13C NMR (101 MHz, CDCl3) δ 153.07, 152.02, 151.04, 146.83, 143.01, 134.67, 133.85, 127.44, 126.64, 126.01, 122.73, 120.19, 120.06, 119.97, 115.19, 52.65, 34.82, 31.33, 29.28, 27.39, 23.62, 19.77, HRMS: calc. for C28H33N7, m/z 467.279, found 468.286 [M + H]+.
Synthesis of compound 9e: 1-isobutyl-2-(2-(1-(4-nitrophenyl)-1H-1,2,3-triazol-4-yl)ethyl)-1H-imidazo[4,5-c]quinolin-4-amine. Compound 8 (70 mg, 0.240 mmol) and 1-azido-4-nitrobenzene (47 mg, 0.288 mmol) reacted together according to the above general procedure to afford the product in good yield (96 mg, 88%) as light yellow solid; melting point: 190–191 °C; 1H NMR (400 MHz, CDCl3) δ 8.70–8.60 (m, 1H), 8.36 (d, J = 10.2 Hz, 1H), 8.26 (d, J = 8.2 Hz, 1H), 8.15 (d, J = 8.1 Hz, 1H), 7.93 (d, J = 8.5 Hz, 1H), 7.83–7.72 (m, 2H), 7.59 (q, J = 8.3 Hz, 1H), 7.48–7.43 (m, 1H), 4.33 (s, 2H), 3.51–3.38 (m, 4H), 2.34–2.23 (m, 1H), 1.01 (d, J = 6.6 Hz, 6H). 13C NMR (101 MHz, CDCl3) δ 155.35, 153.63, 149.37, 147.80, 134.77, 133.55, 132.80, 131.48, 128.46, 126.22, 124.68, 123.67, 123.57, 121.10, 120.73, 115.65, 53.04, 29.62, 27.38, 23.35, 19.81, HRMS: calc. for C24H24N8O2, m/z 456.202, found 457.209 [M + H]+.
Synthesis of compound 9f: 1-isobutyl-2-(2-(1-(4-methoxyphenyl)-1H-1,2,3-triazol-4-yl)ethyl)-1H-imidazo[4,5-c]quinolin-4-amine. Compound 8 (100 mg, 0.342 mmol) and 1-azido-4-methoxybenzene (61 mg, 0.410 mmol) were reacted together according to the above general procedure to obtain the product in good yield (140 mg, 93%). White solid; melting point: 179–180 °C; 1H NMR (400 MHz, CDCl3 + CD3OD) δ 8.17 (s, 1H), 7.98 (d, J = 8.3 Hz, 1H), 7.70 (dd, J = 8.4, 1.1 Hz, 1H), 7.65–7.62 (m, 2H), 7.50 (ddd, J = 8.4, 7.1, 1.3 Hz, 1H), 7.37–7.32 (m, 1H), 7.06–7.02 (m, 2H), 4.35 (d, J = 7.6 Hz, 2H), 3.84 (s, 3H), 3.42 (t, J = 2.8 Hz, 4H), 2.35–2.27 (m, 1H), 1.00 (d, J = 6.7 Hz, 6H). 13C NMR (101 MHz, CDCl3 + CD3OD) δ 159.68, 152.64, 150.84, 146.29, 143.31, 133.68, 130.19, 127.36, 126.00, 125.73, 122.45, 121.98, 120.22, 119.81, 114.77, 114.62, 55.45, 52.43, 29.00, 27.04, 23.18, 19.41. HRMS: calc. for C25H27N7O, m/z 441.227, found 442.234 [M + H]+.
Synthesis of compound 9g: 2-(2-(1-(4-chlorophenyl)-1H-1,2,3-triazol-4-yl)ethyl)-1-isobutyl-1H-imidazo[4,5-c]quinolin-4-amine. Compound 8 (100 mg, 0.342 mmol) and 1-azido-4-chlorobenzene (63 mg, 0.410 mmol) were reacted according to the above general procedure to give the product in good yield (129 mg, 85%) as white solid; melting point: 188–190 °C; 1H NMR (400 MHz, CDCl3 + CD3OD) δ 7.91–7.81 (m, 2H), 7.75 (s, 1H), 7.64–7.58 (m, 2H), 7.57–7.48 (m, 3H), 7.35–7.30 (m, 1H), 5.70 (s, 2H), 4.25 (d, J = 7.6 Hz, 2H), 3.48 (t, J = 6.7 Hz, 2H), 3.38 (dd, J = 10.8, 4.1 Hz, 2H), 2.39–2.29 (m, 1H), 0.99 (d, J = 6.7 Hz, 6H). 13C NMR (101 MHz, CDCl3 + CD3OD) δ 156.22, 154.09, 152.07, 147.99, 143.81, 136.52, 135.30, 134.99, 130.67, 128.45, 126.89, 125.78, 123.60, 122.49, 121.66, 121.23, 115.71, 53.26, 30.08, 27.95, 23.90, 19.84. HRMS: calc. for C24H24ClN7, m/z 445.178, found 446.185 [M + H]+.
Synthesis of compound 9h: 1-isobutyl-2-(2-(1-(3-nitrophenyl)-1H-1,2,3-triazol-4-yl)ethyl)-1H-imidazo[4,5-c]quinolin-4-amine. Compound 8 (70 mg, 0.240 mmol) and 1-azido-3-nitrobenzene (47 mg, 0.288 mmol) were reacted according to the above general procedure to afford the product in good yield (100 mg, 92%) as light yellow solid; melting point: 196–197 °C; 1H NMR (400 MHz, DMSO) δ 8.96 (s, 1H), 8.68 (t, J = 2.1 Hz, 1H), 8.37 (ddd, J = 8.1, 2.1, 0.8 Hz, 1H), 8.31 (ddd, J = 8.3, 2.2, 0.8 Hz, 1H), 8.00 (d, J = 8.0 Hz, 1H), 7.90 (t, J = 8.2 Hz, 1H), 7.63 (d, J = 8.1 Hz, 1H), 7.44 (t, J = 7.2 Hz, 1H), 7.29 (t, J = 7.1 Hz, 1H), 6.72 (s, 2H), 4.37 (d, J = 7.4 Hz, 2H), 3.17 (d, J = 3.7 Hz, 2H), 2.24–2.13 (m, 1H), 0.94 (d, J = 6.6 Hz, 6H). 13C NMR (101 MHz, DMSO) δ 152.59, 151.45, 148.60, 147.59, 137.34, 132.81, 131.64, 126.66, 125.87, 125.71, 122.95, 121.53, 121.45, 120.41, 114.72, 114.48, 51.51, 28.91, 26.49, 23.18, 19.25. HRMS: calc. for C24H24N8O2, m/z 456.202, found 457.209 [M + H]+.
Synthesis of compound 9i: 1-isobutyl-2-(2-(1-(3-methoxyphenyl)-1H-1,2,3-triazol-4-yl)ethyl)-1H-imidazo[4,5-c]quinolin-4-amine. Compound 8 (100 mg, 0.342 mmol) and 1-azido-3-methoxybenzene (61 mg, 0.410 mmol) were reacted according to the above general procedure to get the product in good yield (139 mg, 92%) as white solid; melting point: 188–190 °C; 1H NMR (400 MHz, CDCl3) δ 7.89 (d, J = 8.6 Hz, 2H), 7.81 (s, 1H), 7.59–7.51 (m, 1H), 7.42–7.34 (m, 2H), 7.28 (t, J = 2.2 Hz, 1H), 7.18 (ddd, J = 8.0, 2.0, 0.8 Hz, 1H), 6.93 (ddd, J = 8.4, 2.5, 0.8 Hz, 1H), 4.29 (d, J = 7.6 Hz, 2H), 3.85 (s, 3H), 3.49–3.41 (m, 4H), 2.38–2.26 (m, 1H), 1.01 (d, J = 6.7 Hz, 6H). 13C NMR (101 MHz, CDCl3) δ 159.84, 152.82, 151.16, 146.87, 144.13, 133.75, 130.63, 127.27, 126.87, 122.54, 122.21, 120.14, 120.00, 115.54, 114.85, 55.74, 52.69, 29.33, 27.46, 23.75, 19.82. HRMS: calc. for C25H27N7O, m/z 441.227, found 442.234 [M + H]+.
Synthesis of compound 9j: 1-isobutyl-2-(2-(1-(2-methoxyphenyl)-1H-1,2,3-triazol-4-yl)ethyl)-1H-imidazo[4,5-c]quinolin-4-amine. Compound 8 (100 mg, 0.342 mmol) and 1-azido-2-methoxybenzene (61 mg, 0.410 mmol) according to the above general procedure to afford the product in good yield (136 mg, 90%) as white solid; melting point: 182–184 °C; 1H NMR (400 MHz, CDCl3) δ 7.92–7.87 (m, 2H), 7.86 (s, 1H), 7.72 (dd, J = 7.9, 1.7 Hz, 1H), 7.57–7.51 (m, 1H), 7.41–7.36 (m, 2H), 7.09–7.00 (m, 2H), 6.60 (s, 2H), 4.24 (d, J = 7.6 Hz, 2H), 3.71 (s, 3H), 3.44 (dd, J = 15.2, 6.2 Hz, 4H), 2.36–2.25 (m, 1H), 1.00 (d, J = 6.7 Hz, 6H). 13C NMR (101 MHz, CDCl3) δ 154.26, 151.13, 150.43, 145.39, 139.86, 134.41, 130.15, 128.28, 126.37, 126.09, 125.45, 124.14, 123.98, 123.74, 121.33, 120.31, 114.53, 112.36, 55.96, 52.79, 29.38, 27.51, 23.77, 19.81, HRMS: calc. for C25H27N7O, m/z 441.227, found 442.234 [M + H]+.
Synthesis of compound 9k: 2-(2-(1-benzyl-1H-1,2,3-triazol-4-yl)ethyl)-1-isobutyl-1H-imidazo[4,5-c]quinolin-4-amine. Compound 8 (100 mg, 0.342 mmol) and (azidomethyl)benzene (55 mg, 0.410 mmol) were reacted as per the above general procedure to afford the product in good yield (135 mg, 93%) as yellow solid; melting point: 187–189 °C; 1H NMR (400 MHz, CD3OD) δ 7.94 (d, J = 8.3 Hz, 1H), 7.71 (d, J = 6.2 Hz, 2H), 7.56–7.50 (m, 1H), 7.41–7.35 (m, 1H), 7.24–7.15 (m, 5H), 5.49 (s, 2H), 4.19 (d, J = 7.6 Hz, 2H), 3.36–3.30 (m, 4H), 2.24–2.13 (m, 1H), 0.91 (d, J = 6.7 Hz, 6H). 13C NMR (101 MHz, CD3OD) δ 155.11, 152.23, 147.68, 142.93, 136.77, 135.31, 129.92, 129.48, 128.98, 126.98, 125.07, 124.15, 123.98, 121.90, 115.76, 54.83, 53.29, 30.42, 28.32, 24.28, 19.65, HRMS: calc. for C25H27N7, 425.232, m/z found 426.240 [M + H]+.
Synthesis of compound 9l: 3-(4-(2-(4-amino-1-isobutyl-1H-imidazo[4,5-c]quinolin-2-yl)ethyl)-1H-1,2,3-triazol-1-yl)propane-1,2-diol. Compound 8 (100 mg, 0.342 mmol) and 3-azidopropane-1,2-diol (48 mg, 0.410 mmol) were allowed to react according to the above general procedure given above to form the product in good yield (127 mg, 91%) as white solid; melting point: 178–179 °C; 1H NMR (400 MHz, CD3OD) δ 8.17 (d, J = 8.3 Hz, 1H), 7.87 (s, 1H), 7.78–7.69 (m, 2H), 7.62 (t, J = 7.6 Hz, 1H), 4.53 (dd, J = 14.0, 3.7 Hz, 1H), 4.41 (d, J = 7.5 Hz, 2H), 4.34 (dd, J = 14.0, 7.8 Hz, 1H), 4.00–3.92 (m, 1H), 3.49 (dd, J = 5.4, 2.7 Hz, 2H), 3.40 (dd, J = 9.0, 4.9 Hz, 4H), 2.31–2.19 (m, 1H), 1.01 (d, J = 6.6 Hz, 6H). 13C NMR (101 MHz, CD3OD) δ 157.49, 150.41, 137.10, 135.23, 130.89, 126.61, 125.97, 125.29, 123.02, 119.73, 116.78, 114.54, 71.93, 64.50, 54.18, 53.51, 30.56, 28.44, 23.62, 19.68. HRMS: calc. for C21H27N7O2, m/z 409.222, found 410.229 [M + H]+.
Synthesis of compound 9m: 8-(4-(4-(2-(4-amino-1-isobutyl-1H-imidazo[4,5-c]quinolin-2-yl)ethyl)-1H-1,2,3-triazol-1-yl)phenyl)octan-1-ol. Compound 8 (100 mg, 0.342 mmol) and 8-azidooctan-1-ol (70 mg, 0.410 mmol) were reacted according to the above general procedure to afford the product in good yield (142 mg, 90%) as white solid; melting point: 188–190 °C; 1H NMR (400 MHz, CDCl3 + CD3OD) δ 7.99 (d, J = 8.1 Hz, 1H), 7.74–7.69 (m, 2H), 7.55–7.50 (m, 1H), 7.41–7.36 (m, 1H), 4.31 (dd, J = 9.5, 4.4 Hz, 4H), 3.49 (t, J = 6.7 Hz, 2H), 3.35 (s, 4H), 2.32–2.21 (m, 1H), 1.85–1.77 (m, 2H), 1.45–1.41 (m, 2H), 1.25–1.13 (m, 8H), 0.98 (d, J = 6.7 Hz, 6H). 13C NMR (101 MHz, CDCl3 + CD3OD) δ 154.90, 151.97, 147.05, 142.48, 135.30, 128.91, 126.82, 124.82, 124.14, 123.70, 121.63, 115.57, 62.79, 53.28, 51.13, 33.33, 31.07, 30.27, 30.10, 29.79, 28.24, 27.19, 26.58, 23.96, 19.77, HRMS: calc. for C26H37N7O, m/z 463.630, found 464.312 [M + H]+.
Molecular docking studies
The crystal structure of high resolution TLR7 heterodimers with a tri acylated lipopeptides (Pam3CysSK4) was downloaded from Protein Data Bank (PDB ID: 6LW0). The resolution is 2.60 Å. The docking study with the native ligand and the 9a–m molecules was performed using Schrodinger suite 2016 software. All the crystallographic water molecules and other small molecules were removed. Hydrogen atoms were added using OLPS. Then the docking protocol file was generated using the Glide-Dock module using SP mode. The active site was detected using the ligand bound protein ligand complex. The active site was confirmed with reference to the ligand, which was prepared using the Ligprep option. The native ligand for docking was extracted from the complex structure retrieved from Protein Data Bank. The molecular structures of 9a–m were prepared using Chemdraw 3D software and initially all the prepared molecules were minimized using the MMFF protocol. Three conformations with the top-ranking scores for molecules were saved for further study. Generation of an accurate complex structure for 9a–m of the synthesized ligands showed activity but due to large hydrophobic pockets the docking in an existing (rigid) structure of the receptor induces higher standard deviation from the experimental data. Thus, rescue of false negatives (poorly scored true binders) in virtual screening experiments, where instead of screening against a single conformation of the receptor, additional conformations obtained with the induced fit protocol are used. We performed docking of the designed ligands with TLR7.
HEK TLR7/8 reporter assay
HEK-Blue hTLR7/8reporter cells were cultured in low glucose DMEM. For TLR7/8 reporter assay, 2.5 × 104 cells were accumulated in HEK-Blue™ selection medium and incubated with 20 μg of test compounds 9a–m and standard imiquimod at 37 °C for 24 hours with 5% CO2. Secreted embryonic alkaline phosphatase (SEAP) was evaluated by HEK-Blue detection method. After 24 hours incubation, the SEAP can be read at 650 nm.19
Hemolysis
All the analogues were evaluated for their hemolytic activity using 1% RBC solution with blood collected from mice. Briefly, mouse blood was collected in a vacutainer and then washed using PBS to get RBC pellet. The pellet was re-suspended in PBS at 1% concentration. All the compounds were added in 96 well plate at a concentration of 100 μg and RBC solution (100 μl) was added and incubated at RT for 30 min. After incubation the plate was centrifuged at 2000 rpm for 10 min and the supernatant was carefully collected and scanned at 520 nm using a multimode reader to determine the absorbance values. De-ionized water was taken as positive control that is capable of causing 100% hemolysis and the % hemolysis of the compounds was back calculated from the positive control.
Cytotoxicity
The cytotoxicity effect of novel imidazoquinoline analogues was evaluated by incubating them with splenocytes isolated from mouse. Briefly, mouse splenocytes were harvested and incubated with novel analogues at a concentration of 100 μg for 24 h at 37 °C after incubation cells were added with 20 μl of MTT reagent (5 mg ml−1) and further incubated for 3 h then the plate was centrifuged at 2000 rpm for 5 min and 100 μl supernatant was replaced with DMSO to transform the MTT reagent to formazine crystals. The plate was incubated for 15 min at RT and then scanned at 630 nm using a multimode reader and the % viability of the analogues treated cells was calculated by considering untreated cells (cell control) as 100% viability.
Mouse DC isolation and stimulation
2 × 104 cells per well were seeded in 96 well plates and incubated with 1, 10 and 100 μg of test compounds and standard imiquimod at 37 °C for 48 hours with 5% CO2. After completion of incubation, sandwich ELISA was implemented to quantify IL-6, TNF-α, IL-12 a Th1 cytokine prime indicator for stimulated DC and CD40, 80 and 86 markers using FACS.20
Human PBMC culture and treatment
hPBMC were acquired from Lonza commercially and were cultured according to the suppliers recommendations. 1 × 105 cells per well were seeded in 96 well plate and were stimulated with the novel imidazoquinoline analogues at three different concentrations. After 24 h incubation supernatant was collected and cytokines (IFN-γ and IL-12) were quantified using sandwich ELISA method. Total T cell population enhancement after treatment was also quantified using anti human CD3 marker with flowcytometric analysis.21
Results and discussion
Chemistry
The synthesis of target triazolyl imidazoquinoline was envisaged through the dipolar cycloaddition of the acetylene intermediate 8 with appropriate azide following standard click chemistry protocol. The intermediate alkyne was prepared in eight steps starting from the commercially available 3-nitroquinolin-4-ol (Scheme 1). Chlorination of 3-nitroquinolin-4-ol using POCl3 under reflux conditions gave the corresponding chloro intermediate (2) which as subjected to condensation of isobutyl amine under CH2Cl2 reflux conditions in the presence of trimethylamine. Construction of N-isobutyl, 3-butylated imidazoquinoline was carried out in good yields via the initial reduction of intermediate nitro-compound under hydrogenolysis conditions using H2, Pd/C in ethyl acetate followed by concomitant cyclization of the intermediate amino compound with 4-pentynoic acid in presence of HBTU, Et3N, DMAP, DMF at 90 °C to afford acetylenic intermediate compound 5 in good yields (72%). Compound 5 upon treatment with mCPBA in CH2Cl2 at 45 °C, underwent N-oxidation (compound 6) which on further reaction with benzoyl isocyanate, in CH2Cl2 at 45 °C gave compound 7 (73%). Hydrolytic cleavage of amide 7 using NaOCH3 in MeOH at 65 °C afforded the desired acetylenic intermediate compound 8 (65%) useful for click chemistry with appropriate azide moieties. Final construct was assembled following triazolyl click chemistry protocol to afford triazolyl imidazoquinolines in high yields (Scheme 2). All the final compounds and their intermediates were purified by column chromatography on silica gel using appropriate eluent and characterized spectroscopically by 1H, 13C NMR and mass spectral analysis. The structure of the final constructs along with their yields and melting points are provided in the Scheme 2. Additional information regarding the synthetic methods and compound characterization data is provided in the online ESI.†
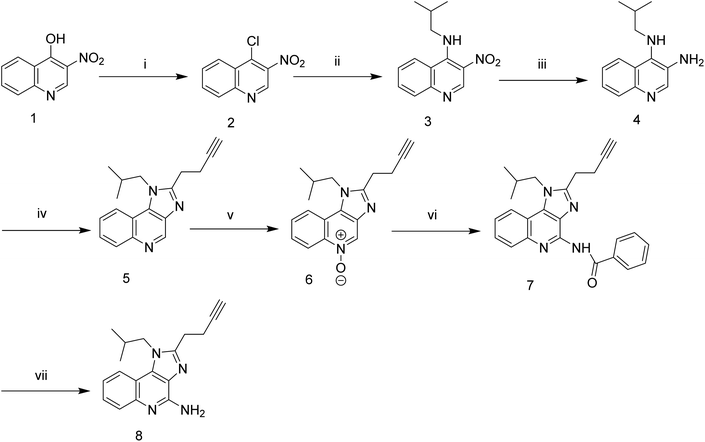 |
| Scheme 1 Reagents and conditions: (i) POCl3, DMF, reflux; (ii) isobutylamine, Et3N, DCM, reflux 45 °C; (iii) Pd/C, H2, Na2SO4, EtOAc; (iv) 4-pentynoic acid, HBTU, Et3N, DMAP, DMF, 90 °C; (v) m-CPBA, DCM, CHCl3, MeOH, 45 °C; (vi) benzoyl isocyanate, DCM, 45 °C; (vii) NaOCH3, MeOH, 65 °C. | |
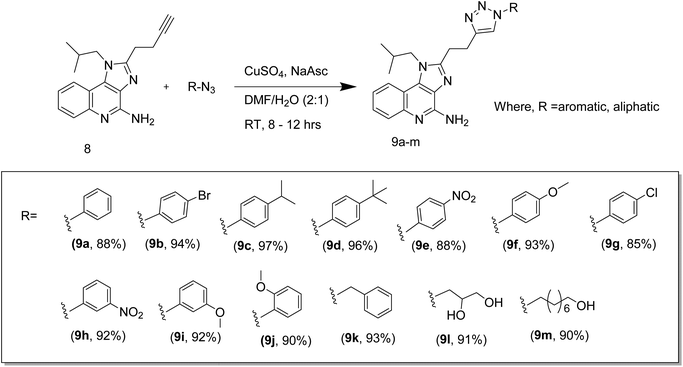 |
| Scheme 2 | |
All the above novel imidazoquinoline analogues were synthesized, purified and characterized using various analytical techniques for determining their mass, structure and purity. All the compounds taken for biological activity had ≥95% purity as determined by HPLC.
In silico studies
The docking showed that all the ligands are effectively forming TLR7 complex as it is clearly evident from their XP score. The residues 264, 351, 356, 381, 408, 506, 507, 528, 557, 579, 585 (as hydrophobic pocket) and 356, 410, 432, 434, 408, 555, 586 (hydrogen bonding sites) were selected for the Prime Refinement stage. Hydrogen bond forming amino acid residues were considered to be most crucial for the docking. Total 2009 binding poses were analysed for the best ligand 9k, 9c, 9h, 9e, 9j, 9i and 9g out of the designed ligand set it has been found that in 9k, 9h, 9j showed interactions Lys432 and Lys410 with triazolyl centre 9d, 9g and 9m showed interactions Lys432 with triazolyl centre (Fig. 1). Almost all molecules have been revealed hydrophobic interaction with Phe351, Phe408. Pi–cation bond forming amino acid residues was showed interaction with Lys432 and Lys410 of 9a, 9c, 9d, 9f, 9g, 9j, 9k, 9m. Pi–pi stacking also showed with the cyclic aromatic ring of all synthesised compounds. The MM/PBSA studies disclosed that 9k and 9i interacted TLR7 complex with ΔGbinding −3056.79 and −4135.43 kcal mol−1 though the values are lower than the standard ligand which showed very high ΔGbinding of −1157 kcal mol−1 due to the high number of hydrogen bonding site offering more stable protein–ligand complex (Table 1).
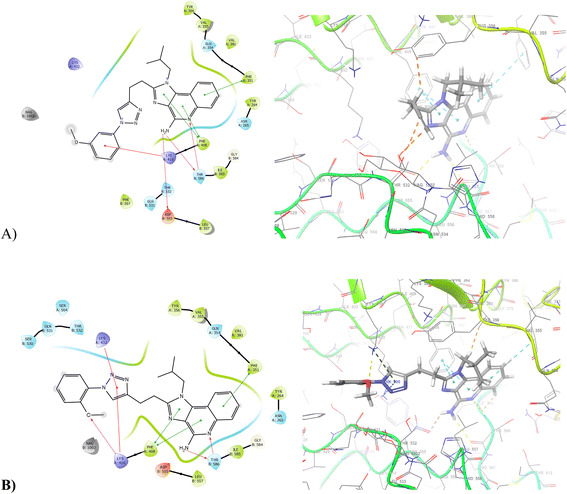 |
| Fig. 1 Representative dockings of 9i and 9j with TLR7 receptor. | |
Table 1 Molecular docking score and binding interaction residues
Compound |
Docking score (PDB: 3LBL) |
Interaction |
9a |
−11.78 |
Lys410, Lys432, Phe408, Thr586, Phe351 |
9b |
−12.59 |
Phe408, Lys432, Lys410, Ser434, Leu528, Asp555, Thr586 |
9c |
−9.64 |
Lys410, Lys432, Phe408, Thr586, Phe351 |
9d |
−10.61 |
Lys432, Lys410, Asp555, Thr586, Phe408, Phe351 |
9e |
−10.61 |
Ser434, Lys432, Lys410, Phe408, Thr586, Tyr386, Phe351 |
9f |
−10.28 |
Lys410, Asp555, Phe408, Thr586, Phe351 |
9g |
−10.91 |
Lys410, Phe408, Asp555, Thr586, Phe351, Lys432 |
9h |
−8.34 |
Phe408, Phe351, Tyr356, Thr586 |
9i |
−10.73 |
Lys410, Asp555, Thr586, Phe408, Phe351 |
9j |
−11.54 |
Phe351, Phe408, Lys410, Asp555, Thr586 |
9k |
−10.67 |
Lys410, Asp555, Phe408, Thr586, Phe351 |
9l |
−10.22 |
Lys410, Phe408, Ile586, Asp555, Phe351 |
9m |
−10.62 |
Lys432, Phe351, Phe408, Thr586, Asp555, Lys410 |
Immunological evaluation
Hemolysis and cytotoxicity study. All the novel imidazoquinoline analogs synthesized were primarily evaluated for determining their toxicity before performing any cell based assays and cytotoxicity assay on splenocytes revealed the non toxic nature of these novel analogues as shown in Fig. S1.†22 The analogues in turn have aided in proliferation of splenocytes suggesting their non toxic nature towards the cells. Hemolytic assay of the novel analogues on murine RBCs revealed non-hemolytic nature of these compounds.
HTLR assay. After synthesizing the C2 substituted imidazoquinoline analogues their ability to target the TLR7/8 receptors was evaluated using transfected cell lines by measuring the SEAP secreted in to the media. From the ligand binding assay as shown in Table 2 we can infer that all the molecules had higher binding affinity to TLR7 receptors in a dose dependent manner in particular the analogues 9f, 9i, 9j had the lowest EC50 values, where as the molecule 9m which had an octanol substitution elicited a profound binding efficacy to TLR8 receptor. This variation in binding may be due to the chain length where in any substitution beyond butyl had an affinity to TLR8 as stated by Charles et al.23 This relative variation in activity may be due to the tolerating effect of TLR7 to modification at N1 and C2 which are apparently not required for activity, however, substitutions at these particular positions typically enhances the potency.24 The C4 amine position which is required for activity was kept intact as any changes at this site would result in loss of activity. The other analogues had also elicited a TLR7 specific activity but relatively at higher concentrations than the standard.
Table 2 EC 50 values of novel analogues to TLR7 receptor transfected HEK cell lines using SEAP quantification
Analogue |
EC 50 (μg ml−1) |
EC 50 (μM) |
9a |
2.327 ± 0.44 |
5.6481 |
9b |
2.713 ± 0.34 |
5.51 |
9c |
2.996 ± 0.17 |
6.5859 |
9d |
2.655 ± 0.84 |
5.6731 |
9e |
2.195 ± 0.91 |
4.8031 |
9f |
0.452 ± 0.01 |
1.0226 |
9g |
3.521 ± 0.72 |
7.8946 |
9h |
2.390 ± 0.77 |
5.2298 |
9i |
0.366 ± 0.24 |
0.828 |
9j |
0.384 ± 0.22 |
0.868 |
9k |
3.442 ± 0.82 |
8.0751 |
9l |
2.718 ± 0.57 |
6.6015 |
9m |
3.515 ± 0.54 |
7.5754 |
Imiquimod |
0.361 ± 0.07 |
1.5042 |
In vitro. All the analogues (9a–m) were initially screened for their immune modulating ability using DC activation assay where in cells were treated with various concentrations of compounds and pro inflammatory cytokines TNF-α, IL-6 were estimated from the supernatant. From the Fig. 2 we can infer that compounds 9a, 9f, 9i, 9j and 9m analogues have elicited significant levels of both the cytokines which indicate the ability of molecules to activate the innate immune system. Additionally the capability of these analogues to activate the dendritic cells-barrier between innate and adaptive immunity was also evaluated by quantifying costimulatory markers like CD40, 80 and 86 along with IL-12 and IFN-γ cytokines. Antigen presenting cells (APCs) derived from dendritic cells play crucial role in the antigen presentation to T cells (CD4+, CD8+), expressing the complimentary ligands to co-stimulatory receptors on T cells, and release of vital stimulatory cytokines. Murine DCs enable the possibility to directly measure of response specific to immune cells upon its activation by TLR agonists and thus provide a well established model for cell based assay to quantify cytokine induction. From the graph, it is evident that all above molecules which have shown significant cytokine response were able to elicit a potent DC activation in comparison to standard Imiquimod. From this preliminary data, comparing the structure and activity, we can get a gross idea that in compound 9f, 9i and 9j the phenyl methoxy substitution at all the three positions like ortho, meta and para at C2 have enhanced the activity of the molecule significantly and in addition in compound 9m the octanol substitution at same position has also elicited an enhanced activity as reported by Shukla et al.8 From the above preliminary data as shown in Fig. 2 and S1† we can get an idea regarding the immunostimulatory properties of these novel analogues which were able to activate the immune system precursors and also our basic hypothesis of modification at C2 position using various aromatic entities with a triazole moiety had a significant impact on the immune enhancement.
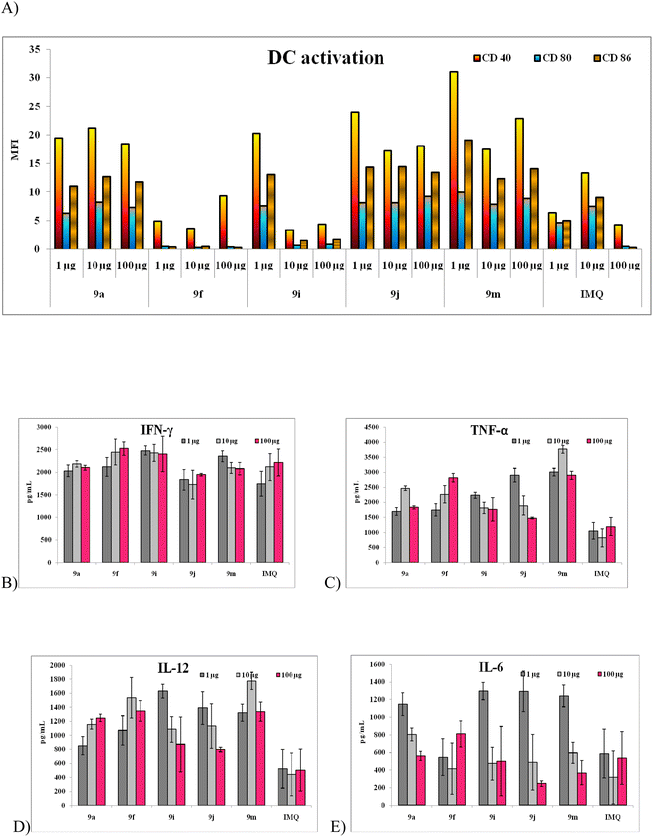 |
| Fig. 2 (A) Cell surface marker and (B–E) cytokines quantification from stimulated DCs. | |
With the above preliminary data we have further evaluated the adjuvant ability of these molecules using human PBMC assays wherein all the analogues at three different concentrations were used to treat the cells and cytokines like IFN-γ and IL-12 were evaluated from the supernatant and CD3 marker was used to quantify the total lymphocyte population. The stimulation of cytokines production from numerous immune cell types, viz., DCs, B cells, monocytes natural killer (NK) cells and T cells, is revealed through this assay. The most active compounds recognized using DCs are the effective stimulants of PBMCs as well (e.g., the C2-octanol, 9m) and the methoxy phenol substituted derivatives 9f–j. IFN-γ, is mainly produced by NK and T cells, mediates by up-regulation of antigen specific responses in the immune system and by activating macrophages. The data pertaining IFN-γ is in coherence with the trends seen in structure-based studies. From the Fig. 3 and S2,† we can infer that all the analogues which were active in macrophage treatment were also potent in human PBMCs but all the analogues 9f, 9i and 9j had a significant response in comparison to imiquimod and other imidazoquinoline analogues.
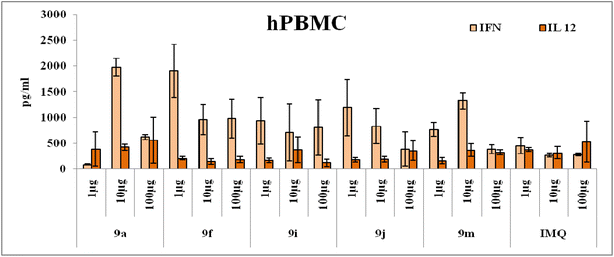 |
| Fig. 3 IFN-γ and IL-12 cytokine quantification from hPBMC supernatant. | |
From the above data it is evident that the C2 position modification of the imidazoquinoline molecules had not altered its activity but in turn, has enhanced its potency in terms of IFN-γ production and target specificity.11 The triazole moiety offered flexibility in positioning various aromatic substituent's at the C2 position as hypothesized and has greatly enhance the solubility.25 Different aromatic substitutions at various positions like ortho, meta and para had varying activities depending upon the concentration of the molecules. A major trend of activity was observed at lower concentration of 1 and 10 μg for most of the analogues. And the most interesting point noted in the whole series was that all the electron donating groups like 9f, 9i and 9j had elicited a significant activity in comparison to the imiquimod whereas the electron with drawing group substituted analogues had relatively minimal immune-stimulatory activity.
Conclusion
To sum up, the novel imiquimod analogues exhibit potential immune modulator properties arising out of their ability to bind to TLR7/8 and stimulating the DCs, hPBMCs. The structure–activity relation between various substitutions at C2 positions using a triazole linker was derived taking into consideration of the above in vitro data. Overall the novel imiquimod analogues induce superior immune response with Th1 bias qualitatively and quantitatively, which is desirable to protect from the intracellular pathogenesis like viral infections such as SARS COV-2 wherein a potent cellular response in addition to antibodies is essential to develop prophylactic vaccine against viral infections.
Statistical analysis
All statistical analyses were performed using GraphPad Prism, version for Windows (GraphPad Software, La Jolla, CA, USA). The differences of cytokine levels were evaluated by the test and difference between groups were evaluated by two-tailed Student's t-test and p < 0.05 was considered to represent a significant difference. All data are shown as mean ± SEM.
Conflicts of interest
There are no conflicts to declare.
Acknowledgements
The financial support from DBT (GAP 0872), for the India-EU collaborative project on Influenza vaccine (INDIGO), is gratefully acknowledged. AA is thankful to UGC New Delhi for the award of Junior Research Fellowship. SS thanks DST, India for the award of Inspire Senior Research Fellowship. Biorender software is acknowledged for the graphics used to generate the graphical abstract. IICT manuscript number; IICT/Pubs./2022/302.
References
- J. P. Vasilakos and M. A. Tomai, The use of Toll-like receptor 7/8 agonists as vaccine adjuvants, Expert Rev. Vaccines, 2013, 12(7), 809–819 CrossRef CAS PubMed.
- J. K. Dowling and A. Mansell, Toll-like receptors: the swiss army knife of immunity and vaccine development, Clin. Transl. Immunol., 2016, 5(5), e85 CrossRef PubMed.
- M. Luchner, S. Reinke and A. Milicic, TLR agonists as vaccine adjuvants targeting cancer and infectious diseases, Pharmaceutics, 2021, 13(2), 142 CrossRef CAS PubMed.
- D. B. Salunke, E. Yoo, N. M. Shukla, R. Balakrishna, S. S. Malladi, K. J. Serafin, V. W. Day, X. Wang and S. A. David, Structure–activity relationships in human Toll-like receptor 8-active 2, 3-diamino-furo [2, 3-c] pyridines, J. Med. Chem., 2012, 55(18), 8137–8151 CrossRef CAS PubMed.
- D. Kaushik, A. Kaur, N. Petrovsky and D. B. Salunke, Structural evolution of toll-like receptor 7/8 agonists from imidazoquinolines to imidazoles, RSC Med. Chem., 2021, 12, 1065–1120 RSC.
- D. Kaushik, S. Dhingra, M. T. Patil, S. Piplani, V. Khanna, Y. Honda-Okubo, L. Li, J. Fung, I. G. Sakala and D. B. Salunke, BBIQ, a pure TLR7 agonist, is an effective influenza vaccine adjuvant, Hum. Vaccines Immunother., 2020, 16(8), 1989–1996 CrossRef CAS PubMed.
- B. Pulendran, P. S Arunachalam and D. T. O'Hagan, Emerging concepts in the science of vaccine adjuvants, Nat. Rev. Drug Discovery, 2021, 20(6), 454–475 CrossRef CAS PubMed.
- N. M. Shukla, S. S. Malladi, C. A. Mutz, R. Balakrishna and S. A. David, Structure− activity relationships in human Toll-like receptor 7-active imidazoquinoline analogues, J. Med. Chem., 2010, 53(11), 4450–4465 CrossRef CAS PubMed.
- S. Khoshnood, M. Arshadi, S. Akrami, M. Koupaei, H. Ghahramanpour, A. Shariati, N. Sadeghifard and M. Heidary, An overview on inactivated and live-attenuated SARS-CoV-2 vaccines, J. Clin. Lab. Anal., 2022, 36(5), e24418 CrossRef CAS PubMed.
- V. Algieri, C. Algieri, L. Maiuolo, A. De Nino, A. Pagliarani, M. A. Tallarida, F. Trombetti and S. Nesci, 1, 5-Disubstituted-1, 2, 3-triazoles as inhibitors of the mitochondrial Ca2+-activated F1FO-ATP (hydrol) ase and the permeability transition pore, Ann. N. Y. Acad. Sci., 2021, 1485(1), 43–55 CrossRef CAS PubMed.
- J. F. Gerster, K. J. Lindstrom, R. L. Miller, M. A. Tomai, W. Birmachu, S. N. Bomersine, S. J. Gibson, L. M. Imbertson, J. R. Jacobson and R. T. Knafla, Synthesis and structure− activity-relationships of 1 H-imidazo [4, 5-c] quinolines that induce interferon production, J. Med. Chem., 2005, 48(10), 3481–3491 CrossRef CAS PubMed.
- N. M. Shukla, M. R. Kimbrell, S. S. Malladi and S. A. David, Regioisomerism-dependent TLR7 agonism and antagonism in an imidazoquinoline, Bioorg. Med. Chem. Lett., 2009, 19(8), 2211–2214 CrossRef CAS PubMed.
- R. Saroa, D. Kaushik, U. Bagai, S. Kaur and D. B. Salunke, Efficacy of TLR7 agonistic imidazoquinoline as immunochemotherapeutic agent against P. berghei ANKA infected rodent host, Bioorg. Med. Chem. Lett., 2019, 29(9), 1099–1105 CrossRef CAS PubMed.
-
(a) Q. Liu and Y. Tor, Simple conversion of aromatic amines into azides, Org. Lett., 2003, 5(14), 2571–2572 CrossRef CAS PubMed;
(b) P. S. Gribanov, M. A. Topchiy, Y. D. Golenko, Y. I. Lichtenstein, A. V. Eshtukov, V. E. Terekhov, A. F. Asachenko and M. S. Nechaev, An unprecedentedly simple method of synthesis of aryl azides and 3-hydroxytriazenes, Green Chem., 2016, 18(22), 5984–5988 RSC.
- A. A. Siddiki, B. S. Takale and V. N. Telvekar, One pot synthesis of aromatic azide using sodium nitrite and hydrazine hydrate, Tetrahedron Lett., 2013, 54(10), 1294–1297 CrossRef CAS.
- W. D. Castro-Godoy, A. A. Heredia, L. C. Schmidt and J. E. Argüello, A straightforward and sustainable synthesis of 1, 4-disubstituted 1, 2, 3-triazoles via visible-light-promoted copper-catalyzed azide–alkyne cycloaddition (CuAAC), RSC Adv., 2017, 7(54), 33967–33973 RSC.
- C. Menendez, S. Gau, C. Lherbet, F. Rodriguez, C. Inard, M. R. Pasca and M. Baltas, Synthesis and biological activities of triazole derivatives as inhibitors of InhA and antituberculosis agents, Eur. J. Med. Chem., 2011, 46(11), 5524–5531 CrossRef CAS PubMed.
- T. B. Mhamane, S. Sambyal, S. Vemireddy, I. A. Khan and S. Shafi, Novel 1, 2, 3-triazole-tethered Pam3CAG conjugates as potential TLR-2 agonistic vaccine adjuvants, Bioorg. Chem., 2021, 111, 104838 CrossRef CAS PubMed.
- L. Ganapathi, S. Van Haren, D. J. Dowling, I. Bergelson, N. M. Shukla, S. S. Malladi, R. Balakrishna, H. Tanji, U. Ohto and T. Shimizu, The imidazoquinoline toll-like receptor-7/8 agonist hybrid-2 potently induces cytokine production by human newborn and adult leukocytes, PLoS One, 2015, 10(8), e0134640 CrossRef PubMed.
- S. Vemireddy and P. Pallavi, Chitosan stabilized nasal emulsion delivery system for effective humoral and cellular response against recombinant tetravalent dengue antigen, Carbohydr. Polym., 2018, 190, 129–138 CrossRef CAS PubMed.
- K. B. Gorden, K. S. Gorski, S. J. Gibson, R. M. Kedl, W. C. Kieper, X. Qiu, M. A. Tomai, S. S. Alkan and J. P. Vasilakos, Synthetic TLR agonists reveal functional differences between human TLR7 and TLR8, J. Immunol., 2005, 174(3), 1259–1268 CrossRef CAS PubMed.
- P. J. Mcenaney and D. A. Spiegel, Synthetic Immunology, Biotherapeutics: Recent Developments Using Chemical and Molecular Biology, 2013, p. 1 Search PubMed.
- C. E. Schiaffo, C. Shi, Z. Xiong, M. Olin, J. R. Ohlfest, C. C. Aldrich and D. M. Ferguson, Structure–activity relationship analysis of imidazoquinolines with Toll-like receptors 7 and 8 selectivity and enhanced cytokine induction, J. Med. Chem., 2014, 57(2), 339–347 CrossRef CAS PubMed.
- A. Talukdar, D. Ganguly, S. Roy, N. Das and D. Sarkar, Structural evolution and translational potential for agonists and antagonists of endosomal toll-like receptors, J. Med. Chem., 2021, 64(12), 8010–8041 CrossRef CAS PubMed.
- N. S. Vatmurge, B. G. Hazra, V. S. Pore, F. Shirazi, P. S. Chavan and M. V. Deshpande, Synthesis and antimicrobial activity of β-lactam–bile acid conjugates linked via triazole, Bioorg. Med. Chem. Lett., 2008, 18(6), 2043–2047 CrossRef CAS PubMed.
|
This journal is © The Royal Society of Chemistry 2023 |
Click here to see how this site uses Cookies. View our privacy policy here.