DOI:
10.1039/D2RA06273A
(Paper)
RSC Adv., 2023,
13, 1659-1671
Seasonal metabolic profiling of Valencia orange leaf essential oil using GC coupled with chemometrics, nano-formulation, and insecticidal evaluation: in vivo and in silico
Received
6th October 2022
, Accepted 23rd December 2022
First published on 9th January 2023
Abstract
Mosquitoes and mosquito-borne infectious diseases are a global challenge, especially with increased resistance to synthetic insecticides. The foregoing study aimed to utilize the essential oil of leaves of Citrus sinensis var. Valencia as a cheap, safe, eco-friendly (green), and effective alternative to chemical insecticides. Essential oil samples were collected from fresh and dried leaves across different seasons. They are subjected to hydrodistillation and then GC analysis to be compared. Seventy-seven compounds were detected in all samples where monoterpene hydrocarbons represented the most abundant class of hydrocarbons in fresh leaves (52.6–74.4%) and dried leaves (58.6–66.9%). Sabinene (8.26–29.2%), delta-3-carene (8.23–16.4%), D-limonene (2.50–11.2%), and β-myrcene (2.40–4.93%) were the major monoterpene hydrocarbons in all seasons. Oxygenated monoterpenes comprising β-linalool, citronellal, terpinen-4-ol, β-citral, and α-citral exhibited also appreciable percentages in fresh (21.2–43.4%) and dried leaves (23.4–33.0%). Hierarchical cluster analysis (HCA) and principal component analysis (PCA) effectively segregated all samples into three discriminate clusters where, β-linalool, terpinen-4-ol, β-elemene enantiomer, sabinene, and β-phellandrene constitute the main discriminatory biomarkers. Essential oil of fresh spring leaves (FS) was chosen for nano-formulation adopting the hot emulsification method. Both FS sample and the prepared nano-hexosomal formula were screened against the 3rd instar larvae Culex pipiens L. (common house mosquito). LC50 and LC95 values of FS and oil loaded nano-formula were (48 and 30
552 mg L−1) and (30 and 1830 mg L−1) respectively. α-Citral followed by citronellal showed the best fitting within the binding sites of acetylcholine esterase enzyme utilizing molecular docking. Thus, it can be concluded that Valencia orange leaf as a nano-formulation could serve as an effective and sustainable insecticidal agent.
1 Introduction
Mosquitoes constitute one of the most dangerous vectors that transmit many infectious diseases. The common house mosquito (Culex pipiens L., family Culicidae) transmits serious diseases, such as avian malaria, Japanese encephalitis, West Nile virus infection, and filariasis.1 However, potential side effects were anticipated with the usage of synthetic insecticides, including carcinogenicity and irritation of the eyes and skin.2 Effective and safe pesticides are a matter of interest, especially after insect resistance to many insecticides and thus searching for naturally occurring pesticides with fewer adverse effects compared to synthetic ones is mandatory worldwide.
Essential oils (EO) are eco-friendly alternatives to synthetic insecticides.3 Citrus is an important genus of the Rutaceae family, comprising many odoriferous species, e.g., oranges (C. sinensis), limes (C. aurantifolia), and tangerine (C. reticulate).4,5 Citrus sinensis is commonly known as sweet orange; it possess many bioactive secondary metabolites such as flavonoids, steroids, EOs, and coumarins.6 Many Citrus species are used in folk medicine as insecticides,7 whereas orange peels are used traditionally as insecticidal agents via burning of peel to control mosquitoes and houseflies in Ghana.8 The essential oil of Citrus sinensis peel revealed insecticidal activity against mosquitoes, cockroaches, and houseflies.7 Furthermore, the peel and leaves EO showed potential effects on Cx. pipiens mosquitos.6,9 Various techniques are utilized for the extraction of essential oil from the plant such as solvent extraction, steam-hydrodistillation, steam distillation, and hydrodistillation.10,11 Hydrodistillation is the most common technique for essential oil extraction on small or industrial scale.12 No need for consumption of organic solvent compared with the traditional methods such as solvent extraction which has many drawbacks.13 The Clevenger hydrodistillation apparatus is widely available in low cost and easily to be operated without need of expensive equipment compared with the advanced techniques such as Supercritical Fluid Extraction (SFE).
Nevertheless, the volatile nature, possible environmental decomposition, and poor water solubility could negatively affect the biological activity despite being potent, natural, and safe insecticides.14 Therefore, nano-encapsulation of EOs was sought to minimize these problems along with enhanced efficacy by the induction of systemic activity due to the small size of such particles, which facilitate the penetration inside the insect.15
Species variation, seasonal variation, organ type, and extraction methods led to quantitative variation in the profile of constituents of Citrus EOs.16,17 For instance, drying processes affected some EOs yield and pattern of constituents.18 Besides, the changes in the chemical composition of EOs as a result of seasonal variations could strongly influence the pharmacological properties.
One of the useful approaches to create a green insecticide is to use inexpensive, easily biodegradable plant components. The current study aimed to use Citrus sinensis var. Valencia leaves' essential oil (EO) as a less expensive, environmentally friendly, and efficient substitute for chemical pesticides. However, to the best of our knowledge, a relatively limited data could be found in the literature exploring both the effects of the seasonal variation and drying process on yield and composition of the EOs obtained from the leaves of Citrus sinensis (L.) Osbeck var. Valencia. Moreover, the lack of published information concerning the effect of EOs of Valencia orange leaf as a nano-hexosomal formula to fight house mosquito and postulating the mechanism of pesticidal activity through molecular docking warranted the execution of the present study. As a consequence, essential oil samples from fresh and dried Valencia orange leaves collected in different seasons were compared using GC-mass analysis coupled with chemometric study. The season with the greatest oil yield was chosen for subsequent insecticidal study against the third instar larvae Cx. pipiens in comparison with the oil loaded nano-formula to reach maximum insecticidal activity with minimum side effects. Additionally, major identified volatile components were screened in silico against acetylcholine esterase.
2 Experimental
2.1. Plant material
Valencia orange leaves (500 g) were collected from two Citrus trees have leaf flushes at 20–30 days age at the middle of each season starting from October 2019 till July 2020 from El Safwa Farm, kilo 127, El Alamein Road https://goo.gl/maps/4V73u9ogLat8zdkx5. Collected leaves samples were divided into two halves by weighing each of them separately; the first half was the fresh part which was subjected directly to the hydro-distillation, while the second half was allowed to dry for 3 days by spreading leaves in open air and away from sunlight. Plant material was kindly authenticated by Dr Latif Fahmy Gunidy, Emeritus Chief Researcher, Citrus Research Department, Horticultural Research Institute, Agricultural Research Center. A voucher specimen (No. 29.09.2021) is kept at the herbarium of the Department of Pharmacognosy, Faculty of Pharmacy, Cairo University https://goo.gl/maps/v6PsvJp6KJW52PkH8.
2.2. Extraction of the essential oil
Valencia orange EO were extracted from both fresh and air-dried leaves. Each sample (250 g) was cut off and, separately subjected to hydro-distillation for 3 h using Clevenger-type apparatus.19 Each leaves sample was done in triplicate. Then the collected oil was subjected to drying over anhydrous sodium sulfate. The percentage yield of each sample was calculated and kept at 4 °C in completely sealed vials for further analysis. The percentage yield was calculated following this equation:
% Yield = [Oil volume (mL)/Plant material (g)] × 100 |
In each sample, the refractive index was measured using an Abbe Hand refractometer (ATAGO, Tokyo, Japan), while optical rotation was estimated using a polarimeter (PerkinElmer 241, Waltham, Massachusetts, USA) and specific gravity was detected through an ignition tube.20
2.3. Gas chromatography coupled with mass spectrometry analysis of the essential oil
The composition of the Valencia orange leaves essential oils was determined by chemical analysis utilizing gas chromatography coupled to mass spectrometry (GC-MS).21 It was carried out at the Faculty of Pharmacy, Ain Shams University (Cairo, Egypt) on Shimadzu GC system (QP2010, Koyoto, Japan) equipped with Rtx-5MS fused bonded column (Restek, USA) (30 m × 0.25 mm i.d. × 0.25 μm film thickness) and coupled to a quadruple mass spectrometer (SSQ 7000; Thermo-Finnigan, Bremen, Germany). The initial column temperature was isothermal (45 °C for 2 min), programmed (300 °C at 5 °C min−1) then kept constant at 300 °C for 5 min. The injector temperature was 250 °C and the flow rate of helium carrier gas was 1.41 mL min−1. All the mass spectra were recorded applying the following conditions; filament emission current, 60 mA; electron energy, 70 eV; ion source, 200 °C. Diluted samples (1% v/v) were injected with split mode (split ratio 1
:
15). The constituents were de-convoluted using AMDIS software (http://www.amdis.net/). The constituents' identification was performed by comparing their retention indices (RI) and mass spectra with those deposited in NIST mass spectral library and The Pherobase database (https://pherolist.org/) and confirmed by the reported literature.22 Reagents and controls used for GC analysis were purchased from Sigma (Sigma Aldrich, GmbH, Sternheim, Germany).
2.4. Seasonal metabolic discrimination of Valencia orange leaves using multivariate data analysis
Chemometric analysis was performed using unsupervised pattern recognition techniques including Hierarchical cluster analysis (HCA) and Principle component analysis (PCA). This was carried out based upon data obtained from GC analyses of essential oils obtained from fresh and dried leaves of Valencia orange collected in different seasons. HCA was done using the entire linkage manner adopted for group classification whereas both PCA and HCA were done utilizing CAMO's Unscrambler® X 10.4 software (Computer-Aided Modeling, As, Norway) as previously reported.23
2.5. Preparation and characterization of hexosomal nanodispersion
2.5.1. Chemicals. Oleic acid (OA) (purity 99%), Pluronic F127 (purity 99), and Glyceryl monooleate (GMO) (purity 99%), of analytical grade were purchased from Sigma-Aldrich Co. (St. Louis, MO, USA).
2.5.2. Experimental procedures. Hexosomal nano-particles possess unique properties to enclose hydrophilic and hydrophobic small drug molecules.24 Nano-hexosomal formula was efficiently prepared by the hot emulsification method25 with minor adjustments. Briefly, glyceryl monooleate (GMO) and oleic acid (OA) (0.30 and 0.15 g, respectively) were weighed accurately and then transferred to glass vials after melting at 70 °C to obtain a homogenous mixture to which the EO of leaves (0.10 g) were added to obtain the lipid layer. The lipoidal phase is emulsified into an aqueous phase containing Pluronic F127 (0.05 g), which acts as a stabilizer. The obtained milky dispersions were allowed to cool to room temperature and then centrifuged at 1000 rpm for 15 min at 4 °C. The formed nano-particles were re-dispersed in deionized water and kept in glass vials for future studies. Final concentrations of OA and GMO were 1.5 and 3% w/w, respectively, F127 (0.5% w/w), water (94% w/w), and essential oil concentration (1% w/w).
2.5.3. Particle size and zeta potential analysis. The particle size (PS) and polydispersity index (PDI) were investigated using Zetasizer Nano ZS (Ver.6.12, Malvern Instruments Ltd, Worcestershire, England) using the dynamic light scattering technique at room temperature.26 Hexosomal-nanoparticles were previously diluted with distilled water (100-fold) before any measurements. The zeta potential (ZP) of hexosomal-nanodispersions was determined using the same equipment at room temperature and measurements of both parameters were run in duplicate.27
2.5.4. Transmission electron microscope (TEM). The structure of the EO-loaded hexosomal nano-dispersions was examined by transmission electron microscope, (H-7650, Hitachi, Japan); the prepared formulation was already diluted with distilled water before the examination. One drop of the EO-loaded formulation was put on a film-coated copper grid before staining with a (2% w/v) phosphotungstic acid solution. The grid was allowed to dry for 10 min at ambient temperature before visualization under a transmission electron microscope.28
2.6. Insecticidal activity
2.6.1. Mosquitoes rearing. Cx. pipiens egg rafts were found in an insectary at Entomology Department, Faculty of Science, Ain Shams University. They were reared under certain growth conditions of temperature (25 ± 2 °C) and humidity (70 ± 10%) with a photoperiod of 14
:
10 light
:
dark h (Shah et al., 2016). Hatched larvae were fed fish food (Tetra-min) two times per day (Gerberg, 1970; Kasap and Demirhan, 1992).29,30
2.6.2. Experimental. The larvicidal activity of EO sample and its oil-loaded nano-hexosomal formula were assessed against the 3rd instar larvae of Cx. pipiens following (WHO, 2005),31 where groups of twenty-five early third instar larvae of Cx. pipiens were transferred using a plastic dropper into small test cups. Each cup held 100 mL of water under specific laboratory temperature and humidity conditions (25 ± 2 °C, RH 70 ± 10%, and 14–10 light–dark regime). The EO was dissolved in absolute ethanol of HPLC grade to prepare many concentrations where three different concentrations of each EO samples and oil loaded nano-hexosomal formulation were conducted in the experiment namely 13, 21, and 25 mg L−1. Blank formula composed of nano-hexosomal formula without volatile oil was prepared at the same concentration in order to eliminate any interference. For each treatment, three replicates of twenty-five larvae were used, and three replicates of twenty-five larvae were maintained in distilled water served as untreated (negative control). For positive control, absolute ethanol only was used at the same concentrations as the tested samples. Assessment of larval mortality was recorded after 24 h post-treatment.
2.6.3. Statistical analysis. Lethal concentrations were determined at the 95% confidence level using a probit regression line. LC50 and LC95 were calculated.32 The percentages of larval mortality were calculated for each concentration of the tested samples. Correction for control mortality was conducted using Abbott's formula. One-way ANOVA followed by Tukey's multiple comparison tests (P < 0.05) were carried out to illustrate the significance of the tested samples from the control groups. All statistical analysis was conducted using the statistical Package for Social Science (SPSS) software version 11.5.
2.7. Molecular docking
Molecular docking was done for the main volatile constituents identified in the essential oils of Valencia orange leaves within the binding sites of acetylcholine esterase (PDB ID: 5X61; 3.40 Å) downloaded from the protein data bank (PDB). This was carried out utilizing Discovery Studio 4.5 (Accelrys Inc., San Diego, CA, USA) with C-Docker protocol as previously reported.33,34 Meanwhile, the binding energies (ΔG) were calculated in accordance with the following equation.35
ΔGbinding = Ecomplex − (Eprotein + Eligand) |
where ΔGbinding is the ligand–protein interaction binding energy, Ecomplex is the potential energy for the complex of protein bound with the ligand, Eprotein is the potential energy of protein alone and Eligand is the potential energy for the ligand alone.
3 Results and discussion
3.1. Yield and physical characters of Valencia orange leaves essential oils
The yield percentages of EO extracted from fresh and dried leaves of Valencia orange were calculated in triplicate for each season and expressed as an average value in Table 1 and Fig. 1. It ranged from 0.160 ± 0.15–0.44 ± 0.10% for fresh leaf samples. The highest percentage was obtained from fresh spring oil (0.44 ± 0.10%) meanwhile the lowest percentage was observed in fresh summer (0.160 ± 0.15%). Dried leaves gave a higher yield than fresh ones (0.240 ± 0.07–0.480 ± 0.10%) where the highest oil percentage was observed with dried spring oil (0.480 ± 0.10%) and the lowest percentage was that in the dried summer sample (0.240 ± 0.07%) similarly like fresh leaves. Dried samples generally give higher oil yields than fresh samples, which may be due to the high moisture content.36 Besides, spring is the best harvest season for the leaves as the highest essential oil yield could be obtained. The distilled oil samples were obtained as yellow oily liquids with an aromatic odor from fresh leaves, while darker yellow and more fragrant oils were obtained from dried leaves. Specific gravity, refractive index, and optical rotation were measured and recorded in (Table 1) with closely similar results.
Table 1 Percentage yield and physical characters of the Essential oils of Valencia orange leaves
Tested sample |
% Yield (v/w) |
Optical rotation |
Refractive index |
Specific gravity |
Fresh autumn |
0.24 ± 0.14 |
+92.8° |
1.39 |
0.841 |
Dried autumn |
0.32 ± 0.2 |
+92.8° |
1.38 |
0.825 |
Fresh winter |
0.32 ± 0.14 |
+92.8° |
1.38 |
0.837 |
Dried winter |
0.36 ± 0.07 |
+92.8° |
1.39 |
0.837 |
Fresh spring |
0.44 ± 0.1 |
+91.4° |
1.40 |
0.804 |
Dried spring |
0.48 ± 0.1 |
+91.3° |
1.40 |
0.839 |
Fresh summer |
0.16 ± 0.15 |
+91.3° |
1.40 |
0.839 |
Dried summer |
0.24 ± 0.07 |
+90.5° |
1.40 |
0.842 |
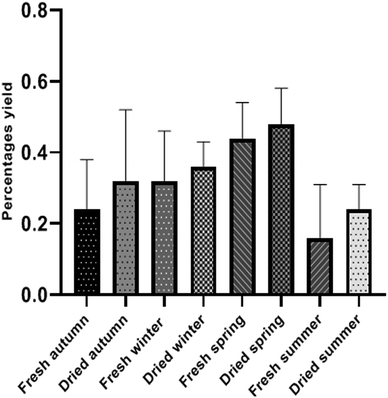 |
| Fig. 1 Histograms showing the percentage yield of oil samples collected from fresh and dried leaves of Valencia orange during the four seasons. | |
3.2. Metabolic profiling of Valencia orange leaves essential oils using gas chromatography analysis
Different oil components were displayed based upon their elution order from the Rtx-5MS capillary column. Gas chromatographic analysis of the tested oil samples was done in triplicate for each sample. It revealed the presence of 77 compounds in all samples (Table 2). It is observed that 46, 41, 38, and 42 constituents were detected in autumn, winter, spring, and summer fresh samples constituting relative area percentages of 99.6, 98.8, 99.3, and 99.6 of the oil composition, respectively. Dried samples displayed a higher number of components where 44, 42, 40, and 47 were identified in autumn, winter, spring, and summer respectively with the corresponding percentages of 98.9, 97.8, 99.4, and 98.2, respectively. Qualitative variations as a result of seasonal variation and the drying process affected the yield and composition of oil samples. By comparing peak area % of the identified components, monoterpene hydrocarbons showed significant amounts in all EO samples which are in accordance with previously published data.18 They constituted a range of 52.6–74.4% of fresh leaves collected at different seasons. Relatively, spring oil was the richest one monoterpenes accounting for 74.4% meanwhile autumn oil showed the lowest percentage with 52.6%. Samples obtained from the dried leaves displayed a lower percentage of monoterpenes ranging between 58.6–66.9%. The greatest percentage was recorded in winter (66.9%) whereas the lowest was observed in autumn (58.6%). Monoterpene hydrocarbons may strongly affect the biological action of EOs.37 Sabinene was detected in a considerable amount in all analyzed samples where in fresh spring leaves, sabinene represented the greatest component (27.4%) while the lowest abundance was observed in fresh autumn sample (8.26%) which was in agreement with the previously reported studies of sweet orange.17 Meanwhile, delta 3-carene accounted for 16.4% in dried leaves of winter as the second major monoterpene and a minimum percentage was detected in fresh leaves of summer (8.23%). Limonene hydrocarbon displayed the highest amount in fresh autumn (11.2%) meanwhile the least amount was observed in the EO of dried spring leaves (2.50%). In addition, β-myrcene hydrocarbon was present in an appreciable amount in all tested samples ranging between 2.40%–4.93% for both fresh and dried samples. Oxygenated monoterpenes were the second predominant class of constituents. A significant percentage was detected in oil samples obtained from fresh autumn and fresh winter showing 43.4% and 33.4% respectively. On the other side, dried autumn and dried winter afforded a lower number of oxygenated monoterpenes accounting for 33.0 and 28.8% respectively. Percentages of oxygenated monoterpenes in fresh spring and fresh summer were 21.2% and 24.8% respectively and slightly increased after drying to 23.8% and 30.5%, respectively. Beta linalool is the predominant alcohol in all leaves samples and showed a higher amount in dried leaves than fresh ones except for fresh autumn oil which accounted for 16.3% of β-linalool. These findings were compatible with the previously reported data about linalool.17 Citronellal, terpinen-4-ol, β-citral, and α-citral were also considered the major oxygenated monoterpenes however sesquiterpenes were detected in smaller amounts in comparison to monoterpenes. Dried spring oil showed the highest percentage of sesquiterpenes (9.45%) with β-elemene enantiomer as a major sesquiterpene and represented 5.70%. Generally, sesquiterpenes showed a lower abundance in all oil samples than monoterpenes whereas a higher sesquiterpenes percentage was recorded in the dried leaves compared to the fresh ones meanwhile the minimum amount of sesquiterpenes was found in leaves of fresh summer (1.31%). The oxygenated sesquiterpenes percentages were relatively low compared to other constituents (0.040–0.959%). β-Sinensal was the main oxygenated sesquiterpene (0.040–0.640%) additionally α-cubebene (sesquiterpene) and caryophyllene oxide (oxygenated sesquiterpene) were absent in most fresh samples but present in dried leaf oil samples. Such findings may be due to the artifact formation via esterification, oxidation, glycoside hydrolysis, and other processes during drying.38 Concerning the Egyptian Valencia orange cultivar, results were following formerly published data with some variations in composition, which could be attributed to seasonal variation together with the influence of drying. Identified oil components of various classes are summarized in (Table 2 and Fig. 2). The major constituents prevailing in analyzed oil samples of Valencia orange leaves are displayed in (Fig. 3). It is possible to predict that oil derived from fresh spring leaves is the best one to be conducted for the insecticidal activity against Cx. pipiens. Spring leaves had the highest oil yield, ranging from 0.400–0.480 percent for fresh and dried leaves, respectively. Although the oil yield from dried leaves of spring is higher than fresh ones, oil obtained from fresh leaves was chosen for the insecticidal activity to avoid any changes in the chemical profile of essential oil during the drying process to evaluate the potential of fresh leaves implementation in traditional practices. Drying factors such as temperature affect the biological activity of oil. The insecticidal activity of the EO obtained from leaves of sweet orange was greatly varied according to the duration of the drying process.18 Additionally, GC-mass analysis displayed that the oil of fresh spring leaves has the greatest relative percentages of monoterpenes and sesquiterpene (77.4%), which may be related to the essential oil's insecticidal effects. Fresh spring leaves are a rich source of essential oils, which could be used to generate acceptable nano-formulations with potent insecticidal action. In a subsequent step of this study, we will utilize the essential oil obtained from fresh leaves of spring to optimize the hydrodistillation method using surface response method in order to reach the optimum yield.39
Table 2 Identified constituents in the analyzed oil samples of leaves of Valencia orange collected in different seasons
Peak no.a |
Rt.b |
R.I. Calc.c |
R.I. Rep.d |
Identified constituent |
Relative percentages of oil components |
Autumn |
Winter |
Spring |
Summer |
Fresh |
Dried |
Fresh |
Dried |
Fresh |
Dried |
Fresh |
Dried |
Peak number. Retention time. Retention index (calculated). Retention index (reported). |
1 |
7.12 |
909 |
924 |
α-Thujene |
0.280 |
0.290 |
0.340 |
0.320 |
0.440 |
0.430 |
0.350 |
0.510 |
2 |
7.31 |
915 |
932 |
α-Pinene |
1.22 |
1.21 |
1.30 |
1.40 |
2.10 |
2.60 |
1.53 |
2.00 |
3 |
7.60 |
940 |
945 |
α-Fenchene |
— |
0.010 |
— |
— |
— |
0.010 |
— |
0.023 |
4 |
7.70 |
948 |
946 |
Camphene |
— |
0.020 |
— |
0.010 |
0.040 |
0.050 |
0.030 |
0.040 |
5 |
8.58 |
960 |
969 |
Sabinene |
8.26 |
15.5 |
23.8 |
29.2 |
27.4 |
17.4 |
23.6 |
18.8 |
6 |
8.62 |
962 |
974 |
β-Pinene |
0.650 |
0.960 |
1.32 |
1.89 |
2.00 |
2.91 |
1.80 |
2.28 |
7 |
9.06 |
979 |
988 |
β-Myrcene |
3.49 |
2.40 |
4.15 |
4.93 |
4.62 |
4.6 |
4.11 |
3.76 |
8 |
9.44 |
993 |
1001 |
(+)-4-Carene |
— |
— |
— |
— |
— |
— |
— |
5.50 |
9 |
9.64 |
998 |
1002 |
α-Phellandrene |
0.230 |
0.350 |
0.500 |
— |
0.680 |
0.590 |
0.060 |
0.580 |
10 |
9.65 |
1004 |
1008 |
Delta-3-carene |
13.5 |
12.7 |
8.50 |
16.4 |
10.3 |
12.1 |
8.23 |
9.99 |
11 |
9.82 |
1006 |
1010 |
(+/−)-2-Carene |
— |
— |
— |
— |
0.850 |
0.760 |
0.620 |
1.43 |
12 |
9.83 |
1011 |
1014 |
α-Terpinene |
0.760 |
0.510 |
0.630 |
— |
— |
— |
— |
— |
13 |
10.0 |
1013 |
1022 |
o-Cymene |
0.190 |
0.020 |
0.030 |
2.70 |
— |
0.130 |
0.020 |
0.046 |
2.00 |
14 |
10.0 |
1014 |
1023 |
m-Cymene |
— |
— |
0.130 |
— |
0.070 |
— |
0.200 |
0.150 |
15 |
10.1 |
1015 |
1025 |
Sylvestrene |
0.150 |
0.140 |
0.200 |
— |
0.180 |
0.210 |
0.170 |
— |
16 |
10.2 |
1017 |
1025 |
β-Phellandrene |
1.45 |
9.39 |
— |
— |
9.70 |
10.6 |
11.7 |
0.630 |
17 |
10.2 |
1018 |
1029 |
D-Limonene |
11.2 |
4.96 |
8.18 |
6.60 |
2.75 |
2.50 |
10.0 |
4.43 |
18 |
10.3 |
1026 |
1031 |
Eucalyptol |
0.290 |
— |
0.180 |
— |
— |
— |
— |
— |
19 |
10.5 |
1028 |
1032 |
cis-β-Ocimene |
0.120 |
0.120 |
0.230 |
— |
0.260 |
0.270 |
0.260 |
0.180 |
20 |
10.8 |
1039 |
1044 |
trans-β-Ocimene |
6.85 |
7.43 |
12.0 |
2.98 |
11.0 |
8.61 |
9.13 |
6.10 |
21 |
11.1 |
1050 |
1054 |
γ-Terpinene |
2.00 |
0.910 |
0.940 |
— |
1.33 |
1.18 |
0.880 |
2.24 |
22 |
11.4 |
1056 |
1065 |
cis-Sabinene hydrate |
0.140 |
0.280 |
0.460 |
0.390 |
0.350 |
0.920 |
0.420 |
0.160 |
23 |
12.0 |
1078 |
1086 |
Terpinolene |
1.65 |
1.40 |
0.280 |
0.080 |
0.270 |
0.280 |
0.240 |
1.60 |
24 |
12.4 |
1091 |
1095 |
β-Linalool |
16.3 |
14.8 |
13.6 |
14.6 |
10.2 |
11.8 |
8.43 |
12.9 |
25 |
13.1 |
1111 |
1098 |
trans-Sabinene hydrate |
0.170 |
— |
0.070 |
— |
0.060 |
0.150 |
0.040 |
0.130 |
26 |
13.6 |
1130 |
1136 |
trans-ρ-Menth-2-en-1-ol |
0.080 |
— |
— |
— |
0.030 |
— |
0.050 |
— |
27 |
14.0 |
1142 |
1148 |
(3R)-(+)-Citronellal |
2.62 |
3.20 |
5.05 |
3.63 |
1.43 |
2.08 |
2.86 |
1.60 |
28 |
14.4 |
1154 |
1158 |
Isomenthone |
0.140 |
— |
— |
— |
— |
— |
2.30 |
— |
29 |
14.5 |
1155 |
1160 |
cis-Isoneral |
— |
— |
— |
— |
— |
— |
0.006 |
— |
30 |
14.6 |
1158 |
1165 |
p-Mentha-1,5-dien-8-ol |
0.030 |
— |
— |
— |
— |
— |
— |
0.007 |
31 |
14.7 |
1163 |
1167 |
DL-Menthol |
0.870 |
— |
— |
— |
— |
— |
— |
0.080 |
32 |
14.8 |
1167 |
1174 |
(−)-Terpinen-4-ol |
8.88 |
3.20 |
3.71 |
3.00 |
3.42 |
3.47 |
2.30 |
4.82 |
33 |
15.0 |
1174 |
1177 |
trans-Isoneral |
— |
— |
— |
— |
— |
— |
0.017 |
— |
34 |
15.1 |
1178 |
1179 |
p-Cymen-8-ol |
— |
— |
— |
0.280 |
— |
— |
— |
— |
35 |
15.2 |
1180 |
1186 |
α-Terpineol |
— |
0.420 |
0.340 |
0.360 |
0.640 |
0.740 |
0.420 |
0.950 |
36 |
15.3 |
1182 |
1192 |
Dihydrocarveol |
— |
0.930 |
0.200 |
— |
— |
— |
— |
— |
37 |
15.4 |
1185 |
1195 |
cis-Piperitol |
0.080 |
— |
— |
— |
— |
— |
— |
— |
38 |
15.4 |
1187 |
1200 |
trans-Dihydrocarvone |
0.210 |
— |
— |
— |
— |
— |
— |
— |
39 |
16.1 |
1210 |
1215 |
trans-Carveol |
— |
0.280 |
0.073 |
— |
— |
— |
— |
— |
40 |
16.3 |
1218 |
1226 |
Nerol |
— |
— |
— |
0.350 |
1.38 |
1.38 |
— |
— |
41 |
16.4 |
1220 |
1228 |
Citronellol |
4.41 |
0.230 |
0.340 |
0.110 |
— |
1.45 |
0.250 |
0.490 |
42 |
16.5 |
1222 |
1232 |
cis-Carveol |
— |
0.380 |
0.110 |
— |
— |
— |
— |
— |
43 |
16.7 |
1230 |
1233 |
Pulegone |
0.041 |
— |
— |
— |
0.180 |
— |
— |
— |
44 |
16.8 |
1231 |
1235 |
β-Citral |
0.440 |
1.85 |
3.22 |
1.81 |
1.92 |
1.17 |
3.58 |
3.56 |
45 |
16.9 |
1235 |
1239 |
Carvone |
7.80 |
5.03 |
2.25 |
— |
— |
— |
— |
0.032 |
46 |
17.1 |
1248 |
1257 |
Geraniol |
0.730 |
— |
— |
— |
0.34 |
0.04 |
0.03 |
0.69 |
47 |
17.6 |
1262 |
1264 |
α-Citral |
0.410 |
2.06 |
3.78 |
2.30 |
1.70 |
1.14 |
3.20 |
3.90 |
48 |
18.2 |
1284 |
1273 |
Menthol, 1′-(butyn-3-one-1-yl)-, (1S,2S,5R)- |
0.130 |
— |
— |
— |
— |
— |
— |
— |
49 |
18.3 |
1285 |
1284 |
Dihydroedulan II |
0.050 |
0.040 |
— |
— |
— |
— |
— |
— |
50 |
18.4 |
1287 |
1289 |
Thymol |
— |
— |
— |
— |
— |
— |
0.720 |
— |
51 |
19.2 |
1315 |
1322 |
trans-Geranic acid methyl ester |
— |
0.030 |
0.020 |
0.230 |
— |
— |
0.050 |
0.060 |
52 |
19.3 |
1318 |
1305 |
Dihydrocarvyl acetate |
0.100 |
0.260 |
— |
— |
— |
— |
— |
— |
53 |
19.5 |
1326 |
1339 |
(−)-trans-Carvyl acetate |
0.080 |
— |
— |
0.010 |
— |
— |
— |
— |
54 |
19.8 |
1338 |
1380 |
(+)-3-Carene, 2-(acetylmethyl)- |
— |
— |
— |
0.020 |
— |
— |
— |
— |
55 |
19.9 |
1342 |
1354 |
Citronellol acetate |
— |
0.310 |
0.270 |
0.780 |
— |
0.130 |
0.270 |
1.20 |
56 |
20.3 |
1354 |
1365 |
Neryl acetate |
— |
— |
0.500 |
1.00 |
— |
— |
0.380 |
0.260 |
57 |
20.6 |
1365 |
1345 |
(−)-α-Cubebene |
— |
— |
— |
— |
— |
0.020 |
— |
0.040 |
58 |
20.7 |
1374 |
1387 |
(−)-β-Bourbonene |
0.120 |
— |
0.040 |
— |
0.080 |
— |
— |
— |
59 |
20.8 |
1374 |
1389 |
(−)-β-Elemene |
— |
0.070 |
— |
— |
0.130 |
0.360 |
0.110 |
0.320 |
60 |
21.0 |
1381 |
1389 |
β-Elemene enantiomer |
1.60 |
4.20 |
0.910 |
1.40 |
2.00 |
5.70 |
0.790 |
3.60 |
61 |
21.8 |
1409 |
1417 |
Caryophyllene |
0.760 |
1.60 |
0.270 |
0.300 |
0.50 |
1.80 |
0.280 |
1.80 |
62 |
21.8 |
1423 |
1411 |
Isocaryophyllene |
— |
— |
0.260 |
— |
— |
— |
— |
— |
63 |
22.1 |
1424 |
1432 |
trans-α-Bergamotene |
— |
— |
— |
— |
— |
— |
— |
0.039 |
64 |
22.7 |
1444 |
1452 |
Humulene |
0.200 |
0.600 |
0.400 |
0.200 |
0.230 |
0.980 |
0.110 |
0.670 |
65 |
23.4 |
1465 |
1463 |
cis-Muurola-4(14),5-diene |
0.040 |
— |
0.020 |
— |
— |
— |
— |
— |
66 |
23.4 |
1472 |
1484 |
Germacrene D |
— |
0.180 |
— |
— |
— |
— |
— |
— |
67 |
23.8 |
1488 |
1476 |
β-Chamigrene |
— |
— |
— |
— |
— |
— |
— |
0.150 |
68 |
23.9 |
1489 |
1492 |
Elixene |
— |
0.090 |
— |
— |
— |
0.140 |
— |
— |
69 |
24.0 |
1497 |
1509 |
α-Bulnesene |
— |
0.100 |
0.020 |
0.030 |
0.130 |
0.450 |
0.020 |
0.230 |
70 |
24.5 |
1522 |
1513 |
Cadina-1(10),4-diene |
— |
0.060 |
— |
— |
— |
— |
— |
0.010 |
71 |
25.1 |
1530 |
1546 |
Hedycaryol |
— |
— |
— |
— |
— |
0.060 |
— |
— |
72 |
25.9 |
1575 |
1577 |
Spathulenol |
0.069 |
— |
— |
— |
— |
— |
— |
— |
73 |
26.0 |
1580 |
1582 |
Caryophyllene oxide |
0.250 |
0.010 |
— |
0.200 |
— |
— |
— |
0.050 |
74 |
26.3 |
1612 |
1622 |
10-Epi-γ-eudesmol |
— |
— |
— |
— |
0.020 |
0.010 |
— |
— |
75 |
27.8 |
1688 |
1658 |
Neointermedeol |
— |
— |
— |
— |
— |
— |
— |
0.010 |
76 |
28.7 |
1690 |
1686 |
β-Sinensal |
0.640 |
0.400 |
0.210 |
0.290 |
0.600 |
0.250 |
0.040 |
0.200 |
77 |
29.0 |
1710 |
1714 |
6-Isopropenyl-4,8a-dimethyl 1,2,3,5,6,7,8,8a-octahydro-naphthalen-2-ol |
— |
— |
— |
0.020 |
— |
— |
— |
— |
|
|
|
|
Total |
99.6 |
98.9 |
98.8 |
97.8 |
99.3 |
99.4 |
99.6 |
98.2 |
|
|
|
|
Monoterpene hydrocarbons |
52.6 |
58.6 |
63.2 |
66.9 |
74.4 |
66.3 |
73.4 |
60.5 |
|
|
|
|
Sesquiterpene hydrocarbons |
2.72 |
6.90 |
1.92 |
1.93 |
3.07 |
9.45 |
1.31 |
6.85 |
|
|
|
|
Total hydrocarbons |
55.3 |
65.5 |
65.16 |
68.8 |
77.5 |
75.7 |
74.7 |
67.4 |
|
|
|
|
Oxygenated monoterpenes |
43.4 |
33.0 |
33.46 |
28.4 |
21.2 |
23.4 |
24.8 |
30.5 |
|
|
|
|
Oxygenated sesquiterpenes |
0.959 |
0.410 |
0.21 |
0.510 |
0.620 |
0.32 |
0.040 |
0.26 |
|
|
|
|
Total oxygenated hydrocarbons |
44.3 |
33.4 |
33.67 |
29.0 |
21.8 |
23.72 |
24.9 |
30.8 |
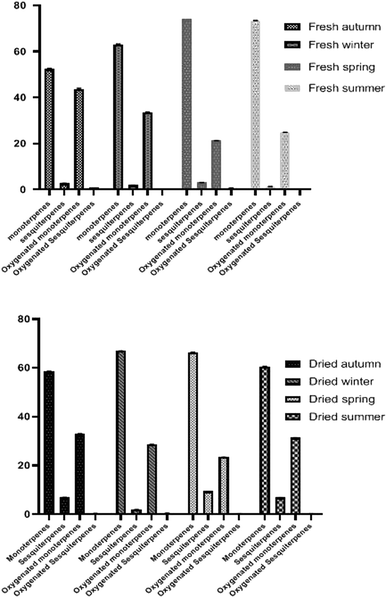 |
| Fig. 2 Histograms showing the percentage of different classes of oil constituents of Valencia orange leaves. (a) Fresh leaves oil samples (b) dried leaves oil samples. | |
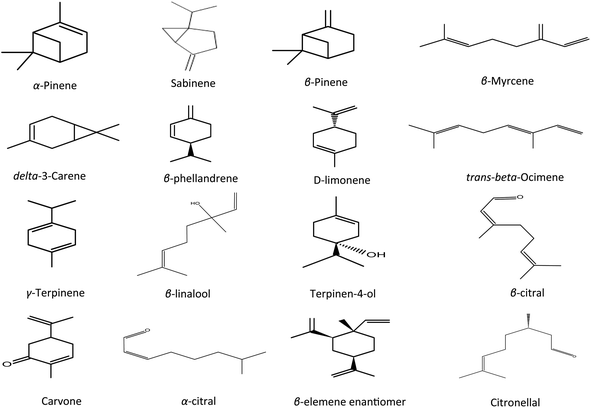 |
| Fig. 3 Chemical structure of the major components of the essential oils obtained from the leaves of Valencia orange. | |
3.3. Seasonal metabolic discrimination of Valencia orange leaves using multivariate data analysis
Chemometric analysis was done using unsupervised pattern recognition techniques via Hierarchical cluster analysis (HCA) and Principal component analysis (PCA) as illustrated in (Fig. 4). This was done relied upon the data obtained from GC analyses of the essential oils of Valencia orange fresh and dried leaves gathered in the four seasons. This was performed to give a discriminant insight into all the data gathered for the samples and to categorize them into different clusters based upon the similarities and differences in the chemical profile of the volatile constituents predominating in the oils that greatly influence their biological activity.35,40 Results of PCA and HCA illustrated in (Fig. 4) showed clear discrimination of the tested samples reflecting the effect of different seasons and conditions on the composition of essential oils. Through analysis of the score plot obtained from PCA, actually six principle components were extracted where PC1 and PC2 accounted for 46% and 26% of the total variances, respectively constituting 72% of the total variances, meanwhile PC3, PC4, PC5 and PC6 represented 12%, 9%, 4% and 2%, respectively and thus all of the extracted PCs accounted for 99% of the total variances (Fig. 4a). The multivariate data analysis performed on MS data revealed a significant separation among samples (Fig. 4a) with the majority of samples derived from fresh samples fresh spring (FS), fresh winter (FW), and fresh summer (FM) distinguished from the sample of fresh autumn (FA) located to the left of the vertical line representing PC1(negative PC1 values). PCA loading plots which define the most important components concerning the clustering behavior revealed that the differentiation was mainly linked to monoterpene hydrocarbons which were present at much higher levels in FS and FM as shown from PCA loading plots (Fig. 4b). In contrast, FA sample is more enriched in oxygenated monoterpenes. Three discriminate clusters were observed where essential oils obtained from (FW) and dried winter (DW) leaves were allocated in the same cluster together with that of dried summer leaves (DM) and thus reflecting the similarity in their essential oil composition and accounting for the minor effect of drying on the composition of winter leaves essential oils. However, FM and FS leaves are clustered together in one cluster in the upper right quadrant that in turn highlighted their closeness to their constituents that certainly affect their bioactivities. Similarly, dried Spring (DS) and dried Autumn (DA) leaves that are clustered together in one cluster in the upper left quadrant in contrast FA leaves sample that was scattered in the plot in the left lower quadrant. It is noteworthy to mention that both PC1 and PC2 effectively discriminate FM and FS leaves from FA leaves meanwhile PC1 differentiates FM and FS leaves from DS and DA leaves. Furthermore, PC2 distinguishes DS, DA, FM, and FS leaves from FW, DW, DM, and FA leaves. By comprehensive explanation of the loading plot illustrated in (Fig. 4b), it is obvious that the highest percentage of oxygenated monoterpenes in the FA sample (43.4%) could be responsible for its complete segregation from FS and FM with β-linalool and terpinen-4-ol as the major markers in FA essential oil (16.3% and 8.88% respectively). The similarity between DA and DS samples is the presence of sesquiterpenes at a higher percentage than in the other samples (6.90% and 9.45% respectively) where β-elemene enantiomer was the major marker in both samples (4.20 and 5.70% for DA and DS respectively). FS and FM were located in the same cluster and differentiated from the other samples as they possess the highest amount of monoterpene hydrocarbons (74.4 and 73.3% for FS and FM respectively) and β-phellandrene was the leading metabolite discriminating this cluster from the others. Sabinene was the major monoterpene of the third cluster (FW, DW, and DM) with percentages of 23.8%, 29.2%, and 18.8%, respectively. The results obtained from PCA were further confirmed by HCA (Fig. 4c) where the samples are clustered into three main clusters as revealed by the HCA dendrogram forming cluster 1 (DA and DS); cluster 2 (FS and FM); cluster 3 (FW, DW, and DM) in contrast to FA that fails to cluster in any one of them and thus reflecting its discriminant nature. From the results obtained from the chemometric analysis, it can be concluded that both season and condition could affect the essential oil secondary metabolites and thus their activities. This comes in accordance with many studies that showed the effect of seasonal variation on the diversity of secondary metabolites.41,42
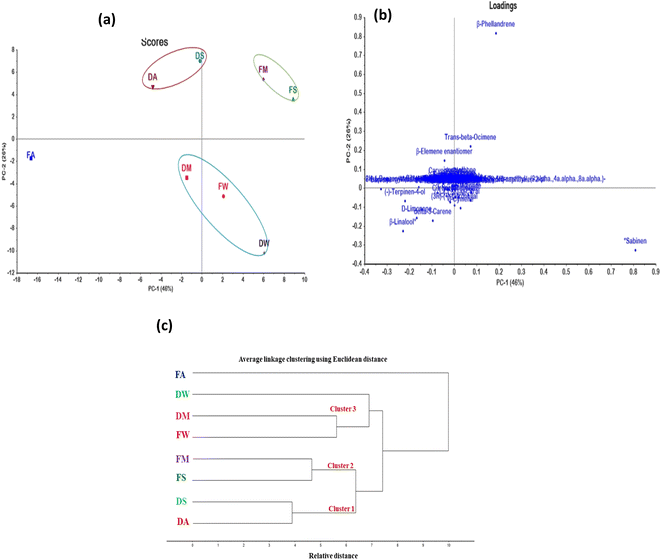 |
| Fig. 4 GC-based chemometrics analysis of Valencia orange leaves showing (a) score plot of PC1 versus PC2 scores; (b) loading plot for PC1 and PC2 with their assignments; (c) HCA; FA: fresh autumn leaves; FW: fresh winter leaves; FS: fresh spring leaves; FM: fresh summer leaves whereas DA: dried autumn leaves; DW: dried winter leaves; DS: dried spring leaves; DM: dried summer leaves. | |
3.4. Characterization of the nano-hexosomal formula
In the current study, EO-based larvicide was prepared in the form of nano-hexosomal particles, and the PS, PDI, and ZP were characterized. Notably, the PS determination is essential to enhance intracellular uptake. Basically, small-sized particles (267.8 nm) possess two folds higher uptake efficiency than large-size ones (567.7 nm).43 The prepared essential oil-loaded hexosomal nano-dispersions were found to have an average particle size of 150.5 ± 1.20 nm (Fig. 5a). The PDI of the prepared formula was 0.15, which means small and uniform size distributions and indicates that the hot emulsification method was the best one for its preparation.44 The small value of PDI that is below 5 was strongly correlated with the homogenous distribution of the formulation.45 The mean value of zeta potential was −27.4 ± 0.28 mV (Fig. 5b) where ZP of the prepared oil-loaded hexosomal nanoparticles was determined in order to ensure that the formula is stable. Larger values of zeta potential mean a lower probability of nanoparticles to aggregate which could be due to the repulsive forces between the surfaces of the particles.46 The negative values of ZP are attributed to the presence of free OA in the GMO, in addition to ionization of OA which was added during the preparation of the formulation.47 Transmission electron microscope (TEM) of the essential oil-loaded hexosomal nanodispersions was presented in (Fig. 5c), where nearly non-aggregated hexagonal nanoparticles were observed. The particle size of the image appears to be smaller than measured by zeta sizer as the dynamic light scattering is a multi-angle measuring technique that determines the hydrodynamic radius of the particle based on the intensity of the light scattering.
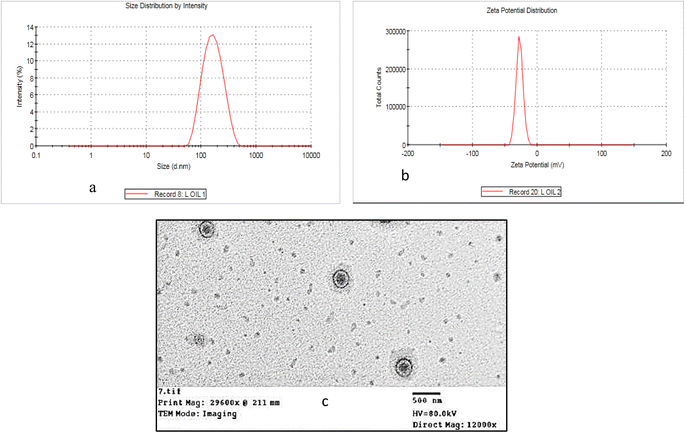 |
| Fig. 5 (a) Size distribution, (b) zeta potential, and (c) transmission electron microscopy photograph of the essential oil-loaded hexosomal nano-dispersions. | |
3.5. Determination of the insecticidal activity of Valencia orange spring leaves essential oil
Susceptibility of Cx. pipiens larva to the EO of fresh spring leaves and the oil loaded nano-hexosomes is presented in (Tables 3 and 4). Lethal concentrations killing 50 and 95% of the larvae (LC50 and LC95) for the unformulated EO were 48 and 30
552 mg L−1, respectively. In contrast, those of oil-loaded nano-hexosomal formula were 30 and 1830 mg L−1, respectively, equivalent to concentrations of 0.3 and 1.83 mg L−1 of pure EO (essential oil represents 1% of the oil-based formula). Noteworthy to mention that it is preferable for any insecticidal drug used to fight Cx. pipiens to possess certain water solubility as it is an aquatic insect which was difficult to achieve with the EOs.9 The lipoid nature of the EO could hinder the penetration of oil through the mosquito and thus it was critical to encapsulate the EO in a suitable formulation to enhance its insecticidal effect. Hexosomal nano-particles were the most convenient as GMO, which is one of its lipid components that is able to incorporate both hydrophilic and lipophilic constituents.48–50 Therefore, the EO was incorporated within the lipid portion of the nano-hexosomal dispersions, improving the bioavailability and activity of the EO. Other primary concerns are the volatile nature and instability of EO, which may lead to a decrease or even loss of the biological activity.51 Nano-encapsulation could minimize this loss and consequently enhance its activity via enhanced pharmacokinetics. In the foregoing study, a tiny amount of EO was consumed to formulate the nano-hexosomal dispersions, which in turn guarantee the safety and efficacy of the prepared formula.
Table 3 Effect to the formulated and unformulated essential oil of fresh spring leaves on the 3rd larval instar of Cx. pipiens (24 h post-treatment)
Unformulated oil of FSa |
Oil loaded nano-hexosomal formula |
Conc. (mg L−1) |
Mortality (Mean ± SEb) |
Conc. (mg L−1) |
Mortality (Mean ± SEb) |
Fresh spring leaves. Standard error; * significant (P < 0.05) differences from the control groups. |
13 |
38* ± 2.3 |
13 |
40* ± 0.8 |
21 |
42* ± 1.1 |
21 |
43* ± 3.5 |
25 |
47* ± 0.6 |
25 |
57* ± 0.5 |
Table 4 Susceptibility of 3rd larval instar of Cx. pipiens to the formulated and unformulated essential oil of fresh spring leaves (24 h post-treatment)
Tested sample |
LC50a and 95% confidence interval (mg L−1) |
LC95a and 95% confidence interval (mg L−1) |
Slope ± SEb |
X2 c |
Lethal concentrations value. Standard error. Chi square. |
Unformulated EO |
48 (1.17–2505) |
30 552 (2 × 10−8 to 2 × 1017) |
0.50 ± 1.1 |
0.30 |
EO loaded hexosomal nano-dispersion |
30 (9–106) |
1830 (3 × 10−2 to 1.5 × 108) |
0.90 ± 1.1 |
0.70 |
The insecticidal activity spring oil could be attributed to the major constituents of leaves (oxygenated and non-oxygenated). As mentioned previously, sabinene (27.4%) was the most abundant constituent, followed by delta-3-carene (10.3%), β-phellandrene (9.70%), and D-limonene (2.8%) as non-oxygenated monoterpenes. Linalool (10.2%), (−)-terpinen-4-ol (3.42%), and citronellal (1.43%) are the major oxygenated terpenes in this oil sample. Sabinene's effectiveness was proved as a repellent against adults of the granary weevil (Sitophilus granaries L.) at low concentrations.52 Linalool was reported as a repellent against mosquitoes (Aedes aegypti L.),53 Tribolium castaneum (H.), and Rhyzopertha dominica (F.).54 Fumigant toxicity of linalool, limonene, and β-pinene against fire ants (Solenopsis invicta B.) has also been reported.55 Limonene and citronellal of Citrus limon exerted a strong insecticidal action against Callosobruchus maculatus (F.).56 Due to the richness of Citrus EO with many constituents showing insecticidal action, thus synergism between these bioactive constituents may be responsible for the obtained larvicidal action.18
3.6. Molecular docking
The production of detoxification enzymes by insects is one of its defense mechanisms to maintain their normal physiological functions,57 such as glutathione-S-transferase, esterase, and phosphatase.58 Generally, EOs could act as a repellent and neurotoxic agents for insects through a diverse mechanism of action, including inhibition of acetylcholinesterase enzymes, GABA receptors antagonism in addition to acting as a ligand on octopamine receptors.59 The inhibition of acetylcholinesterase activity is the most critical mechanism as it causes major signs of intoxication, such as restlessness, convulsions, tremors, and paralysis60 Essential oil of Citrus sinensis peel causes some biochemical changes in the third instar larvae of Cx. pipiens via an increase in the levels of the acid phosphatase and alkaline phosphatase with a decrease in the level of acetylcholinesterase.61 Accordingly, in silico study was performed on the main volatile constituents identified in the EOs of Valencia orange leaves within the binding sites of acetylcholine esterase (PDB ID: 5X61; 3.40 Å). The α-citral showed the best fitting within the binding site displaying binding energies (ΔG) of −10.43 kcal mol−1, whereas citronellal showed mild fitting in the active centre with ΔG equals −2.32 kcal mol−1 (Table 5). In contrast, the rest of the tested compounds that showed positive values for binding energies that may account for the unfavourable interaction and the presence of steric hindrance at the active site. The tight binding of α-citral with the active centre is mainly attributed to the formation of multiple bonds with the allocated amino acid residues. α-Citral forms one conventional H-bond with Thr234; one π-alkyl bond with Trp441 in addition to many van der Waals interactions with the existing amino acid moieties (Fig. 6a). Citronellal forms one conventional H-bond with Cys 447; two-alkyl bonds with Tyr493 and Ile231; one alkyl interaction with Tyr282 in addition to van der Waals interactions (Fig. 6b). Interestingly, monoterpenoids were reported to have anticholinesterase properties, where citral, pulegone, gossypol, linalool, bornyl acetate, and cineole were reported as insecticidal for Tribolium castaneum and inhibitors for AChE.62 Additionally, citral of the essential oil of Citrus aurantifolia is potent larvicidal, ovicidal, and adulticidal against Aedes aegypti.63 Altogether, the molecular docking results further consolidated the bioassay results where acetylcholine esterase inhibition is one of the mechanisms some volatile compounds exert to kill the insects.
Table 5 Binding energies (Δg) of the main volatile constituents identified in the essential oils of Valencia orange leaves within the binding sites of acetylcholine esterase (PDB ID: 5X61; 3.40 Å)
Compounds |
Binding energy to the acetylcholine esterase (kcal mol−1) |
Citronellal |
−2.3 |
3-Carene |
18 |
α-Pinene |
7.4 |
α-Citral |
−10 |
β-Citral |
18 |
β-Elemene |
28 |
β-Myrcene |
14 |
β-Phellandrene |
2.7 |
Carvone |
15 |
β-Pinene |
5 |
D-Limonene |
23 |
γ-Terpinene |
25 |
β-Linalool |
12 |
Terpinen-4-ol |
14 |
trans-β-Ocimene |
21 |
Sabinene |
13 |
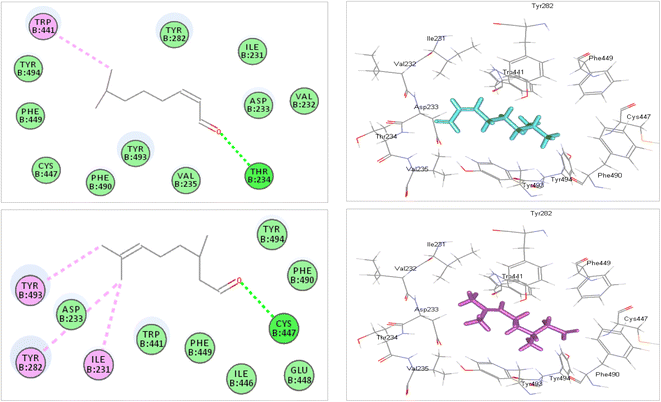 |
| Fig. 6 2D and 3D binding mode of α-citral (a) and citronellal (b) identified in the essential oils of Valencia orange leaves within the binding sites of acetylcholine esterase (PDB ID: 5X61; 3.40 Å). | |
4 Conclusion
The chemical profile of EOs obtained from fresh and dried Valencia orange leaves in each season disclosed a significant variation in the distribution and relative percentages of many constituents. Seventy-seven compounds were detected in all oil samples with sabinene represented the predominant monoterpene hydrocarbon in all samples. Oxygenated monoterpenes were the second major class of volatile constituents, with β-linalool as the chief oxygenated monoterpene in all samples, followed by citronellal, terpinen-4-ol, and β-citral. Chemometric analysis successfully segregated fresh and dried leaves of Valencia orange gathered in various seasons into three main groups which confirm that both seasonal variation and sample condition affect the volatile oil composition. The nano-hexosomal formula of EO obtained from the FS sample exerted a more potent insecticidal action than oil alone which ensures the role of nanoencapsulation of essential oil to increase biological activity. Oxygenated monoterpenes play a major role in fighting Cx. pipiens via inhibition of acetylcholine esterase enzyme as confirmed by molecular docking. Additionally, the observed variations between the analyzed samples and the published literature may be attributed to climatic or geographical factors. Further GC/FID analysis of the essential oil samples could be required in order to investigate any quantitative variation between oil samples as result of different seasons or drying effect. In another phase, we will optimize the hydrodistillation method using surface response method. In another phase, different extraction techniques will be compared such as conventional steam distillation and microwave assisted hydrodistillation. For the best extraction method, different kinetics extraction model will be applied and compared.
Author contributions
Eman M. Abd Elghani: writing – original draft, data curation, formal analysis, investigation, resources, methodology. Abeer M. El Sayed: conceptualization, supervision, data curation, formal analysis, writing – review & editing, writing – original draft. Marwa M. Abdel-Aziz Emam: data curation, supervision, writing – review & editing. Abdulaziz M. Al-Mahallawi: data curation, formal analysis. Soad H. Tadros: supervision, writing – review & editing. Fathy M. Soliman: conceptualization, supervision, writing – review & editing. Fadia S. Youssef: data curation, formal analysis, software, writing – review & editing, methodology.
Conflicts of interest
There are no conflicts to declare.
Acknowledgements
We are grateful to Dr Mohammed N. A. Khalil, Associate Professor of Natural Products Chemistry, Pharmacognosy Department, Faculty of Pharmacy, Cairo University for his guidance.
References
- D. A. Ewing, B. V. Purse, C. A. Cobbold, S. M. Schafer and S. M. White, Parasit Vectors, 2019, 12, 1–19 CrossRef PubMed , https://parasitesandvectors.biomedcentral.com/articles/10.1186/s13071-019-3321-2.
- C. J. Smith and T. A. Perfetti, Toxicol. Res., 2020, 4, 1–15, DOI:10.1177/2F2397847320940561.
- J. Liu, J. Hua, B. Qu, X. Guo, Y. Wang, M. Shao and Sh. Luo, Front. Plant Sci., 2021, 12, 720816, DOI:10.3389/fpls.2021.720816.
- U. Burcu, S. Fazli, A. Ozgur, S. O. Birsen and O. K. Elif, Int. J. Food Sci., 2011, 46, 1455–1461, DOI:10.1111/j.1365-2621.2011.02640.x.
- A. A. Mohamed, G. A. El-Emary and H. F. Ali, J. Am. Sci., 2010, 6, 820–826 Search PubMed.
- J. M. J. Favela-Hernandez, O. Gonźalez-Santiago, M. RamırezCabrera, P. Esquivel-Ferrĩno and M. Camacho-Corona, Molecules, 2016, 21, 247, DOI:10.3390/molecules21020247.
- B. Khanikor, K. Adhikari and B. Rabha, Citrus Essential Oils: A Suite of Insecticidal Compounds, in Citrus – Research, Development and Biotechnology, ed. M. S. Khan and I. A. Khan, IntechOpen, 2021, DOI:10.5772/intechopen.95887.
- F. Adusei-Mensah, E. I. Inkum, C. M. Agbale and A. Eric, Int. J. Novel Res. Eng. Sci., 2014, 1, 19–25 Search PubMed.
- R. M. Azmy, E. El Gohary, D. M. Mahmoud, D. Salem, M. A. Abdou and M. S. Salama, Egypt. J. Aquat. Biol. Fish., 2019, 23, 61–67 CrossRef.
- H. S. Kusuma and M. Mahfud, The extraction of essential oils from patchouli leaves (Pogostemon cablin benth) using a microwave air-hydrodistillation method as a new green technique, RSC Adv., 2017, 7(3), 1336–1347, 10.1039/c6ra25894h.
- H. S. Kusuma and M. Mahfud, Comparison of kinetic models of oil extraction from sandalwood by microwave-assisted hydrodistillation, Int. Food Res. J., 2017, 24(4), 1697–1702 CAS.
- H. S. Kusuma, T. I. Rohadi, E. F. Daniswara, A. Altway and M. Mahfud, Preliminary Study: Comparison of Kinetic Models of Oil Extraction from Vetiver (Vetiveria Zizanioides) by Microwave Hydrodistillation, Korean Chem. Eng. Res., 2017, 55(4), 574–577 CAS.
- H. H. A. Rassem, A. H. Nour and R. M. Yunus, Techniques For Extraction of Essential Oils From Plants: A Review, Aust. J. Basic Appl. Sci., 2016, 10(16), 117–127 CAS.
- J. O. Werdin González, M. M. Gutiérrez, A. A. Ferrero and B. Fernandez Band, Chemosphere, 2014, 100, 130–138, DOI:10.1016/j.chemosphere.2013.11.056.
- O. Campolo, A. Cherif, M. Ricupero, G. Siscaro, K. Grissa-Lebdi, A. Russo, L. M. Cucci, P. Pietro, C. Satriano, N. Desneux, A. Biondi, Z. Lucia and V. Palmeri, Sci. Rep., 2017, 7, 13036, DOI:10.1038/s41598-017-13413-0.
- K. A. Khalid, A. M. A. Ahmed and A. E. El-Gohary, J. Essent. Oil Res., 2020, 32, 1–12, DOI:10.1080/10412905.2020.1749947.
- B. B. Darjazi, Int. J. Adv. Biol. Biomed. Res., 2017, 5, 98–107 Search PubMed.
- L. A. Usman, I. R. Olanrewaj, O. Watti and A. O. Ojumoola, J. Turk. Chem. Soc., 2016, 3, 1–18, DOI:10.18596/jotcsa.98689.
- Central Administration of Pharmaceutical Affairs (CAPA), Egyptian Pharmacopoeia, Ministry of Health and Population, Cairo, Egypt, 4th edn, 2005 Search PubMed.
- E. Guenther, The Essential Oils. D. Van Nostrand Company, Princeton, New Jersey: New York I, 1960, pp. 237–306 Search PubMed.
- H. S. Kusuma and M. Mahfud, Microwave-assisted hydrodistillation for extraction of essential oil from Patchouli (Pogostemon cablin) leaves, Period. Polytech., Chem., 2017, 61, 82–92, DOI:10.3311/PPch.8676.
- R. P. Adams, Identification of Essential oil Components by Gas Chromatography/Mass Spectrometry, Allured Business Media, Carol Stream, Illinois: USA, 4th edn, 2009 Search PubMed.
- F. S. Youssef, R. M. Labib, H. A. Gad, S. Y. Eid, M. L. Ashour and H. H. Eid, Pimenta dioica and Pimenta racemosa: GC-based metabolomics for the assessment of seasonal and organ variation in their volatile components, in silico and in vitro cytotoxic activity estimation, Food Funct., 2021, 12, 5247–5259, 10.1039/d1fo00408e.
- J. Zhai, C. Fong, N. Tran and C. J. Drummond, ACS Nano, 2019, 13, 6178–6206, DOI:10.1021/acsnano.8b07961.
- M. M. Gabr, M. S. M. ortada and M. A. Sallam, J. Pharm. Sci., 2017, 106, 3103–3112, DOI:10.1016/j.xphs.2017.04.060.
- H. S. El Said, A. Lalatsa, A. M. Al-Mahallawi, E. S. El Leithy and D. M. Ghorab, Int. J. Pharm., 2022, 625, 122080, DOI:10.1016/j.ijpharm.2022.122080.
- E. M. Abd Elghani, F. A. Omar, M. M. Emam, A. M. Al-Mahallawi, S. H. Tadros, F. M. Soliman and A. M. ElSayed, Nat. Prod. Res., 2022, 1–6, DOI:10.1080/14786419.2022.2106484.
- A. M. Fahmy, M. Hassan, D. A. El-Setouhy, S. A. Tayel and A. M. Al-Mahallawi, Drug Delivery, 2021, 28, 77–86, DOI:10.1080/10717544.2020.1858997.
- E. Gerberg, Bulletin 5, Manual for mosquito rearing and experimental techniques, American Mosquito Control Association, Lake Charles, Louisiana, USA, 1970 Search PubMed.
- M. Kasap and L. Demirhan, The effect of various larval foods on the rate of adult emergence and fecundity of mosquitoes, Turk. Parazitolojii Derg., 1992, 161, 87–97 Search PubMed.
- WHO 2005, Guidelines for laboratory and field testing of mosquito larvicides (WHO/CDS/WHOPES/GCDPP/2005.13), http://www.who.int/whopes/gcdpp/publications/en/index1.html.
- D. Finney, Probit analysis, Cambridge University Press, Third edn, 1971, p. 333 Search PubMed.
- R. M. Labib, S. S. Ebada, F. S. Youssef, M. L. Ashour and S. A. Ross, Pharmacogn. Mag., 2016, 12(48), 319–325, DOI:10.4103/0973-1296.192207.
- A. N. Talaat, S. S. Ebada, R. M. Labib, A. Esmat, F. S. Youssef and A. B. Singab, J. Ethnopharmacol., 2018, 226, 44–47, DOI:10.1016/j.jep.2018.07.026.
- A. E. Altyar, M. L. Ashour and F. S. Youssef, Biomolecules, 2020, 10, 879, DOI:10.3390/biom10060879.
- Z. Liang-Liang, L. Shuang, X. Jian-Guo and Z. Li-Fang, Nat. Prod. Res., 2018, 32, 1184–1188, DOI:10.1080/14786419.2017.1320791.
- L. J. Danielli, T. de Souza, A. J. Maciel, M. F. Ferrão, A. M. Fuentefria and M. A. Apel, Biomolecules, 2019, 9, 112, DOI:10.3390/biom9030112.
- R. Farahmandfar, B. Tirgarian, B. Dehghan and A. Nemati, Food Sci. Nutr., 2019, 8, 124–138 CrossRef.
- A. W. Ismanto, H. S. Kusuma and M. Mahfud, Solvent-free microwave extraction of essential oil from Melaleuca leucadendra L., MATEC Web of Conferences, 2018, vol. 156, p. 03007 Search PubMed.
- A. K. Kammoun, A. E. Altyar and H. A. Gad, J. Pharm. Biomed. Anal., 2021, 198, 113991, DOI:10.1016/j.jpba.2021.113991.
- J. A. Llorens-Molina, S. Vacas, V. Castell and M. Verdeguer, J. Essent. Oil Res., 2020, 32(5), 419–428, DOI:10.1080/10412905.2020.1773328.
- N. Badalamenti, M. Bruno, R. Schicchi, A. Geraci, M. Leporini, L. Gervasi, R. Tundis and M. R. Loizzo, Molecules, 2022, 27, 1580, DOI:10.3390/molecules27051580.
- J. Um, H. Chung, K. Kim, I. Kwon and S. Jeong, Int. J. Pharm., 2003, 253, 71–80 CrossRef.
- M. M. Gabr, S. M. Mortada and M. A. Sallam, J. Pharm. Sci., 2017, 106, 3103–3112, DOI:10.1016/j.xphs.2017.04.060.
- H. Chavda, J. Patel, G. Chavada, S. Dave, A. Patel and C. Patel, J. Powder Technol., 2013, 108569, DOI:10.1155/2013/108569.
- D. Mahmoud, W. Ismail, Y. Moatasim, O. Kutkat, A. El Meshad, Sh. Ezzat, K. El Deeb, A. Fishawy, M. Gomaa, A. Kandeil, A. Al-karmalawy, M. Ali and A. Mostafa, J. Drug Delivery Sci. Technol., 2021, 66, 102845, DOI:10.1016/j.jddst.2021.102845.
- H. M. Abdel-Bar, S. E. Khater, D. M. Ghorab and A. M. Al-Mahallawi, ACS Omega, 2020, 5, 26697–26709, DOI:10.1021/acsomega.0c03569.
- M. Nasr, M. K. Ghorab and A. Abdelazem, Acta Pharm. Sin. B, 2019, 5, 79–88 CrossRef.
- Z. Karami and M. Hamidi, Drug Discovery Today, 2016, 21, 789–801, DOI:10.1016/j.drudis.2016.01.004.
- S. V. Rao, B. N. Sravya and K. Padmalatha, GSC Biol. Pharm. Sci., 2018, 5, 76–81 CrossRef.
- C. Cimino, O. M. Maurel, T. Musumeci, A. Bonaccorso, F. Drago, E. M. B. Souto, R. Pignatello and C. Carbone, Pharmaceutics, 2021, 13, 327 CrossRef PubMed.
- S. H. Zhou, C. Wei, C. H. Zhang, C. Han, N. Kuchkarova and H. Shao, Toxins, 2019, 11, 598 CrossRef.
- G. C. Müller, A. Junnila, J. Butler, V. D. Kravchenko, E. E. Revay, R. W. Weiss and Y. Schlein, J. Vector Ecol., 2009, 34, 2–8, DOI:10.1111/j.1948-7134.2009.00002.x.
- D. A. Ukeh and S. B. A. Umoetok, Crop Prot., 2011, 30, 1351–1355, DOI:10.1016/j.cropro.2011.05.016.
- W. Hu, N. Zhang, H. Chen, B. Zhong, A. Yang, F. Kuang, Z. Ouyang and J. Chun, J. Econ. Entomol., 2017, 110, 1556–1562 CrossRef.
- A. K. Sahla and E. Pushpalatha, Int. J. Recent Sci. Res., 2020, 11, 39095–39099, DOI:10.24327/ijrsr.2020.1107.5450.
- X. Z. Li and Y. H. Liu, Acta Ecol. Sin., 2007, 50, 989–995 Search PubMed.
- A. Zibaee, I. Zibaee and J. J. Sendi, Pestic. Biochem. Physiol., 2011, 100, 289–298 CrossRef.
- M. Jankowska, J. Rogalska, J. Wyszkowska and M. Stankiewicz, Molecules, 2018, 23, 34 CrossRef.
- P. Rao, D. Goswami and R. M. Rawal, Sci. Rep., 2021, 11, 17474, DOI:10.1038/s41598-021-96963-8.
- R. M. Azmy, E. El Gohary, D. A. Salem, M. A. Abdou, M. S. Salama and D. M Mahmoud, Aquat. Insects, 2021, 42, 78–90, DOI:10.1080/01650424.2020.1871025.
- M. D. López and M. J. Pascual-Villalobos, Ind. Crops Prod., 2010, 31, 284–288, DOI:10.1016/j.indcrop.2009.11.005.
- R. Sarma, K. Adhikari, S. Mahanta and B. Khanikor, Toxicol. Rep., 2019, 6, 1091–1096, DOI:10.1016/j.toxrep.2019.10.009.
|
This journal is © The Royal Society of Chemistry 2023 |
Click here to see how this site uses Cookies. View our privacy policy here.