DOI:
10.1039/D3QO00909B
(Research Article)
Org. Chem. Front., 2023,
10, 4658-4664
An unusual four-step cascade reaction for accessing furo[3,4-c]pyridine-1,4-diones via rhodium catalysis†
Received
19th June 2023
, Accepted 5th August 2023
First published on 15th August 2023
Abstract
The development of efficient cascade reactions is highly important and appealing because of their desirable step-economy and convenience in constructing multiple chemical bonds and complex molecules in one shot. Herein, we report a rare four-step tandem reaction between acrylamides and 4-hydroxy-2-alkynoates to prepare novel furo[3,4-c]pyridine-1,4-diones which are difficult to synthesize by traditional methods. This unique domino reaction includes C–H activation, Lossen rearrangement, annulation, and lactonization. Additionally, this protocol features good functional group tolerance, obtainment of products by simple filtration, room temperature, and air compatibility. DFT calculations were conducted to shed some light on the reaction mechanism.
Introduction
The prevalence of heterocycles in natural products and pharmaceuticals has led to the importance and benefits of synthesizing such compounds efficiently. In the past decades, directing group (DG) assisted C–H activation/annulation reactions via transition-metal catalysis for the synthesis of heterocycles have achieved tremendous advances.1,2 DGs play crucial roles in controlling chemical reactivity and regioselectivity in C–H activation reactions. On the other hand, DGs simply adhere to primordial positions and function as auxiliary groups in these reactions without other transformations. Amide-type DGs are frequently applied to C–H activation/annulation reactions for the synthesis of prevalent isoquinolones and 2-pyridones, where DGs remain static in most cases (Scheme 1a).1,2a–f,3 The rearrangement or migration of DGs in C–H activation reactions provides an intriguing strategy to construct C–C/C–N bonds and also contributes to the synthesis of novel heterocyclic scaffolds.4,5 Therefore, it is fascinating to apply this emerging strategy to organic synthesis. Nevertheless, the development of this emerging strategy involving DG rearrangement or migration still falls far behind when compared to the numerous reports of static DGs participating in C–H activation reactions.
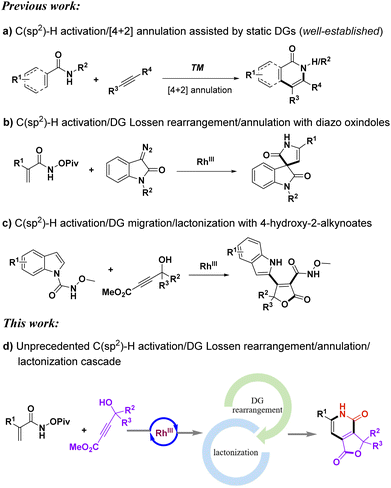 |
| Scheme 1 Transition-metal catalyzed C–H activation/annulation reactions. | |
Even though DGs could improve chemoselectivity and regioselectivity, they may be present as undesired moieties on products at times, and additional steps are required to remove unwanted DGs. If DGs undergo a rearrangement process, it will not only create a new and efficient avenue for synthesizing heterocycles, but also possibly generate novel heterocycles that traditional approaches can hardly furnish. By taking advantage of the strategy of directing group rearrangement, a series of intriguing spirooxindole pyrrolones were synthesized elegantly by Dai's group (Scheme 1b).6 Acrylamides involving the rearrangement of DGs undergo three processes: C–H activation, directing group rearrangement, and annulation. Recently, we developed an efficient rhodium-catalyzed domino C–H alkenylation, DG migration, and lactonization reaction between N-carbamoyl indoles and 4-hydroxy-2-alkynoates for the synthesis of the furan-2(5H)-one scaffold (Scheme 1c).7 Efficient cascade reactions are highly appealing due to their desirable step-economy and convenience in constructing complex molecules with multiple chemical bonds in one shot.8–11
To our knowledge, the implementation of a multistep cascade reaction involving four distinct processes of C–H activation, directing group rearrangement, annulation, and lactonization has yet to be documented. In this study, we report a rare four-step tandem reaction between acrylamides and 4-hydroxy-2-alkynoates12 to prepare novel furo[3,4-c]pyridine-1,4-diones which are difficult to synthesize by traditional methods. The four-step tandem reaction involves C–H activation, DG Lossen rearrangement, [4 + 2] annulation, and lactonization. The method can tolerate a variety of functional groups. Furthermore, the products can be easily purified by filtration and the reaction is compatible with both room temperature and air. These features contribute to the practicality and versatility of the protocol.
Results and discussion
We embarked on our studies with the optimization of the reaction conditions for the cyclization between acrylamide 1a and ethyl 4-hydroxy-4-phenylbut-2-ynoate 2a. No desired product 3a was obtained when catalyzed by MnBr(CO)5, [RuCl2(p-cym)]2, Cp*Co(CO)I2 and Pd(OAc)2, respectively (Table 1, entries 1–6). Switching to [Cp*IrCl2]2 led to only 6% yield (entry 2). In contrast, the yield of 3a was improved to 73%, and 43% of the product could be obtained by simple filtration when using [Cp*RhCl2]2 (entry 5). The structure of 3a was confirmed using 1D-NMR and NOESY experiments as well as mass spectra. Solvent screening revealed that TFE outperformed other common solvents, such as THF (only trace), MeOH (15% yield), DCE (32% yield with 40% substrate not being consumed) and EA (20% yield) (entries 7–11). When using ethanol and adding Na2CO3 as a base, the yield of 3a was 0% (entry 12). After extensive screening of additives, such as Na2CO3, K2CO3, CsOAc, and KOAc, Na2CO3 was identified as the optimal one (entries 13–16). The control experiments confirmed that no desired reaction occurred in the absence of an additive or rhodium (entries 17 and 18).
Table 1 Optimization of reaction conditionsa

|
Entry |
Catalyst |
Additive |
Solvent |
Yieldb,c (%) |
Reaction conditions: 1a (0.2 mmol), 2a (0.24 mmol), catalyst (3 mol%), additive (0.2 mmol), under air, solvent (1.0 mL), room temperature, 6 h.
Total isolated yield.
Isolated yields obtained by simple filtration are shown in parenthesis.
Na2CO3 was not used.
[Cp*RhCl2]2 was not used.
|
1 |
MnBr(CO)5 |
NaOAc |
TFE |
0 |
2 |
[Cp*IrCl2]2 |
NaOAc |
TFE |
6 |
3 |
Cp*Co(CO)I2 |
NaOAc |
TFE |
0 |
4 |
[Ru(p-cym)Cl2]2 |
NaOAc |
TFE |
0 |
5 |
[Cp*RhCl2]2 |
NaOAc |
TFE |
73 (47) |
6 |
Pd(OAc)2 |
NaOAc |
TFE |
0 |
7 |
[Cp*RhCl2]2 |
NaOAc |
THF |
Trace |
8 |
[Cp*RhCl2]2 |
NaOAc |
MeOH |
15 |
9 |
[Cp*RhCl2]2 |
NaOAc |
DCE |
32 |
10 |
[Cp*RhCl2]2 |
NaOAc |
CH3CN |
0 |
11 |
[Cp*RhCl2]2 |
NaOAc |
EA |
20 |
12 |
[Cp*RhCl2]2 |
Na2CO3 |
EtOH |
0 |
13 |
[Cp*RhCl2]2 |
Na2CO3 |
TFE |
78 (53) |
14 |
[Cp*RhCl2]2 |
K2CO3 |
TFE |
19 |
15 |
[Cp*RhCl2]2 |
CsOAc |
TFE |
54 (37) |
16 |
[Cp*RhCl2]2 |
KOAc |
TFE |
46 (33) |
17d |
[Cp*RhCl2]2 |
— |
TFE |
0 |
18e |
— |
Na2CO3 |
TFE |
0 |
With the optimal conditions in hand, we next examined the scope of this cyclization reaction (Scheme 2). Aryl-substituted propynol bearing electron-donating groups are all tolerated in this system, such as CH3, t-Bu, OMe, and CH2CH3, and the corresponding products can be obtained in high yields by direct filtration (3a–3e). To our delight, electron-withdrawing groups including F, Cl, Br, and I were also tolerated well with the isolated yields ranging from 62% to 72% (3f–3i). Although the yield of the product dropped dramatically when the group was changed to cyclopentyl (3k), the reaction was compatible with other different rings (naphthalene, furan, and thiophene) and afforded the products smoothly (3j, 3l, and 3m). We also explored tertiary alkynol and obtained the desired product in a moderate yield (3n). Next, we investigated R1 of acrylamide 1 bearing electron-donating and electron-withdrawing groups at the para position of the phenyl group, and the reactions proceeded well to generate the corresponding products. In comparison, when the phenyl group was changed to the benzyl and methyl group, the yield dropped to 47% and 20%, respectively (3o–3u). Additionally, the structures of 3h, 3n, 3p, and 3q were further confirmed by NOESY experiments.
 |
| Scheme 2 Substrate scope. Reaction conditions: 1 (0.2 mmol), 2 (0.24 mmol), [Cp*RhCl2]2 (3 mmol%), Na2CO3 (0.2 mmol), under air, trifluoroethanol (1.0 mL), rt, 6–12 h. Total yields are shown, and yields obtained by simple filtration are shown in parentheses. | |
Several experiments were conducted to probe the reaction mechanism. In the presence of 1a, Na2CO3, [Cp*RhCl2]2, and 1.0 ml methanol-d4, the experiment of H/D exchange occurring at the olefinic bond of acrylamide was performed (Scheme 3a). And no obvious deuterium incorporation was observed at this position (8% D). In addition, there was also almost no D at the olefinic bond of 3a after adding 2a. The result demonstrated that the cleavage of the C–H bond could be irreversible. Additionally, two parallel reactions were performed giving a KIE value of 0.98 (Scheme 3b), which suggested that the C–H activation step was less likely to be involved in the rate-determining step.
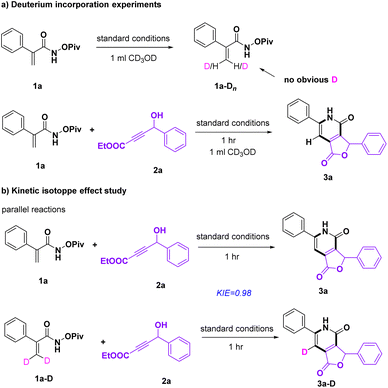 |
| Scheme 3 Deuteration and KIE experiments. | |
To further obtain the mechanistic features of the unprecedented Rh(III)-catalyzed sequential C–H activation/Lossen rearrangement/[4 + 2] annulation/lactonization cascade, we next performed DFT calculations on the key reaction steps: alkyne insertion, Lossen rearrangement, [4 + 2] annulation and lactonization. As shown in Scheme 4, the five-membered rhodacycle INT-0 derived from C–H activation was rationally selected as the starting point, which coordinated with propargyl alcohol 2a and produced the intermediate INT-1 (ΔG‡ = −11.3 kcal mol−1) involving hydrogen-bonding between the hydroxyl group of 2a and the pivalate group. Then the C–C unsaturated bond of 2a was inserted into the Rh–C bond viaTS-1 (ΔG‡ = −4.6 kcal mol−1) to deliver INT-2 with a free energy of −35.8 kcal mol−1. The subsequent facile coordination change viaTS-2 (ΔG‡ = −28.1 kcal mol−1) produced the unstable intermediate INT-3 which afforded different reaction paths. The concerted Lossen rearrangement/N–O bond cleavage from INT-3 occurred viaTS-3 (ΔG‡ = −26.9 kcal mol−1) which demanded an energy barrier of 8.9 kcal mol−1 (from INT-2 to TS-3) to give the intermediate INT-4 along with a considerable quantity of heat. The relatively elongated N–O1 bond (1.48 Å vs. 1.42 Å in INT-0) in the geometry of INT-3 and the significantly elongated N–O1 bond (2.41 Å) and shortened C1–N bond (1.98 Å) in the transition state TS-3 suggest that the Lossen rearrangement was assisted by the pivalate group and the rhodium center. Alternatively, classic C–N bond reductive elimination viaTS-3ii (ΔG‡ = −14.7 kcal mol−1) involved a higher energy barrier of 21.1 kcal mol−1 (from INT-2 to TS-3ii). Moreover, the Rh(III)–Rh(V)–Rh(III) reaction path involving an oxidative addition process from INT-3 was also ruled out owing to the relatively high free energies of INT-4i/TS-4i. After low-barrier isomerization viaTS-4, INT-4 was transformed into the isocyanate intermediate INT-5 from which the direct C–C bond formation/annulation occurred viaTS-5i (ΔG‡ = −50.2 kcal mol−1) and required a barrier of 22.4 kcal mol−1 (from INT-5 to TS-5i). However, the coordination change viaTS-5/TS-6 led to a more favorable C–C bond formation reaction viaTS-7 (ΔG‡ = −55.1 kcal mol−1) which only needs an activation energy of 17.5 kcal mol−1. Afterwards, the protonolysis reaction viaINT-9/TS-8 was followed by lactonization viaTS-9 (ΔG‡ = −81.0 kcal mol−1). Alternatively, the metal-free lactonization without the rhodium complex goes through TS-9i (ΔG‡ = −60.2 kcal mol−1) which is of a much higher barrier and can be ruled out.
 |
| Scheme 4 DFT calculations of the reaction pathways. | |
In order to shed light on the driving force of the Lossen rearrangement, we examined closely the geometry change along the intrinsic reaction coordinate (IRC) corresponding to TS-3 (see the ESI for details†). The results revealed that at the beginning the N–O1 bond distance increases which seems to drive the C1 atom attack on the N atom. The reaction goes through TS-3 and then the N–O1 bond breaks which is followed by C1–N bond formation and C1–C2 bond cleavage. This gives a chance for the O1 atom to attack the positively charged C2 atom. With the assistance of the pivalate group and the rhodium center, the Lossen rearrangement involves multiple σ-bond metathesis processes.
Conclusions
In summary, we have developed a rare four-step tandem reaction between acrylamides and 4-hydroxy-2-alkynoates to prepare novel furo[3,4-c]pyridine-1,4-diones. This unique tandem reaction consists of C–H activation, Lossen rearrangement, [4 + 2] annulation, and lactonization. The protocol features good functional group tolerance, obtainment of pure products by simple filtration, room temperature, and air compatibility. In addition, DFT calculations were conducted to shed some light on the reaction mechanism.
Conflicts of interest
There are no conflicts to declare.
Acknowledgements
Financial support from the Basic and Applied Basic Research Foundation of Guangdong Province (2021A1515110468), Shanghai Pujiang Program (21PJ1415800), Natural Science Foundation of Guangdong Province (2019A1515010935), High-level New R&D Institute (2019B090904008), High-level Innovative Research Institute (2021B0909050003), and NSFC (21877020 and 22007020) is gratefully acknowledged. We also thank Wei Zhang from the Zhongshan Institute for Drug Discovery for X-ray crystallographic analysis.
References
-
(a) P. Gandeepan, T. Müller, D. Zell, G. Cera, S. Warratz and L. Ackermann, 3d Transition Metals for C–H Activation, Chem. Rev., 2019, 119, 2192–2452 CrossRef CAS PubMed;
(b) D. J. Abrams, P. A. Provencher and E. J. Sorensen, Recent applications of C-H functionalization in complex natural product synthesis, Chem. Soc. Rev., 2018, 47, 8925–8967 RSC;
(c) C. Sambiagio, D. Schönbauer, R. Blieck, T. D. Huy, G. Pototschnig, P. Schaaf, T. Wiesinger, M. F. Zia, J. W. Delord, T. Besset, B. U. W. Maes and M. Schnürch, A comprehensive overview of directing groups applied in metal-catalysed C-H functionalisation chemistry, Chem. Soc. Rev., 2018, 47, 6603–6743 RSC;
(d) Y. Yang, J. Lan and J. You, Oxidative C-H/C-H Coupling Reactions between Two (Hetero)arenes, Chem. Rev., 2017, 117, 8787–8863 CrossRef CAS PubMed;
(e) T. Gensch, M. N. Hopkinson, F. Glorius and J. W. Delord, Mild metal-catalyzed C-H activation: examples and concepts, Chem. Soc. Rev., 2016, 45, 2900–2936 RSC;
(f) P. Augustin, G. Catherine and C. Laurent, Recent Advances in [Cp*MIII] (M = Co, Rh, Ir)-Catalyzed Intramolecular Annulation Through C–H Activation, Eur. J. Org. Chem., 2018, 5777–5794 Search PubMed;
(g) D. Mandal, S. Roychowdhury, J. P. Biswas, S. Maiti and D. Maiti, Transition-metal-catalyzed C-H bond alkylation using olefins: recent advances and mechanistic aspects, Chem. Soc. Rev., 2022, 51, 7358–7426 RSC;
(h) L. Ackermann, Metalla-electrocatalyzed C-H Activation by Earth-Abundant 3d Metals and Beyond, Acc. Chem. Res., 2020, 53, 84–104 CrossRef CAS PubMed.
-
(a) S. Mochida, N. Umeda, K. Hirano, T. Satoh and M. Miura, Rhodium-catalyzed Oxidative Coupling/Cyclization of Benzamides with Alkynes via C–H Bond Cleavage, Chem. Lett., 2010, 39, 744–746 CrossRef CAS;
(b) T. K. Hyster and T. Rovis, Rhodium-Catalyzed Oxidative Cycloaddition of Benzamides and Alkynes via C−H/N−H Activation, J. Am. Chem. Soc., 2010, 132, 10565–10569 CrossRef CAS PubMed;
(c) N. Guimond, C. Gouliaras and K. Fagnou, Rhodium(III)-catalyzed isoquinolone synthesis: the N-O bond as a handle for C-N bond formation and catalyst turnover, J. Am. Chem. Soc., 2010, 132, 6908–6909 CrossRef CAS PubMed;
(d) Y. Su, M. Zhao, K. Han, G. Song and X. Li, Synthesis of 2-pyridones and iminoesters via Rh(III)-catalyzed oxidative coupling between acrylamides and alkynes, Org. Lett., 2010, 12, 5462–5465 CrossRef CAS PubMed;
(e) X. Xu, Y. Liu and C.-M. Park, Rhodium(III)-catalyzed intramolecular annulation through C-H activation: total synthesis of (±)-antofine, (±)-septicine, (±)-tylophorine, and rosettacin, Angew. Chem., Int. Ed., 2012, 51, 9372–9376 CrossRef CAS PubMed;
(f) N. Quiñones, A. Seoane, R. G. Fandiño, J. L. Mascareñas and M. Gulías, Rhodium(III)-catalyzed intramolecular annulations involving amide-directed C–H activations: synthetic scope and mechanistic studies, Chem. Sci., 2013, 4, 2874–2879 RSC;
(g) X. Wu, B. Wang, Y. Zhou and H. Liu, Propargyl Alcohols as One-Carbon Synthons: Redox-Neutral Rhodium(III)-Catalyzed C-H Bond Activation for the Synthesis of Isoindolinones Bearing a Quaternary Carbon, Org. Lett., 2017, 19, 1294–1297 CrossRef CAS PubMed;
(h) X. Wu, B. Wang, S. Zhou, Y. Zhou and H. Liu, Ruthenium-Catalyzed Redox-Neutral [4 + 1] Annulation of Benzamides and Propargyl Alcohols via C–H Bond Activation, ACS Catal., 2017, 4, 2494–2499 CrossRef;
(i) L. Grigorjeva and O. Daugulis, Cobalt-catalyzed, aminoquinoline-directed C(sp2)-H bond alkenylation by alkynes, Angew. Chem., Int. Ed., 2014, 53, 10209–10212 CrossRef CAS PubMed;
(j) A. Lerchen, T. Knecht, M. Koy, C. G. Daniliuc and F. Glorius, A General Cp*CoIII-Catalyzed Intramolecular C−H Activation Approach for the Efficient Total Syntheses of Aromathecin, Protoberberine, and Tylophora Alkaloids, Chem. – Eur. J., 2017, 23, 12149–12152 CrossRef CAS PubMed;
(k) X. Wu and H. Ji, Rhodium-Catalyzed [4 + 1] Cyclization via C-H Activation for the Synthesis of Divergent Heterocycles Bearing a Quaternary Carbon, J. Org. Chem., 2018, 83, 4650–4656 CrossRef CAS PubMed;
(l) X. Wu, P. Li, Y. Lu, J. Qiao, J. Zhao, X. Jia, H. Ni, L. Kong, X. Zhang and F. Zhao, Rhodium-Catalyzed Cascade Reactions of Indoles with 4-Hydroxy-2-Alkynoates for the Synthesis of Indole-Fused Polyheterocycles, Adv. Synth. Catal., 2020, 362, 2953–2960 CrossRef CAS;
(m) Y. Yu, L. Huang, W. Wu and H. Jiang, Palladium-catalyzed oxidative annulation of acrylic acid and amide with alkynes: a practical route to synthesize α-pyrones and pyridines, Org. Lett., 2014, 16, 2146–2149 CrossRef CAS PubMed.
-
(a) H. Meng, H. Xu, Z. Zhou, Z. Tang, Y. Li, Y. Zhou, W. Yi and X. Wu, Recyclable rhodium-catalyzed C-H activation/[4 + 2] annulation with unconventional regioselectivity at ambient temperature: experimental development and mechanistic insight, Green Chem., 2022, 24, 7012–7021 RSC;
(b) T. von Münchow, S. Dana, Y. Xu, B. Yuan and L. Ackermann, Enantioselective electrochemical cobalt-catalyzed aryl C-H activation reactions, Science, 2023, 379, 1036–1042 CrossRef PubMed;
(c) P. Wang, H. Wu, X.-P. Zhang, G. Huang, R. H. Crabtree and X. Li, Sigma-Bond Metathesis as an Unusual Asymmetric Induction Step in Rhodium-Catalyzed Enantiodivergent Synthesis of C-N Axially Chiral Biaryls, J. Am. Chem. Soc., 2023, 145, 8417–8429 CAS;
(d) Y. Chao, L. Shi, F. Wang, Y. Su, J.-B. Xia and F. Li, Rhodium-Catalyzed Asymmetric (3 + 2 + 2) Annulation via N−H/C−H Dual Activation and Internal Alkyne Insertion toward N-Fused 5/7 Bicycles, ACS Catal., 2022, 12, 14194–14208 CrossRef;
(e) T. Kurihara, M. Kojima, T. Yoshino and S. Matsunaga, Cp*Rh(III)/Chiral Lewis Base Cooperative Catalysis for Enantioselective Cyclization via C-H Activation, J. Am. Chem. Soc., 2022, 144, 7058–7065 CrossRef CAS PubMed;
(f) R. K. Shukla, A. M. Nair and C. M. R. Volla, Pd(ii)-catalyzed β- and γ-C-(sp3)-H dienylation with allenyl acetates, Chem. Sci., 2022, 14, 955–962 RSC.
-
(a) C.-Q. Wang, Y. Zhang and C. Feng, Fluorine Effects on Group Migration via a Rhodium(V) Nitrenoid Intermediate, Angew. Chem., Int. Ed., 2017, 56, 14918–14922 CrossRef CAS PubMed;
(b) T. Yamada, Y. Shibata and K. Tanaka, Formal Lossen Rearrangement/Alkenylation or Annulation Cascade of Heterole Carboxamides with Alkynes Catalyzed by CpRhIII Complexes with Pendant Amides, Chem. – Eur. J., 2019, 25, 16022–16031 CrossRef CAS PubMed;
(c) T. Yamada, Y. Shibata, S. Kawauchi, S. Yoshizaki and K. Tanaka, Formal Lossen Rearrangement/[3 + 2] Annulation Cascade Catalyzed by a Modified Cyclopentadienyl RhIII Complex, Chem. – Eur. J., 2018, 24, 5723–5727 CrossRef CAS PubMed;
(d) M. Bian, H. Mawjuda, H. Gao, H. Xu, Z. Zhou and W. Yi, Lossen Rearrangement vs C-N Reductive Elimination Enabled by Rh(III)-Catalyzed C-H Activation/Selective Lactone Ring-Opening: Chemodivergent Synthesis of Quinolinones and Dihydroisoquinolinones, Org. Lett., 2020, 22, 9677–9682 CrossRef CAS PubMed;
(e) J.-F. Tan, C. T. Bormann, K. Severin and N. Cramer, Alkynyl Triazenes as Fluoroalkyne Surrogates: Regioselective Access to 4-Fluoro-2-pyridones by a Rh(III)-Catalyzed C–H Activation–Lossen Rearrangement–Wallach Reaction, ACS Catal., 2020, 10, 3790–3796 CrossRef CAS;
(f) M. Peng, C.-S. Wang, P.-P. Chen, T. Roisnel, H. Doucet, K. N. Houk and J. F. Soulé, Merging C-H Bond Activation, Alkyne Insertion, and Rearrangements by Rh(III)-Catalysis: Oxindole Synthesis from Nitroarenes and Alkynes, J. Am. Chem. Soc., 2023, 145, 4508–4516 CrossRef CAS PubMed;
(g) Y. Li, H. Xu, L. Huang, Z. Zhou, Z. Tang, H. Meng, W. Zhang, W. Yi and X. Wu, Rhodium-catalyzed Regioselective C-H Activation/Lossen Rearrangement/Annulation for the Green Synthesis of Trisubstituted 2-Pyridones, Org. Chem. Front., 2023, 10, 3000–3009 RSC;
(h) R. B. Dateer and S. Chang, Selective Cyclization of Arylnitrones to Indolines under External Oxidant-Free Conditions: Dual Role of Rh(III) Catalyst in the C-H Activation and Oxygen Atom Transfer, J. Am. Chem. Soc., 2015, 137, 4908–4911 CrossRef CAS PubMed;
(i) J.-L. Pan, P. Xie, C. Chen, Y. Hao, C. Liu, H.-Y. Bai, J. Ding, L.-R. Wang, Y. Xia and S.-Y. Zhang, Rhodium(III)-Catalyzed Redox-Neutral Cascade [3 + 2] Annulation of N-Phenoxyacetamides with Propiolates via C-H Functionalization/Isomerization/Lactonization, Org. Lett., 2018, 20, 7131–7136 CrossRef CAS PubMed.
-
(a) Y. Wu, C. Pi, Y. Wu and X. Cui, Directing group migration strategy in transition-metal-catalysed direct C-H functionalization, Chem. Soc. Rev., 2021, 50, 3677–3689 RSC;
(b) X. Zhou, Z. Fan, Z. Zhang, P. Lu and Y. Wang, Construction of Pyrrolo[1,2-a]indoles via Cobalt(III)-Catalyzed Enaminylation of 1-(Pyrimidin-2-yl)-1H-indoles with Ketenimines and Subsequent Base-Promoted Cyclization, Org. Lett., 2016, 18, 4706–4709 CrossRef CAS PubMed;
(c) S.-Y. Chen, X.-L. Han, J.-Q. Wu, Q. Li, Y. Chen and H. Wang, Manganese(I)-Catalyzed Regio- and Stereoselective 1,2-Diheteroarylation of Allenes: Combination of C−H Activation and Smiles Rearrangement, Angew. Chem., Int. Ed., 2017, 56, 9939–9943 CrossRef CAS PubMed;
(d) C. Wang, A. Wang and M. Rueping, Manganese Catalyzed C−H Functionalizations: Hydroarylations and Alkenylations Involving an Unexpected Heteroaryl Shift, Angew. Chem., Int. Ed., 2017, 56, 9935–9938 CrossRef CAS PubMed;
(e) C. Zhu, R. Kuniyil, B. B. Jei and L. Ackermann, Domino C−H Activation/Directing Group Migration/Alkyne Annulation: Unique Selectivity by d6-Cobalt(III) Catalysts, ACS Catal., 2020, 10, 4444–4450 CrossRef CAS;
(f) X. Wang, T. Gensch, A. Lerchen, C. G. Daniliuc and F. Glorius, Cp*Rh(III)/Bicyclic Olefin Cocatalyzed C−H Bond Amidation by Intramolecular Amide Transfer, J. Am. Chem. Soc., 2017, 139, 6506–6512 CrossRef CAS PubMed;
(g) Y. Wu, Z. Chen, Y. Yang, W. Zhu and B. Zhou, Rh(III)-Catalyzed Redox Neutral Unsymmetrical C−H Alkylation and Amidation Reactions of N-Phenoxyacetamides, J. Am. Chem. Soc., 2018, 140, 42–45 CrossRef CAS PubMed.
- B. Ma, P. Wu, X. Wang, Z. Wang, H.-X. Lin and H.-X. Dai, Efficient Synthesis of Spirooxindole Pyrrolones by a Rhodium(III)-Catalyzed C-H Activation/Carbene Insertion/Lossen Rearrangement Sequence, Angew. Chem., Int. Ed., 2019, 58, 13335–13339 CrossRef CAS PubMed.
- F. Zhao, Z. Zhou, Y. Lu, J. Qiao, X. Zhang, X. Gong, S. Liu, S. Lin, X. Wu and W. Yi, Chemo, Regio, and Stereoselective Assembly of Polysubstituted Furan-2(5H) ones Enabled by Rh(III)-Catalyzed Domino C-H Alkenylation/Directing Group Migration/Lactonization: A Combined Experimental and Computational Study, ACS Catal., 2021, 11, 13921–13934 CrossRef CAS.
- A review on cascade reactions: A. Baccalini, G. Faita, G. Zanoni and D. Maiti, Transition Metal Promoted Cascade Heterocycle Synthesis through C-H Functionalization, Chem. – Eur. J., 2020, 26, 9749–9783 CrossRef CAS PubMed.
- A review on cascade reactions: L. Song and E. V. Van der Eycken, Transition Metal-Catalyzed Intermolecular Cascade C-H Activation/Annulation Processes for the Synthesis of Polycycles, Chem. – Eur. J., 2021, 27, 121–144 CrossRef CAS PubMed.
- K. K. Gollapelli, S. Kallepu, N. Govindappa, J. B. Nanubolu and R. Chegondi, Carbonyl-assisted reverse regioselective cascade annulation of 2-acetylenic ketones triggered by Ru-catalyzed C-H activation, Chem. Sci., 2016, 7, 4748–4753 RSC.
- Y. Fukui, P. Liu, Q. Liu, Z.-T. He, N.-Y. Wu, P. Tian and G.-Q. Lin, Tunable arylative cyclization of 1,6-enynes triggered by rhodium(III)-catalyzed C-H activation, J. Am. Chem. Soc., 2014, 136, 15607–15614 CrossRef CAS PubMed.
-
(a) G. Liao, H. Song, X. Yin and B. Shi, Expeditious synthesis of pyrano[2,3,4-de]quinolines via Rh(iii)-catalyzed cascade C-H activation/annulation/lactonization of quinolin-4-ol with alkynes, Chem. Commun., 2017, 53, 7824–7827 RSC;
(b) Y. Xu, B. Li, X. Zhang and X. Fan, One-Pot Synthesis of Fused N,O-Heterocycles through Rh(III)-Catalyzed Cascade Reactions of Aromatic/Vinylic N-Alkoxy-Amides with 4-Hydroxy-2-Alkynoates, Adv. Synth. Catal., 2018, 360, 2613–2620 CrossRef CAS;
(c) A. Kumar, V. Hanchate and K. R. Prabhu, Rhodium(III)-Catalyzed Cascade Reactions of Imines/Imidates with 4-Hydroxy-2-alkynoates to Synthesize Regioselective Furanone-Fused Isoquinoline Scaffolds, J. Org. Chem., 2021, 86, 17965–17974 CrossRef CAS PubMed;
(d) Y.-Y. Wang, M. Liu and L. Dong, Rh(III)-Catalyzed multi-site-selective C–H bond functionalization: condition-controlled synthesis of diverse fused polycyclic benzimidazole derivatives, Org. Chem. Front., 2021, 8, 2487–2493 RSC;
(e) N. Muniraj, A. Kumar and K. R. Prabhu, Cobalt-Catalyzed Regioselective [4 + 2] Annulation/Lactonization of Benzamides with 4-Hydroxy-2-Alkynoates under Aerobic Conditions, Adv. Synth. Catal., 2020, 362, 152–159 CrossRef CAS;
(f) A. Kumar and K. R. Prabhu, Rhodium(III)-Catalyzed C-H Activation: A Cascade Approach for the Regioselective Synthesis of Fused Heterocyclic Lactone Scaffolds, J. Org. Chem., 2020, 85, 3548–3559 CrossRef CAS PubMed;
(g) Y. Liu, Z. Yang, R. Chauvin, W. Fu, Z. Yao, L. Wang and X. Cui, One-Pot Synthesis of Furo[3,4-c]indolo[2,1-a]isoquinolines through Rh(III)-Catalyzed Cascade Reactions of 2-Phenylindoles with 4-Hydroxy-2-alkynoates, Org. Lett., 2020, 22, 5140–5144 CrossRef CAS PubMed.
Footnotes |
† Electronic supplementary information (ESI) available. CCDC 2281784. For ESI and crystallographic data in CIF or other electronic format see DOI: https://doi.org/10.1039/d3qo00909b |
‡ These authors contributed equally. |
|
This journal is © the Partner Organisations 2023 |
Click here to see how this site uses Cookies. View our privacy policy here.