DOI:
10.1039/D3QO00322A
(Research Article)
Org. Chem. Front., 2023,
10, 3460-3466
Synthesis of atropisomeric phosphino-triazoles and their corresponding gold(I) complexes†
Received
1st March 2023
, Accepted 17th April 2023
First published on 18th April 2023
Abstract
The synthesis of atropisomeric phoshino-triazoles is disclosed. It was found that the introduction of a phosphine functionality onto the 5-position of a 1,2,3-triazole ring could be highly restrictive towards the rotation around a triazole-aryl bond. VT NMR and chiral HPLC studies demonstrated that rotation was restricted even at high temperatures. Gold(I) chloride complexes of single-enantiomer phosphines were prepared and again demonstrated to be conformationally stable.
Atropisomerism is one of the fundamental families of chirality, where rotational restriction around a single bond in a molecule creates two non-superimposable mirror images.1–3 Atropisomerism is present in many compounds with several natural products, ligands and organocatalysts incorporating restricted rotation.4–6 Phosphine ligands for transition metals that rely on atropisomerism to engender chirality into products are well established, with compounds such as BINAP and the phosphoramidite family commonly being employed in a variety of asymmetric catalytic applications (Fig. 1a).7–9 In general heterocyclic-containing phosphines have seen a wide variety of application and new scaffolds in this space have great value.10
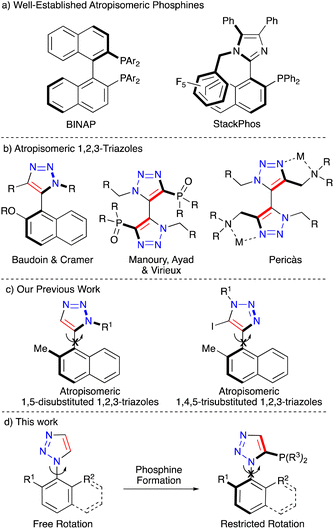 |
| Fig. 1 (a) Atropisomeric phosphines commonly employed in asymmetric catalysis (b) atropisomeric 1,2,3-triazoles (c) our previous work on the synthesis of atropisomeric 1,2,3-triazoles (d) this work on the synthesis of atropisomeric 1,2,3-triazole phosphines. | |
Whilst atropisomeric metal ligands are common, most are built around a 1,1′-bi-2-naphthyl scaffold.11–14 Ligands built around the 1,2,3-triazole core are in comparison extremely sparse. Reports in this area are usually centred around a 5–5′ bistriazole architecture15–17 apart from a small number of notable exceptions (Fig. 1b).18,19 In extension atropisomeric 1,2,3-triazole-phosphines to the best of our knowledge have not been previously reported and there appears to be only limited reports of atropisomeric 1,2,4-triazole-phosphines.20 This is particularly intriguing as accessing 1,2,3-triazoles can be readily achieved through the ubiquitous copper-catalysed azide–alkyne cycloaddition (CuAAC) which is part of the family of commonly employed “click” reactions.14,21–23 Triazoles have been widely employed in biorthogonal conjugation, which in part led to the awarding of the 2022 Nobel Prize in Chemistry to Meldal, Sharpless and Bertozzi for their pioneering work in the field.24–26 The CuAAC is well known as a reaction that can be carried out under mild conditions with exquisite regioselectivity and up to quantitative yields.27–29
We have an ongoing interest into the utility of the CuAAC reaction and how it can employed to generate chiral molecules. Previously we have reported on the generation of point chiral and axially chiral triazoles as well as utilising triazoles in a range of linkage applications.30–34 Recently we have reported on the synthesis of a range of phosphine-appended triazoles35,36 and on the establishment of how bi-aryl 1,2,3-triazoles can be rendered atropisomeric (Fig. 1c).37 Therefore we wished to expand on these concepts and investigate if we could readily access atropisomeric and point chiral triazole-containing phosphines utilising the CuAAC reaction and in turn generate their corresponding gold complexes (Fig. 1d).
To begin, we took inspiration from our previous studies on the 2-methyl-1-naphthyl moiety which can be incorporated into atropisomeric triazoles which are stable up to high temperatures.37 The triazole 2 was successfully synthesised in two steps from the commercially available 2-methyl-1-naphthylamine. Phosphine incorporation was then conducted using a lithiation followed by trapping with a range of chlorophosphines. This protocol led to the successful isolation of compounds 3a–c incorporating tBu, Ph and iPr groups around the phosphorus in 65%, 65% and 42% yields, respectively (Fig. 2a). Immediately it was noticed in the 1H NMR spectrum of 3a that the resonances corresponding to the tBu protons were diastereotopic. This was encouraging as it suggested the bi-aryl bond was no longer freely rotating. To confirm the stability of the observed atropisomers, a VT NMR study was undertaken (see ESI†). In toluene-d8 at 105 °C no coalescence of resonances was observed, pointing to a highly rotationally restricted compound. In addition, a single-crystal X-ray structure was determined with both enantiomers of 3a being clearly visible in the unit cell (Fig. 2b).
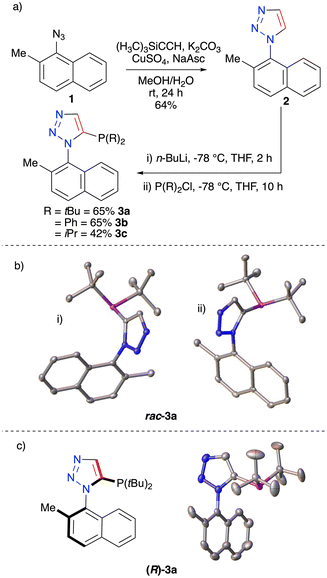 |
| Fig. 2 (a) Synthesis of 1,2,3-triazole phosphines (b) molecules from the crystal structure of rac-3a with ellipsoids drawn at the 50% probability level. Symmetry codes used to generate the equivalent atoms: (i) x, y, z. (ii) 1 − x, 1 − y, 1 − z. Hydrogen atoms omitted for clarity (c) single-crystal X-ray diffraction structure of (R)-3a. Ellipsoids are drawn at the 50% probability level. Hydrogen atoms omitted for clarity. | |
Compound 3b, in which two phenyl groups are attached to the phosphorus was also successfully crystallised and again two enantiomers were visible within the unit cell (Fig. 3c). The isopropyl-substituted compound 3c was observed to display characteristics of atropisomerism with diastereotopic protons being clearly visible in the 1H NMR spectrum. In all, this series of compounds was highly encouraging as all substitutions around the phosphorous appeared to significantly restrict the rotational freedom of the molecules.
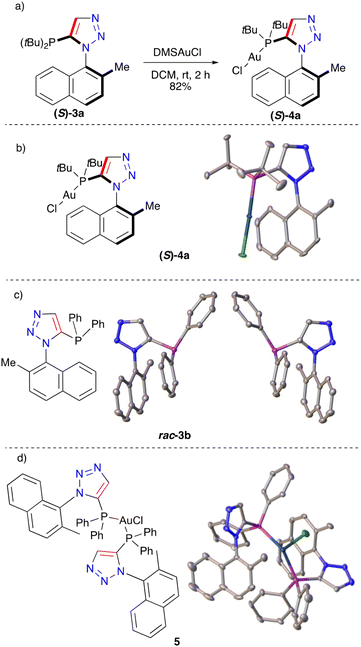 |
| Fig. 3 (a) Synthesis of a single enantiomer gold(I) chloride complex (b) single-crystal X-ray diffraction structure of (S)-4a. Ellipsoids are drawn at the 50% probability level. A molecule of DCM has been omitted for clarity. Hydrogen atoms omitted for clarity. (c) Molecules from the crystal structure of rac-3b with ellipsoids drawn at the 50% probability level. Symmetry codes used to generate the equivalent atoms: (i) x, y, z. (ii) 1 − x, 1 − y, 1 − z. Hydrogen atoms omitted for clarity. (d) Single-crystal X-ray diffraction structure of homo-chiral complex 5. Ellipsoids are drawn at the 50% probability level. A molecule of DCM has been omitted for clarity. Hydrogen atoms have been omitted for clarity. | |
The next step was to obtain single-enantiomer compounds. Initially a Staudinger reaction was trialled to resolve the two enantiomers. Unfortunately, this was unsuccessful and thus led us to pursue chiral-prep HPLC to separate and isolate the single-enantiomer triazoles. Separations for both compounds (R)-3a and (S)-3a were successful (see ESI†) and the single enantiomers of 3a were obtained in sufficient quantities for characterisation and metal complexation.
X-ray crystallographic studies of (R)-3a were conducted and the conformation around the restricted bi-aryl bond was determined (Fig. 2c). This pure material (S)-3a was complexed with DMSAuCl, leading to the formation of (S)-4a in 82% and a corresponding crystal structure obtained (Fig. 3a and b). This structure demonstrated no racemisation had occurred during complexation and that a single-enantiomer complex had been successfully prepared. The phenyl substituted compound 3b in its racemic form was trialled in a gold complexation and the homo-chiral complex 5 was obtained (Fig. 3c and d). We also attempted to use chiral-prep HPLC to obtain a single-enantiomer sample of 3c, however after many attempts baseline resolution was not achieved. This series of compounds clearly demonstrated that the CuAAC reaction is a powerful tool to access atropisomerically stable phosphines and gold complexes.
Next, we wished to explore the ability to generate P-chirogenic triazole phosphines. This class of ligand was most famously used by Knowles in his seminal synthesis of L-DOPA using asymmetric hydrogenation.38,39 However, P-chirogenic phosphines have been underutilised mainly due to their synthetic routes relying on phosphine oxide intermediates. We believed that through trapping a lithiated triazole with a chlorophosphine with two different substituents, an atropisomeric P-chirogenic ligand could be prepared. We have previously disclosed the preparation of triazole 6 which was utilised to prepare a range of bulky triazole phosphines. Taking 6 and treating it with n-BuLi followed by PPh(tBu)Cl gave the triazole rac-7 in 74% yield (Fig. 4a). The 1H NMR spectrum of this compound clearly showed diastereotopic protons with the OMe groups giving two clearly defined singlets of integration 3. Preparative chiral HPLC was then employed to resolve the enantiomers of 7. Both enantiomers were successfully isolated (see ESI†) and the corresponding gold chloride complex (S)-8 was prepared in 94% yield. Single-crystal X-ray crystallography was then used to unambiguously determine the chirality of the gold complex (S)-8 (Fig. 4b). In addition a racemic crystal structure of 8 was also determined (see ESI†).
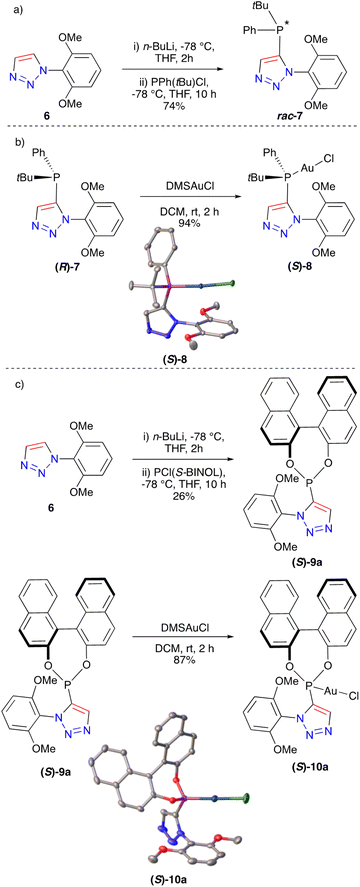 |
| Fig. 4 (a) Synthesis of 1,2,3-triazolephosphine rac-7 (b) synthesis and single-crystal X-ray diffraction structure of gold(I) chloride complex (S)-8. Ellipsoids are drawn at the 50% probability level (c) Synthesis of 1,2,3-triazole phosphonite (S)-9a and subsequent complexation. Single-crystal X-ray diffraction structure of (S)-10a. Ellipsoids are drawn at the 50% probability level. | |
Finally, we wished to expand our study to investigate the synthesis of a new class of ligand incorporating the phosphonite functional group in combination with a triazole. It was decided that quenching the litathiated triazole 6 with PCl((S)-BINOL) would give a highly unusual species which incorporated both BINOL and 1,2,3-triazole units. Using this approach phosphonite triazole (S)-9a was successfully prepared in 26% yield (Fig. 4c). Taking this triazole and treating it with DMSAuCl furnished the gold complex (S)-10a with the stereochemical configuration confirmed through single-crystal X-ray analysis (Fig. 4c).
Interestingly whilst conducting the same reaction sequence with the iPr substituted triazole 11, there was no observed formation of the desired phosphonite (S)-9b; instead, a solid by-product was formed. Single-crystal X-ray analysis led to the identification of the multi-aryl species 12 (Fig. 5). Whilst this was not the desired outcome this structure was highly unusual both with multiple phosphorus atoms and multiple stereodefined bi-aryls. This compound could therefore be of use in applications where highly sterically hindered phosphorus ligands are required.
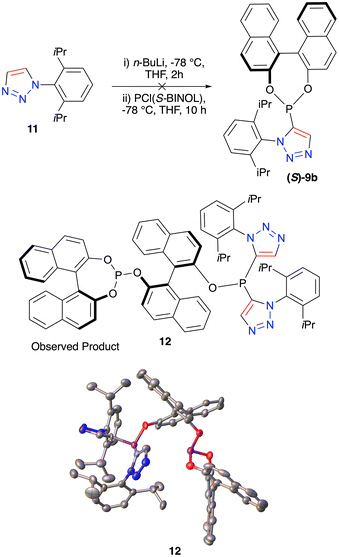 |
| Fig. 5 Attempted synthesis of phosphonite (S)-9b and subsequent acquisition of the single-crystal X-ray diffraction structure 12. Ellipsoids are drawn at the 50% probability level. Hydrogen atoms are omitted for clarity. | |
We and others have previously discussed the importance of various parameters including steric effects of bulky phosphines relating to suitability and efficacy in catalysis (primarily as ligands for metals in metal-mediated catalysis).35,36 The use of the Tolman cone angle has been an effective descriptor of ligand bulkiness for many years,40 more recently Nolan's percentage buried volume parameter (%Vbur) has been widely applied to various ligand types (eg NHC's) to describe and correlate ligand/catalyst activity to the environment surrounding the metal catalyst. The use of %Vbur has undoubtedly been accelerated through the use of the simple free web tool SambVca developed by Cavallo and co-workers.41 So we set about determining some steric parameters of our novel Au phosphines using this tool.42–44 Utilising the X-ray crystal structure CIF file data obtained for complexes 4a, 5, 8 and 10, we were able to gain the %Vbur data utilising the Au–P bond lengths observed (Fig. 6). Interestingly, the dimer complex 5 has a very significant buried volume surrounding the Au atom (77.5% Vbur).
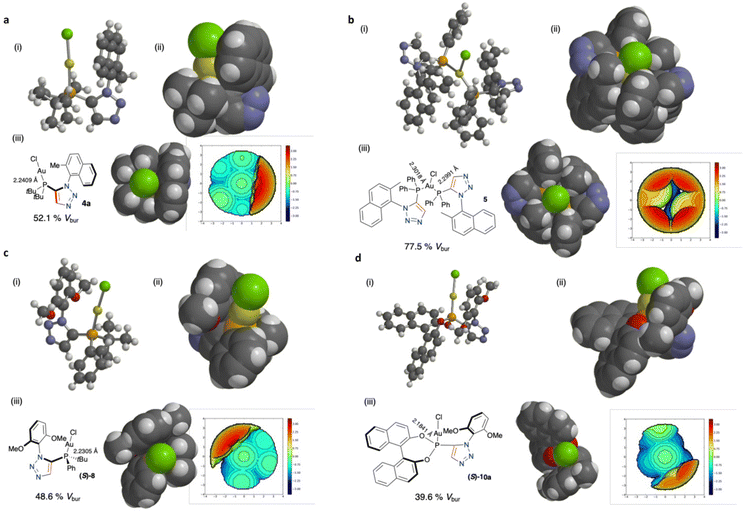 |
| Fig. 6 Single-crystal X-ray diffraction structures of (a) 4a, (b) 5, (c) 8 and (d) 10: (i) Ortep representation, ellipsoid probability 50% (rendered in Spartan). (ii) Space-filling representation. (iii) Percentage buried volume determined from the crystal structures steric map of ligand depicted (right). | |
Conclusions
Overall, we have been able to demonstrate that the formation of triazole-phosphines is a viable option for the generation of stable atropisomeric species. This is the first time to the best of our knowledge that a post CuAAC modification to a triazole ring has been utilised to engender atropisomerism. This approach offers added flexibility in being able to readily change the other groups around the phosphorous atom. We were also able to successfully access new classes of P-chirogenic phosphines and phosphonites. Preparative chiral HPLC was used to access enantiomerically pure samples of all of these species. These ligand architectures in general were readily amenable to the formation of gold(I) chloride complexes. We believe that the strategies outlined in chiral ligand design here may have an impact in catalysis and in particular gold- and palladium-mediated reactions.
Author contributions
All authors contributed in varying degrees to planning the experiments, evaluating results and writing of the manuscript; specific contributions in addition to this are listed below: J. S. F. led and co-conceived the overarching project, providing critical assessment of data, directed most aspects, and supervised the experimental work; B. R. B. helped direct aspects of the research and gave input and critical assessment throughout the progress of the project; Y. Z., F. M., L. M. and W. D. G. B co-conceived aspects of the project, conducted the synthesis and drafted a proportion of the ESI,† offered critical suggestions and conducted the XRD data collection and analysis herein, along with L. M. C. S. L. D. conducted and advised on the VT NMR experiments. W. D. G. B. and B. R. B. wrote the manuscript.
Conflicts of interest
There are no conflicts to declare.
Acknowledgements
The authors would like to thank the University of Birmingham, Durham University and Loughborough University for support. The CASE consortium is thanked for networking opportunities.45,46
References
- J. K. Cheng, S.-H. Xiang, S. Li, L. Ye and B. Tan, Recent Advances in Catalytic Asymmetric Construction of Atropisomers, Chem. Rev., 2021, 121, 4805–4902 CrossRef CAS.
- M. Basilaia, M. H. Chen, J. Secka and J. L. Gustafson, Atropisomerism in the Pharmaceutically Relevant Realm, Acc. Chem. Res., 2022, 55, 2904–2919 CrossRef CAS.
- G.-J. Mei, W. L. Koay, C.-Y. Guan and Y. Lu, Atropisomers beyond the C–C axial chirality: Advances in catalytic asymmetric synthesis, Chem, 2022, 8, 1855–1893 CAS.
- J. E. Smyth, N. M. Butler and P. A. Keller, A twist of nature – the significance of atropisomers in biological systems, Nat. Prod. Rep., 2015, 32, 1562–1583 RSC.
- Z. Xu and L.-W. Xu, Development of Ar-BINMOL-Derived Atropisomeric Ligands with Matched Axial and sp3 Central Chirality for Catalytic Asymmetric Transformations, Chem. Rec., 2015, 15, 925–948 CrossRef CAS PubMed.
- S. Brandes, B. Niess, M. Bella, A. Prieto, J. Overgaard and K. A. Jørgensen, Non-Biaryl Atropisomers in Organocatalysis, Chem. – Eur. J., 2006, 12, 6039–6052 CrossRef CAS.
- M. Berthod, G. Mignani, G. Woodward and M. Lemaire, Modified BINAP: The How and the Why, Chem. Rev., 2005, 105, 1801–1836 CrossRef CAS PubMed.
- T. H. Wöste and M. Oestreich, BINAP versus BINAP(O) in Asymmetric Intermolecular Mizoroki–Heck Reactions: Substantial Effects on Selectivities, Chem. – Eur. J., 2011, 17, 11914–11918 CrossRef PubMed.
- J. F. Teichert and B. L. Feringa, Phosphoramidites: Privileged Ligands in Asymmetric Catalysis, Angew. Chem., Int. Ed., 2010, 49, 2486–2528 CrossRef CAS PubMed.
- M. H. Tse, P. Y. Choy and F. Y. Kwong, Facile Assembly of Modular-Type Phosphines for Tackling Modern Arylation Processes, Acc. Chem. Res., 2022, 55, 3688–3705 CrossRef CAS PubMed.
- M. P. Walsh, J. M. Phelps, M. E. Lennon, D. S. Yufit and M. O. Kitching, Enantioselective synthesis of ammonium cations, Nature, 2021, 597, 70–76 CrossRef CAS PubMed.
- D. Parmar, E. Sugiono, S. Raja and M. Rueping, Complete Field Guide to Asymmetric BINOL-Phosphate Derived Brønsted Acid and Metal Catalysis: History and Classification by Mode of Activation; Brønsted Acidity, Hydrogen Bonding, Ion Pairing, and Metal Phosphates, Chem. Rev., 2014, 114, 9047–9153 CrossRef CAS PubMed.
- J. M. Brunel, BINOL: A Versatile Chiral Reagent, Chem. Rev., 2005, 105, 857–898 CrossRef CAS PubMed.
- F. Yu, Y. Chen, H. Jiang and X. Wang, Recent advances of BINOL-based sensors for enantioselective fluorescence recognition, Analyst, 2020, 145, 6769–6812 RSC.
- P. Etayo, E. C. Escudero-Adán and M. A. Pericàs, 5,5′-Bistriazoles as axially chiral, multidentate ligands: synthesis, configurational stability and catalytic application of their scandium(III) complexes, Catal. Sci. Technol., 2017, 7, 4830–4841 RSC.
- N. Sevrain, J.-N. Volle, J.-L. Pirat, T. Ayad and D. Virieux, 1,1′-Dibenzyl-bis-(triazolyl)diphenylphosphine dioxide: a new efficient organocatalyst for silicon tetrachloride-mediated enantioselective Abramov-type phosphonylation of aldehydes with trialkyl phosphites, RSC Adv., 2017, 7, 52101–52104 RSC.
- C. Laborde, M.-M. Wei, A. van der Lee, E. Deydier, J.-C. Daran, J.-N. Volle, R. Poli, J.-L. Pirat, E. Manoury and D. Virieux, Double [3+2]-dimerisation cascade synthesis of bis(triazolyl)bisphosphanes, a new scaffold for bidentate bisphosphanes, Dalton Trans., 2015, 44, 12539–12545 RSC.
- Q.-H. Nguyen, S.-M. Guo, T. Royal, O. Baudoin and N. Cramer, Intermolecular Palladium(0)-Catalyzed Atropo-enantioselective C–H Arylation of Heteroarenes, J. Am. Chem. Soc., 2020, 142, 2161–2167 CrossRef CAS.
- R. Vroemans, S. R. Ribone, J. Thomas, L. Van Meervelt, T. Ollevier and W. Dehaen, Synthesis of homochiral sulfanyl- and sulfoxide-substituted naphthyltriazoles and study of the conformational stability, Org. Biomol. Chem., 2021, 19, 6521–6526 RSC.
- A. A. Kirilchuk, A. A. Yurchenko, Y. G. Vlasenko, A. N. Kostyuk and A. B. Rozhenko, Synthesis and Structure of Phosphanylated Bis-Triazoles as Potential Ligands for Chiral Catalysts, Chem. Heterocycl. Compd., 2015, 50, 1559–1566 CrossRef CAS.
- N. K. Devaraj and M. G. Finn, Introduction: Click Chemistry, Chem. Rev., 2021, 121, 6697–6698 CrossRef CAS.
- H. C. Kolb, M. G. Finn and K. B. Sharpless, Click Chemistry: Diverse Chemical Function from a Few Good Reactions, Angew. Chem., Int. Ed., 2001, 40, 2004–2021 CrossRef CAS.
- S. L. Scinto, D. A. Bilodeau, R. Hincapie, W. Lee, S. S. Nguyen, M. Xu, C. W. am Ende, M. G. Finn, K. Lang, Q. Lin, J. P. Pezacki, J. A. Prescher, M. S. Robillard and J. M. Fox, Bioorthogonal chemistry, Nat. Rev. Methods Primers, 2021, 1, 30 CrossRef CAS PubMed.
- V. V. Rostovtsev, L. G. Green, V. V. Fokin and K. B. Sharpless, A Stepwise Huisgen Cycloaddition Process: Copper(I)-Catalyzed Regioselective “Ligation” of Azides and Terminal Alkynes, Angew. Chem., Int. Ed., 2002, 41, 2596–2599 CrossRef CAS PubMed.
- C. W. Tornøe, C. Christensen and M. Meldal, Peptidotriazoles on Solid Phase: [1,2,3]-Triazoles by Regiospecific Copper(I)-Catalyzed 1,3-Dipolar Cycloadditions of Terminal Alkynes to Azides, J. Org. Chem., 2002, 67, 3057–3064 CrossRef PubMed.
- E. M. Sletten and C. R. Bertozzi, Bioorthogonal Chemistry: Fishing for Selectivity in a Sea of Functionality, Angew. Chem., Int. Ed., 2009, 48, 6974–6998 CrossRef CAS PubMed.
- J. E. Hein and V. V. Fokin, Copper-catalyzed azide–alkyne cycloaddition (CuAAC) and beyond: new reactivity of copper(I) acetylides, Chem. Soc. Rev., 2010, 39, 1302–1315 RSC.
- M. Meldal and C. W. Tornøe, Cu-Catalyzed Azide−Alkyne Cycloaddition, Chem. Rev., 2008, 108, 2952–3015 CrossRef CAS PubMed.
- V. Hong, S. I. Presolski, C. Ma and M. G. Finn, Analysis and Optimization of Copper-Catalyzed Azide–Alkyne Cycloaddition for Bioconjugation, Angew. Chem., Int. Ed., 2009, 48, 9879–9883 CrossRef CAS PubMed.
- W. D. G. Brittain, B. R. Buckley and J. S. Fossey, Kinetic resolution of alkyne-substituted quaternary oxindoles via copper catalysed azide–alkyne cycloadditions, Chem. Commun., 2015, 51, 17217–17220 RSC.
- W. D. G. Brittain, B. M. Chapin, W. Zhai, V. M. Lynch, B. R. Buckley, E. V. Anslyn and J. S. Fossey, The Bull–James assembly as a chiral auxiliary and shift reagent in kinetic resolution of alkyne amines by the CuAAC reaction, Org. Biomol. Chem., 2016, 14, 10778–10782 RSC.
- W. D. G. Brittain, A. G. Dalling, Z. Sun, C. S. L. Duff, L. Male, B. R. Buckley and J. S. Fossey, Coetaneous catalytic kinetic resolution of alkynes and azides through asymmetric triazole formation, Sci. Rep., 2019, 9, 15086 CrossRef.
- W. Zhai, L. Male and J. S. Fossey, Glucose selective bis-boronic acid click-fluor, Chem. Commun., 2017, 53, 2218–2221 RSC.
- W. Zhai, B. M. Chapin, A. Yoshizawa, H.-C. Wang, S. A. Hodge, T. D. James, E. V. Anslyn and J. S. Fossey, “Click-fluors”: triazole-linked saccharide sensors, Org. Chem. Front., 2016, 3, 918–928 RSC.
- Y. Zhao, M. G. Wakeling, F. Meloni, T. J. Sum, H. van Nguyen, B. R. Buckley, P. W. Davies and J. S. Fossey, Balancing Bulkiness in Gold(I) Phosphino-triazole Catalysis, Eur. J. Org. Chem., 2019, 5540–5548 CrossRef PubMed.
- Y. Zhao, H. van Nguyen, L. Male, P. Craven, B. R. Buckley and J. S. Fossey, Phosphino-Triazole Ligands for Palladium-Catalyzed Cross-Coupling, Organometallics, 2018, 37, 4224–4241 CrossRef CAS PubMed.
- F. Meloni, W. D. G. Brittain, L. Male, C. S. Le Duff, B. R. Buckley, A. G. Leach and J. S. Fossey, Enantiomer stability of atropisomeric 1,5-disubstituted 1,2,3-triazoles, Tetrahedron Chem, 2022, 1, 100004 CrossRef.
- W. S. Knowles, Application of organometallic catalysis to the commercial production of L-DOPA, J. Chem. Educ., 1986, 63, 222 CrossRef CAS.
- W. S. Knowles and R. Noyori, Pioneering Perspectives on Asymmetric Hydrogenation, Acc. Chem. Res., 2007, 40, 1238–1239 CrossRef CAS PubMed.
- C. A. Tolman, Steric effects of phosphorus ligands in organometallic chemistry and homogeneous catalysis, Chem. Rev., 1977, 77, 313–348 CrossRef CAS.
- SambVca 2.1 web application, https://www.molnac.unisa.it/OMtools/sambvca2.1/index.html (accessed February 2023).
- A. Poater, B. Cosenza, A. Correa, S. Giudice, F. Ragone, V. Scarano and L. Cavallo, SambVca: A Web Application for the Calculation of the Buried Volume of N-Heterocyclic Carbene Ligands, Eur. J. Inorg. Chem., 2009, 1759–1766 CrossRef CAS.
- L. Falivene, R. Credendino, A. Poater, A. Petta, L. Serra, R. Oliva, V. Scarano and L. Cavallo, SambVca 2. A Web Tool for Analyzing Catalytic Pockets with Topographic Steric Maps, Organometallics, 2016, 35, 2286–2293 CrossRef CAS.
- L. Falivene, Z. Cao, A. Petta, L. Serra, A. Poater, R. Oliva, V. Scarano and L. Cavallo, Towards the online computer-aided design of catalytic pockets, Nat. Chem., 2019, 11, 872–879 CrossRef CAS PubMed.
- D. T. Payne, J. S. Fossey and R. B. P. Elmes, Catalysis and Sensing for our Environment (CASE2015) and the Supramolecular Chemistry Ireland Meeting (SCI 2015): Dublin and Maynooth, Ireland. 8th–11th July, Supramol. Chem., 2016, 28, 921–931 CrossRef CAS.
- J. S. Fossey and W. D. G. Brittain, The CASE 2014 symposium: Catalysis and sensing for our environment, Xiamen 7th–9th November 2014, Org. Chem. Front., 2015, 2, 101–105 RSC.
Footnotes |
† Electronic supplementary information (ESI) available. CCDC 2245022–2245029 and 2245473. For ESI and crystallographic data in CIF or other electronic format see DOI: https://doi.org/10.1039/d3qo00322a |
‡ In memory of John S. Fossey, a superb scientist, mentor and friend who will be sadly missed. |
|
This journal is © the Partner Organisations 2023 |